- 1Molecular Virology and Immunology Research Group, Atta-ur-Rahman School of Applied Bio-Sciences, National University of Sciences and Technology, Islamabad, Pakistan
- 2Department of Surgery, Faculty of Medicine in Pilsen, Charles University, Pilsen, Czechia
- 3Laboratory of Cancer Treatment and Tissue Regeneration, Biomedical Centre, Faculty of Medicine in Pilsen, Charles University, Prague, Czechia
Wound healing and tissue regeneration is an intricate biological process that involves repair of cellular damage and maintenance of tissue integrity. Cascades involved in wound healing and tissue regeneration highly overlap with cancer causing pathways. Usually, subsequent tissue damage events include release of a number of cytokines to accomplish post-trauma restoration. IL-22 is one of the cytokines that are immediately produced to initiate immune response against several tissue impairments. IL-22 is a fundamental mediator in inflammation, mucous production, protective role against pathogens, wound healing, and tissue regeneration. However, accumulating evidence suggests pivotal role of IL-22 in instigation of various cancers due to its pro-inflammatory and tissue repairing activity. In this review, we summarize how healing effects of IL-22, when executed in an uncontrollable fashion can lead to carcinogenesis.
Introduction
IL-22 is a key signaling molecule that plays a significant role in number of essential physiological processes ranging from innate immune responses to tissue regeneration. Up-regulation and down-regulation of IL-22 generate several consequences that define its biological and pathological activities (1, 2). Owing to pivotal role of IL-22 in normal biological functions, any impairment in its activity can lead to chronic inflammatory diseases, disturbed wound healing, infections, and cancers (3, 4). This dual role highlights the therapeutic prospective of modulating the cytokine network to achieve tumor prevention and treatment.
Aims and Objectives
IL-22 is implicated in a large variety of functions in the body, given its involvement in signaling pathways. IL-22 has been shown to be a critical signaling molecule in regenerative processes and on the other hand, certain pathological conditions in several organs depending on its environmental factors, cytokine milieu and context. Because of its extensive involvement in regeneration, host defense and pathological conditions, IL-22 has become an important target for clinical and therapeutic development. Therefore, it is imperative to understand the detailed pathways of IL-22 that can convert a normal physiological process into a pathological environment.
In this review, we have, in detail, discussed the present state of knowledge about the biology of IL-22, its sources and targets, and its role in regeneration that could, in uncontrolled conditions, lead to tissue pathology or carcinogenesis.
IL-22 Biochemical Properties
IL-22, a novel protein, was discovered for the first time by Dumoutier et al. in the year 2000 (5). Initially, IL-22 was called Interleukin-10-related T cell-derived inducible factor due to its significant structural resemblance (homology of 22%) to mouse interleukin-10 (5). Further characterization and cloning of IL-22 revealed the structure of this cytokine to be alpha helical, comprising of 179 amino acids containing six α-helices which are usually known as A to F helices. A monomeric bundle like conformation is formed by these helices, which bind together in an anti-parallel fashion (6–8). IL-22 is a class II cytokine and has been categorized in the IL-10 family of cytokines due to its biochemical and functional characteristics, along with other interleukins including IL-10, IL-28, IL-26, IL-29, IL-24, and IL-20 (9). In humans, chromosome 12 contains the gene responsible for encoding IL-22. It is present upstream of the genetic loci for IFN-γ (interferon gamma) and IL-26 (10). The secreted form of IL-22 generally consists of 146 amino acids (5, 8, 11). Characteristically, the functional form of IL-22 exists as a monomer, but on the other hand, it also has the ability to form dimers and tetramers, which serve as non-functional storage forms (12).
