- 1Department of Infectious and Parasitic Diseases, Faculdade de Medicina, University of São Paulo, São Paulo, Brazil
- 2Department of Medicine, Divisions of Molecular Medicine and Nephrology, Faculdade de Medicina, University of São Paulo, São Paulo, Brazil
- 3Laboratory of Immunology (LIM 48), Hospital das Clínicas, Faculdade de Medicina, University of São Paulo, São Paulo, Brazil
- 4Heart Institute, Hospital das Clínicas, Faculdade de Medicina, University of São Paulo, São Paulo, Brazil
- 5Division of Infectious Diseases, Hospital das Clinicas, Faculdade de Medicina, University of São Paulo, São Paulo, Brazil
- 6Instituto de Medicina Tropical, University of São Paulo, São Paulo, Brazil
- 7Departament of Immunology, Instituto de Ciências Biomédicas (ICB), University of São Paulo, São Paulo, Brazil
Background: Chagas disease caused by Trypanosoma cruzi (T. cruzi) affects approximately six million individuals worldwide. Clinical manifestations are expected to occur due to the parasite persistence and host immune response. Herein we investigated potential associations between IL1B, IL6, IL17A, or IL18 polymorphism profiles and cardiomyopathy or T. cruzi parasitemia, as well as the impact of HIV infection on cardiopathy.
Methods: Two hundred twenty-six patients and 90 control individuals were analyzed. IL1B rs1143627 T>C, IL6 rs1800795 C>G, IL17A rs2275913 G>A, IL18 rs187238 C>G, and IL18 rs1946518 C>A SNVs were analyzed by real-time PCR and T. cruzi parasitemia by PCR.
Results: Our data revealed association between a cytokine gene polymorphism and parasitemia never previously reported. The IL6 rs1800795 CG genotype lowered the risk of positive parasitemia (OR = 0.45, 95% CI 0.24–0.86, P = 0.015). Original findings included associations between IL17A rs2275913 AA and IL18 s1946518 AA genotypes with decreased risk of developing cardiomyopathy (OR = 0.27, 95% CI 0.07–0.97, P = 0.044; and OR = 0.35, 95% CI 0.14–0.87, P = 0.023, respectively). IL18 rs1946518 AA and IL1B rs1143627 TC were associated with reduced risk for cardiomyopathy severity, including NYHA (New York Heart Association) class ≥ 2 (OR = 0.21, 95% CI 0.06–0.68, P = 0.009; and OR = 0.48, 95% CI 0.24–0.95, P = 0.036, respectively) and LVEF (left ventricular ejection fraction) <45% for IL18 rs1946518 AA (OR = 0.22, 95% CI 0.05–0.89, P = 0.034). A novel, unexpected protective effect of HIV infection against development/progression of cardiomyopathy was identified, based on a lower risk of developing cardiopathy (OR = 0.48, 95% CI 0.23–0.96, P = 0.039), NYHA class ≥ 2 (OR = 0.15, 95% CI 0.06–0.39, P < 0.001), and LVEF < 45% (OR = 0.03, 95% CI 0.00–0.25, P = 0.001). Digestive involvement was negatively associated with NYHA ≥ 2 and LVEF < 45% (OR = 0.20, 95% CI 0.09–0.47, P < 0.001; and OR = 0.24, 95% CI 0.09–0.62, P = 0.004, respectively).
Conclusions: Our data support a protective role of IL17A AA, IL18 AA, and IL1B TC genotypes against development/progression of cardiomyopathy and a modulatory effect of the IL6 CG genotype on the risk of parasitemia in Chagas disease. Notably, HIV infection was shown to protect against development/progression of cardiopathy, potentially associated with a synergistic effect of HIV and highly active antiretroviral therapy (HAART), attenuating a Th1-mediated response in the myocardium. This proposed hypothesis requires confirmation, however, in larger and more comprehensive future studies.
Introduction
Chagas disease, a potentially life-threatening illness caused by the protozoan Trypanosoma cruzi (T. cruzi) and endemic in Latin America, affects approximately 6 million individuals worldwide. The natural course of the infection comprises acute and chronic phases. A clinically relevant acute form is observed in only 1/30 infected people, including fever, adenopathy, palpebral or legs edema and/or hepatosplenomegaly and, less often, myocarditis and meningoencephalitis. In the chronic phase, on the other hand, ~30% of the patients develop the cardiac form, expressed as arrhythmia, heart failure, and/or thromboembolic events. The chronic phase also includes a digestive form, which comprises dysphagia and/or constipation in 10–15% of the cases and, in ~5% of them, a cardio-digestive form. The indeterminate form, in turn, represented by asymptomatic patients with normal electrocardiogram and thorax and digestive tract X-rays, constitutes the most frequent chronic form, affecting 60–70% of the referred group (1, 2).
Chagas cardiopathy is a major cause of cardiovascular-related mortality within the 30–50-year age range in Latin American endemic areas (1, 3). Previous studies have recognized the fundamental role of host features in Chagas disease pathophysiology, revealing different immunological profiles in patients affected and not affected by cardiopathy (4). While the development of cardiopathy has been linked to a Th1 response in heart tissue associated with mononuclear cell infiltrate, cardiomyocyte damage, and fibrosis, a Th2 response predominates in the indeterminate form (5, 6). Reports of higher levels/expression of IL-6, IL1β, TNFα, and IFNγ in the cardiac form (7, 8) and IL6 and/IL1β or C-reactive protein in the cardiac and/or cardio-digestive forms (9, 10) support a potential role for such cytokines in the adaptative immune response at heart tissue damaging process. IL6 and IL1β could be considered, therefore, a marker of cardiac lesion. In contrast, the finding of higher plasma levels of IL17A directly associated with better systolic function in the indeterminate form suggests a protective role for this cytokine against the development of clinically relevant chronic Chagas cardiopathy, despite inverse association with diastolic performance indices (11, 12).
Previous studies in patients with indeterminate and cardiac forms suggested a role for T reg cells in the modulation of inflammatory immune responses associated with the cardiac form (6, 13). Increased number of Foxp3+, CD25+, CD4+ T cells, T reg cells that stimulate secretion of IL-17 A, IL-10, and granzyme B, was observed in the indeterminate form, in association with less inflammation and better myocardium function. In contrast, more pronounced immune responses to the parasite, associated with higher levels of inflammatory cytokines and myocardial lesion, correlated with higher densities of IL6+, IFNγ+, TNFα+, CTLA4+ T reg cells. Such findings suggest a role for T reg cells in controlling the exacerbated immune response and morbidity as well as mortality in T. cruzi infection, likely by stimulating Th17 cells and modulating killing effector cells (14).
An important role in the control of cardiac inflammatory lesions and remodeling has been proposed for IL18 (15, 16). This cytokine stimulates IFNγ expression independently of IL12, allowing an IL18-IFNγ cross-talk at the myocardium level during T. cruzi infection (17). IL18 upregulation and correlation between IL18 messenger RNA (mRNA) and IFNγ expression have in fact been reported in human Chagas heart tissue (6, 18).
T. cruzi parasitemia is another major factor implicated in the progression of chronic Chagas cardiopathy, even if intermittent and low at this disease stage (19–22). Available reports, however, are controversial on this point (23–25). Cytokines have also been shown to control T. cruzi parasitic burden and myocardial inflammatory lesions in both chronic and acute phases of murine infection. In chronic infection induced with the Colombian strain in IL18-KO mice, a significant reduction in the myocardium parasitic load was observed (26). Acute infections of IL17A-KO mice, on the other hand, were associated with lack of activation of immune-related cells that are critical for the killing of T. cruzi (27) and with increased mortality and higher-degree myocarditis. Variable parasitic loads were detected, however, depending on the mouse line and the parasite strain (27, 28). This finding unraveled a protective effect of IL17 A, evinced by the association between better cardiac function and higher plasma levels of IL17A in patients without cardiomyopathy (11, 12). In addition, in acute murine infection IL6 was shown to drive the survival of T. cruzi infected cardiomyocytes. IL6-KO mice lack the innate immunity crucial for host survival and rapidly die with high levels of IL1 β and inflammatory products, a process reverted by adding recombinant IL6 (29). This finding suggests a critical role for IL6 in modulating inflammatory lesions. Due to the modulating effect of IL6 on Chagas disease cardiomyopathy, the data on human disease likely represent a less severe degree of this inflammation (7, 10).
In immunosupressed patients with HIV infection, elevated IL18 serum concentrations have been reported to influence both Aids and the course of associated infections (30). Interestingly, whereas Chagas disease reactivation does not occur in immunocompetent subjects, this illness natural history is changed in HIV infected patients, being associated with increased morbidity and mortality (31).
As part of the genetic assessment of cytokine roles in Chagas disease, single nucleotide variants (SNVs) associated with various cytokine genes have been previously analyzed in T. cruzi infection and cardiopathy progression. No significant association involving the IL6 SNV rs1800795 −174 C>G was identified in Colombia (32), in a context where the G allele has been associated with higher IL6 levels (33, 34) and patients with heart Chagas disease have been found to present higher IL6 levels (7, 10). Positive findings, in turn, included association between increased risk of cardiomyopathy and the CT haplotype for the IL1B −31 T>C and +3954 C>T (rs1143634, synonymous coding sequence variant) polymorphisms in Colombia (35). The possible proinflammatory nature of this haplotype (35) is unclear, since the IL1B −31 T allele has been associated with increased IL1β secretion (36), and other haplotype TCT, for IL1B −511 C>T, IL1B −31 T>C, and IL1B +3954 C>T, in turn, has been considered a proinflammatory haplotype, with a predominant role of ILB −511 C>T (37). Moreover, association between cardiomyopathy and the IL1B +5810 G>A (rs1143633, intronic SNV) G allele and GG genotype was also identified, despite no established effect of this allele or genotype on IL1β production (35). It is possible, however, that other biological effects or linkage disequilibrium with other polymorphisms related to functional significance may exist. By comparing seronegative individuals with seropositive cardiac patients in Colombia, a study suggested protection against T. cruzi infection and development of cardiopathy for patients that carry the C allele of IL17A rs8193036 C>T (promoter SNV) (38). These data were a priori not expected, since the C allele has been associated with lower IL17A mRNA expression (39) and previous reports revealed a role of IL17A in resistance to T. cruzi in infected mice (27) and higher IL17A levels in patients with no cardiopathy (11, 12). It should be noted, however, that this study conducted with Colombian patients shows that the IL17A rs8193036 SNV is probably in linkage disequilibrium with some causative variant that modulates IL17A production (38). This investigation also found no association between the IL17A rs7747909 G>A 3’ UTR SNV and Chagas cardiopathy and between two other IL17A promoter SNVs (rs4711998 and rs3819024) or IL17A rs2275913 and heart disease, in a context where the latter A allele is associated with increased IL17 A levels (40, 41). A previous study, in turn, reported association of the IL17A rs2275913 G>A (promoter SNV) A allele and AA genotype with chronic cardiomyopathy in Brazil (42). This observation disagrees with previous studies that detected association between higher IL17A levels and absence of cardiomyopathy (11, 12) and are not in line with the often reported association between the A allele and increased IL17A levels (40, 41). Associations between T. cruzi infection and the IL18 rs187238 C>A (promoter SNV) C, rs360719 A>G (promoter SNV) G, rs2043055 A>G (intronic SNV) A, and rs1946518 C>A (promoter SNV) A alleles were also found in Colombia, a finding mainly driven by the rs360719 G allele (43). Of note, this observation was confirmed in a larger cohort in Latin America (44). Importantly, another study detected association between the rs360719 G carrier status and higher serum IL18 mRNA expression in healthy individuals (45) while an additional report revealed increased IL18 mRNA expression at the myocardium level in Chagas disease cardiomyopathy (18). In addition, the IL18 rs2043055 G allele was found to associate with absence of cardiomyopathy in Colombia (44). In Brazil the IL18 rs2043055 AG genotype was more frequent in patients with a moderate than a severe cardiac phenotype. This study also suggested that it is possibly that this intronic SNV is in linkage disequilibrium with another variant functionally relevant (46). This SNV, however, has not yet been shown to affect IL18 production.
