- 1Research Centre Medicine National Autonomous University of Mexico-Benito Juárez Autonomous University of Oaxaca (UNAM-UABJO), Faculty of Medicine, Benito Juárez Autonomous University of Oaxaca, Oaxaca, Mexico
- 2Biochemistry and Immunology Unit, National Technological of Mexico/ITOaxaca, Oaxaca, Mexico
- 3CONACyT—Faculty of Medicine, Benito Juárez Autonomous University of Oaxaca, Oaxaca, Mexico
- 4Department of Biochemistry, School of Medicine, UNAM, Mexico City, México
- 5School of Medicine, Branch at National Institute of Genomic Medicine, Mexico City, Mexico
It is generally understood that the entry of semen into the female reproductive tract provokes molecular and cellular changes facilitating conception and pregnancy. We show a broader picture of the participation of prostaglandins in the fertilization, implantation and maintenance of the embryo. A large number of cells and molecules are related to signaling networks, which regulate tolerance to implantation and maintenance of the embryo and fetus. In this work, many of those cells and molecules are analyzed. We focus on platelets, polymorphonuclear leukocytes, and group 2 innate lymphoid cells involved in embryo tolerance in order to have a wider view of how prostaglandins participate. The combination of platelets and neutrophil extracellular traps (Nets), uterine innate lymphoid cells (uILC), Treg cells, NK cells, and sex hormones have an important function in immunological tolerance. In both animals and humans, the functions of these cells can be regulated by prostaglandins and soluble factors in seminal plasma to achieve an immunological balance, which maintains fetal-maternal tolerance. Prostaglandins, such as PGI2 and PGE2, play an important role in the suppression of the previously mentioned cells. PGI2 inhibits platelet aggregation, in addition to IL-5 and IL-13 expression in ILC2, and PGE2 inhibits some neutrophil functions, such as chemotaxis and migration processes, leukotriene B4 (LTB4) biosynthesis, ROS production, and the formation of extracellular traps, which could help prevent trophoblast injury and fetal loss. The implications are related to fertility in female when seminal fluid is deposited in the vagina or uterus.
Introduction
Prostaglandins (PGs) belong to a subclass of eicosanoids known as prostanoids, these are comprised of C20 atoms, including a cyclopentane ring. PGs are hormone-like chemical messengers which act as autacoids (1) through prostanoid receptors (G protein-coupled receptors) and their variants or isoforms such as E1-4, DP1-2, FP, TP, and IP (1, 2). The main precursor of eicosanoids is arachidonic acid (AA), this is released by the action of phospholipases A2 (PLA2) and C (PLC) (3), AA is then converted into different metabolites through the COX, LOX, and CYP450 pathways (4). The importance of prostaglandins becomes evident when ovulation and fertilization are affected, e.g., as cyclooxygenase (COX) is inhibited by aspirin or indomethacin (5).
PGs have a significant role in maternal immune tolerance and the conception process. We consider prostaglandins in seminal fluid as key in modulating responses in different types of cells participating in fetal-maternal tolerance.
The balance of the immune response in maintaining fetal-maternal tolerance is due to a complex network of soluble molecules and cells, such as macrophages, and dendric, decidual, and NK cells. In Table 1, cells and biological processes are summarized. Moreover, many molecules are released by these cells and have a fundamental role in the tolerance process. Table 2 summarizes the most important of these.
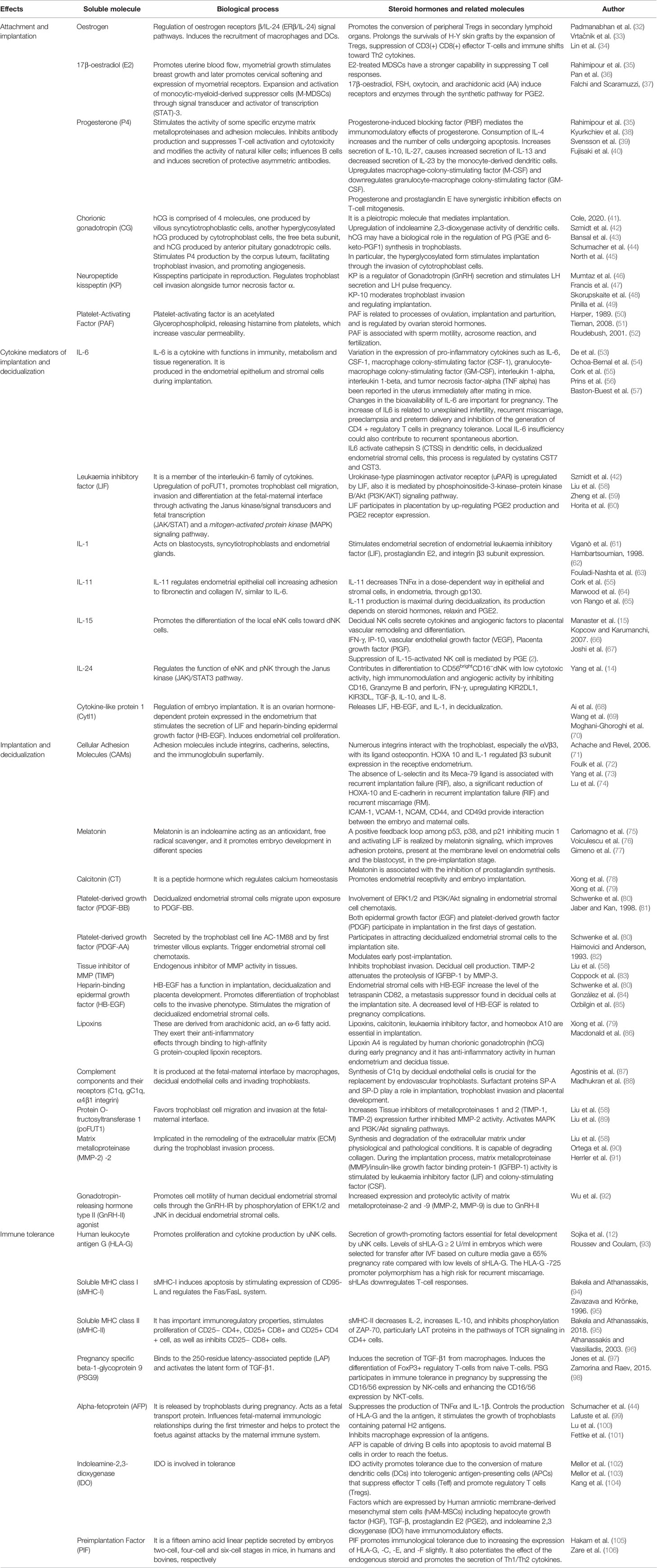
Table 2 Principal soluble molecules acting in implantation (apposition/adhesion/invasion) to maintain fetal-maternal tolerance.
The molecules are released through macro-, micro-, and nanovesicles, including exosomes from placenta cells, syncytiotrophoblasts, denudated syncytiotrophoblasts, and extravillous trophoblasts. All are part of the complex intercommunication between the foetus and the mother. These vesicles transport immunomodulatory proteins such as Fas ligand, TRAIL, CD274, CD276, HLA-G5, Syncytin-1, hCG, glycodelin, galectin-1 (107), which may maintain fetal-maternal tolerance, and may even be related to recurrent early miscarriage (108).
The accumulated evidence indicates that when sexual intercourse occurs and seminal fluid is deposited in the female reproductive tract, the prostaglandins in the seminal fluid, i.e., PGE2, PGE1, PGE3, and PGF2 (109), initiate a signaling cascade toward the woman’s innate immune cells. The cells mentioned in Table 1, such as platelets, polymorphonuclear leukocytes, and Group 2 innate lymphoid cells participate in the physiological mechanisms in embryo tolerance and implantation, allowing successful fertilization.
Preimplantation, Implantation, and Decidualization
Implantation begins by apposition and adhesion of the embryo to the luminal epithelium of the endometrium. Following its invasion toward the stromal bed, the union of the embryo to the luminal epithelium transforms the underlying stromal fibroblasts into secretory cells of the epithelioid type, or decidualization (110). Through different molecules such as IL-1β, steroid hormones, insulin-like growth-factor-binding protein-1 (IGFBP-1) and prostaglandin-endoperoxide synthase-2 (PTGS-2), the decidualized cells regulate this stage with the invasion of embryos, and the formation of the placenta (110).
Prostaglandins participate in each stage of the interaction of the embryo with the endometrium, for example in preimplantation, implantation (apposition, adhesion/attachment, invasion/penetration) and decidualization; as well as affecting many other cells and molecules. PGs have a complex role in each of these stages, e.g., the essential role of prostaglandin E2 (PGE2) in the oocyte is to enhance the cumulus expansion in ovulation for sperm penetration, to regulate extracellular matrices disassembly (111), and also, importantly, to participate during transport and embryo implantation (112).
Prostaglandin Signaling by Seminal Fluid and Fertilization
Preceding evidence shows that sperm induces immunosuppression against hapten-modified self and alloantigens, including cytotoxic T-cell in mice responses (113). Also, seminal plasma contains high concentrations of prostaglandins, key molecules in the regulation of sexual intercourse signaling (114). The female immune response tolerates seminal plasma and supplies cytokines and prostaglandins, which are synthesized in the male accessory glands. In addition, it causes molecular and cellular changes in the endometrium. This facilitates the development and implantation of the embryo when prostaglandins, cytokines and hormones bind to receptors in target cells in the cervix and uterus (115).
The prostaglandins present in seminal fluid have a role in immune modulation. They regulate the pathways that may exacerbate inflammation in the female reproductive tract during physiological processes such as ovulation, implantation, and parturition (116), e.g., ejaculation or the spermatozoa induce an inflammatory response in the endometrium in the preimplantation period after mating, in which IL-1 (alpha and beta), and TNF-alpha participate (117).
Seminal plasma derived from the male accessory sex glands performs a fundamental function in fertilization in animals. The components of seminal plasma participate in the transport and survival of viable sperm and the elimination of non-viable sperm from the uterus (118). In the quail species, the cloacal gland produces prostaglandin F2α (PGF2α), which contributes to successful fertilization and acts as a natural mechanism for the protection of sperm from rejection or death by the female reproductive tract (119). Seminal fluid factors exert significant effects on the female reproductive tract, as shown by Shahnazi et al. (120). Also, in the uterine tissues of mice that were paired with mice without seminal vesicles, implantation rates, enzyme cytosolic PGE synthase (cPGES), microsomal PGE synthase (mPGES) and receptors EP2 and EP4 involved in the signaling pathway of PGE2, were all significantly low (120). In addition, 19-hydroxy PGE and 19-hydroxy PGF are regulators of sperm motility, and its effects may be mediated by the content of ATP in sperm (121). Prostaglandins such as PGE-1 are potent stimulators of adenylate cyclase in various cellular systems (122). An increase in adenylate cyclase activity and subsequent entry into cAMP levels may also be involved. PGs stimulate the fertilization capacity of human sperm by facilitating the transport of calcium through their plasma membrane (123).
The amplification of effects by microparticles from epididymal fluid (epididymosomes) and prostasomes could lead to the activation of many genes and the expression of related molecules, as reported in humans and mice, some species of cows, pigs and sheep (123, 124). More specifically, signaling may affect the enzymes of the cyclooxygenase pathway and other molecules related to the metabolism of arachidonic acid, e.g., Cytochrome P450 in blastocyst implantation (125), and prostaglandin D2 in the maintenance of pregnancy through Th1/Th2 and T-cytotoxic (Tc) 2 cells balance (126, 127).