IL-22 Receptors
For elicitation of its downstream effector functions, IL-22 employs its receptor complex which functions as a heterodimeric complex and is composed of two receptors; IL-22R1 and IL-10R2 (6, 13, 14). This receptor complex is able to further activate a multitude of downstream signaling cascades (14). IL-22R1 and IL-10R2 have been categorized in the class II cytokine receptor family (13). The gene for IL-22R1 is present on chromosome 1p36.11 in close proximity to the IL-28RA gene, and the gene responsible for encoding IL-10R2 is found on the chromosome 21q22.11, close to the IFNARA, IFNARB, and IFNGRB genes (15, 16). A very high binding affinity has been reported between IL-22 and IL-22R1, whereas it has been observed that IL-22 shows a low affinity for IL-10R2 (17, 18). When IL-22 encounters IL-22R1, it binds to the receptor owing to its high binding affinity, which in-turn results in the occurrence of a conformational modification of the cytokine. This modification causes an increase in the affinity of IL-22 to bind to IL-10R2 thereby allowing IL-22 and IL-22R1 complex to bind to IL-10R2 (7, 19). IL-22 receptor complex is transmembrane complex associated with Janus kinase (JAK) and Tyrosine Kinases (TYK). When IL-22 binds with its receptor complex, it triggers a number of downstream cascades including JAK, TYK2 phosphorylation which further phosphorylates and activates STAT-3 (signal transducer and activator of transcription 3) which is responsible for a wide array of downstream effects. Some other molecules including p38, ERK (extracellular signal-regulated kinases), JNK (c-Jun N-terminal kinases), PI3K (phosphoinositide 3-kinases) are also activated downstream of IL-22 binding, thereby mediating IL-22 in eliciting its effector functions (1, 20, 21).
IL-22 Binding Protein
Another receptor for IL-22, namely IL-22 binding protein (IL-22BP) exists in the form of a soluble receptor. It has been reported that IL-22 has a very high affinity for binding to IL-22BP (22, 23). IL-22BP acts as a natural antagonist of IL-22 since it binds to IL-22 at specific sites that are also utilized by IL-22R1, thereby blocking the binding of IL-22 to its membrane bound receptor (24). Owing to a stable association with IL-22, IL-22BP sequesters IL-22 away from IL-22R1 thereby precluding its downstream signaling activation (25). IL-22BP has been reported to be expressed in numerous tissues of the body, including lungs, skin, lymphatic tissue, placental tissue, breast, and gastrointestinal tract. Dendritic cells play an important role in producing IL-22BP, along with epithelial cells, macrophages, and eosinophils (26).
IL-22 Sources and Targets
T lymphocytes specifically CD4+ T cells or T helper (Th) cells act as the primary sources of IL-22 production (27). A unidirectional flow of cytokine signaling can be seen in the case of IL-22, as immune cells act as the main source of secretion for IL-22, while its main targets include non-hematopoietic epithelial cells. For this reason, IL-22 is considered as an essential factor of immune-epithelial cross talk. Th1 and Th17 cells are major producers of IL-22 [Figure 1; (28–30)]. IL-22 production by CD4+ cells is mainly mediated by cytokines like IL-23, IL-21, IL-12, IL-1β, IL-7, IL-6, TNF-α (Tumor Necrosis Factor) and some other molecules like Notch, RORyt and aryl hydrocarbon receptor ligand like FICZ [6-formylindolo[3,2-b] carbazole (31, 32)]. In Th17 cells, TGFβ (transforming growth factor beta), in the presence or absence of IL-6, plays an important role in the production of both IL-17 as well as IL-22 (9). Furthermore, in peripheral blood, another major source of IL-22 production is the Th22 cells. In the presence of TNF-α and IL-6, naïve T cells can be differentiated into Th22 cells and produce IL-22 (33, 34), which play an important role in tissue remodeling (35, 36). Notch receptor family and AhR androgen ligands mediate Th22 cells to produce IL-22 (37, 38). Transcription factor HIF-1a has also been reported to stimulate CD4+ cells to produce IL-22 (39). Furthermore, IL-22 Is also secreted by natural killer T cells, γδT cells, and CD8+ T cells when they are activated, particularly when IL-23 is present (40). Innate lymphoid cells are another key producer of IL-22 which include LTi cells (lymphoid tissue inducer cells), NCR (natural cytotoxicity triggering receptor) positive cells and NK cells (41–43). Non-hematopoietic cells, macrophages, and monocytes do not produce IL-22 in humans (28).