While meaningful progress has been made in linking cytokine gene polymorphisms to Chagas disease mechanisms, associations between cytokine-related SNVs with different clinical scenarios of this disease remain largely limited. Establishing their consequences in the corresponding cytokine circulating and local levels, moreover, as well as their implications on specific biological pathways at the host environment, comprise even more complexes problems to be addressed. In spite of such challenges, thoughtful and well supported hypotheses can be made to progressively add pieces to this ongoing puzzle. In this context, association studies involving appropriate cytokine-related SNVs and strategic immune-affected medical conditions, in one side, and key Chagas phenotypes, on the other side, should be encouraged as potentially significant contributors to the comprehension of this disease pathogenesis.
Facing the biological and clinical scenarios just described, in the current study we investigated potential associations between SNVs linked to IL1B, IL6, IL17A, or IL18 and the risks of positive T. cruzi parasitemia or development/progression of cardiomyopathy. With the assumption that immunocompromised conditions frequently associated with Chagas disease could potentially bring key mechanistic and clinical insights to the understanding of this illness, we also analyzed possible associations involving HIV infection and Chagas disease phenotypes. Surprisingly, our data revealed that positiveness for HIV protected against the development and/or progression of cardiomyopathy. We also describe novel associations between specific IL18, IL17A, and IL1B variant profiles and the risk of cardiomyopathy as well as between a specific IL6 polymorphic genotype and the risk of positive T. cruzi parasitemia.
Patients, Materials, and Methods
Study Subjects
Our cohort included 206 patients with Chagas disease: 78 from the Heart Institute of Hospital das Clínicas da Faculdade de Medicina, University of São Paulo (HCFMUSP), and 129 from the Infectious Diseases Division of HCFMUSP, 49 of whom with HIV co-infection. These 49 cases were followed at Serviço de Extensão e Atendimento aos Paciente com Infecção por HIV/Aids of the same Division. All participants were adults. The diagnosis of trypanosomiasis was based on the 2nd Brazilian Guidelines for Chagas Diseases (1). Two positive tests of the following ones, therefore, were used to establish the diagnosis: ELISA—enzyme linked immunosorbent assay, indirect immunofluorescence, and hemagglutination (47). All patients from the Infectious Diseases Division with positive epidemiology were sent to the HIV/Aids Clinic, where HIV infection was confirmed by ELISA and immunoblot (31). Patients from the Heart Institute were tested for HIV as previously described (31). When included in the current protocol, 26 of the 49 HIV patients were not on regular HAART therapy or had therapeutic failure due to antiretroviral resistance (three patients). In 23 of them, the median viral load was 14,000 (2,618–100,000, 25th–75th percentiles) DNA viral copies/µl while the median CD4 count was 340 (93–510) cells/mm3. Data from 23 patients under HAART for 1 to >5 years showed a median viral load of 0.0 (0.0–0.0) DNA viral copies/µl. In these 23 patients, the median CD4 count was 631 (439–715) cells/mm3.
The patients underwent electrocardiography, echocardiography, and thorax, esophagus, and colon radiological examinations, being then classified within one of the clinical forms of Chagas disease (1). Individuals without signs and symptoms and with no alteration in the mentioned tests were classified as having the indeterminate form (n = 51), patients with abnormalities suggestive of Chagas disease on electrocardiography or on dynamic electrocardiography and without digestive involvement as with the cardiac form (n = 96), the cases with abnormal findings on esophagus and/or colon without Chagas cardiac manifestation as presenting the digestive form (n = 32). Twenty-seven patients presented both digestive and cardiac alterations, constituting the cardio-digestive group. Cardiac involvement was detected in 123 patients (cardiac and cardiac/digestive forms) while heart disease was not identified in 83 patients, a group composed of individuals with the indeterminate and digestive forms.
Patients with cardiomyopathy were evaluated for heart failure according to the New York Heart Association (NYHA) classification (48), which includes the following criteria: class 1. Patients with cardiac disease without limitation of physical activity; class 2. Patients with cardiac disease with mild limitation of physical activity and symptomatic in routine physical activity; class 3. Patients with cardiac disease with marked limitation of physical activity and symptomatic in less than ordinary physical activity; and class 4. Patients with cardiac disease and unable to carry any physical activity without discomfort, symptoms may be present even at rest. For the purposes of our study, however, Chagas patients were classified according to two different scenarios related to their cardiac status, being divided in two groups in each of the cases: A) based on the NYHA classification/absence of cardiopathy—group 1: patients without cardiopathy (no CA) and with mild cardiopathy (NYHA 1), and group 2: patients with more severe cardiopathy (NYHA ≥ 2); and B) Based on the left ventricular ejection fraction (LVEF) yielded by echocardiography—group 1: patients with LVEF ≥ 45%, and group 2: patients with LVEF < 45%.
The control group for the SNV analyses consisted of 90 healthy individuals with negative serological tests for T. cruzi antigens. Controls and patients comprised a total population of 296 individuals, including 149 females and 147 males: 215 white and 73 non-white. This classification was not available for eight control individuals. Among the 206 patients included in this study, 135 were classified within these groups according to self-declaration; 71 of them, in turn, following a distinct institutional policy, were classified according to their government-issued identification document or by the hospital employee who processed their hospital registration.
Ethics Statement
The present study was approved by the Ethics Committee for Research Project Analysis of Hospital das Clínicas da Faculdade de Medicina da Universidade de São Paulo (HCFMUSP), São Paulo, Brazil (CAPPesq 0174/11). All subjects gave written informed consent in accordance with the Declaration of Helsinki.
Evaluation of Parasitemia
Patients’ peripheral blood samples were collected in EDTA (ethylenediaminetetraacetic acid) tubes. DNA extraction was performed using the QIAmp DNA Mini Kit (Qiagen). DNA concentration and purity were analyzed with a spectrophotometer. The parasitemia status was determined employing a qualitative polymerase chain reaction (PCR) performed with the S35/S36 primers designed to the kinetoplastic DNA of T. cruzi (Invitrogen, Thermo Fisher Scientific, Carlsbad, CA, USA), as previously described (49).
DNA Extraction and Genotyping
In most patients genomic DNA was extracted from peripheral blood samples using the QIAampTM DNA Mini Kit (Qiagen, Hilden, Germany). For the cases the DNA concentration was inappropriately low, an additional extraction was carried out using the salt precipitation method (DTAB/CTAB; Sigma-Aldrich, Merck, St. Louis, MO, USA) (50). The DNA samples underwent spectrophotometric evaluation to assess yield and purity. SNVs were investigated by real-time PCR with the TaqMan® Genotyping Master Mix assay and the corresponding SNV-specific primers in the StepOnePlus platform (Applied Biosystems, Thermo Fisher Scientific, Foster City, CA, USA). The investigated SNPs included IL1B −31 rs1143627 T>C, IL6 −174 rs1800795 C>G, IL17A −152 rs2275913 G>A, IL18 −137 rs187238 C>G, and IL18 −607 rs1946518 C>A.
Serum IL-18 Quantification
IL-18 levels were measured using commercial ELISA Kits (R&D Systems, Minneapolis, MN, USA) according to the manufacturer’s instructions. Sera from 43 patients, 15 HIV-infected and 28 non-infected, were stored at −70°C. Twenty-four of such patients had cardiac disease. At the time of blood collection, the median viral load in the 15 HIV-infected patients was 0.0 DNA copies/µl. In 14 of them the median of CD4 count was 873.5 (769.8–974.0) cells/mm3. Three of the 15 HIV-infected patients were not on regular antiretroviral therapy. Two had detectable viremia, with one of them displaying a CD4 count below 350 cells/mm3. The third is an HIV-1-infected patient with an undetectable viral load and high CD4 level despite the absence of HAART therapy, belonging to the rare group named as “elite suppressor” (51).
Statistical Analyses
The calculations of Hardy-Weinberg equilibrium (HWE) were performed using chi square tests in MS Excel 365 (Microsoft Corporation, Redmond, WA). Multiple contingency analyses were performed to investigate potential associations among elected dependent variables and covariates. Fisher’s exact test was used for 2x2 contingency tables; otherwise the chi-square test was applied. When chi square test was used and was applicable, we performed a post-hoc test using standardized and adjusted residues with the Bonferroni correction. The P values obtained with single association tests (Fisher exact test or Pearson chi square test) were used as triage to elect candidate variables for multivariable analyses. We included in the logistic regression all variables that reached P < 0.2 in the single variable association test with the dependent variable. In the genetic models, when more than one model reached P < 0.2, we assumed the model with lower P value as the best-fit model and included it in the logistic regression analysis. To increase the modeling performance, continuous variables were categorized using a ROC (receiving operator characteristic) curve and clinical/biological criteria. Some variables presented missing values in 1–20% of cases and displayed a non-monotone missing pattern. In such cases, we performed multiple imputations including 100 imputed values for each missing one (52). This step was accomplished using MCMC (Markov chain-Monte Carlo simulation) for value imputation. The genotypes were clustered according to four possible models of genetic effects: additive (each genotype was analyzed separately), dominant (the presence of at least one SNV allele is associated with the effect), heterozygous (the heterozygous genotype is associated with the effect on phenotype), and recessive (the presence of SNV homozygosity is associated with the effect on phenotype). We adopted the best-fit model in the chi-square/Pearson exact test analyses, defined by the most significant P-value.
Since rs1946518 and rs187238 are just 470 bp apart from each other in the IL18 promoter, we investigated linkage disequilibrium between these two SNVs using the Haploview software (53) and evaluated potential associations involving the haplotypes.