The change induced by seminal plasma in a porcine uterus makes conception and pregnancy possible (128), it also reduces embryonic mortality in pigs and other livestock (129). In addition, seminal plasma possesses potent immunosuppressive activity caused by immune-deviating soluble factors, inducing tolerance, with molecules, such as Transforming growth factor-β (TGFB) and prostaglandin E (PGE).
Effects of Prostaglandins and Related Molecules on Innate Immunity and Female Reproductive Tract Cells
Cells of the innate immune response are modulated by prostaglandins (130), among them, are the following:
1. M1 macrophages (Mø1) which produce proinflammatory cytokines (TNFα, IL-6, IL-12, IL-23, and IL-1β), M2 macrophages(Mø2) which produce IL-10 and TGFβ (transforming growth factor β) and have anti-inflammatory and immune down-regulating properties. Both are regulated by prostaglandins in pregnancy (9) (Table 1).
2. Dendritic cells (DCs) have several subclasses, e.g., CD103+, myeloid, plasmacytoid, the latter are related to the production of high IFNα levels. In infertile patients with endometriosis, CD4+, CD25+, and CD103+ dendritic cells are increased in peritoneal fluid (131), dendritic cells CD103+ have a relevant role in implantation (132); in addition, CD103+ dendritic cells are regulated by prostaglandin D2 in different disorders (133).
3. Endothelial cells have innate and immune tolerogenic function (134). In patients with preeclampsia (PE), in the presence of vascular endothelial growth factor (VEGF), these cells increase levels of prostacyclin (135). In the pathogenesis of PE, VEGF (VEGF-A) participates in the proliferation, migration and angiogenesis of endothelial cells, and works through the receptors VEGFR-1 (or Flt-1) and VEGFR-2. In PE this increases the release of FMS-like tyrosine kinase-1 (sFlt-1) and blocks free VEGF to protect the fetus from toxicity (136).
4. Neutrophils (PMN) are regulated by cytokines and prostaglandins (137). The aspirin (ASA) is used for prevention of preeclampsia in high-risk patients (138, 139). ASA triggers transcellular biosynthesis of eicosanoids by acetylation of PGHS-2. Eicosanoids correspond to 15R-epimers of lipoxins (ATL) and are potent inhibitors of leukotriene B4-mediated neutrophils (140). Considering that preeclampsia is associated with increased proinflammatory, antiangiogenic and PMN-endothelial cell adhesion, Gil-Villa et al. (141) shows that PMN adhesion in patients with preeclampsia is reduced by Aspirin-triggered lipoxin (ATL) when aspirin is used.
5. Natural killer and innate lymphoid cells (ILC). According to the cytokine profile and transcription factor, ILCs are divided into two groups, cytotoxic and “helper”-ILC (17). The cytotoxic ILC group is represented by Natural Killer (NK). The “helper”-ILC in humans has three subclasses, ILC1 with two subsets, producing IFNγ; ILC2 produces IL-5, IL-13, and IL-4; and ILC3 releases IL-17 and IL-22. The NK cells in a decidua (dNK) microenvironment are around 50% to 70% of the total of lymphoid cells in decidual tissue. They have CD56bright CD16− KIR+ CD9+, and activate the NK receptor phenotype, participate with cytokines, which mediate new vessel formation, aid in the renovation of existing tissues and placentation through the release of VEGF, stromal-derived factor-1 (SDF-1) and IFN-γ-inducing protein 10 (9). In stromal tissue, the decidual stromal cells (DSCs) participate in the induction of maternal tolerance, physically concur and have a regulatory mechanism in dNK, and CD14+ myelomonocytic cells, and induce regulatory Treg. Also, DSCs inhibit dendritic cells through prostaglandin E2 (PGE2) and Indoleamine 2,3-dioxygenase (IDO), this inhibition favors the maintenance of the pregnancy (18).
In the normal eutopic endometrium, the Mø2 together with the Tregs predominate, providing an anti-inflammatory environment for the implantation of the embryo, while in endometriosis, they can cause infertility. The Mø1 provide a pro-inflammatory environment which affects embryo implantation, the dendritic cells (DC) do not increase in endometrial tissue, also the Treg is dysregulated. Therefore, DC does not eliminate the cellular debris which could migrate to the peritoneal cavity and grow in ectopic sites, developing as endometriosis. On the other hand, Treg and NK have abnormal behavior, the first favors a pro-inflammatory state and the second is less cytotoxic which impacts embryo implantation (142). COX2 and PGE2 are related to the pathogenesis of endometriosis. A high level of COX-2 due to various factors such as estrogens, hypoxia and environmental pollutants could suppress apoptosis and increase cell proliferation through PGE2 and its receptors EP2, and EP4 in endometriosis (143). In addition, experimental studies with intralesional injections of ASA, in rabbits with peritoneal endometriosis, eliminate endometriotic lesions (144).
Prostaglandins in Implantation and Maintenance of Gestation
The generation of prostaglandins and expression of receptors in a mouse uterus has demonstrated their importance during implantation and decidualization (145). In mice, PGE2 levels increase from the 2-cell embryo stage to the blastocyst, demonstrating the importance of PGE2 in early development (112). PGE2 also plays a significant role in peri-implantation in a mouse uterus through the expression of EP2 and EP4 receptors, which increase cAMP levels during the implantation and decidualization processes. EP4 induces the activation of VEGF (growth factor vascular endothelial), increasing vascular permeability of the endometrium (146), implantation and decidualization, together with PGF2 (132).
Inadequate production of prostaglandins in mice, and possibly in humans, may explain some cases of infertility (147). Low concentrations of PGE2, PGF and PGI2 cause failure in ovulation, fertilization, implantation, and decidualization (133). In mice, prostacyclin (PGI2) is the primary prostaglandin at the implantation site. It participates in implantation and decidualization through the peroxisome proliferator-activated receptor (PPAR-δ) and the RXRα signaling pathway in the uterus (148).
As an example, PGF2α is used in fertilization procedures, in addition to GnRH, to pre-synchronize ovulation before applying for a resynchronization program in cows in dairy herds with acceptable pregnancy outcomes (149).
Prostaglandins in Maternal Immune Tolerance
When intercourse occurs, endothelial cells release IL-8, IL-1, INF-α, and TNF-α to recruit immune cells (150). Neutrophils are mobilized in the oviduct in female mammals in response to the presence of sperm (151). This process may also induce a state of unresponsiveness by the presence of anti-inflammatory cytokines, such as IL-4, IL-10, IL-13, and TGF-β (152) Figure 1.
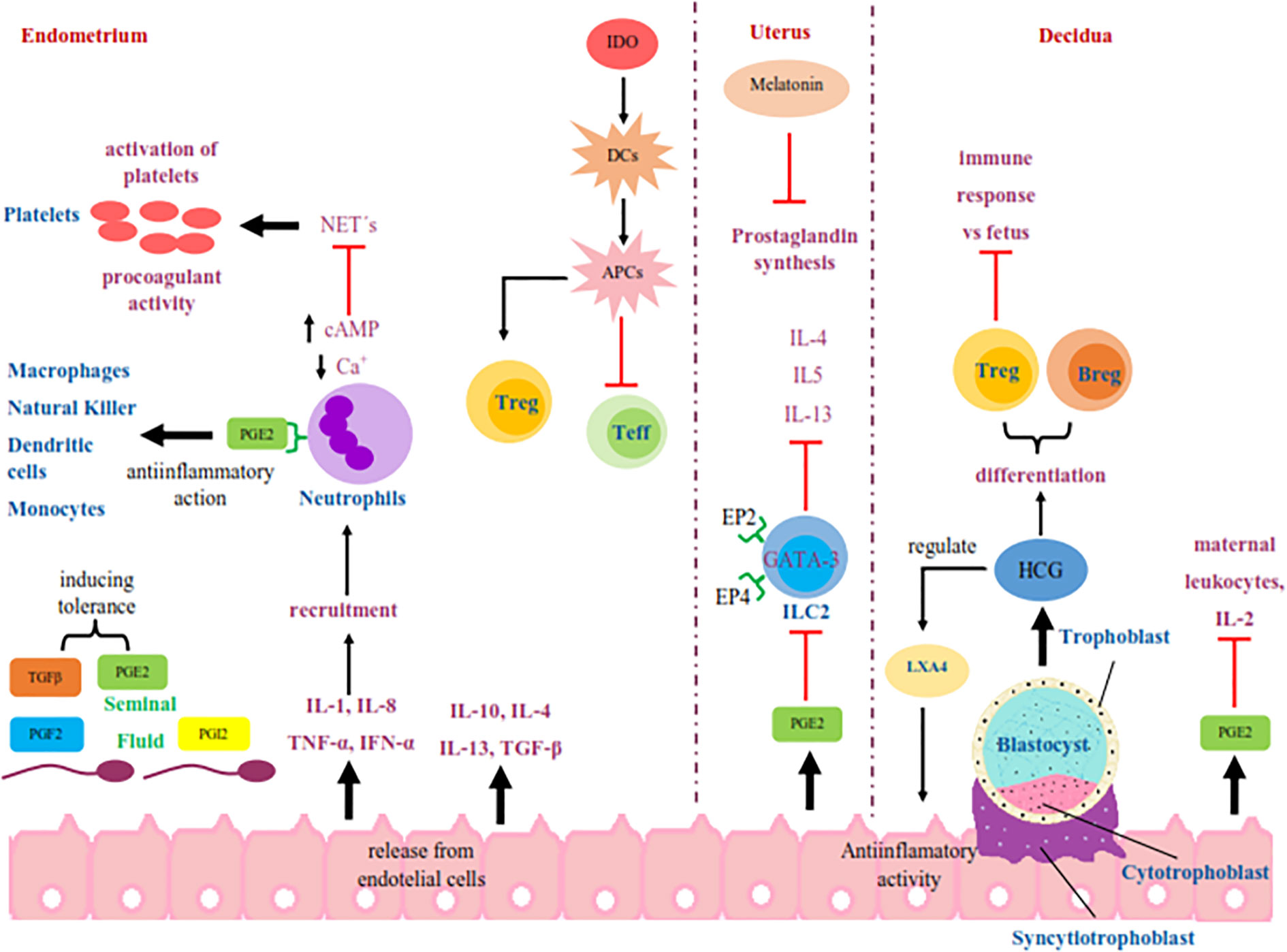
Figure 1 Schematic representation of the signaling in the maternal immune response that begins with the deposition of seminal fluid in the female reproductive tract during intercourse. The seminal fluid start an immune signaling pathways mediated by PGE2 and PGI2 in the functions of endothelial cells, platelets, neutrophils, ILC2, lymphocytes, macrophages, natural killer, dendritic cells and monocytes during oocyte fertilization and early implantation. In addition, the molecules released by these cells like interleukins, HCG, IDO, and LXA4 have a fundamental role in this tolerance process. PGE2, prostaglandin E2; PG12, prostaglandin I2; PGF2, prostaglandin F2; TGFβ, transforming growth factor beta; IL-1, interleukin-1; IL-2, interleukin-2; IL-4, interleukin-4; IL-5, interleukin-5; IL-8, interleukin-8; IL-10, interleukin-10; IL-13, interleukin-13; TNF-α, tumor necrosis factor-alpha; INF-α, interferón alpha; Ca+, calcio; cAMP, cyclic adenosine monophosphate; NET´s, neutrophil extracellular traps; IDO, indoleamine-2,3-dioxygenase; DCs, mature dendritic cells; APCs, tolerogenic antigen presenting cells; Treg, regulatory T cells; Teff, effector T cells; GATA-3, GATA-3 transcription factor; EP2, prostaglandin E2 receptor 2; EP4, prostaglandin E2 receptor 4; ILC2, group 2 innate lymphoid cells; Breg, regulatory B cells; HCG, human chorionic gonadotropin; LXA4, Lipoxin A4.