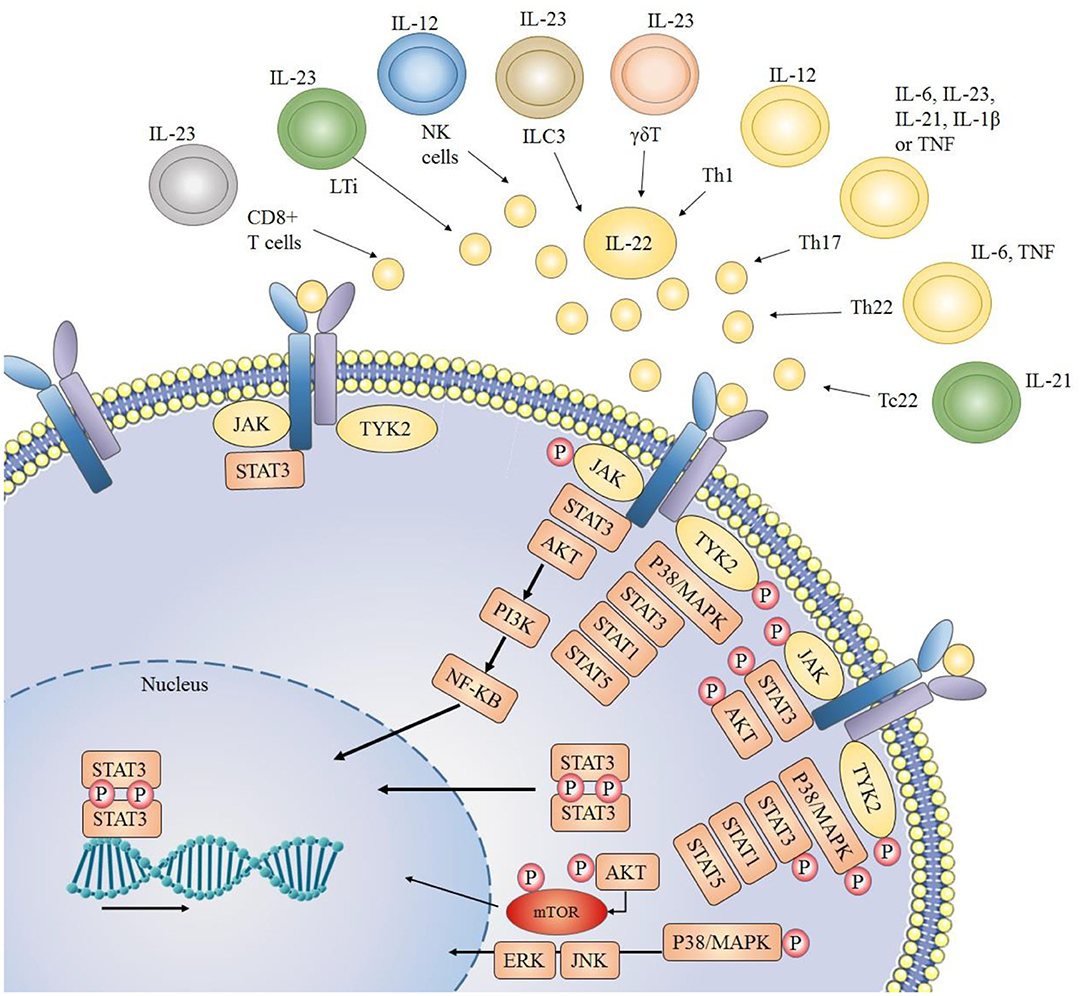
Figure 1. Sources of IL-22 and downstream signaling pathways: IL-22 is mainly secreted by immune cells. JAK1 (Janus kinase 1) and TYK2 (Tyrosine kinase 2) molecules are associated with the cytoplasmic region of IL-22 receptor. When the IL-22 receptor is activated (due to a complex formation between its two heterodimeric receptors), JAK1 and TYK2 are phosphorylated, which further phosphorylate STAT (signal transducer and activator of transcription) molecules thus resulting in dimerization and consequent translocation of STATs to the nucleus. Usually STAT3 molecules play key role, but STAT1 and STAT5 may also be involved. Other than JAK/STAT activation and downstream signaling, IL-22 also activates PI3K/AKT (phosphoinositide 3-kinases/protein kinase B) and MAPK (mitogen-activated protein kinase) pathways through JAK and TYK2 molecules.