Chagas cardiopathy, NYHA (New York Heart Association) score, LVEF, and parasitemia were selected upfront as dependent variables for the multiple logistic regressions. Since there was potential interference of the clinical form in the analyzed endpoints, we considered the digestive form as an independent variable in addition to traditional potential confounding factors. To address this point, such a variable was included in the triage as a potential predictor in the model applied to the logistic regression analysis.
Continuous variables were tested for normality with the Shapiro-Wilk test. Continuous parametric variables were analyzed using t test to compare two groups, with the Welch correction if the Levine test pointed to unequal variance between the groups. Continuous non-parametric variables were analyzed using the Mann-Whitney U test when comparing two groups, or Kruskal-Wallis test when comparing more than two groups, followed by post-hoc analysis with the Bonferroni correction. Categorical variables were compared using the chi-square or Fisher exact test. Categorical variables are expressed as the number of cases and percentages while continuous variables as mean ± standard deviation when parametric, and median (25–75% range) when non-parametric. We accepted an α risk of 5% in this exploratory research. The statistical analyses were run in SPSS 24.
Results
Single Nucleotide Variant Genotype and Allele Distribution in the Analyzed Patient Population and Potential Implications
The studied Chagas disease patient population presented a balanced gender composition, predominance of whites and a median age of 50 (42-60) years (Table 1). Notably, 49 patients (23.8%) had HIV co-infection. The distribution of SNV-related genotypes, additional clinical features and the Brazilian state of birth are shown in Supplementary Table 1.
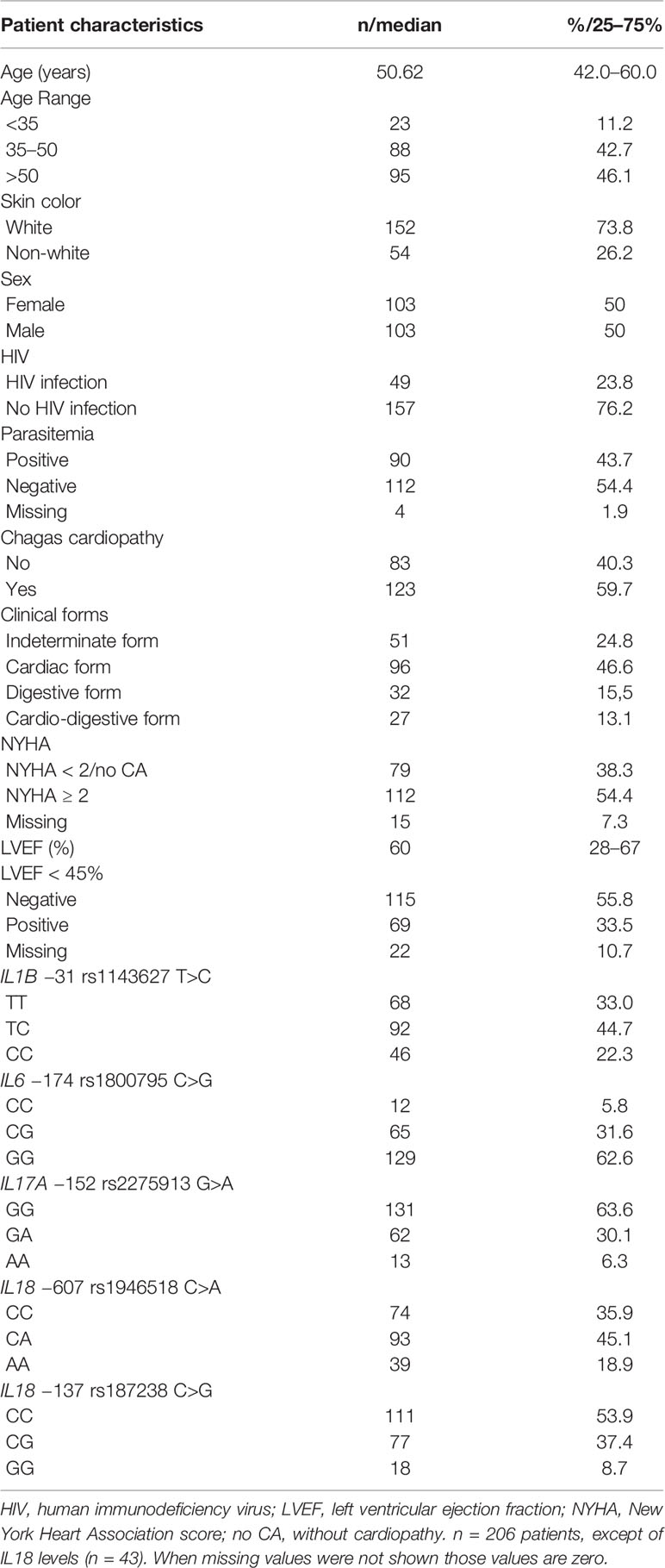
Table 1 Patient distribution according to demographic features, HIV status, parasitemia, cardiac phenotypes, and single nucleotide variant (SNV) genotypes.
The entire study population was in Hardy-Weinberg equilibrium (HWE) (Supplementary Table 2). The genotype frequencies were also in HWE in whites and non-whites (Supplementary Table 2). Interestingly, HWE equilibrium was not present in some disease conditions. We detected HWE violation in IL6 −174 rs1800795 C>G in patients with detectable T. cruzi DNA in peripheric blood; in patients without cardiopathy (IL17 rs2275913 G>A and IL18 −137 rs187238 C>G) and in patients with cardiopathy (IL1B rs1143627 T>C); in individuals with NYHA < 2 (NYHA 1) or without heart failure (IL17 rs2275913 G>A and IL18 −607 rs1946518 C>A); and in subjects with NYHA ≥ 2 (IL1B rs1143627 T>C) (Supplementary Table 2). Those data suggest that the mentioned disease conditions could be influenced by the allelic composition in the selected loci.
We analyzed self-declared/documented ancestry as a potential confounding factor for all dependent variables. The P values are mentioned in Supplementary Table 3 and the comparisons can be appreciated in Supplementary Table 4, which comprises the frequencies of self-declared/documented ancestry for each of the dependent variables. The P values show that there was no significant interference of self-declared/documented ancestry in the analyzed comparisons carried out in this study.
IL1B, IL17A, and IL18 Polymorphic Genotypes Decrease the Risk or Attenuate Progression of Cardiomyopathy in Chagas Disease
Preliminary univariate analyses showed potential associations between cardiomyopathy and sex (P = 0.023), IL18 −607 rs1946518 C>T applying the recessive model (P = 0.011), IL18 −137 rs187238 C>G using the recessive model (P = 0.078), IL17 rs2275913 G>A based on the recessive model (P = 0.039), and digestive involvement (P = 0.012) (Supplementary Table 3).
These initial analyses also predicted potential associations between NYHA score ≥ 2 and gender (p = 0.019), IL1B rs1143627 T>C using the heterozygous model (P = 0.008), IL18 −607 rs1946518 C>A applying the recessive model (P = 0.009), IL18 −137 rs187238 C>G applying the recessive model (P = 0.194), and digestive involvement (P = 0.001) (Supplementary Table 3). LVEF-based analyses, in turn, showed potential associations between LVEF < 45% and sex (P = 0.128), IL18 −607 rs1946518 C>A applying the recessive model (P = 0.003), IL18 −137 rs187238 C>G applying the recessive model (P = 0.052), IL1B rs1143627 T>C using the heterozygous model (P = 0.033), IL6 rs 1800795 C>G applying the recessive model (P = 0.028), IL17 rs2275913 G>A using the recessive model (P = 0.157), and digestive involvement (P < 0.001) (Supplementary Table 3).
As for Parasitemia, potential associations were shown with LVEF < 45% (P = 0.031), NYHA score ≥ 2 (P = 0.180), and IL6 rs1800795 heterozygous model (P = 0.023) (Supplementary Table 3).
In this scenario, we performed multiple logistic regression analyses for each of the elected dependent variables. This assessment revealed that males with Chagas disease had an OR of 2.22 (P = 0.011, 95% CI 1.20–4.09) to develop cardiomyopathy (Table 2). Interestingly, homozygosity for the IL17 rs2275913 A allele carried a heart protective effect, being associated with an OR of 0.27 (P = 0.044, 95% CI 0.07–0.97) (Table 2). A similar effect was detected for homozygosity of the IL18 −607 rs1946518 A allele (OR = 0.35; P = 0.023, 95% CI 0.14–0.87).
Our analyses also established a positive association between NYHA score ≥ 2 and male gender (OR = 2.62, P = 0.007, 95% CI 1.30–5.27) and a negative association between this NYHA score range and the IL18 −607 rs1946518 AA genotype (OR = 0.21, P = 0.009, 95% CI 0.06–0.68) and IL1B rs1143627 T>C using the heterozygous model (OR = 0.48, P = 0.036, 95% CI 0.24–0.95) and digestive involvement (OR = 0.20, P < 0.001, 95% CI 0.09–0.47) (Table 3). A similar effect was observed for the IL18 −607 rs1946518 AA genotype with respect to LVEF < 45% (OR = 0.22, P = 0.034, CI 0.05–0.89), and digestive involvement (OR = 0.24, P = 0.004, 95% CI 0.09–0.62) (Table 4).
When investigating linkage disequilibrium between rs1946518 and rs187238, we found D` of 0.89 and r2 of 0.43, indicating strong linkage disequilibrium between the two loci. Our analysis also revealed that the haplotype IL18 −607 C/IL18 −137 C was associated with Chagas cardiopathy (P = 0.0186) and LVEF < 45% (P = 0.0149) (Supplementary Table 5). This same haplotype also reached a marginal P value for association with NYHA ≥ 2 (P = 0.0554). No haplotype involving these two SNVs showed significant association with the parasitemia status.
A Specific IL6 Polymorphism Profile Modulates the Risk of Trypanosoma cruzi Parasitemia
Based on univariate analyses, potential associations were predicted to occur between positive T. cruzi parasitemia and IL6 rs1800795 C>G applying the heterozygous model (P = 0.023), NYHA ≥ 2 (P = 0.180) and HIV infection (P = 0.008) (Supplementary Table 3).
Notably, multiple logistic regression analyses uncovered a negative association between parasitemia and the IL6 rs1800795 CG genotype (OR = 0.45, P = 0.015, 95% CI 0.24 −0.86) and a positive association between parasitemia and HIV infection (OR = 2.18, P = 0.039, 95% CI 1.04–4.58) (Table 5).
HIV Infection Is Protective Against Development and Progression of Cardiomyopathy in Chagas Disease
Our preliminary univariate analyses suggested a protective cardiac effect of HIV infection in patients with Chagas disease, based on the development of cardiomyopathy (P = 0.045), the NYHA score (P < 0.001), and the LVEF values (P < 0.001) (Supplementary Table 3).