In order to prevent a compromised systemic maternal immune response, local immune regulation in the fetal-maternal interface is very important. This is achieved by several mechanisms. One of these is local immunoregulation at the fetal-maternal interface, e.g., Human amniotic membrane-derived mesenchymal stem cells (hAM-MSCs) release factors such as indoleamine 2,3 dioxygenase (IDO), TGF-β, prostaglandin E2 (PGE2), and others inducing immunomodulatory effects (153).
PGs release or regulate different kinds of cells, such as Tolerogenic dendritic cells (tol-DCs), Mø1 and Mø2 macrophages, Decidual NK cells (dNK) (CD56brightCD16-), Decidual stromal cells (DSCs), Endometrial stromal cells, Tregs (CD4+CD25+FOXP3+), and Decidual CD8+EM cells (CD45RA−CCR7−) (Table 1).
Prostaglandin E (PGE), specifically, induces T-helper type 3 (Th3) and T-regulatory 1 cells (Tr1), as shown by Lewis´ rat and mouse test (154, 155). PGE2 secretion by human deciduous cells in the first trimester of pregnancy blocks the activation of maternal leukocytes in the decidua and inhibits IL-2 production and its receptor (156).
Other cells assisting in the decidualization of endometrial stromal cells (ESCs) and pregnancy maintenance are decidual natural killer (dNK) cells (157) and CD14+ cells for Treg induction and immunosuppression (158). Also, Treg and Breg may contribute to the regulation of type 1 and 2-like T helper anti-fetal immune mechanisms during human pregnancy (159) (Table 1).
Platelets
It is evident that platelets may be important in tolerance mechanisms. Platelet activity is inhibited post-coitus, and this inhibition depends on prostaglandins (160). Seminal fluid has factors that favor clot formation, similar to peripheral blood, such as Factor VIII: Ag, FVIII: C and Von Willebrand factor (vWF), in addition to other factors (161). vWF (162), fibronectin (163), and vitronectin (164) are proteins that favor platelet adhesion (165). This implies that inhibition of platelet aggregation by PGI2 could be a compensatory mechanism for pro-adhesive molecules.
Using a mouse model, Etulain et al. (166) found that platelets act through P-selectin glycoprotein ligand-1 (PSGL-1), and directly affect neutrophil extracellular traps (NETosis). Platelet P-selectin is crucial for neutrophil recruitment (167). Furthermore, NETs cause the recruitment and activation of platelets and induce procoagulant activity due to the expression of histones H3 and H4, toll-like receptor 2 (TLR2) and TLR4 platelets. NETs present a surface for the activation of coagulation factor XII (168) in order to promote thrombosis as a mechanism of rejection (169).
Platelets cause a decrease in the formation of extracellular traps when preincubated with PGI2, followed by stimulation with lipopolysaccharide (LPS), arachidonic acid, and a synthetic diacylated lipopeptide (Pam3SCK4). This highlights the physiological role of PGI2 in platelet modulation (170). Prostaglandins may also inhibit the function of neutrophils by increasing levels of cyclic adenosine monophosphate (cAMP) (171).
The interaction of PMN-platelets releases products of arachidonic acid serving as precursors of neutrophil eicosanoids (172). In polymorphonuclear neutrophils (PMN), PGE2 modulates their response through the expression of EP2 and EP4 receptors (173).
In addition, other mechanisms of maternal immune tolerance are mediated by placental trophoblast derived microvesicles (MVs) and maternal thrombocyte-derived MVs. These bind to circulating peripheral T lymphocytes through P-selectin (CD62P)–PSGL-1 (CD162) interaction induces STAT3 phosphorylation in T cells (174).
The above mentioned may explain why platelet aggregation is inhibited post-intercourse and has a possible reduction in the formation of NETs to protect the embryo. It is possible that the release of extracellular traps may contribute to trophoblast lesions.
Many other cells mentioned above participate through high complexity fetal-maternal interface interaction to induce a tolerance stage, which protects the embryo (175).
Polymorphonuclear Cells
In mammalian species, PMNs are implicated in endometrial remodeling as being receptive to oocyte implantation. Human neutrophils exposed to progesterone and estriol hormones promote the establishment of maternal tolerance through the induction of CD4+ T cells (176).
In humans, during coitus, sperm is deposited into the female reproductive tract (FRT). Neutrophils are then recruited for the elimination of excess sperm through phagocytosis (177).
However, bovine seminal plasma is shown to reduce the ability of PMNs to phagocytize bull sperm. Furthermore, equine seminal plasma is reported to contain factors that reduce the binding of neutrophils to sperm, avoiding the formation of NETs (178). In humans, when granulocytes are exposed to the seminal plasma, the respiratory burst is inhibited (179). These mechanisms allow more of the healthy motile sperm to reach the oviduct, which makes it clear that seminal plasma contains factors that modulate the response of PMN.
In addition, PGE2 can exert anti-inflammatory action on neutrophils and other innate immune cells such as macrophages, natural killer cells, dendritic cells, and monocytes (180, 181). Also, it inhibits the production of IFN-α in plasmacytoid dendritic cells and the production of IL-12 in myeloid dendritic cells.
Finally, polymorphonuclear leukocytes contribute to preterm labor by activating prostaglandin production from human fetal membranes (182).
Group 2 Innate Lymphoid Cells
Specific ILC2s (Group 2 innate lymphoid cells) and uterine innate lymphoid cells (uILCs, uILC1, uILC2, and uILC3) (183) in the uterus are regulated by PGD2, PGE2, PGI2, and sex hormones, in particular, oestrogen (151, 184). Together, these may play a role in the balance between immunity and tolerance at the beginning of placenta formation and could be related to pregnancy loss, as shown in mice (185). Some studies show that ILC2 is the most abundant subset in the human fetal-maternal interface during premature and full-term pregnancies, in which its presence is regulated by sex hormones (e.g., oestrogen) (186). PGI2 decreases the proliferation of ILC2 and significantly inhibits the expression of IL-5 and IL-13 induced by IL-33 (187).
The production of PGE2 could also suppress the function of neutrophils and uILCs, a particular cell, similar to ILC2, through its EP2 and EP4 receptors in both healthy humans and mouse models (188, 189). PGE2 inhibits the expression of GATA-3, as well as the production of type 2 cytokines (IL-5 and IL-13) (144). These effects are mediated by the action of the EP2 and EP4 prostanoid receptors, which are specifically expressed in ILC2 (151, 190).
In addition, Group 1ILCs, uNK cells, and uILC3s significantly increase in abortion in mice. They also have a lower proportion of uILC2s (183).
Discussion
Of the hundreds of molecules released with cells in the preimplantation, implantation, and decidualization processes; prostaglandins are integrated into each of these stages by seminal fluid, even until parturition. In particular, some of these molecules are found to be related to infertility and abortions, such as PGE2, PGF, and PGI2, which, in turn, are related to ovulation, fertilization, implantation, and decidualization (133). Increased levels of IL6 are also related to unexplained infertility, recurrent miscarriage, and pre-eclampsia among other disorders (9), e.g., in humans, cases of placental insufficiency, manifesting as intrauterine fetal growth restriction, are observed where the level of melatonin, a molecule with pleiotropic effects that regulates inflammatory processes (191), is decreased (192). Melatonin inhibits prostaglandin synthesis and is a potent inducer of uterine contractility (54, 193), in addition, there is evidence that in fish, melatonin is produced in the granulosa cells and is a critical factor for ovulation (194). Likewise, in women, it increases progesterone and regulates the corpus luteum (195). Also in a recent clinical trial, melatonin is shown to improve intrafollicular oxidative balance and gives a slight increase in the rate of human live births (196). Another example is Polish landrace gilts treated with pregnant mare serum gonadotropin (PMSG) and human chorionic gonadotropin (hCG) (PMSG/hCG-induced). Treatment with exogenous progesterone increases pregnancy success through the expression of genes responsible for vascular function and PGE2 synthesis (197). Therefore, the administration of inhibitors of prostaglandin synthesis, e.g., PGE2, must be carefully considered due to the multiple mechanisms of female fertility in which they participate (111).
Also, the mechanism of control over the rate of gene transcription or transcriptional regulation is altered in genes involved in chronic endometritis and the inflammatory response (IL-11, CCL4), growth factors (IGFBP1), and apoptotic proteins (BCL2, BAX, CASP8) in infertile patients (198).
Another mechanism of transcriptional regulation is that of Uterine Vascular Endothelial Growth Factor (UVEGF), in which PGE2 regulates vascular development through receptors EP2 and EP4.
Conclusions
To maintain fetal-maternal tolerance in the process of implantation (apposition/adhesion/invasion), a whole network of cells and molecules regulate different factors and responses according to the stage of pregnancy. Among the most highly studied cells and molecules are tolerogenic dendritic cells (tol-DCs), M1 and M2 macrophages, Decidual NK cells (dNK) (CD56brightCD16−), Decidual stromal cells (DSCs), Endometrial stromal cells, Tregs (CD4+ CD25+ FOXP3+) and Decidual CD8+ EM cells (CD45RA− CCR7−), progesterone, oestrogen, Leukaemia inhibitory factor (LIF), Indoleamine-2,3-dioxygenase (IDO), and melatonin. Within this complex network, prostaglandins, specifically, PGD2, PGF2α, and PGE2, are important modulators and regulators in maintaining maternal-fetal tolerance, as we deduced. Nevertheless, other cells such as platelets, uILCs, and polymorphonuclear leukocyte/Nets require more research.
Author Contributions
Conceptualization: EP-C and GM. Writing—original draft preparation: GM, GV, LP-C, MH-H, EC-P. Manuscript revision: GM, LP-C, MH-H, EZ, EP-CM, MM, RM, CM-C, NM, CR, EC-P, and EP-C. All authors contributed to the article and approved the submitted version.
Funding
This research was supported by from National Technological of Mexico/ITOaxaca (project 5302.19-P) and Benito Juárez Autonomous University of Oaxaca (UABJO-CA-056). This work was supported by the Clinical Pathology Laboratory “Dr Eduardo Perez Ortega” in Oaxaca, Mexico.
Conflict of Interest
The authors declare that the research was conducted in the absence of any commercial or financial relationships that could be construed as a potential conflict of interest.
Acknowledgments
The authors would like to thank Charlotte Grundy for her support throughout the work, and also UABJO and National Institute of Technology in Mexico, (TecNM) project 5302.19-P, for financial support.