Although ubiquitous expression of IL-10R2 is found throughout the body, the defining factor for the cellular sensitivity of IL-22 is expression of IL-22R1 (44). It is now well-recognized that T cells, B cells, Natural Killer cells, and other immune cells are not targeted by IL-22 (28). Instead, IL-22R1 expression is seen in tissue cell types that primarily construct epithelial barriers like bronchial epithelial cells (45), intestinal epithelial and subepithelial myofibroblast (46), skin keratinocytes and fibroblast (47), and thymic epithelial cells (48). Other than that IL-22 receptors are also expressed in hepatocytes (10), pancreatic acinar cells (49) and islet β-cells (50), kidney epithelial cells (51), and specific tissue resident stem cells (52). Varied levels of IL-22 has been observed depending upon the tissues and extracellular environment. A significantly reduced expression of IL-22 has been noticed in intestinal mucosa of ulcerative colitis patients due to increased concentration of TGF-β as compared to healthy colon tissues (53). On the other hand, over expression of Hes1 enhanced the Il-22 transcription in Intestinal Epithelial Tissue via STAT3 phosphorylation (54).
IL-22 Biological Effects
IL-22 carries out a number of biological effects including innate immune response, protective role against pathogens, and tissue regeneration. However, over generation of such responses can gradually lead to carcinogenesis (Figure 2).
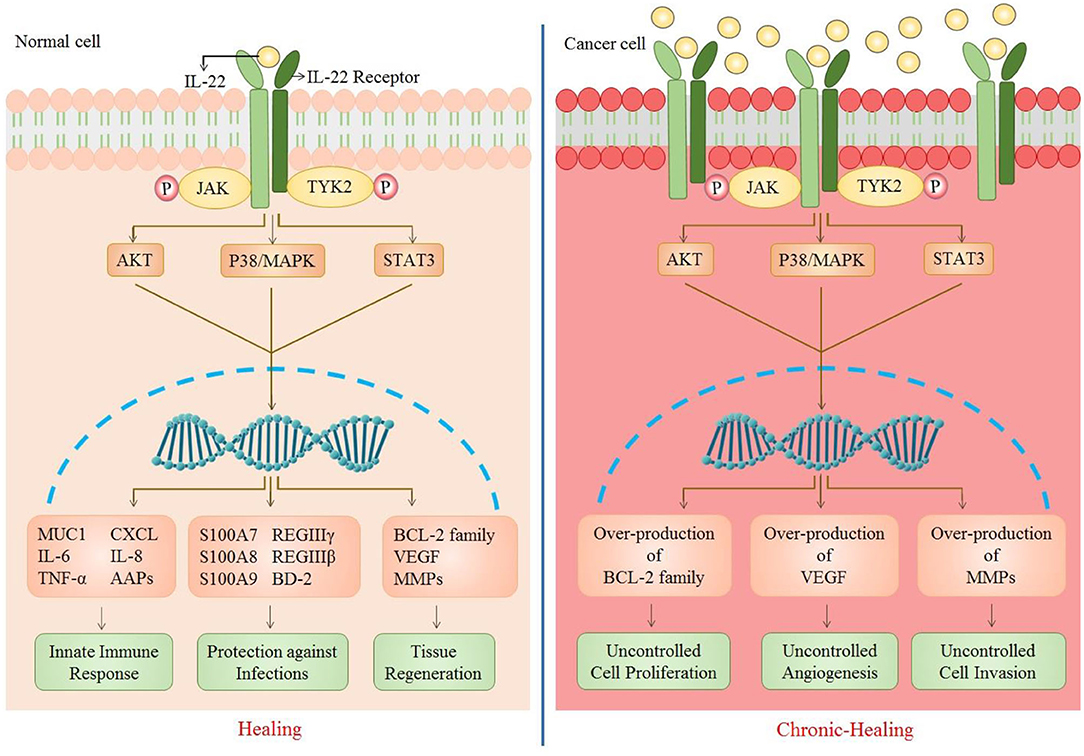
Figure 2. Role of IL-22 in normal vs. cancer cell. As IL-22 attaches to its receptor, a number of downstream signaling pathways are activated that further result in the upregulation of many genes. This causes an enhancement of biological functions including innate immune response (MUC1, IL-6, TNF-a, CXCL, IL-8, AAPs), protections against infections (S100A7, S100A8, S100A9, REGIIIy, REGIIIb, BD-2), and tissue regeneration (Bcl-2 family, VEGF, MMPs). The up-regulation of these genes is caused by multiple signaling pathways (JAK/STAT3, P38/MAPK, AKT). However, in the case of increased IL-22 or IL-22R, there is persistent activation of such signaling pathways which results in over-production of cell survival, angiogenic and metastatic genes [CXCL, chemokine (C-X-C motif) ligand 1; MUC1, Mucin1; AAP, Amyloid Precursor Protein; S100A7/8/9, S100 calcium-binding protein A7/8/9; REG, regenerating islet-derived protein 3; BD-2, beta-defensin 2; VEGF, vascular endothelial growth factor; MMPs, matrix metalloproteinases].