To evaluate the potential effect of HIV infection on the cardiac form of Chagas disease, we initially compared the HIV population with the non-HIV patients with respect to potentially interfering parameters (Table 6). This analysis showed no differences in age, sex, groups (white x non white), and the SNV genotypes analyzed in the current study.
Remarkably, multiple logistic regression analyses revealed a protective effect of HIV infection against the development of cardiomyopathy, displaying an OR of 0.48 (P = 0.039, 95% CI 0.23–0.96) (Table 2). In the same line, negative associations were shown for NYHA score ≥ 2 and HIV infection (OR = 0.15, P < 0.001, 95% CI 0.06–0.39) (Table 3) as well as for LVEF < 45% and positive HIV status (OR = 0.03, P < 0.001, 95% CI 0.00–0.25) (Table 4).
IL18 Levels, Cardiopathy, New York Heart Association, Left Ventricular Ejection Fraction, and HIV
IL18 levels were analyzed in 43 patients and assessed with respect to the genotypes/alleles and the presence of cardiopathy, HIV infection, NYHA score, and LVEF (Table 7). The IL18 levels did not significantly vary among IL18 −607 or IL18 −137 SNV genotypes/alleles. No significant differences in this interleukin levels were detected either between patients with and without cardiopathy or HIV infection as well as among the different NYHA score or LVEF-related groups. Three patients without HAART therapy displayed serum levels of 737.49, 739.87, and 744.29 pg/ml. These last two were viremic and only one had a CD4 count below 350 cells/mm3. The 13 aviremic individuals under HAART therapy presented a median value of 308.24 pg/ml and all of them had CD4 cells higher than 350 cell/mm3.
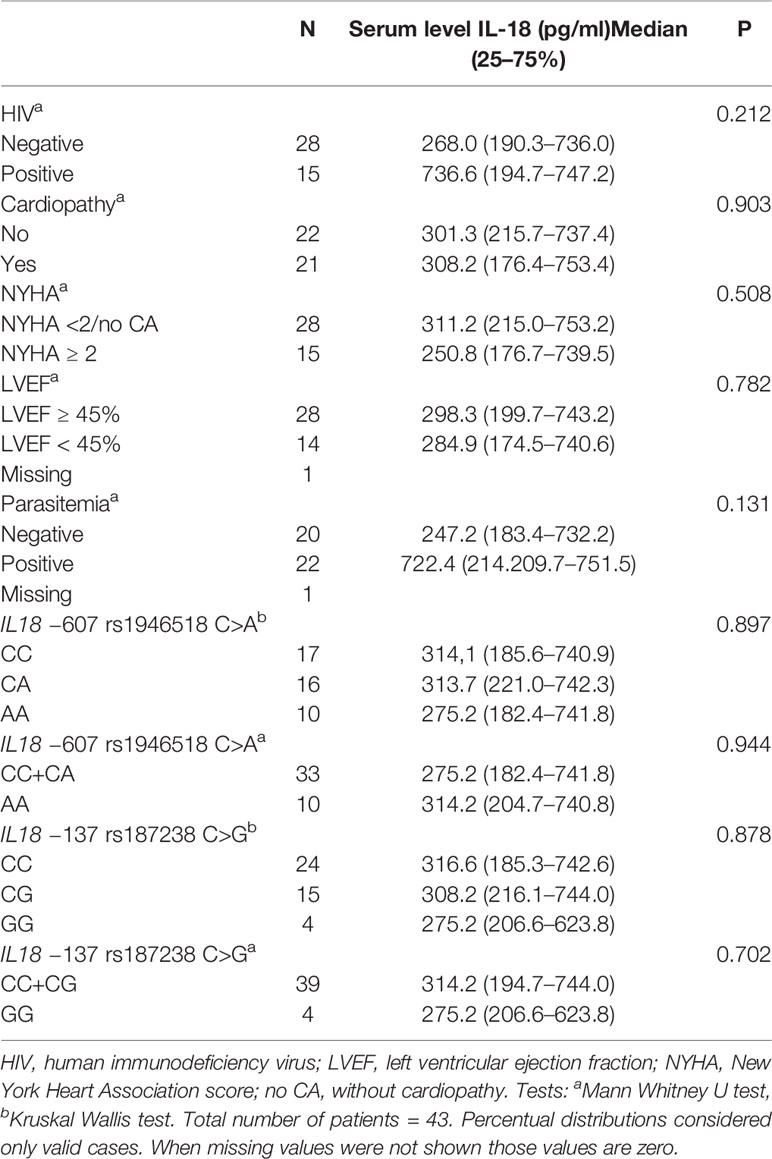
Table 7 IL18 serum levels in Chagas disease patients according to the HIV status, cardiac phenotypes, parasitemia, and IL 18 −607 and IL 18 −137 genotypes.
Discussion
IL6, IL1B, IL17A, and IL18 Single Nucleotide Variants and Parasitemia in Chagas Disease
In a still largely unclear scenario relating cytokines and pathogenesis of Chagas disease, our study seeked for potential associations between strategic cytokine-related SNVs and key Chagas phenotypes restricted to the disease or combined with immune dysregulation.
T. cruzi parasitemia has been recognized as an element that accelerates chronic Chagas cardiopathy (19, 20, 22), although some reports question this effect (23–25). PCR-based T. cruzi detection in human biopsies supports that parasite persistence in the heart favors the progression of cardiomyopathy (19, 21). Identification of T. cruzi DNA in human blood, moreover, has been associated with a higher risk of development and deterioration of cardiomyopathy (20, 22).
IL6 is a key cytokine in mouse-induced T. cruzi myocarditis, triggering protection mechanisms in cardiomyocytes but favoring a higher parasite load (29). Acute T. cruzi experimental infection in mice with high inoculum, in fact, was followed by increased IL-6, high parasite load in tissue and marked inflammation (54, 55) while IL6-KO mice developed different levels of parasite load in the blood and myocardium depending on the T. cruzi strain (29, 54). These animals, however, presented increased mortality (29, 54). Such findings suggest that avoidance of high IL6 levels may play a role in lowering the parasite load whereas a certain tissue level of IL6 must be reached to allow a protective response against the parasite-induced lesion. A balanced level of IL6, therefore, is expected to reduce tissue damage and cardiomyocyte death, mitigating the disease. Of note, our data revealed that IL6 −174 CG heterozygosity is protective against T. cruzi parasitemia. This original finding raises an enticing mechanistic hypothesis for the relationship between IL6 and parasitemia. This SNV is located in the IL6 promoter, having the G allele been associated with higher IL6 blood levels than the C allele (33, 34, 56, 57). It is likely, therefore, that the low IL6 levels associated with the CC genotype lead to a lack of immune-mediated protection against parasitemia, while the high IL6 levels observed in GG individuals create a relatively severe inflammatory background that also favors T. cruzi replication. On the other hand, a balanced scenario of protection and controlled inflammation, displayed by heterozygous patients, would provide the best setting to fight parasitemia.
Facing the possible role of IL18 in reducing parasitic load in IL18-KO mice (26), we investigated the influence of IL18 SNVs on T. cruzi parasitemia. The IL18 human gene harbors several SNVs in its promoter. IL18 −607 C>A and IL18 −137 C>G are located in nuclear factor binding sites for CREB (cyclic AMP-responsive element binding protein) and H4TF-1 (human histone H4 gene specific transcription fator-1), respectively. Variants of these two sites can not only affect IL18 transcription but also IFNγ expression (58). The −607 A allele and AA genotype have been associated with lower IL18 transcriptional rate and/or protein levels than the C allele and CC genotype in different clinical and physiological settings (59–63), whereas the haplotype C-607/G-137 is associated with higher expression of IL18. Our analyses, however, did not identify a significant impact of IL18 −607 or IL18 −137 alleles/genotypes on the T. cruzi parasitemic status.
The observation of increased parasitemia in IL17A-KO mice infected by Leishmania infantum supports a role of IL17A in controlling parasitemia (64). IL17A and IFNγ activate macrophages to phagocyte T. cruzi for further killing in the endosomal/lysosomal compartment (65). This finding is not a surprise, since association between higher levels of IL17A and resistance to Leishmania donovani had been previously reported (66).
The IL17A −152 G>A SNV is a critical regulator of the IL17A promoter, being located within a binding motif for NFAT (nuclear factor activated T cells) (67). The A allele is more often associated with higher IL17A serum levels (40, 41, 67). Despite these observations, our analysis did not show association between IL17A −152 G>A and T. cruzi parasitemia, not supporting a protective role of the A allele against parasitemia in human Chagas disease.
Effects of IL1B, IL17A, IL18, and IL6 Single Nucleotide Variants on Cardiomyopathy in Chagas Disease
Previous studies showed direct correlation between IL-6 serum levels and cardiomyopathy (7–9) and an inverse correlation between IL-6 levels and LVEF in the setting of cardiopathy (7). Based on these observations and the association of IL6 −174 C with lower IL6 levels (33, 34, 56, 57), we hypothesized that this allele might be protective. Our findings, however, did not reveal such an impact, corroborating results from Colombia and Peru obtained in an ethnically distinct population affected by different parasite lineages (32, 68). It is possible, therefore, that other factors may also play a role in determining IL6 levels that modulate the cardiac phenotype in Chagas disease.
IL1β triggers an inflammation cascade, leads to generation of reactive free radicals, and may promote IFNγ production (69) and facilitate the differentiation of pro-inflammatory cells such as human Th17 (70). The assessed IL1B −31 T>C transition causes disruption of a TATA‐box, potentially changing the binding site affinity to regulatory proteins (36). We showed association between the IL1B −31 TC genotype and lower NYHA in Chagas disease. It is known that IL1β promotes inflammatory activity in acute experimental myocarditis induced by T. cruzi (71) and induces hypertrophy in primary cultures of cardiomyocytes infected by this parasite (72). Based on these data and because the IL1B −31 T allele is associated with increased IL1B transcription (36), heterozygosity may attenuate inflammation and cardiomyocyte hypertrophy in Chagas myocarditis, still allowing enough IL1β to fight T. cruzi infection. This balanced response likely explains the observed protective effect of the IL1B −31 TC genotype against cardiac disease severity. Interestingly, the CT haplotype for IL1B −31 T>C and +3,954 C>T was more prevalent in patients with Chagas cardiomyopathy in a Colombian population (35).