References
1. Seo MJ, Oh DK. Prostaglandin synthases: Molecular characterization and involvement in prostaglandin biosynthesis. Prog Lipid Res (2017) 66:50–68. doi: 10.1016/j.plipres.2017.04.003
2. Smyth EM, Grosser T, Wang M, Yu Y, FitzGerald GA. Prostanoids in health and disease. J Lipid Res (2009) 50:S423–8. doi: 10.1194/jlr.R800094-JLR200
3. Hanna VS, Hafez EAA. Synopsis of arachidonic acid metabolism: A review. J Adv Res (2018) 11:23–32. doi: 10.1016/j.jare.2018.03.005
4. Wang T, Fu X, Chen Q, Patra JK, Wang D, Wang Z, et al. Arachidonic Acid Metabolism and Kidney Inflammation. Int J Mol Sci (2019) 20(15):3683. doi: 10.3390/ijms20153683
5. Sugimoto Y, Inazumi T, Tsuchiya S. Roles of prostaglandin receptors in female reproduction. J Biochem (2015) 157(2):73–80. doi: 10.1093/jb/mvu081
6. Liu S, Wei H, Li Y, Huang C, Lian R, Xu J, et al. Downregulation of ILT4+ dendritic cells in recurrent miscarriage and recurrent implantation failure. Am J Reprod Immunol (2018) 80(4):e12998. doi: 10.1111/aji.12998
7. Flórez-Grau G, Cabezón R, Borgman KJE, España C, Lozano JJ, Garcia-Parajo MF, et al. Up-regulation of EP 2 and EP 3 receptors in human tolerogenic dendritic cells boosts the immunosuppressive activity of PGE 2. J Leukocyte Biol (2017) 102(3):881–95. doi: 10.1189/jlb.2A1216-526R
8. Robertson SA, Care AS, Moldenhauer LM. Regulatory T cells in embryo implantation and the immune response to pregnancy. J Clin Invest (2018) 128(10):4224–35. doi: 10.1172/JCI122182
9. Brown MB, von Chamier M, Allam AB, Reyes L. M1/M2 macrophage polarity in normal and complicated pregnancy. Front Immunol (2014) 5:606. doi: 10.3389/fimmu.2014.00606
10. Liu M, Gong Y, Wei JY, Xie D, Wang J, Yu YH, et al. [Media of rat macrophage NR8383 cells with prostaglandins E2-induced VEGF over-expression promotes migration and tube formation of human umbilical vein endothelial cells]. Nan Fang Yi Ke Da Xue Xue Bao (2016) 36(7):936–40.
11. Magatti M, Vertua E, De Munari S, Caro M, Caruso M, Silini A, et al. Human amnion favours tissue repair by inducing the M1-to-M2 switch andenhancing M2 macrophage features. J Tissue Eng Regener Med (2017) 11(10):2895–911. doi: 10.1002/term.2193
12. Sojka DK, Yang L, Yokoyama WM. Uterine natural killer cells: To protect and to nurture. Birth Defects Res (2018) 110(20):1531–8. doi: 10.1002/bdr2.1419
13. Fu B, Zhou Y, Ni X, Tong X, Xu X, Dong Z, et al. Natural killer cells promote fetal development through the secretion of growth-promoting factors. Immunity (2017) 47(6):1100–13. doi: 10.1016/j.immuni.2017.11.018
14. Yang HL, Zhou WJ, Lu H, Lei ST, Ha SY, Lai ZZ, et al. Decidual stromal cells promote the differentiation of CD56bright CD16- NK cells by secreting IL-24 in early pregnancy. Am J Reprod Immunol (2019) 81(6):e13110. doi: 10.1111/aji.13110
15. Manaster I, Mizrahi S, Goldman-Wohl D, Sela HY, Stern-Ginossar N, Lankry D, et al. Endometrial NK Cells Are Special Immature Cells That Await Pregnancy. J Immunol (2008) 181(3):1869–76. doi: 10.4049/jimmunol.181.3.1869
16. Yu Z, Croy BA, King GJ. Lysis of Porcine Trophoblast Cells by Endometrial Natural Killer-Like Effector Cells in Vitro does not Require Interleukin-21. Biol Reprod (1994) 51(6):1279–84. doi: 10.1095/biolreprod51.6
17. Vacca P, Vitale C, Munari E, Cassatella MA, Mingari MC, Moretta L. Human Innate Lymphoid Cells: Their Functional and Cellular Interactions in Decidua. Front Immunol (2018) 9:1897:1897. doi: 10.3389/fimmu.2018.01897
18. Croxatto D, Vacca P, Canegallo F, Conte R, Venturini PL, Moretta L, et al. Stromal cells from human decidua exert a strong inhibitory effect on NK cell function and dendritic cell differentiation. PloS One (2014) 9(2):e89006. doi: 10.1371/journal.pone.0089006
19. Ernerudh J, Berg G, Mjösberg J. Regulatory T helper cells in pregnancy and their roles in systemic versus local immune tolerance. Am J Reprod Immunol (2011) 66(1):31–43. doi: 10.1111/j.1600-0897.2011.01049.x
20. Powell RM, Lissauer D, Tamblyn J, Beggs A, Cox P, Moss P, et al. Decidual T Cells Exhibit a Highly Differentiated Phenotype and Demonstrate Potential Fetal Specificity and a Strong Transcriptional Response to IFN. J Immunol (2017) 199(10):3406–17. doi: 10.4049/jimmunol.1700114
21. Kieffer TEC, Laskewitz A, Scherjon SA, Faas MM, Prins JR. Memory T Cells in Pregnancy. Front Immunol (2019) 10:625. doi: 10.3389/fimmu.2019.00625
22. Tilburgs T, Schonkeren D, Eikmans M, Nagtzaam NM, Datema G, Swings GM, et al. Decidual Tissue Contains Differentiated CD8+ Effector-Memory T Cells with Unique Properties. J Immunol (2010) 185(7):4470–7. doi: 10.4049/jimmunol.0903597
23. Ouellette MJ, St-Jacques S, Lambert RD. CD8 membrane expression is down-regulated by transforming growth factor (TGF)-beta 1, TGF-beta 2, and prostaglandin E2. Am J Reprod Immunol (1999) 41(3):183–91. doi: 10.1111/j.1600-0897.1999.tb00531.x
24. Erkers T, Nava S, Yosef J, Ringdén O, Kaipe H. Decidual stromal cells promote regulatory T cells and suppress alloreactivity in a cell contact-dependent manner. Stem Cells Dev (2013) 22(19):2596–605. doi: 10.1089/scd.2013.0079
25. Grasso E, Paparini D, Agüero M, Mor G, Pérez Leirós C, Ramhorst R. VIP contribution to the decidualization program: regulatory T cell recruitment. J Endocrinol (2014) 221(1):121–31. doi: 10.1530/JOE-13-0565
26. Ahmadi M, Abdolmohamadi-Vahid S, Ghaebi M, Dolati S, Abbaspour-Aghdam S, Danaii S, et al. Sirolimus as a new drug to treat RIF patients with elevated Th17/Treg ratio: A double-blind, phase II randomized clinical trial. Int Immunopharmacol (2019) 74:105730. doi: 10.1016/j.intimp.2019.105730
27. Wang S, Qian J, Sun F, Li M, Ye J, Li M, et al. Bidirectional regulation between 1st trimester HTR8/SVneo trophoblast cells and in vitro differentiated Th17/Treg cells suggest a fetal-maternal regulatory loop in human pregnancy. Am J Reprod Immunol (N Y NY 1989) (2019) 81(5):e13106. doi: 10.1111/aji.13106
28. Dunn CL, Critchley HO, Kelly RW. IL-15 regulation in human endometrial stromal cells. J Clin Endocrinol Metab (2002) 87(4):1898–901. doi: 10.1210/jcem.87.4.8539
29. De Clercq K, Hennes A, Vriens J. Isolation of Mouse Endometrial Epithelial and Stromal Cells for In Vitro Decidualization. J Visualized Experiments: JoVE (2017) 121):55168. doi: 10.3791/55168
30. Gnecco JS, Ding T, Smith C, Lu J, Bruner-Tran KL, Osteen KG. Hemodynamic forces enhance decidualization via endothelial-derived prostaglandin E2 and prostacyclin in a microfluidic model of the human endometrium. Hum Reprod (Oxford England) (2019) 34(4):702–14. doi: 10.1093/humrep/dez003
31. Oettel A, Lorenz M, Stangl V, Costa SD, Zenclussen AC, Schumacher A. Human Umbilical Vein Endothelial Cells foster conversion of CD4+CD25-Foxp3- T cells into CD4+Foxp3+ Regulatory T Cells via Transforming Growth Factor-β. Sci Rep (2016) 6:23278. doi: 10.1038/srep23278
32. Padmanabhan RA, Laloraya M. Estrogen-Initiated Protein Interactomes During Embryo Implantation. Am J Reprod Immunol (N Y NY 1989) (2016) 75(3):256–62. doi: 10.1111/aji.12455
33. Vrtačnik P, Ostanek B, Mencej-Bedrač S, Marc J. The many faces of estrogen signaling. Biochem Med (Zagreb) (2014) 24(3):329–42. doi: 10.11613/BM.2014.035
34. Lin XG, Zhou Q, Wang L, Gao Y, Zhang WN, Luo ZL, et al. Pregnancy estrogen drives the changes of T-lymphocyte subsets and cytokines and prolongs the survival of H-Y skin graft in murine model. Chin Med J (2010) 123(18):2593–9. doi: 10.3760/cma.j.issn.0366-6999.2010.18.019
35. Rahimipour M, Salehnia M, Jafarabadi M. Morphological, Ultrastructural, and Molecular Aspects of In Vitro Mouse Embryo Implantation on Human Endometrial Mesenchymal Stromal Cells in The Presence of Steroid Hormones as An Implantation Model. Cell J (2018) 20(3):369–76. doi: 10.22074/cellj.2018.5221
36. Pan T, Zhong L, Wu S, Cao Y, Yang Q, Cai Z, et al. 17β-Oestradiol enhances the expansion and activation of myeloid-derived suppressor cells via signal transducer and activator of transcription (STAT)-3 signalling in human pregnancy. Clin Exp Immunol (2016) 185(1):86–97. doi: 10.1111/cei.12790
37. Falchi L, Scaramuzzi RJ. An in vitro investigation of the actions of reproductive hormones on the cervix of the ewe in the follicular stage: the effects of 17β-estradiol, oxytocin, FSH, and arachidonic acid on the cervical pathway for the synthesis of prostaglandin E2. Theriogenology (2015) 83(6):1007–14. doi: 10.1016/j.theriogenology.2014.12.003
38. Kyurkchiev D, Ivanova-Todorova E, Kyurkchiev SD. New target cells of the immunomodulatory effects of progesterone. Reprod Biomed Online (2010) 21(3):304–11. doi: 10.1016/j.rbmo.2010.04.014
39. Svensson J, Jenmalm MC, Matussek A, Geffers R, Berg G, Ernerudh J. Macrophages at the fetal-maternal interface express markers of alternative activation and are induced by M-CSF and IL-10. J Immunol (Baltimore Md: 1950) (2011) 187(7):3671–82. doi: 10.4049/jimmunol.1100130
40. Fujisaki S, Kawano K, Haruyama Y, Mori N. Synergistic effect of progesterone on prostaglandin E modulation of the mitogenic response of human peripheral lymphocytes. J Reprod Immunol (1985) 7(1):15–26. doi: 10.1016/0165-0378(85)90018-x
41. Cole LA. Biological functions of hCG and hCG-related molecules. Reprod Biol Endocrinol RBE (2010) 8:102. doi: 10.1186/1477-7827-8-102
42. Szmidt M, Sysa P, Bartłomiej JB, Niemiec T. Gonadotropina kosmówkowa jako kluczowy czynnik regulujacy implantacje zarodka [Chorionic gonadotropin as the key factor for embryo implantation]. Ginekol Polska (2008) 79(10):692–6.