Innate Immune Response
Like other cytokines, IL-22 plays pivotal role in generating innate immune response against various infections. These responses involve in maintaining homeostasis at epithelial surface, recruitment of other immune cells by provoking inflammation and mucus production against pathogens (Figure 2).
Inflammation
IL-22 exhibits many pro-inflammatory effects in order to generate an immune response. In Colo205, a colon epithelial cell line, IL-22 effectively generates an acute phase response by up-regulating expression of serum amyloid protein, α-chymotrypsin, and haptoglobin (55). In response to any tissue damage or invasion, IL-22 is known to recruit neutrophils toward the site of infection as an inflammatory response. IL-22 attained it by persuading the production of certain chemokines like CXCL1, CXCL2, and CXCL5 in primary human keratinocytes (56). It has been observed both in mice and humans that in order to orchestrate inflammatory response, IL-22 affects the production of other pro-inflammatory cytokines which include IL-6, IL-8, and TNF-α. Such cascade reactions have been reported in hepatic stellate cells, myofibroblasts, and keratinocytes (57, 58). In cell line like human keratinocyte cell line HaCaT, IL-22 enhances NLRP3 inflammasome which yields IL-1β (59). This further insinuates IL-22 provoked oxidative signaling, achieved by Nicotinamide Adenine Dinucleotide Phosphate (NADPH) oxidase mediated STAT3 activation. This might synergize with IFN-γ to generate nitric oxide species (60). Pro-inflammatory activity performed by IL-22 has also been associated with several inflammatory diseases such as psoriasis and atopic dermatitis (61, 62). Furthermore, IL-22 mediated airway inflammation promotes atopic march which usually proceeds to asthma (63). However, the modulation of IL-22 expression done by IL-22BP lessens the damaging effect of inflammation in murine model (64).
On the other hand, in conditions like hepatitis, asthma, inflammatory bowel disease and cholangitis, anti-inflammatory response of IL-22, to counteract the disparaging effect of immune response, has also been observed (65–68).
Deficiency of IL-22, as observed in patients and Reconstituted Human Epidermis (RHE) tissues, leading to chronic inflammatory acne inversa points toward its anti-inflammatory role in homeostatic condition (69). It allows us to conclude that IL-22 is capable to perform both pro- and anti-inflammatory responses, depending upon tissue microenvironment including cytokine milieu (70).
Mucus Production
Mucus layer in certain epithelial cells serves as innate mechanism against both bacterial as well as viral infections and also for other toxins released by parasites (71). IL-22 serves to up-regulate a number of mucus associated genes, such as MUC1, MUC3, MUC10, and MUC13 in colonic and tracheal epithelial cells (46, 72). IL-22 along with another cytokine IL-17A upholds production of pulmonary mucus as a control against Gram negative pathogens like Klebsiella pneumonia (72). STAT3 activation via IL-22 gene delivery within colonic epithelial cells restitutes mucus-producing goblet cells. It rapidly ameliorates local intestinal inflammation in acute colitis murine model (46). Decline in IL-22 production is known to play role in HIV associated immunopathogenesis (73). It is suggested that IL-22 may persuade resistance against HIV infection in people who are exposed to virus several times by enhancing mucus production (74). As IL-22 enhances and maintains mucosal barrier integrity, its transient inhibition is also suggested for mucosal vaccines to increase T cell response (75).
Protective Role Against Infections
The well-documented role of IL-22 is prevention and defense against bacterial and other parasitic infections in various organs. It also protects against viral infections by reducing the follow-up infections and assisting in tissue retrieval (Figure 2).