Higher frequencies of CD4+ IL-17A+ T-cells, lower levels of IL10 and IL10, and higher levels of IFNγ and TNFα are observed in individuals with more severe cardiomyopathy (6, 7). These findings are in agreement with the observation of lower lymphocyte IL17A expression in chronic Chagas cardiomyopathy than in seronegative individuals and patients with the indeterminate form (6). In addition, high IL17A levels were also associated with better heart function in Chagas disease (11). Based on these observations, IL17A was expected to potentially modulate inflammation in cardiac tissue and protect against T. cruzi infection (27, 28). A study in Brazil, however, unexpectedly revealed associations between the IL17A −152 A allele and the AA genotype with chronic cardiomyopathy (42). In contrast, another study in a Colombian cohort reported a protective effect of the C Allele of IL17A rs 8193036 C>T in seronegative individuals in comparison with cardiac seropositive patients (38), in a context of previously established association between the C allele and lower IL 17A mRNA expression (39). These data were a priori not expected, since previous reports showed a role for IL17A in mouse resistance to T. cruzi infection (27) and/or higher IL17A levels in patients without cardiopathy (11, 12). This study also found no association between the IL17A G>A 3’ UTR rs7747909 SNV, other two IL17A promoter SNVs (rs4711998 and rs3819024) or IL17A rs2275913 and Chagas cardiopathy.
In agreement with the expected role of IL17A, but in contrast to most clinical results, we found association between IL17A rs2275913 G>A and cardiac protection following a recessive model. Indeed, carriers of the A allele and the AA genotype were found to have a lower risk to develop cardiomyopathy. Since A allele has been found in association with higher IL17A serum levels (40, 41, 67), our data are in accordance with reports that show association between better cardiac function and higher IL17 A levels (11, 12). Our findings suggest that IL17A modulates the host inflammatory response to parasites in the myocardium, impacting the risk of developing cardiac dysfunction. Additional studies are necessary, however, to establish the effect of the AA genotype on IL17A serum levels in Chagas disease patients. It must be pointed out that our study differs from the previous ones (38, 42) with respect to patient geographical origin, ethnicity, classification, sample size, subgroup design, and possibly parasite lineage (68). Given the significant ethnical differences among Brazilian, Colombian, and Bolivian populations, genetic background may be a major determinant for the different results.
Since IL18 induces pathological cardiac remodeling (16), cardiomyocyte hypertrophy (73) and increased fibronectin expression (74), high levels of IL18 are expected to lead to cardiac hypertrophy and fibrosis, with a deleterious impact on heart function. IL18 is expressed in myocardium early in the course of experimental myocarditis induced by T. cruzi (15), leading to production of IFNγ. IL18 upregulation and correlation between IL18 mRNA and IFNγ expression have been shown in human Chagas heart tissue (18). Standing as a novel observation, we showed association between the IL18 −607 AA genotype and decreased risk of developing cardiomyopathy, progressing to NYHA ≥ 2, and progressing to LVEF < 45%. These data are in accordance to previously reported association between this AA genotype and low IL18 production in the presence and in the absence of mitogen stimuli (60–66). Unlike these studies, however, our data did not reveal significant associations between IL18 levels and any of the IL18 −607 or IL18 −137 genotypes/alleles. Such differences may rely on the nature and size of the studied populations (55–101 patients), including other diseases, and/or the nature the biological samples and/or experimental conditions, including plasma and mucosal biopsies and peripheral blood cells under mitogen stimuli (58–63). The lack of association between IL18 serum levels and IL18 −607 C>A or IL18 −137 C>G genotypes/alleles observed in our study does not allow a straight association between the mentioned cardiac findings and IL18 serum levels. This finding raises the hypothesis that IL18 serum levels may not significantly correlate with IL18 heart tissue levels in Chagas disease. However, since our study did not reproduce the association between IL18 levels and the IL18 −607 SNV in other contexts, more representative patient samples should be evaluated under different conditions to more conclusively investigate this relationship in this disease. It must be noted that a previous study conducted in Colombian and Latin American Chagas patients did not detect significant frequency differences of IL18 −607 A allele and AA genotype between asymptomatic patients and ones with cardiac dysfunction (43, 44). Data from an Argentinian population, on the other hand, display a trend similar to our study (44). Our data strengthens the potential benefit of targeting IL18 as a therapy in Chagas cardiomyopathy. IL18 levels have indeed been associated with increased mortality and are inversely correlated with LVEF in heart failure (75). Moreover, neutralization of IL18 has been proposed in heart failure (76), Fabry cardiomyopathy (77) and sepsis-induced cardiac dysfunction (78). Our data also revealed association of the IL18 −607 C/IL18 −137 C haplotype with Chagas cardiopathy and LVEF < 45%. Our results on IL18 −137, however, do not support an additive susceptibility status linking such SNVs.
Our study revealed that men have higher risks for cardiopathy and NYHA ≥ 2. These findings are in line with previous data that report male gender as one of the six independent variables that constitute risk factors for death in Chagas heart disease (3, 79). Male sex, therefore, appears as a robust risk factor for worse prognosis in Chagas cardiomyopathy. As a novel finding, digestive involvement associated negatively with NYHA ≥ 2 and LVEF < 45%, suggesting that such patients may develop a milder cardiopathy than affected individuals without digestive disease. This observation has never been reported (1, 31, 80). Alternative explanations for this finding may comprise a potential inclusion of more severely affected cardiac patients in our study and a potentially smaller than real proportion of patients with the indeterminate form due to underdiagnosis and/or loss of follow-up, raising the cardiac form/indeterminate form ratio. The relatively high proportion of cardio-digestive cases previously reported (1, 31), however, adds further support to the influence of digestive involvement in NYHA and LVEF.
Impact of HIV Infection on Development and Progression of Cardiomyopathy in Chagas Disease
In immunosuppressed patients with HIV infection, elevated IL18 serum concentrations have been shown to favor the development of Aids and associated infections (30). In addition, Chagas disease reactivation does not occur in immunocompetent subjects, while in HIV-infected patients this illness has been associated with increased morbidity and mortality (31). Based on a severe combined immunodeficient (SCID) animal model (81), this observation may be explained by dissociation between a high parasite load and lower tissue inflammation, which is associated with decreased IFNγ levels and early mortality. Interestingly, T. cruzi inhibits HIV replication in macrophage-derived monocyte culture (82). In our study the HIV infected and non-infected groups did not differ with respect to the evaluated SNV profiles, age or gender. In the HIV-infected group, 53.1% of the patients were not on HAART therapy. Our findings revealed higher parasitemia in HIV-infected Chagas individuals, confirming previous data (83). Of note, in T. cruzi/HIV coinfection a Th2 response likely contributes to the impairment of parasite control (84). Altogether, our data suggest that antiretroviral therapy interferes positively in the host-HIV-T. cruzi interaction.
To our surprise, our analyses revealed a previously not described finding: HIV infection exerts a strong protective effect on Chagas disease cardiomyopathy. HIV infection was associated with lower risks to develop cardiopathy and to progress to NYHA ≥ 2 and LVEF < 45%. This a priori unexpected effect may be based on actions of the HAART regimens, reported to decrease the prevalence of HIV-associated cardiomyopathy in about 30% of the HIV-infected patients (85). In contrast, HAART failure was associated with continued IL18 elevation (86).
IL18 contributes to Aids pathogenesis by increasing viral replication, death of endothelial cells, and cardiomyocyte proliferation (30). A previous study from our service showed that Chagas reactivation occurred only in patients not in HAART therapy, and progression to a more severe chronic disease and death were observed in 35 and 30% of them, respectively (31). Moreover, progression to severe chronic forms was verified in less than 10% of patients on HAART. In those under HAART therapy, we detected high levels of IL18 (>700 pg/ml) in two of viremic cases and a median of 308.24 pg/ml in 13 aviremic individuals. The small number of analyzed patients, however, did not allow statistical evaluation with respect to IL18 levels and viral load, and no differences in IL18 levels were detected between HIV and non-HIV patients. A previous report did not find difference between 32 HIV viremic and 15 aviremic patients either (87). While our data suggest that IL18 serum levels may not correlate with IL18 levels in cardiac tissue in Chagas disease, a larger future study should address a still possible IL18-reducing effect of HAART on Chagas cardiomyopathy.
Another potential explanation for this positive impact of HIV infection on Chagas disease could be a growth-reduction effect of antiretroviral drugs on T. cruzi. HIV protease inhibitors have in fact been reported to restrain epimastigote, trypomastigote, and amastigote growth (88–90). Pretreatment of trypomastigotes with nelfinavir and lopinavir inhibited their association with LLC-MK2 epithelial cells and RAW macrophages and decreased the number of intracellular amastigotes (88). In addition, interaction of ritonavir, lopinavir and nelfinavir and T. cruzi epimastigotes showed potent anti-proliferative effects and led to changes in differentiation (89).
Nelfinavir and lopinavir target vital cellular structures of trypomastigotes, leading to irreversible, lethal metabolic injuries in the size and structure (90). A trypanocidal effect of HIV proteinase inhibitors on the T. cruzi aspartyl peptidase intracellular target was also reported using a docking simulation model (91), showing a great affinity of ritonavir and lopinavir to bind to this active enzyme intracellular site. In this setting, saquinavir has been integrated to the computer-guided drug repositioning as a potentially new treatment for Chagas disease (92).
A synergistic interaction between a Th2 predominant response in T. cruzi-HIV infected patients and HAART could also potentially lead to reduction in IL18 levels (30). This combined effect might decrease the predominant Th1 response in myocardium (5) and reduce inflammatory cardiac lesions. Other cardiovascular actions of antiretrovirals are unlikely to contribute to the cardioprotective effect, since HAART has been linked to increased risk of cardiovascular illness (30).
While the scientific relevance and possible clinical applications raised by the HIV-related cardioprotective effect on Chagas cardiomyopathy are potentially remarkable, its underlying mechanisms remain unclear. Future studies with larger and more diverse coinfected patient populations, expansion of cardiac function/lesion markers, detailed characterization of the cardiac microenvironment associated this coinfection, and deep characterization of cardiac tissue response to HAART should bring significant contributions to this elucidation.
Our study brought novel and meaningful data to field of Chagas disease, but it also carries some limitations. Such limitations comprise the inclusion of a single center, Brazilian heterogeneous ethnicity, limited analyses of interleukin levels and respective functional impact, and lack of data integration involving the use of HAART, CD4+ count, HIV load, and molecular genetics assessment. The appropriate statistical model employed for small samples and the level of significance detected in key analyses, however, strongly support our conclusions and provide a robust platform for sequential future studies.
In conclusion, our findings revealed a novel association between the IL6 −174 C>G SNV and parasitemia in Chagas disease, expressed as a decreased risk of its detection in patients with the CG genotype. As additional original findings, our data showed that the IL17A −152 AA and the IL18 −607 AA genotypes are associated with a reduced risk of developing cardiomyopathy in individuals affected by this illness. The IL18 −607 AA and IL1B TC genotypes were also found to associate with a lower risk of manifesting severe cardiac dysfunction. Most of our findings are consistent with previously reported clinical data on Chagas disease and data on cytokine expression. Unexpectedly, our results uncovered a novel protective effect of HIV infection in Chagas disease patients, translated into a decreased risk of developing cardiomyopathy. Available information suggests that a synergistic effect of HIV and HAART may mitigate a Th1-mediated response in the myocardium. The findings revealed by this report not only expand the understanding of Chagas disease pathogenesis and its relationship with other clinical scenarios but also add pieces of information to the field that can be potentially employed in the development of therapeutic interventions.