43. Bansal AS, Bora SA, Saso S, Smith JR, Johnson MR, Thum MY. Mechanism of human chorionic gonadotrophin-mediated immunomodulation in pregnancy. Expert Rev Clin Immunol (2012) 8(8):747–53. doi: 10.1586/eci.12.77
44. Schumacher A, Costa SD, Zenclussen AC. Endocrine factors modulating immune responses in pregnancy. Front Immunol (2014) 5:196. doi: 10.3389/fimmu.2014.00196
45. North RA, Whitehead R, Larkins RG. Stimulation by human chorionic gonadotropin of prostaglandin synthesis by early human placental tissue. J Clin Endocrinol Metab (1991) 73(1):60–70. doi: 10.1210/jcem-73-1-60
46. Mumtaz A, Khalid A, Jamil Z, Fatima SS, Arif S, Rehman R. Kisspeptin: A Potential Factor for Unexplained Infertility and Impaired Embryo Implantation. Int J Fertility Steril (2017) 11(2):99–104. doi: 10.22074/ijfs.2017.4957
47. Francis VA, Abera AB, Matjila M, Millar RP, Katz AA. Kisspeptin regulation of genes involved in cell invasion and angiogenesis in first trimester human trophoblast cells. PloS One (2014) 9(6):e99680. doi: 10.1371/journal.pone.0099680
48. Skorupskaite K, George JT, Anderson RA. The kisspeptin-GnRH pathway in human reproductive health and disease. Hum Reprod Update (2014) 20(4):485–500. doi: 10.1093/humupd/dmu009
49. Pinilla L, Aguilar E, Dieguez C, Millar RP, Tena-Sempere M. Kisspeptins and reproduction: physiological roles and regulatory mechanisms. Physiol Rev (2012) 92(3):1235–316. doi: 10.1152/physrev.00037.2010
50. Harper MJ. Platelet-activating factor: a paracrine factor in preimplantation stages of reproduction? Biol Reproduct (1989) 40(5):907–13. doi: 10.1095/biolreprod40.5.907
51. Tiemann U. The role of platelet-activating factor in the mammalian female reproductive tract. Reprod Domest Anim Zuchthygiene (2008) 43(6):647–55. doi: 10.1111/j.1439-0531.2007.00959.x
52. Roudebush WE. Role of platelet-activating factor in reproduction: sperm function. Asian J Androl (2001) 3(2):81–5.
53. De M, Sanford TR, Wood GW. Expression of interleukin 1, interleukin 6 and tumour necrosis factor alpha in mouse uterus during the peri-implantation period of pregnancy. J Reprod Fertility (1993) 97(1):83–9. doi: 10.1530/jrf.0.0970083
54. Ochoa-Bernal MA, Fazleabas AT. Physiologic Events of Embryo Implantation and Decidualization in Human and Non-Human Primates. Int J Mol Sci (2020) 21(6):1973. doi: 10.3390/ijms21061973
55. Cork BA, Tuckerman EM, Li TC, Laird SM. Expression of interleukin (IL)-11 receptor by the human endometrium in vivo and effects of IL-11, IL-6 and LIF on the production of MMP and cytokines by human endometrial cells in vitro. Mol Hum Reproduct (2002) 8(9):841–8. doi: 10.1093/molehr/8.9.841
56. Prins JR, Gomez-Lopez N, Robertson SA. Interleukin-6 in pregnancy and gestational disorders. J Reprod Immunol (2012) 95(1-2):1–14. doi: 10.1016/j.jri.2012.05.004
57. Baston-Buest DM, Schanz A, Buest S, Fischer JC, Kruessel JS, Hess AP. The embryo’s cystatin C and F expression functions as a protective mechanism against the maternal proteinase cathepsin S in mice. Reprod (Cambridge England) (2010) 139(4):741–8. doi: 10.1530/REP-09-0381
58. Liu S, Wang J, Qin HM, Yan XM, Yang XS, Liu C, et al. LIF upregulates poFUT1 expression and promotes trophoblast cell migration and invasion at the fetal-maternal interface. Cell Death Dis (2014) 5(8):e1396. doi: 10.1038/cddis.2014.335
59. Zheng Q, Dai K, Cui X, Yu M, Yang X, Yan B, et al. Leukemia inhibitory factor promote trophoblast invasion via urokinase-type plasminogen activator receptor in preeclampsia. Biomed Pharmacother Biomed Parmacother (2016) 80:102–8. doi: 10.1016/j.biopha.2016.03.005
60. Horita H, Kuroda E, Hachisuga T, Kashimura M, Yamashita U. Induction of prostaglandin E2 production by leukemia inhibitory factor promotes migration of first trimester extravillous trophoblast cell line, HTR-8/SVneo. Hum Reprod (Oxford England) (2007) 22(7):1801–9. doi: 10.1093/humrep/dem125
61. Viganò P, Mangioni S, Pompei F, Chiodo I. Maternal-conceptus cross talk–a review. Placenta (2003) 24(B):S56–61. doi: 10.1016/s0143-4004(03)00137-1
62. Hambartsoumian E. Endometrial leukemia inhibitory factor (LIF) as a possible cause of unexplained infertility and multiple failures of implantation. Am J Reprod Immunol (N Y NY 1989) (1998) 39(2):137–43. doi: 10.1111/j.1600-0897.1998.tb00345.x
63. Fouladi-Nashta AA, Mohamet L, Heath JK, Kimber SJ. Interleukin 1 signaling is regulated by leukemia inhibitory factor (LIF) and is aberrant in Lif-/- mouse uterus. Biol Reproduct (2008) 79(1):142–53. doi: 10.1095/biolreprod.107.065219
64. Marwood M, Visser K, Salamonsen LA, Dimitriadis E. Interleukin-11 and leukemia inhibitory factor regulate the adhesion of endometrial epithelial cells: implications in fertility regulation. Endocrinology (2009) 150(6):2915–23. doi: 10.1210/en.2008-1538
65. von Rango U, Alfer J, Kertschanska S, Kemp B, Müller-Newen G, Heinrich PC, et al. Interleukin-11 expression: its significance in eutopic and ectopic human implantation. Mol Hum Reproduct (2004) 10(11):783–92. doi: 10.1093/molehr/gah107
66. Kopcow HD, Karumanchi SA. Angiogenic factors and natural killer (NK) cells in the pathogenesis of preeclampsia. J Reprod Immunol (2007) 76(1-2):23–9. doi: 10.1016/j.jri.2007.03.018
67. Joshi PC, Zhou X, Cuchens M, Jones Q. Prostaglandin E2 suppressed IL-15-mediated human NK cell function through down-regulation of common gamma-chain. J Immunol (Baltimore Md 1950) (2001) 166(2):885–91. doi: 10.4049/jimmunol.166.2.885
68. Ai Z, Jing W, Fang L. Cytokine-Like Protein 1(Cytl1): A Potential Molecular Mediator in Embryo Implantation. PloS One (2016) 11(1):e0147424. doi: 10.1371/journal.pone.0147424
69. Wang H, Shi G, Li M, Fan H, Ma H, Sheng L. Correlation of IL-1 and HB-EGF with endometrial receptivity. Exp Ther Med (2018) 16(6):5130–6. doi: 10.3892/etm.2018.6840
70. Moghani-Ghoroghi F, Moshkdanian G, Sehat M, Nematollahi-Mahani SN, Ragerdi-Kashani I, Pasbakhsh P. Melatonin Pretreated Blastocysts along with Calcitonin Administration Improved Implantation by Upregulation of Heparin Binding-Epidermal Growth Factor Expression in Murine Endometrium. Cell J (2018) 19(4):599–606. doi: 10.22074/cellj.2018.4737
71. Achache H, Revel A. Endometrial receptivity markers, the journey to successful embryo implantation. Hum Reprod Update (2006) 12(6):731–46. doi: 10.1093/humupd/dml004
72. Foulk RA, Zdravkovic T, Genbacev O, Prakobphol A. Expression of L-selectin ligand MECA-79 as a predictive marker of human uterine receptivity. J Assisted Reprod Genet (2007) 24(7):316–21. doi: 10.1007/s10815-007-9151-8
73. Yang Y, Chen X, Saravelos SH, Liu Y, Huang J, Zhang J, et al. HOXA-10 and E-cadherin expression in the endometrium of women with recurrent implantation failure and recurrent miscarriage. Fertility Steril (2017) 107(1):136–143.e2. doi: 10.1016/j.fertnstert.2016.09.016
74. Lu DP, Tian L, O’Neill C, King NJ. Regulation of cellular adhesion molecule expression in murine oocytes, peri-implantation and post-implantation embryos. Cell Res (2002) 12(5-6):373–83. doi: 10.1038/sj.cr.7290139
75. Carlomagno G, Minini M, Tilotta M, Unfer V. From Implantation to Birth: Insight into Molecular Melatonin Functions. Int J Mol Sci (2018) 19(9):2802. doi: 10.3390/ijms19092802
76. Voiculescu SE, Zygouropoulos N, Zahiu CD, Zagrean AM. Role of melatonin in embryo fetal development. J Med Life (2014) 7(4):488–92.