Bacterial Infections
Production of antibacterial proteins is one of the main steps of IL-22 mediated immune responses in murine model (76). Commonly IL-22 elevates antimicrobial defense by up-regulating expression of certain antibacterial genes like psoriasin (S100A7), calgranulin-A (S100A8), calgranulin-B (S100A9), and Beta-defensin 2 (BD-2) independently or in combination with IL-17A of IL-17F as per studied in primary human keratinocytes as well as in patients' skin biopsies and blood plasma (29, 77). However, various studies on murine model demonstrated that IL-22 induced STAT3 controls bacterial growth in intestinal epithelial cells by enhancing expression of proteins specifically RegIIIγ (regenerating islet-derived protein 3) and RegIIIβ (78). When present at mucosal surfaces, RegIII proteins serve to exhibit antimicrobial action against gram-positive bacteria by binding to the peptidoglycan moieties of bacteria and induce damage to the bacterial cell wall and separate microbiota from intestinal epithelial cells to maintain a symbiotic host-bacterial relationship (79, 80). Similarly, recombinant porcine IL-22 activates STAT3 signaling to protect against Escherichia coli infection (81).
IL-22 plays crucial role in protecting the skin, gastrointestinal and respiratory tract from both pathogenic and commensal bacterial infections. Its activity is critical for regulation of gut microbiota. Depletion of IL-22 producing innate lymphoid cells causes peripheral diffusion of intestinal commensal bacteria Alcaligenes. This dissemination is sufficient to induce systemic inflammation which has potential to facilitate Crohn's disease and progressive hepatitis C viral infection (82). Similarly, upon respiratory infection with Streptococcus pneumonia, type 3 lymphoid cells (ILC3) accumulate in lung tissue. In murine model, this amassing of ILC3 is followed by production of IL-22 that defends against lethal infection (83). Knocking out of IL-22 binding protein (IL-22BP), which reduces IL-22 activity, is found to decrease pneumococcal murine lung infection (84). Natural killer cells are reported to provide immunity against Mycobacterium tuberculosis (Mtb) by releasing IL-22 in infected patients, which constrains intracellular bacterial growth by accelerating phagolysosomal activity (85). IL-22 also provides immunity against rapidly emerging Mtb HN878 strain in mice (86). Mechanism underlying this inhibition involves IL-22 dependent up-regulation of calgranulin A, an intracellular signaling molecule that induces phagolysosomal fusion (87). IL-23 dependent IL-22 production is shown to protect against Salmonella enterica, which is known to cause diseases ranging from minor gastroenteritis to serious systemic infections. In absence of IL-22, murine model developed liver necrosis upon Salmonella infection (88). In case of chronic Salmonella gastroenteritis model, antibody mediated IL-22 neutralization disrupted the epithelial barrier of intestine and increased the production of pro-inflammatory cytokines in porcine intestinal epithelial cells (81). IL-22 mediated anti-bacterial activity against Salmonella is carried out by phagolysosomal fusion in intestinal epithelial cells (89). That's why prompt up-regulation of IL-22 by dendritic cells is seen at site of Salmonella infection to cause resistance against salmonellosis (90). Furthermore, relative deficiency of IL-22 is seen in Acne Inversa patients which occurs due to chronic inflammation caused by persistence cutaneous bacterial infection (69).
However, contrary to IL-22 protective role against bacterial infections, one study done on BALB/c mice, implied its dispensable role in immunity against opportunistic pathogens like Mycobacterium avium and Mycobacterium tuberculosis (91). Interestingly, IL-22 up-regulation after Listeria monocytogenes infection was seen in murine model, though, no clear effects on both primary and secondary bacterial infection were observed (92). Its pro-inflammatory activity is also connected with bacterial spread leading to organ failure in septic peritonitis observed in murine models (93). Elevated level of IL-22 in plasma has also been related with psoriasis, which markedly reduced after anti-psoriatic therapy. In this case, IL-22 mediated over-expressions of antibacterial proteins become the cause of disease severity (77).
Ample data elucidate how IL-22 exhibits antibacterial activity by inducing various innate defense mechanisms in epithelia. However, in some cases its production is inferred to play roles other than clearance of bacterial infection, which may also lead to pathogenicity.