Data Availability Statement
The datasets generated for this study can be found in the DRYAD Digital Repository (doi: 10.5061/dryad.wstqjq2hn).
Author Contributions
MS-Y, VF, LO: conception and design. AG, EW, DF, JO, EN, CO, EB, AP, CN, FC, NC, PS, EY-K, VF, LO, MS-Y: analysis and interpretation. AG, EW, LO, MS-Y: drafting the manuscript for important intellectual content. All authors contributed to the article and approved the submitted version.
Funding
This work was supported by São Paulo Research Foundation (FAPESP) [#12/50273-0]; and by Conselho Nacional de Desenvolvimento Científico e Tecnológico - Programa Institucional de Bolsas de Iniciação Científica (CNPq - PIBIC) [AGS #100793/2016-9 from January to July 2016], and JOR # 157966/2015-1 from September to December 2015]; and by FAPESP (Technical Training Scholarship [CSO # 2014/07100-3 from May of 2014 to March 2015] and Secretary of Administration of São Paulo State Secretary [DTF # from March of 2014 to February of 2016], and for IL18 analyses by São Paulo Research Foundation (FAPESP) [#19/06363-4].
Conflict of Interest
The authors declare that the research was conducted in the absence of any commercial or financial relationships that could be constructed as a potential conflict of interest.
The reviewers FD and CM-J declared a shared affiliation, with no collaboration, with the authors to the handling editor at the time of the review.
Acknowledgments
The authors acknowledge Expedito Luna for revising the epidemiological design of this manuscript.
Supplementary Material
The Supplementary Material for this article can be found online at: https://www.frontiersin.org/articles/10.3389/fimmu.2020.521409/full#supplementary-material
References
1. Dias JC, Ramos AN Jr, Gontijo ED, Luquetti A, Shikanai-Yasuda MA, Coura JR, et al. 2nd Brazilian Consensus on Chagas Disease, 2015. Rev Soc Bras Med Trop (2016) 49:3–60. doi: 10.1590/0037-8682-0505-2016
2. Bocchi EA, Bestetti RB, Scanavacca MI, Cunha Neto E, Issa VS. Chronic Chagas Heart Disease Management: From Etiology to Cardiomyopathy Treatment. J Am Coll Cardiol (2017) 70:1510–24. doi: 10.1016/j.jacc.2017.08.004
3. Rassi A Jr, Rassi A, Little WC, Xavier SS, Rassi SG, Rassi AG, et al. Development and validation of a risk score for predicting death in Chagas’ heart disease. N Engl J Med (2006) 355:799–808. doi: 10.1056/NEJMoa053241
4. Dutra WO, Gollob KJ. Current concepts in immunoregulation and pathology of human Chagas disease. Curr Opin Infect Dis (2008) 21:287–92. doi: 10.1097/QCO.0b013e3282f88b80
5. Abel LC, Rizzo LV, Ianni B, Albuquerque F, Bacal F, Carrara D, et al. Chronic Chagas’ disease cardiomyopathy patients display an increased IFN-gamma response to Trypanosoma cruzi infection. J Autoimmun (2001) 17:99–107. doi: 10.1006/jaut.2001.0523
6. de Araújo FF, Corrêa-Oliveira R, Rocha MO, Chaves AT, Fiuza JA, Fares RC, et al. Foxp3+CD25 (high) CD4+ regulatory T cells from indeterminate patients with Chagas disease can suppress the effector cells and cytokines and reveal altered correlations with disease severity. Immunobiology (2012) 217:768–77. doi: 10.1016/j.imbio.2012.04.008
7. Sousa GR, Gomes JA, Fares RC, Damásio MP, Chaves AT, Ferreira KS, et al. Plasma cytokine expression is associated with cardiac morbidity in Chagas disease. PloS One (2014) 9:e87082. doi: 10.1371/journal.pone.0087082
8. Reis MM, Higuchi M de L, Benvenuti LA, Aiello VD, Gutierrez PS, Bellotti G, et al. An in situ quantitative immunohistochemical study of cytokines and IL-2R+ in chronic human chagasic myocarditis: correlation with the presence of myocardial Trypanosoma cruzi antigens. Clin Immunol Immunopathol (1997) 83(2):165–72. doi: 10.1006/clin.1997.4335
9. Keating SM, Deng X, Fernandes F, Cunha-Neto E, Ribeiro AL, Adesina B, et al. NHLBI Retrovirus Epidemiology Donor Study-II (REDS-II), International Component. Inflammatory and cardiac biomarkers are differentially expressed in clinical stages of Chagas disease. Int J Cardiol (2015) 199:451–9. doi: 10.1016/j.ijcard.2015.07.040
10. López L, Arai K, Giménez E, Jiménez M, Pascuzo C, Rodríguez-Bonfante C, et al. C-reactive protein and interleukin-6 serum levels increase as Chagas disease progresses towards cardiac failure. Rev Esp Cardiol (2006) 59:50–6. doi: 10.1016/S1885-5857(06)60048-0
11. Magalhaes LM, Villani FN, Nunes M do C, Gollob KJ, Rocha MO, Dutra WO. High interleukin 17 expression is correlated with better cardiac function in human Chagas disease. J Infect Dis (2013) 207:661–5.9. doi: 10.1371/journal.ppat.1005902
12. Sousa GR, Gomes JA, Damasio MP, Nunes MC, Costa HS, Medeiros NI, et al. The role of interleukin 17-mediated immune response in Chagas disease: High level is correlated with better left ventricular function. PloS One (2017) 12:e0172833. doi: 10.1371/journal.pone.0172833
13. Guedes PM, Gutierrez FR, Silva GK, Dellalibera-Joviliano R, Rodrigues GJ, Bendhack LM, et al. Deficient regulatory T cell activity and low frequency of IL-17 – producing T cells correlate with the extent of cardiomyopathy in human Chagas’ disease. PloS Negl Trop Dis (2012) 6:e1630. doi: 10.1371/journal.pntd.0001630
14. Cai CW, Blase JR, Zhang X, Eickhoff CS, Hoft DF. Th17 Cells Are More Protective Than Th1 Cells Against the Intracellular Parasite Trypanosoma cruzi. PloS Pathog (2016) 12(10):e1005902. doi: 10.1371/journal.ppat.1005902
15. Muller U, Kohler G, Mossmann H, Schaub GA, Alber G, Di Santo JP, et al. IL-12- Independent IFN-gamma production by T cells in experimental Chagas’ disease is mediated by IL-18. J Immunol (2001) 167(6):3346–53. doi: 10.4049/jimmunol.167.6.3346
16. Xiao H, Li H, Wang JJ, Zhang JS, Shen J, An XB, et al. IL-18 cleavage triggers cardiac inflammation and fibrosis upon β-adrenergic insult. Eur Heart J (2018) 39:60–9. doi: 10.1093/eurheartj/ehx261
17. Meyer Zum Büschenfelde C, Cramer S, Trumpfheller C, Fleischer B, Frosch S. Trypanosoma cruzi induces strong IL-12 and IL-18 gene expression in vivo: correlation with interferon-gamma (IFN-gamma) production. Clin Exp Immunol (1997) 110:378–85. doi: 10.1046/j.1365-2249.1997.4471463.x
18. Nogueira LG, Santos RH, Fiorelli AI, Mairena EC, Benvenuti LA, Bocchi EA, et al. Myocardial gene expression of T-bet, GATA-3, Ror-γt, FoxP3, and hallmark cytokines in chronic Chagas disease cardiomyopathy: an essentially unopposed TH1-type response. Mediators Inflamm (2014) 2014(914326):9. doi: 10.1155/2014/914326
19. Zhang L, Tarleton RL. Parasite persistence correlates with disease severity and localization in chronic Chagas’ disease. J Infect Dis (1999) 180:480–6. doi: 10.1016/j.micinf.2014.03.007
20. Basquiera AL, Sembaj A, Aguerri AM, Omelianiuk M, Guzman S, Moreno Barral J, et al. Risk progression to chronic Chagas cardiomyopathy: influence of male sex and of parasitaemia detected by polymerase chain reaction. Heart (2003) 89:1186–90. doi: 10.1136/heart.89.10.1186
21. Benvenuti LA, Roggerio A, Freitas HF, Mansur AJ, Fiorelli A, Higuchi ML. Chronic American trypanosomiasis: parasite persistence in endomyocardial biopsies is associated with high-grade myocarditis. Ann Trop Med Parasitol (2008) 102:481–7. doi: 10.1179/136485908X311740
22. Sabino EC, Ribeiro AL, Lee TH, Oliveira CL, Carneiro-Proietti AB, Antunes AP, et al. Detection of Trypanosoma cruzi DNA in blood by PCR is associated with Chagas cardiomyopathy and disease severity. Eur J Heart (2015) 17:416–23. doi: 10.1002/ejhf.220
23. Norman FF, Perez-Ayala A, Perez-Molina JA, Flores-Chavez M, Canavate C, Lopez-Velez R. Lack of association between blood-based detection of Trypanosoma cruzi DNA and cardiac involvement in a non-endemic area. Ann Trop Med Parasitol (2011) 105:425–30. doi: 10.1179/1364859411Y.0000000033
24. Apt W, Arribada A, Zulantay I, Saavedra M, Muñoz C, Toro B. Vega, Rodríguez J.Chronic Chagas cardiopathy in Chile. Importance of Trypanosoma cruzi burden and clinical evaluation. Acta Trop (2016) 162:155–66. doi: 10.1016/j.actatropica.2016.06.025
25. D’Ávila DA, Galvão LMC, Sousa GR, Britto C, Moreira OC, Chiari E. Monitoring the parasite load in chronic Chagas disease patients: comparison between blood culture and quantitative real time PCR. PloS One (2018) 13(11):e0208133. doi: 10.1371/journal.pone.0208133
26. Esper L, Utsch L, Soriani FM, Brant F, Esteves Arantes RM, Campos CF, et al. Regulatory effects of IL-18 on cytokine profiles and development of myocarditis during Trypanosoma cruzi infection. Microb Infect (2014) 16:481–90. doi: 10.1016/j.micinf.2014.03.007
27. Miyazaki Y, Hamano S, Wang S, Shimanoe Y, Iwakura Y, Yoshida H. IL-17 is necessary for host protection against acute-phase Trypanosoma cruzi infection. J Immunol (2010) 185:1150–7. doi: 10.4049/jimmunol.0900047