77. Gimeno MF, Landa A, Sterin-Speziale N, Cardinali DP, Gimeno AL. Melatonin blocks in vitro generation of prostaglandin by the uterus and hypothalamus. Eur J Pharmacol (1980) 62(4):309–17. doi: 10.1016/0014-2999(80)90098-9
78. Xiong T, Zhao Y, Hu D, Meng J, Wang R, Yang X, et al. Administration of calcitonin promotes blastocyst implantation in mice by up-regulating integrin β3 expression in endometrial epithelial cells. Hum Reprod (Oxford England) (2012) 27(12):3540–51. doi: 10.1093/humrep/des330
79. Xiong J, Zeng P, Ye D. Lipoxins: a novel regulator in embryo implantation. TheScientificWorldJournal (2011) 11:235–41. doi: 10.1100/tsw.2011.15
80. Schwenke M, Knöfler M, Velicky P, Weimar CH, Kruse M, Samalecos A, et al. Control of human endometrial stromal cell motility by PDGF-BB, HB-EGF and trophoblast-secreted factors. PloS One (2013) 8(1):e54336. doi: 10.1371/journal.pone.0054336
81. Jaber L, Kan FW. Non-identical distribution pattern of epidermal growth factor and platelet-derived growth factor in the mouse uterus during the oestrous cycle and early pregnancy. Histochem J (1998) 30(10):711–22. doi: 10.1023/a:1003441904274
82. Haimovici F, Anderson DJ. Cytokines and growth factors in implantation. Microscopy Res Tech (1993) 25(3):201–7. doi: 10.1002/jemt.1070250303
83. Coppock HA, White A, Aplin JD, Westwood M. Matrix metalloprotease-3 and -9 proteolyze insulin-like growth factor-binding protein-1. Biol Reproduct (2004) 71(2):438–43. doi: 10.1095/biolreprod.103.023101
84. Gonzalez M, Neufeld J, Reimann K, Wittmann S, Samalecos A, Wolf A. Expansion of human trophoblastic spheroids is promoted by decidualized endometrial stromal cells and enhanced by heparin-binding epidermal growth factor-like growth factor and interleukin-1 β. Mol Hum Reproduct (2011) 17(7):421–33. doi: 10.1093/molehr/gar015
85. Ozbilgin K, Karaca F, Turan A, Köse C, Vatansever S, Ozcakir T. The higher heparin-binding epidermal growth factor (HB-EGF) in missed abortion. Taiwanese J Obstetr Gynecol (2015) 54(1):13–8. doi: 10.1016/j.tjog.2013.08.011
86. Macdonald LJ, Boddy SC, Denison FC, Sales KJ, Jabbour HN. A role for lipoxin A₄ as an anti-inflammatory mediator in the human endometrium. Reprod (Cambridge England) (2011) 142(2):345–52. doi: 10.1530/REP-11-0021
87. Agostinis C, Tedesco F, Bulla R. Alternative functions of the complement protein C1q at embryo implantation site. J Reprod Immunol (2017) 119:74–80. doi: 10.1016/j.jri.2016.09.001
88. Madhukaran SP, Kishore U, Jamil K, Choolani M, Lu J. Decidual expression and localization of human surfactant protein SP-A and SP-D, and complement protein C1q. Mol Immunol (2015) 66(2):197–207. doi: 10.1016/j.molimm.2015.03.001
89. Liu C, Liang X, Wang J, Zheng Q, Zhao Y, Khan MN, et al. Protein O-fucosyltransferase 1 promotes trophoblast cell proliferation through activation of MAPK and PI3K/Akt signaling pathways. Biomed Pharmacother Biomed Parmacother (2017) 88:95–101. doi: 10.1016/j.biopha.2017.01.026
90. Ortega MA, Asúnsolo Á, Álvarez-Rocha MJ, Romero B, De León-Luis J, Álvarez-Mon M, et al. Remodelling of collagen fibres in the placentas of women with venous insufficiency during pregnancy. Histol Histopathol (2018) 33(6):567–76. doi: 10.14670/HH-11-948
91. Herrler A, von Rango U, Beier HM, Wu HM, Huang HY, Lee CL, et al. Embryo-maternal signalling: how the embryo starts talking to its mother to accomplish implantation. Reprod Biomed Online (2003) 6(2):244–56. doi: 10.1016/s1472-6483(10)61717-8
92. Wu HM, Huang HY, Lee CL, Soong YK, Leung PC, Wang HS. Gonadotropin-releasing hormone type II (GnRH-II) agonist regulates the motility of human decidual endometrial stromal cells: possible effect on embryo implantation and pregnancy. Biol Reproduct (2015) 92(4):98. doi: 10.1095/biolreprod.114.127324
93. Roussev RG, Coulam CB. HLA-G and its role in implantation (review). J Assisted Reprod Genet (2007) 24(7):288–95. doi: 10.1007/s10815-007-9148-3
94. Bakela K, Athanassakis I. Soluble major histocompatibility complex molecules in immune regulation: highlighting class II antigens. Immunology (2018) 153(3):315–24. doi: 10.1111/imm.12868
95. Zavazava N, Krönke M. Soluble HLA class I molecules induce apoptosis in alloreactive cytotoxic T lymphocytes. Nat Med (1996) 2(9):1005–10. doi: 10.1038/nm0996-1005
96. Athanassakis I, Vassiliadis S. Interplay between T helper type 1 and type 2 cytokines and soluble major histocompatibility complex molecules: a paradigm in pregnancy. Immunology (2002) 107(3):281–7. doi: 10.1046/j.1365-2567.2002.01518.x
97. Jones K, Ballesteros A, Mentink-Kane M, Warren J, Rattila S, Malech H, et al. PSG9 Stimulates Increase in FoxP3+ Regulatory T-Cells through the TGF-β1 Pathway. PloS One (2016) 11(7):e0158050. doi: 10.1371/journal.pone.0158050
98. Zamorina SA, Raev MB. [The study of human pregnancy specific β1-glycoprotein immunomodulating effects]. Fiziol Cheloveka (2015) 41(1):117–23. doi: 10.1134/S0362119715010144
99. Lafuste P, Robert B, Mondon F, Danan JL, Rossi B, Duc-Goiran P, et al. Alpha-fetoprotein gene expression in early and full-term human trophoblast. Placenta (2002) 23(8-9):600–12. doi: 10.1053/plac.2002.0816
100. Lu CY, Changelian PS, Unanue ER. Alpha-fetoprotein inhibits macrophage expression of Ia antigens. J Immunol (Baltimore Md 1950) (1984) 132(4):1722–7.
101. Fettke F, Schumacher A, Canellada A, Toledo N, Bekeredjian-Ding I, Bondt A, et al. Maternal and Fetal Mechanisms of B Cell Regulation during Pregnancy: Human Chorionic Gonadotropin Stimulates B Cells to Produce IL-10 While Alpha-Fetoprotein Drives Them into Apoptosis. Front Immunol (2016) 7:495:495. doi: 10.3389/fimmu.2016.00495
102. Mellor AL, Lemos H, Huang L. Indoleamine 2,3-Dioxygenase and Tolerance: Where Are We Now? Front Immunol (2017) 8:1360. doi: 10.3389/fimmu.2017.01360
103. Mellor AL, Chandler P, Lee GK, Johnson T, Keskin DB, Lee J, et al. Indoleamine 2,3-dioxygenase, immunosuppression and pregnancy. J Reprod Immunol (2002) 57(1-2):143–50. doi: 10.1016/s0165-0378(02)00040-2
104. Kang JW, Koo HC, Hwang SY, Kang SK, Ra JC, Lee MH, et al. Immunomodulatory effects of human amniotic membrane-derived mesenchymal stem cells. J Veterinary Sci (2012) 13(1):23–31. doi: 10.4142/jvs.2012.13.1.23
105. Hakam MS, Miranda-Sayago JM, Hayrabedyan S, Todorova K, Spencer PS, Jabeen A, et al. Preimplantation Factor (PIF) Promotes HLA-G, -E, -F, -C Expression in JEG-3 Choriocarcinoma Cells and Endogenous Progesterone Activity. Cell Physiol Biochem: Int J Exp Cell Physiol Biochem Pharmacol (2017) 43(6):2277–96. doi: 10.1159/000484378
106. Zare F, Seifati SM, Dehghan-Manshadi M, Fesahat F. Preimplantation Factor (PIF): a peptide with various functions. JBRA Assisted Reproduct (2020) 24(2):214–8. doi: 10.5935/1518-0557.20190082
107. Tong M, Chamley LW. Placental extracellular vesicles and feto-maternal communication. Cold Spring Harb Perspect Med (2015) 5(3):a023028. doi: 10.1101/cshperspect.a023028
108. Garrido-Gimenez C, Alijotas-Reig J. Recurrent miscarriage: causes, evaluation and management. Postgrad Med J (2015) 91(1073):151–62. doi: 10.1136/postgradmedj-2014-132672
109. Samuelsson B. Isolation and identification of prostaglandins from human seminal plasma. 18. Prostaglandins and related factors. J Biol Chem (1963) 238:3229–34.
110. Nimbkar-Joshi S, Katkam RR, Kakar R, Singh P, Chaudhari UK, Manjramkar DD, et al. Proliferation and decidualization of endometrial stromal cells during embryo-attachment stage in bonnet monkeys (Macaca radiata). Cell Tissue Res (2015) 361(2):605–17. doi: 10.1007/s00441-015-2117-8
111. Niringiyumukiza JD, Cai H, Xiang W. Prostaglandin E2 involvement in mammalian female fertility: ovulation, fertilization, embryo development and early implantation. Reprod Biol Endocrinol (2018) 16(1):43. doi: 10.1186/s12958-018-0359-5
112. Tan HN, Liu Y, Diao HL, Yang ZM. Cyclooxygenases and prostaglandin E synthases in preimplantation mouse embryos. Zygote (Cambridge England) (2005) 13(2):103–8. doi: 10.1017/s0967199405003187
113. Shearer GM, Hurtenbach U. Is sperm immunosuppressive in male homosexuals and vasectomized men? Immunol Today (1982) 3(6):153–4. doi: 10.1016/0167-5699(82)90103-7
114. Marcus AJ, Safier LB, Broekman MJ, Ullman HL, Islam N, Sorrell TC, et al. Production of metabolic products of arachidonic acid during cell-cell interactions. J Allergy Clin Immunol (1984) 74(3 Pt 2):338–42. doi: 10.1016/0091-6749(84)90126-x
115. Schjenken JE, Robertson SA. Seminal fluid and immune adaptation for pregnancy–comparative biology in mammalian species. Reprod Domest Anim Zuchthygiene (2014) 49(3):27–36. doi: 10.1111/rda.12383
116. Adefuye AO, Adeola HA, Sales KJ, Katz AA. Seminal Fluid-Mediated Inflammation in Physiology and Pathology of the Female Reproductive Tract. J Immunol Res (2016) 2016:9707252. doi: 10.1155/2016/9707252
117. McMaster MT, Newton RC, Dey SK, Andrews GK. Activation and distribution of inflammatory cells in the mouse uterus during the preimplantation period. J Immunol (Baltimore Md: 1950) (1992) 148(6):1699–705.
118. Troedsson MH, Desvousges A, Alghamdi AS, Dahms B, Dow CA, Hayna J, et al. Components in seminal plasma regulating sperm transport and elimination. Anim Reprod Sci (2005) 89(1-4):171–86. doi: 10.1016/j.anireprosci.2005.07.005
119. Sasanami T, Izumi S, Sakurai N, Hirata T, Mizushima S, Matsuzaki M, et al. A unique mechanism of successful fertilization in a domestic bird. Sci Rep (2015) 5:7700. doi: 10.1038/srep07700
120. Shahnazi M, Nouri M, Mohaddes G, Latifi Z, Fattahi A, Mohammadi M. Prostaglandin E Pathway in Uterine Tissue During Window of Preimplantation in Female Mice Mated With Intact and Seminal Vesicle-Excised Male. Reprod Sci (Thousand Oaks Calif) (2018) 25(4):550–8. doi: 10.1177/1933719117718272
121. Gottlieb C, Svanborg K, Eneroth P, Bygdeman M. Effect of prostaglandins on human sperm function in vitro and seminal adenosine triphosphate content. Fertility Steril (1988) 49(2):322–7. doi: 10.1016/s0015-0282(16)59723-4
122. Aitken RJ, Kelly RW. Analysis of the direct effects of prostaglandins on human sperm function. J Reprod Fertility (1985) 73(1):139–46. doi: 10.1530/jrf.0.0730139
123. Machtinger R, Laurent LC, Baccarelli AA. Extracellular vesicles: roles in gamete maturation, fertilization and embryo implantation. Hum Reprod Update (2016) 22(2):182–93. doi: 10.1093/humupd/dmv055
124. Franz C, Böing AN, Hau CM, Montag M, Strowitzki T, Nieuwland R, et al. Procoagulant tissue factor-exposing vesicles in human seminal fluid. J Reprod Immunol (2013) 98(1-2):45–51. doi: 10.1016/j.jri.2013.02.002
125. Han BC, Xia HF, Sun J, Yang Y, Peng JP. Retinoic acid-metabolizing enzyme cytochrome P450 26a1 (cyp26a1) is essential for implantation: functional study of its role in early pregnancy. J Cell Physiol (2010) 223(2):471–9. doi: 10.1002/jcp.22056
126. Saito S, Tsuda H, Michimata T. Prostaglandin D2 and reproduction. Am J Reprod Immunol (N Y NY: 1989) (2002) 47(5):295–302. doi: 10.1034/j.1600-0897.2002.01113.x
127. Michimata T, Tsuda H, Sakai M, Fujimura M, Nagata K, Nakamura M, et al. Accumulation of CRTH2-positive T-helper 2 and T-cytotoxic 2 cells at implantation sites of human decidua in a prostaglandin D(2)-mediated manner. Mol Hum Reproduct (2002) 8(2):181–7. doi: 10.1093/molehr/8.2.181
128. Kaczmarek MM, Krawczynski K, Filant J. Seminal plasma affects prostaglandin synthesis and angiogenesis in the porcine uterus. Biol Reproduct (2013) 88(3):72. doi: 10.1095/biolreprod.112.103564
129. Robertson SA. Seminal fluid signaling in the female reproductive tract: lessons from rodents and pigs. J Anim Sci (2007) 85(13):E36–44. doi: 10.2527/jas.2006-578
130. Striz I, Brabcova E, Kolesar L, Sekerkova A. Cytokine networking of innate immunity cells: a potential target of therapy. Clin Sci (London England: 1979) (2014) 126(9):593–612. doi: 10.1042/CS20130497
131. Tariverdian N, Siedentopf F, Rücke M, Blois SM, Klapp BF, Kentenich H, et al. Intraperitoneal immune cell status in infertile women with and without endometriosis. J Reprod Immunol (2009) 80(1-2):80–90. doi: 10.1016/j.jri.2008.12.005
132. Yasuda I, Shima T, Moriya T, Ikebuchi R, Kusumoto Y, Ushijima A, et al. Dynamic Changes in the Phenotype of Dendritic Cells in the Uterus and Uterine Draining Lymph Nodes After Coitus. Front Immunol (2020) 11:557720. doi: 10.3389/fimmu.2020.557720
133. Tumala B, Phelps KR, Zhang S, Bhattacharya S, Shornick LP. Prostaglandin D2 Levels Regulate CD103+ Conventional Dendritic Cell Activation in Neonates During Respiratory Viral Infection. Viral Immunol (2018) 31(10):658–67. doi: 10.1089/vim.2018.0090
134. Shao Y, Saredy J, Yang WY, Sun Y, Lu Y, Saaoud F, et al. Vascular Endothelial Cells and Innate Immunity. Arteriosclerosis Thrombosis Vasc Biol (2020) 40(6):e138–52. doi: 10.1161/ATVBAHA.120.314330
135. Brockelsby JC, Anthony FW, Johnson IR, Baker PN. The effects of vascular endothelial growth factor on endothelial cells: a potential role in preeclampsia. Am J Obstetr Gynecol (2000) 182(1 Pt 1):176–83. doi: 10.1016/s0002-9378(00)70510-2
136. Jena MK, Sharma NR, Petitt M, Maulik D, Nayak NR. Pathogenesis of Preeclampsia and Therapeutic Approaches Targeting the Placenta. Biomolecules (2020) 10(6):953. doi: 10.3390/biom10060953
137. Kelly RW. Inflammatory mediators and parturition. Rev Reproduct (1996) 1(2):89–96. doi: 10.1530/ror.0.0010089
138. Phipps E, Prasanna D, Brima W, Jim B. Preeclampsia: Updates in Pathogenesis, Definitions, and Guidelines. Clin J Am Soc Nephrol: CJASN (2016) 11(6):1102–13. doi: 10.2215/CJN.12081115
139. Atallah A, Lecarpentier E, Goffinet F, Doret-Dion M, Gaucherand P, Tsatsaris V. Aspirin for Prevention of Preeclampsia. Drugs (2017) 77(17):1819–31. doi: 10.1007/s40265-017-0823-0
140. Clària J, Serhan CN. Aspirin triggers previously undescribed bioactive eicosanoids by human endothelial cell-leukocyte interactions. Proc Natl Acad Sci U States A (1995) 92(21):9475–9. doi: 10.1073/pnas.92.21.9475
141. Gil-Villa AM, Norling LV, Serhan CN, Cordero D, Rojas M, Cadavid A. Aspirin triggered-lipoxin A4 reduces the adhesion of human polymorphonuclear neutrophils to endothelial cells initiated by preeclamptic plasma. Prostaglandins Leukotrienes Essential Fatty Acids (2012) 87(4-5):127–34. doi: 10.1016/j.plefa.2012.08.003
142. Vallvé-Juanico J, Houshdaran S, Giudice LC. The endometrial immune environment of women with endometriosis. Hum Reprod Update (2019) 25(5):564–91. doi: 10.1093/humupd/dmz018
143. Lai ZZ, Yang HL, Ha SY, Chang KK, Mei J, Zhou WJ, et al. Cyclooxygenase-2 in Endometriosis. Int J Biol Sci (2019) 15(13):2783–97. doi: 10.7150/ijbs.35128
144. Saad-Hossne R, Barretto AB, Siqueira JM, Denadai R. Evaluation of peritoneal endometriosis treatment using intralesional acetylsalicylic acid injection in rabbits. Acta Cirurgica Brasileira (2016) 31(4):227–34. doi: 10.1590/S0102-865020160040000002
145. Yang ZM, Das SK, Wang J, Sugimoto Y, Ichikawa A, Dey SK. Potential sites of prostaglandin actions in the periimplantation mouse uterus: differential expression and regulation of prostaglandin receptor genes. Biol Reproduct (1997) 56(2):368–79. doi: 10.1095/biolreprod56.2.368
146. Lim H, Dey SK. Prostaglandin E2 receptor subtype EP2 gene expression in the mouse uterus coincides with differentiation of the luminal epithelium for implantation. Endocrinology (1997) 138(11):4599–606. doi: 10.1210/endo.138.11.5528
147. Lim H, Paria BC, Das SK, Dinchuk JE, Langenbach R, Trzaskos JM, et al. Multiple female reproductive failures in cyclooxygenase 2-deficient mice. Cell (1997) 91(2):197–208. doi: 10.1016/S0092-8674(00)80402-X
148. Lim H, Gupta RA, Ma WG, Paria BC, Moller DE, Morrow JD, et al. Cyclo-oxygenase-2-derived prostacyclin mediates embryo implantation in the mouse via PPARdelta. Genes Dev (1999) 13(12):1561–74. doi: 10.1101/gad.13.12.1561
149. Mendonça LGD, Rocha LS, Voelz BE, Lima GT, Scanavez ALA, Stevenson JS. Presynchronization strategy using prostaglandin F2α, gonadotropin-releasing hormone, and detection of estrus to improve fertility in a resynchronization program for dairy cows. Theriogenology (2019) 124:39–47. doi: 10.1016/j.theriogenology.2018.09.027
150. Mai J, Virtue A, Shen J, Wang H, Yang XF. An evolving new paradigm: endothelial cells–conditional innate immune cells. J Hematol Oncol (2013) 6:61. doi: 10.1186/1756-8722-6-61
151. Samuchiwal SK, Boyce JA. Role of lipid mediators and control of lymphocyte responses in type 2 immunopathology. J Allergy Clin Immunol (2018) 141(4):1182–90. doi: 10.1016/j.jaci.2018.02.006
152. Marie C, Pitton C, Fitting C, Cavaillon JM. Regulation by anti-inflammatory cytokines (IL-4, IL-10, IL-13, TGFbeta) of interleukin-8 production by LPS- and/or TNFalpha-activated human polymorphonuclear cells. Mediators Inflamm (1996) 5(5):334–40. doi: 10.1155/S0962935196000488
153. Agard M, Asakrah S, Morici LA. PGE(2) suppression of innate immunity during mucosal bacterial infection. Front Cell Infect Microbiol (2013) 3:45. doi: 10.3389/fcimb.2013.00045
154. Robertson SA, Sharkey DJ. The role of semen in induction of maternal immune tolerance to pregnancy. Semin Immunol (2001) 13(4):243–54. doi: 10.1006/smim.2000.0320
155. Weiner HL. Oral tolerance: immune mechanisms and the generation of Th3-type TGF-beta-secreting regulatory cells. Microbes Infect (2001) 3(11):947–54. doi: 10.1016/s1286-4579(01)01456-3
156. Parhar RS, Yagel S, Lala PK. PGE2-mediated immunosuppression by first trimester human decidual cells blocks activation of maternal leukocytes in the decidua with potential anti-trophoblast activity. Cell Immunol (1989) 120(1):61–74. doi: 10.1016/0008-8749(89)90174-3
157. Zhang Y, Wang Y, Wang XH, Zhou WJ, Jin LP, Li MQ. Crosstalk between human endometrial stromal cells and decidual NK cells promotes decidualization in vitro by upregulating IL−25. Mol Med Rep (2018) 17(2):2869–78. doi: 10.3892/mmr.2017.8267
158. Vacca P, Cantoni C, Vitale M, Prato C, Canegallo F, Fenoglio D, et al. Crosstalk between decidual NK and CD14+ myelomonocytic cells results in induction of Tregs and immunosuppression. Proc Natl Acad Sci U States A (2010) 107(26):11918–23. doi: 10.1073/pnas.1001749107
159. Mjösberg J, Berg G, Ernerudh J, Ekerfelt C. CD4+ CD25+ regulatory T cells in human pregnancy: development of a Treg-MLC-ELISPOT suppression assay and indications of paternal specific Tregs. Immunology (2007) 120(4):456–66. doi: 10.1111/j.1365-2567.2006.02529.x
160. Mayoral-Andrade G, Pérez-Campos-Mayoral L, Majluf-Cruz A, Perez-Campos Mayoral E, Perez Campos Mayoral C, Rocha-Núñez A, et al. Reduced platelet aggregation in women after intercourse: a possible role for the cyclooxygenase pathway. Clin Exp Pharmacol Physiol (2017) 44(8):847–53. doi: 10.1111/1440-1681
161. Lwaleed BA, Greenfield R, Royle E, Birch B, Cooper AJ, Seminal Factor VIII. and von Willebrand Factor: a possible role of the conventional clotting system in human semen? Int J Androl (2005) 28(1):31–8. doi: 10.1111/j.1365-2605.2004.00508.x
162. Tomokiyo K, Kamikubo Y, Hanada T, Araki T, Nakatomi Y, Ogata Y, et al. Von Willebrand factor accelerates platelet adhesion and thrombus formation on a collagen surface in platelet-reduced blood under flow conditions. Blood (2005) 105(3):1078–84. doi: 10.1182/blood-2004-05-1827
163. Bastida E, Escolar G, Ordinas A, Sixma JJ. Fibronectin is required for platelet adhesion and for thrombus formation on subendothelium and collagen surfaces. Blood (1987) 70(5):1437–42. doi: 10.1182/blood.V70.5.1437.bloodjournal7051437
164. Asch E, Podack E. Vitronectin binds to activated human platelets and plays a role in platelet aggregation. J Clin Investigat (1990) 85(5):1372–8. doi: 10.1172/JCI114581
165. Xu LC, Bauer JW, Siedlecki CA. Proteins, platelets, and blood coagulation at biomaterial interfaces. Colloids Surf B Biointerf (2014) 124:49–68. doi: 10.1016/j.colsurfb.2014.09.040
166. Etulain J, Martinod K, Wong SL, Cifuni SM, Schattner M. Wagner DD. P-selectin promotes neutrophil extracellular trap formation in mice. Blood (2015) 126(2):242–6. doi: 10.1182/blood-2015-01-624023
167. Singbartl K, Forlow SB, Ley K. Platelet, but not endothelial, P-selectin is critical for neutrophil-mediated acute postischemic renal failure. FASEB J Off Publ Fed Am Soc Exp Biol (2001) 15(13):2337–44. doi: 10.1096/fj.01-0199com
168. Stavrou EX, Fang C, Bane KL, Long AT, Naudin C, Kucukal E, et al. and uPAR upregulate neutrophil functions to influence wound healing. J Clin Invest (2018) 128(3):944–59. doi: 10.1172/JCI92880
169. Gaertner F, Massberg S. Blood coagulation in immunothrombosis-At the frontline of intravascular immunity. Semin Immunol (2016) 28(6):561–9. doi: 10.1016/j.smim.2016.10.010
170. Carestia A, Kaufman T, Rivadeneyra L, Landoni VI, Pozner RG, Negrotto S, et al. Mediators and molecular pathways involved in the regulation of neutrophil extracellular trap formation mediated by activated platelets. J Leukocyte Biol (2016) 99(1):153–62. doi: 10.1189/jlb.3A0415-161R
171. Zurier RB, Weissmann G, Hoffstein S, Kammerman S, Tai HH. Mechanisms of lysosomal enzyme release from human leukocytes. II. Effects of cAMP and cGMP, autonomic agonists, and agents which affect microtubule function. J Clin Invest (1974) 53(1):297–309. doi: 10.1172/JCI107550
172. Marcus AJ, Broekman MJ, Safier LB, Ullman HL, Islam N, Serhan CN, et al. Production of arachidonic acid lipoxygenase products during platelet-neutrophil interactions. Clin Physiol Biochem (1984) 2(2-3):78–83.