28. da Matta Guedes PM, Gutierrez FR, Maia FL, Milanezi CM, Silva GK, Pavanelli WR, et al. IL-17 produced during Trypanosoma cruzi infection plays a central role in regulating parasite-induced myocarditis. PloS Negl Trop Dis (2010) 4:e604. doi: 10.1371/journal.pntd.0000604
29. Sanmarco LM, Ponce NE, Visconti LM, Eberhardt N, Theumer MG, Minguez Á. R. Aoki MP. L-6 promotes M2 macrophage polarization by modulating purinergic signaling and regulates the lethal release of nitric oxide during Trypanosoma cruzi infection. Biochim Biophys Acta Mol Basis Dis (2017) 1863:857–69. doi: 10.1016/j.bbadis.2017.01.006
30. Samarani S, Allam O, Sagala P, Aldabah Z, Jenabian MA, Mehraj V, et al. Imbalanced production of IL-18 and its antagonist in human diseases, and its implications for HIV-1 infection. Cytokine (2016) 82:38–51. doi: 10.1016/j.cyto.2016.01.006
31. Sartori AM, Ibrahim KY, Nunes Westphalen EV, Braz LM, Oliveira OC Jr, Gakiya E, et al. Manifestations of Chagas disease (American trypanosomiasis) in patients with HIV/AIDS. Ann Trop Med Parasitol (2007) 101:31–50. doi: 10.1179/136485907X154629
32. Torres OA, Calzada JE, Beraún Y, Morillo CA, González A, González CI, et al. Lack of association between IL6-174G/C gene polymorphism and Chagas disease. Tissue Antigens (2010) 76:131–4. doi: 10.1111/j.1399-0039.2010.01478.x
33. Lorente L, Martín MM, Pérez-Cejas A, Barrios Y, Solé-Violán J, Ferreres J, et al. Association between interleukin-6 promoter polymorphism (-174 G/C), serum interleukin-6 levels and mortality in severe septic patients. Int J Mol Sci (2016) 17:E1861. doi: 10.3390/ijms17111861
34. Fishman D, Faulds G, Jeffery R, Mohamed-Ali V, Yudkin JS, Humphries S, et al. The effect of novel polymorphisms in the interleukin-6 (IL-6) gene on IL-6 transcription and plasma IL-6 levels, and an association with systemic-onset juvenile chronic arthritis. J Clin Invest (1998) 102:1369–76. doi: 10.1172/JCI2629
35. Flórez O, Zafra G, Morillo C, Martín J, González CI. Interleukin-1 gene cluster polymorphism in Chagas disease in a Colombian case-control study. Hum Immunol (2006) 67:741–8. doi: 10.1016/j.humimm.2006.06.004
36. El-Omar EM, Carrington M, Chow WH, McColl KE, Bream JH, Young HA, et al. Interleukin-1 polymorphisms associated with increased risk of gastric cancer. Nature (2000) 404:398–402. doi: 10.1038/35006081
37. Hall SK, Perregaux DG, Gabel CA. Correlation of poly-morphic variation in the promoter region of the interleukin-1 β gene with secretion of interleukin-1β protein. Arthritis Rheum (2004) 50:1976. 2004. doi: 10.1002/art.20310
38. Leon Rodriguez DA, Echeverría LE, González CI, Martin J. Investigation of the role of IL17A gene variants in Chagas disease. Genes Immun (2015) 16:536–40. doi: 10.1038/gene.2015.42
39. Kim SW, Kim ES, Moon CM, Park JJ, Kim TI, Kim WH, et al. Genetic polymorphisms of IL-23R and IL-17A and novel insights into their associations with inflammatory bowel disease. Gut (2011) 60:1527–36. doi: 10.1136/gut.2011.238477
40. Zidan HE, Rezk NA, Alnemr AA, Moniem MI. Interleukin-17 and leptin genes polymorphisms and their levels in relation to recurrent pregnancy loss in Egyptian females. Immunogenetics (2015) 67:665–73. doi: 10.1007/s00251-015-0876-8
41. Correa JD, Madeira MF, Resende RG, Correia-Silva JF, Gomez RS, Souza DG, et al. Association between polymorphisms in interleukin-17 A and -17 F genes and chronic periodontal disease. Mediators Inflammation (2012) 2012:846052. doi: 10.1155/2012/846052
42. Reis PG, Ayo CM, de Mattos LC, Brandão de Mattos CC, Sakita KM, de Moraes AG, et al. Genetic Polymorphisms of IL17 and Chagas disease in the south and southeast of Brazil. J Immunol Res (2017) 2017(1017621):7. doi: 10.1155/2017/1017621
43. Leon Rodriguez DA, Carmona FD, Echeverría LE, González CI, Martin J. IL18 Gene Variants Influence the Susceptibility to Chagas Disease. PloS Negl Trop Dis (2016) 10:e0004583. doi: 10.1371/journal.pntd.0004583
44. Strauss M, Acosta-Herrera M, Alcaraz A, Casares-Marfil D, Bosch-Niccolau P, Lo Presti MS, et al. Association of IL18 genetic polymorphisms with Chagas disease in Latin American populations. PloS Negl Trop Dis (2019) 13(11):e0007859. doi: 10.1371/journal.pntd.0007859
45. Sanchez E, Palomino-Morales RJ, Ortego-Centeno N, Jimenez-Alonso J, Gonzalez-Gay MA, López-Nevot MA, et al. Identification of a new putative functional IL18 gene variant through an association study in systemic lupus erythematosus. Hum Mol Genet (2009) 18:3739–48. doi: 10.1093/hmg/ddp301 PMID: 19584085
46. Nogueira LG, Frade AF, Ianni BM, Laugier L, Pissetti CW, Cabantous S, et al. Functional IL18 polymorphism and susceptibility to chronic Chagas disease. Cytokine (2015) 73:79–83. doi: 10.1016/j.cyto.2015.01.037
47. Furuchó CR, Umezawa ES, Almeida I, Freitas VL, Bezerra R, Nunes EV, et al. Inconclusive results in conventional serological screening for Chagas’ disease in blood banks: evaluation of cellular and humoral response. Trop Med Int Health (2008) 13:1527–33. doi: 10.1111/j.1365-3156.2008.02172.x
48. Bennett JA, Riegel B, Bittner V, Nichols J. Validity and reliability of the NYHA classes for measuring research outcomes in patients with cardiac disease. Heart Lung (2002) 31:262–70. doi: 10.1067/mhl.2002.124554
49. Britto C, Cardoso MA, Wincker P, Morel CM. A simple protocol for the physical cleavage of Trypanosoma cruzi kinetoplast DNA present in blood samples and its use in polymerase chain reaction (PCR)-based diagnosis of chronic Chagas disease. Mem Inst Oswaldo Cruz (1993) 88:171–2. doi: 10.1590/s0074-02761993000100030
50. Gustincich S, Manfioletti G, Del Sal GS, Schneider G, Carninci PA. Fast method for high quality genomic DNA extraction from whole human blood. Biotechniques (1991) 11(3):298–300.
51. Sedaghat AR, Rastegar DA, O’Connell KA, Dinoso JB, Claus O, Wilke CO, et al. T Cell dynamics and the response to HAART in a cohort of HIV-1-infected elite suppressors. Clin Inf Dis (2009) 49(11):1763–6. doi: 10.1086/648081
52. Collins LM, Schafer JL. Kam CM. A comparison of inclusive and restrictive strategies in modern missing data procedures. Psychol Methods (2001) 6(4):330–51. doi: 10.1037/1082-989X.6.4.330
53. Barrett JC, Fry B, Maller J, Daly MJ. Haploview: analysis and visualization of LD and haplotype maps. Bioinformatics (2005) 21(2):263–5. doi: 10.1093/bioinformatics/bth457
54. Borges DC, Araújo NM, Cardoso CR, Lazo Chica JE. Different parasite inocula determine the modulation of the immune response and outcome of experimental Trypanosoma cruzi infection. Immunology (2013) 138:145–56. doi: 10.1111/imm.12022
55. Gao W, Pereira MA. Interleukin-6 is required for parasite specific response and host resistance to Trypanosoma cruzi. Int J Parasitol (2002) 32:167–70. doi: 10.1016/s0020-7519(01)00322-8
56. Giannitrapani L, Soresi M, Giacalone A, Campagna ME, Marasà M, Cervello M, et al. IL-6 -174G/C polymorphism and IL-6 serum levels in patients with liver cirrhosis and hepatocellular carcinoma. OMICS (2011) 15:183–6. doi: 10.1089/omi.2010.0093
57. Talar-Wojnarowska R, Gasiorowska A, Smolarz B, Romanowicz-Makowska H, Kulig A, Malecka-Panas E. Clinical significance of interleukin-6 (IL-6) gene polymorphism and IL-6 serum level in pancreatic adenocarcinoma and chronic pancreatitis. Dig Dis Sci (2009) 54:683–9. doi: 10.1007/s10620-008-0390-z
58. Giedraitis V, He B, Huang W-X, Hillert J. Cloning and mutation analysis of the human IL-18 promoter: a possible role of polymorphisms in expression regulation. J Neuroimmunol (2001) 112:146–52. doi: 10.1016/s0165-5728(00)00407-0
59. Hasan FT, Naif HM. Association of Gene Polymorphisms and Serum Levels of IL-18 with the Susceptibility to Infection with Hepatitis B Virus. J Infect Dis Med (2017) 2:117. doi: 10.4172/2576-1420.1000117
60. Chen DY, Hsieh CW, Chen KS, Chen YM, Lin FJ, Lan JL. Association of interleukin-18 promoter polymorphisms with WHO pathological classes and serum IL-18 levels in Chinese patients with lupus nephritis. Lupus (2009) 18:29–37. doi: 10.1177/0961203308094559
61. Dziedziejko V, Kurzawski M, Paczkowska E, Machalinski B, Pawlik A. The impact of IL-18 gene polymorphism on mRNA levels and interleukin-18 release by peripheral blood mononuclear cells. Postepy Hig Med Dosw (Online) (2012) 66:409–14. doi: 10.5604/17322693.1000980
62. Jurecekova J, Babusikova E, Sivonova MK, Drobkova H, Petras M, Kliment J, et al. Association between interleukin-18 variants and prostate cancer in Slovak population. Neoplasma (2017) 64:148–55. doi: 10.4149/neo_2017_119. 2017.