173. Turcotte C, Zarini S, Jean S, Martin C, Murphy RC, Marsolais D, et al. The endocannabinoid metabolite prostaglandin E 2 (PGE 2)-glycerol inhibits human neutrophil functions: Involvement of its hydrolysis into PGE 2 and EP receptors. J Immunol (2017) 198(8):3255–63. doi: 10.4049/jimmunol.1601767
174. Pap E, Pállinger E, Falus A, Kiss AA, Kittel A, Kovács P, et al. T lymphocytes are targets for platelet- and trophoblast-derived microvesicles during pregnancy. Placenta (2008) 29(9):826–32. doi: 10.1016/j.placenta.2008.06.006
175. Vazquez J, Chavarria M, Li Y, Lopez GE, Stanic AK. Computational flow cytometry analysis reveals a unique immune signature of the human maternal-fetal interface. Am J Reprod Immunol (N Y NY 1989) (2018) 79(1):10.1111/aji.12774. doi: 10.1111/aji.12774
176. Nadkarni S, Smith J, Sferruzzi-Perri AN, Ledwozyw A, Kishore M, Haas R, et al. Neutrophils induce proangiogenic T cells with a regulatory phenotype in pregnancy. Proc Natl Acad Sci U S A (2016) 113(52):E8415–24. doi: 10.1073/pnas.1611944114
177. Hahn S, Giaglis S, Hoesli I, Hasler P. Neutrophil NETs in reproduction: from infertility to preeclampsia and the possibility of fetal loss. Front Immunol (2012) 3:362:362. doi: 10.3389/fimmu.2012.00362
178. Alghamdi AS, Lovaas BJ, Bird SL, Lamb GC, Rendahl AK, Taube PC, et al. Species-specific interaction of seminal plasma on sperm–neutrophil binding. Anim Reprod Sci (2009) 114(4):331–44. doi: 10.1016/j.anireprosci.2008.10.015
179. Selva KJ, Juno JA, Worley MJ, Chung AW, Tachedjian G, Kent SJ, et al. Effect of seminal plasma on functions of monocytes and granulocytes. AIDS Res Hum Retroviruses (2019) 35(6):553–6. doi: 10.1089/AID.2018.0219
180. Ricciotti E, Fitzgerald GA. Prostaglandins and inflammation. Arterioscler Thromb Vasc Biol (2011) 31(5):986–1000. doi: 10.1161/ATVBAHA.110.207449
181. Agard M, Asakrah S, Morici LA. PGE2 suppression of innate immunity during mucosal bacterial infection. Front Cell Infect Microbiol (2013) 3:45. doi: 10.3389/fcimb.2013.00045
182. Bry K, Hallman M. Prostaglandins, inflammation, and preterm labor. J Perinatol (1989) 9(1):60–5.
183. Pang XL, Yin TL, Yan WJ, Li J, He F, Yang J. Molecular detection of uterine innate lymphoid cells in the immunological mouse model of pregnancy loss. Int Immunopharmacol (2019) 68:1–6. doi: 10.1016/j.intimp.2018.12.046
184. Bartemes K, Chen CC, Iijima K, Drake L, Kita H. IL-33-Responsive group 2 innate lymphoid cells are regulated by female sex hormones in the uterus. J Immunol (2018) 200(1):229–36. doi: 10.4049/jimmunol.1602085
185. Mori M, Bogdan A, Balassa T, Csabai T, Szekeres-Bartho J. The decidua-the maternal bed embracing the embryo-maintains the pregnancy. Semin Immunopathology (2016) 38(6):635–49. doi: 10.1007/s00281-016-0574-0
186. Xu Y, Romero R, Miller D, Silva P, Panaitescu B, Theis KR, et al. Innate lymphoid cells at the human maternal-fetal interface in spontaneous preterm labor. Am J Reprod Immunol (2018) 79(6):e12820. doi: 10.1111/aji.12820
187. Tilley SL, Coffman TM, Koller BH. Mixed messages: modulation of inflammation and immune responses by prostaglandins and tromboxanes. J Clinc Invest (2001) 108(1):15–23. doi: 10.1172/JCI200113416
188. Shishikura K, Horiuchi T, Sakata N, Trinh DA, Shirakawa R, Kimura T, et al. Prostaglandin E 2 inhibits neutrophil extracellular trap formation through production of cyclic AMP. Br J Pharmacol (2016) 173(2):319–31. doi: 10.1111/bph.13373
189. Maric J, Ravindran A, Mazzurana L, Björklund ÅK, Van Acker A, Rao A, et al. Prostaglandin E2 suppresses human group 2 innate lymphoid cell function. J Allergy Clin Immunol (2018) 141(5):1761–73.e6. doi: 10.1016/j.jaci.2017.09.050
190. Maazi H, Akbari O. Type two innate lymphoid cells: the Janus cells in health and disease. Immunol Rev (2017) 278(1):192–206. doi: 10.1111/imr.12554
191. Carrascal L, Nunez-Abades P, Ayala A, Cano M. Role of Melatonin in the Inflammatory Process and its Therapeutic Potential. Curr Pharm Design (2018) 24(14):1563–88. doi: 10.2174/1381612824666180426112832
192. Berbets A, Koval H, Barbe A, Albota O, Yuzko O. Melatonin decreases and cytokines increase in women with placental insufficiency. J Maternal-fetal Neonatal Med: Off J Eur Assoc Perinatal Med Fed Asia Oceania Perinatal Soc Int Soc Perinatal Obstetricians (2019), 1:1–6. doi: 10.1080/14767058.2019.1608432
193. Sandyk R, Anastasiadis PG, Anninos PA, Tsagas N. The pineal gland and spontaneous abortions: implications for therapy with melatonin and magnetic field. Int J Neurosci (1992) 62(3-4):243–50. doi: 10.3109/00207459108999775
194. Ogiwara K, Takahashi T. A Dual Role for Melatonin in Medaka Ovulation: Ensuring Prostaglandin Synthesis and Actin Cytoskeleton Rearrangement in Follicular Cells. Biol Reprod (2016) 94(3):64. doi: 10.1095/biolreprod.115.133827
195. Scarinci E, Tropea A, Notaristefano G, Arena V, Alesiani O, Fabozzi SM, et al. “Hormone of darkness” and human reproductive process: direct regulatory role of melatonin in human corpus luteum. J Endocrinol Investigat (2019) 42(10):1191–7. doi: 10.1007/s40618-019-01036-3
196. Espino J, Macedo M, Lozano G, Ortiz Á, Rodríguez C, Rodríguez AB, et al. Impact of Melatonin Supplementation in Women with Unexplained Infertility Undergoing Fertility Treatment. Antioxid (Basel Switzerland) (2019) 8(9):338. doi: 10.3390/antiox8090338
197. Szymanska M, Blitek A. Endometrial and conceptus response to exogenous progesterone treatment in early pregnant gilts following hormonally-induced estrus. Anim Reprod Sci (2016) 174:56–64. doi: 10.1016/j.anireprosci.2016.09.005
198. Di Pietro C, Cicinelli E, Guglielmino MR, Ragusa M, Farina M, Palumbo MA, et al. Altered transcriptional regulation of cytokines, growth factors, and apoptotic proteins in the endometrium of infertile women with chronic endometritis. Am J Reprod Immunol (N Y NY 1989) (2013) 69(5):509–17. doi: 10.1111/aji.12076
Keywords: prostaglandins, PGE2, platelets, polymorphonuclear leukocyte, group 2 innate lymphoid cells, embryo tolerance
Citation: Mayoral Andrade G, Vásquez Martínez G, Pérez-Campos Mayoral L, Hernández-Huerta MT, Zenteno E, Pérez-Campos Mayoral E, Martínez Cruz M, Martínez Cruz R, Matias-Cervantes CA, Meraz Cruz N, Romero Díaz C, Cruz-Parada E and Pérez-Campos E (2020) Molecules and Prostaglandins Related to Embryo Tolerance. Front. Immunol. 11:555414. doi: 10.3389/fimmu.2020.555414
Received: 24 April 2020; Accepted: 19 October 2020;
Published: 19 November 2020.
Edited by:
Marie-Pierre Piccinni, University of Florence, ItalyReviewed by:
Gerard Chaouat, INSERM U976 Immunologie, Dermatologie, Oncologie, FranceMatthew Cook, Australian National University, Australia
Copyright © 2020 Mayoral Andrade, Vásquez Martínez, Pérez-Campos Mayoral, Hernández-Huerta, Zenteno, Pérez-Campos Mayoral, Martínez Cruz, Martínez Cruz, Matias-Cervantes, Meraz Cruz, Romero Díaz, Cruz-Parada and Pérez-Campos. This is an open-access article distributed under the terms of the Creative Commons Attribution License (CC BY). The use, distribution or reproduction in other forums is permitted, provided the original author(s) and the copyright owner(s) are credited and that the original publication in this journal is cited, in accordance with accepted academic practice. No use, distribution or reproduction is permitted which does not comply with these terms.
*Correspondence: Eduardo Pérez-Campos, cGVyZXpjYW1wb3NAcHJvZGlneS5uZXQubXg=