63. Sakai K, Kita M, Sawai N, Shiomi S, Sumida Y, Kanemasa K, et al. Levels of interleukin-18 are markedly increased in Helicobacter pylori-infected gastric mucosa among patients with specific IL18 genotypes. J Infect Dis (2008) 197:1752–61. doi: 10.1086/588196
64. Nascimento MS, Carregaro V, Lima-Junior DS, Costa DL, Ryffel B, Duthie MS, et al. Interleukin 17A Acts Synergistically With Interferon gamma to Promote Protection Against Leishmania infantum Infection. J Infect Dis (2015) 211:1015–26. doi: 10.1093/infdis/jiu531
65. Erdmann H, Rossnagel C, Bohme J, Iwakura Y, Jacobs T, Schaible UE, et al. IL-17A promotes macrophage effector mechanisms against Trypanosoma cruzi by trapping parasites in the endolysosomal compartment. Immunobiology (2013) 218:910–23. doi: 10.1016/j.imbio.2012.10.005
66. Pitta MG, Romano A, Cabantous S, Henri S, Hammad A, Kouriba B, et al. IL-17 and IL-22 are associated with protection against human kala azar caused by Leishmania donovani. J Clin Invest (2009) 119:2379–87. doi: 10.1172/JCI38813
67. Espinoza JL, Takami A, Nakata K, Onizuka M, Kawase T, Akiyama H, et al. A genetic variant in the IL-17 promoter is functionally associated with acute graft-versus host disease after unrelated bone marrow transplantation. PloS One (2011) 6:e26229. doi: 10.1371/journal.pone.0026229
68. Zingales B, Miles MA, Campbell DA, Tibayrenc M, Macedo AM, Teixeira MM, et al. The revised Trypanosoma cruzi subspecific nomenclature: rationale, epidemiological relevance and research applications. Infect Genet Evol (2012) 12:240–53. doi: 10.1016/j.meegid.2011.12.009
69. Luft T, Jefford M, Luetjens P, Hochrein H, Masterman KA, Maliszewski C, et al. IL-1 beta enhances CD40 ligand-mediated cytokine secretion by human dendritic cells (DC): a mechanism for T cell-independent DC activation. J Immunol (2002) 168(2):713–22. doi: 10.4049/jimmunol.168.2.713
70. Acosta-Rodriguez EV, Napolitani G, Lanzavecchia A, Sallusto F. Interleukins 1 beta and 6 but not transforming growth factor-beta are essential for the differentiation of interleukin 17-producing human T helper cells. Nat Immunol (2007) 8(9):942–9. doi: 10.1038/ni1496
71. Chandrasekar B, Melby PC, Troyer DA, Colston JT, Freeman GL. Temporal expression of pro-inflammatory cytokines and inducible nitric oxide synthase in experimental acute Chagasic cardiomyopathy. Am J Pathol (1998) 152(4):925–34.
72. Petersen CA, Burleigh BA. Role for interleukin-1 beta in Trypanosoma cruzi-induced cardiomyocyte hypertrophy. Infect Immun (2003) 71:4441–7. doi: 10.1128/IAI.71.8.4441-4447.2003
73. Chandrasekar B, Mummidi S, Claycomb WC, Mestril R. Nemer M.Interleukin-18 is a pro-hypertrophic cytokine that acts through a phosphatidylinositol 3-kinase-phosphoinositide-dependent kinase-1-Akt-GATA4 signaling pathway in cardiomyocytes. J Biol Chem (2005) 280(6):4553–67. doi: 10.1074/jbc.M411787200
74. Reddy VS, Harskamp RE, van Ginkel MW, Calhoon J, Baisden CE, Kim IS, et al. Interleukin-18 stimulates fibronectin expression in primary human cardiac fibroblasts via PI3K. J Cell Physiol (2008) 215(3):697–707. doi: 10.1002/jcp.21348
75. Eslick GD, Thampan BV, Nalos M, McLean AS, Sluyter R. Circulating interleukin-18 concentrations and a loss-of-function P2X7 polymorphism in heart failure. Int J Cardiol (2009) 137(1):81–3. doi: 10.1016/j.ijcard.2008.05.017
76. O’Brien LC, Mezzaroma E, Van Tassell BW, Marchetti C, Carbone S, Abbate A, et al. Interleukin-18 as a therapeutic target in acute myocardial infarction and heart failure. Mol Med (2014) 12:221–9. doi: 10.2119/molmed.2014.00034
77. Chien Y, Chien CS, Chiang HC, Huang WL, Chou SJ, Chang WC, et al. Interleukin-18 deteriorates Fabry cardiomyopathy and contributes to the development of left ventricular hypertrophy in Fabry patients with GLA IVS4+919 G>A mutation. Oncotarget (2016) 7(52):87161–79. doi: 10.18632/oncotarget.13552
78. Okuhara Y, Yokoe S, Iwasaku T, Eguchi A, Nishimura K, Li W, et al. Interleukin-18 gene deletion protects against sepsis-induced cardiac dysfunction by inhibiting PP2A activity. Int J Cardiol (2017) 243:396–403. doi: 10.1016/j.ijcard.2017.04.082
79. Fae KC, Drigo SA, Cunha-Neto E, Ianni B, Mady C, Kalil J, et al. HLA and β-myosin heavy chain do not influence susceptibility to Chagas’ disease cardiomyopathy. Microb Infect (2000) 2:745–51. doi: 10.1016/s1286-4579(00)00501-3
80. Matos CS, dos Santos JE Jr., Medeiros FAC, Furtado E, Dias JCP. Current situation and perspectives regarding human Chagas disease in midwestern of the state of Minas Gerais, Brazil. Mem Inst Osw Cruz (2014) 109:374–8. doi: 10.1590/0074-0276130385. Rio de Janeiro.
81. Silva JS, Barral-Netto M, Reed SG. Aggravation of both Trypanosoma cruzi and murine leukemia virus by concomitant infections. Amer J Trop Med Hyg (1997) 49:589–97. doi: 10.4269/ajtmh.1993.49.589
82. Andreani G, Celentano AM, Solana ME, Cazorla S, Malchiodi EL, Martínez Peralta LA, et al. Inhibition of HIV-1 replication in human monocyte-derived macrophages by parasite. Trypanosoma Cruzi PloS One (2009) 4(12):e8246. doi: 10.1371/journal.pone.0008246
83. de Freitas VL, da Silva SC, Sartori AM, Bezerra RC, Westphalen EV, Molina TD, et al. Real-time PCR in HIV/Trypanosoma cruzi coinfection with and without Chagas disease reactivation: association with HIV viral load and CD4 level. PloS Negl Trop Dis (2011) 5(8):e1277. doi: 10.1371/journal.pntd.0001277
84. Rodrigues DBR, Correia D, Marra MD, Giraldo LER, Silva EL, Silva-Vergara ML, et al. Cytokine serum levels in patients infected by human immunodeficiency virus with and without Trypanosoma cruzi coinfection. Rev Soc Bras Med Trop (2005) 38:483–7. doi: 10.1590/s0037-86822005000600007
85. Barbaro G. Reviewing the cardiovascular complications of HIV infection after the introduction of highly active antiretroviral therapy. Curr Drug Targets Cardiovasc Haematol Disord (2005) 5:337–43. doi: 10.2174/1568006054553444
86. Balagopal A, Gupte N, Shivakoti R, Cox AL, Yang WT, Berendes S, et al. Continued Elevation of Interleukin-18 and Interferon-γ After Initiation of Antiretroviral Therapy and Clinical Failure in a Diverse Multicountry Human Immunodeficiency Virus Cohort. Open Forum Infect Dis (2016) 3(3):ofw118. doi: 10.1093/ofid/ofw118
87. Iannello A, Boulassel M-R, Suzanne Samarani S, Tremblay C, Toma E, Routy J-P, et al. HIV-1 Causes an Imbalance in the Production of Interleukin-18 and Its Natural Antagonist in HIV-Infected Individuals: Implications for Enhanced Viral Replication. J Infect Dis (2010) 201:608–17. doi: 10.1086/650314
88. Sangenito LS, d’Avila-Levy CM, Branquinha MH, Santos ALS. Nelfinavir and lopinavir impair Trypanosoma cruzi trypomastigote infection in mammalian host cells and show anti-amastigote activity. Int J Antimicrob Agents (2016) 48(6):703–11. doi: 10.1016/j.ijantimicag.2016.09.017
89. Sangenito LS, Menna-Barreto RF D, Avila-Levy CM, Santos AL, Branquinha MH. Decoding the anti-Trypanosoma cruzi action of HIV peptidase inhibitors using epimastigotes as a model. PloS One (2014) 9(12):e113957. doi: 10.1371/journal.pone.0113957
90. Sangenito LS, Menna-Barreto RFS, Oliveira AC, d’Avila-Levy CM, Branquinha MH, Santos ALS. Primary evidence of the mechanisms of action of HIV aspartyl peptidase inhibitors on Trypanosoma cruzi trypomastigote forms. Int J Antimicrob Agents (2018) 52(2):185–94. doi: 10.1016/j.ijantimicag.2018.03.021
91. Castilho VVS, Gonçalves KCS, Rebello KM, Baptista LPR, Sangenito LS, Santos HLC, et al. Docking simulation between HIV peptidase inhibitors and Trypanosoma cruzi aspartyl peptidase. BMC Res Notes (2018) 11(1):825. doi: 10.1186/s13104-018-3927-z
Keywords: T. cruzi parasitemia, cardiomyopathy, Chagas disease, HIV, IL1 B, IL6, IL17 A and IL18 polymorphisms
Citation: Gomes dos Santos A, Watanabe EH, Ferreira DT, Oliveira J, Nakanishi ÉS, Oliveira CS, Bocchi E, Novaes CTG, Cruz F, Carvalho NB, Sato PK, Yamashiro-Kanashiro EH, Pontillo A, de Freitas VLT, Onuchic LF and Shikanai-Yasuda MA (2020) A Specific IL6 Polymorphic Genotype Modulates the Risk of Trypanosoma cruzi Parasitemia While IL18, IL17A, and IL1B Variant Profiles and HIV Infection Protect Against Cardiomyopathy in Chagas Disease. Front. Immunol. 11:521409. doi: 10.3389/fimmu.2020.521409
Received: 18 December 2019; Accepted: 24 September 2020;
Published: 22 October 2020.
Edited by:
David Courtin, Institut de recherche pour le développement (IRD), FranceReviewed by:
Thomas Jacobs, Bernhard Nocht Institute for Tropical Medicine (BMITM), GermanyFabrício C. Dias, University of São Paulo, Brazil
Celso Teixeira Mendes-Junior, University of São Paulo, Brazil
Copyright © 2020 Gomes dos Santos, Watanabe, Ferreira, Oliveira, Nakanishi, Oliveira, Bocchi, Novaes, Cruz, Carvalho, Sato, Yamashiro-Kanashiro, Pontillo, de Freitas, Onuchic and Shikanai-Yasuda. This is an open-access article distributed under the terms of the Creative Commons Attribution License (CC BY). The use, distribution or reproduction in other forums is permitted, provided the original author(s) and the copyright owner(s) are credited and that the original publication in this journal is cited, in accordance with accepted academic practice. No use, distribution or reproduction is permitted which does not comply with these terms.
*Correspondence: Luiz Fernando Onuchic, bG9udWNoaWNAdXNwLmJy; Maria Aparecida Shikanai-Yasuda, bWFzeWFzdWRhQHlhaG9vLmNvbS5icg==
†These authors have contributed equally to this work