Fungal Infections
IL-22 contribution to protect against fungal infection was first suggested by a study conducted on mice. Aspergillus fumigatus infection in lungs was reported to be limited by IL-22 mediated immunity (94). Acute A. fumigatus exposure instigates IL-7 and IL-21 which further regulate the IL-22 production to carry out anti-fungal effects (95). IL-22 along with IL-17F is a vital natural defender against Chronic Mucocutaneous Candidiasis (CMC). IL-22 provides first line of defense against Candida albicans as observed in humans and murine models, which comprises monitoring of fungal growth. And it is required to seize dissemination of intragastric infection of C. albicans to other organs like stomach and kidney (96, 97). In addition, IL-22 exhibit mild role in protecting against cutaneous candidiasis in mice (98). Likewise, mice deficient in IL-22 were slightly susceptible to oropharyngeal candidiasis, indicating a role of IL-22 against oral candidiasis (99). Contrary to given data, IL-7 mediated IL-22 production orchestrates immunopathogenic reactions in fungal asthmatic patients (100, 101).
Viral Infections
According to previous studies, IL-22 was known to be incapable of having direct antiviral response, though its profound role in post-viral infection tissue repair is well-described. However, recently IL-22 is identified to exhibit a novel role in regulating antiviral T cell response in non-lymphoid and lymphoid organs in the course of acute and persistent viral infections (102). Upon influenza viral infection, Dendritic Cells (DCs), and activated Natural Killer T Cells (NKT cells) are ultimate source of IL-22 (103). This rapid production of IL-22, in murine model is implied to retain beneficial role in preserving bronchoalveolar, tracheal, and lung epithelial integrity (104), and post-infection tissue restoration (105). IL-22 production in sublethal H3N2 influenza virus infection is known to limit lung inflammation and specifically subsequent secondary bacterial infections (106, 107). Human immunodeficiency virus (HIV) and other similar viruses like Simian immunodeficiency virus (SIV) spread via multifocal injury of gastrointestinal epithelial barrier. Perturbance in mucosal immunity on onset of SIV infection is known to be correlated with loss of IL-17 and IL-22 expression (108). Similarly, impairment in IL-22 production due to Th22 depletion in mucosal gut is a significant factor in HIV mediated mucosal immunopathogenesis (73). Anti-HIV candidate Abx464 reduces intestinal inflammation by stimulating activated macrophages to produce more IL-22 in mice model (109). Inoculation of IL-22 along with IL-18 to murine model reiterated the capability of flagellin to inhibit or eradicate Rotavirus (RV) infection (110). In addition, IL-22 and Interferon lambda (IFN- λ) both retain the integrity of intestinal epithelial cells to curtail RV replication (111). Kaposi's sarcoma-associated herpesvirus (KSHV) latency depends upon viral mediated IL-22 down-regulation to suppress antiviral response (112). However, in another study performed on mice pathogenic role of IL-22 is explicated where IL-22 signaling is reported to exacerbate lethal West Nile Virus (WNV) encephalitis prospectively due to WNV neuroinvasion (113). Correspondingly, IL-22 deficient mice upon Zika Virus inoculation revealed lessened weight loss, systemic inflammation, neurological disorders and mortality (114).
IL-22 up-regulation is reported multiple times in liver patients with chronic Hepatitis B Virus (HBV) or Hepatitis C Virus (HCV) infection (115–117). IL-22 can manifest a protective as well as pathogenic role in the liver, by impediment of apoptosis and stimulation of stem cells. Both outcomes can employ their protective tasks by increasing number of hepatocytes or can exhibit their pathological effects by enhancing production of chemokines, matrix metalloproteinases (MMPs), and neutrophil recruitment toward the liver in humans and mice (118, 119). IL-22 pro-inflammatory response following HBV recognition by liver T cells might play beneficial role in infection (120). IL-22, mainly manufactured by hepatic γδ T cells is shown to attenuate liver injury in case of adenovirus-infected murine model (121). In contrast with IL-22 beneficiary effect, its association with prognosis in chronic liver inflammation, fibrosis and Hepatitis B virus-related acute-on-chronic liver failure (HBV-ACLF) have also been studied (122–124). HCV induced IL-22 up-regulation in liver cells does not itself regulate antiviral proteins and HCV replication in cell lines (125). Though, protection against liver fibrosis and cirrhosis caused due to HCV infection is seen which can be varied due to genetic alteration in IL-22 gene (4, 126).
Wound Healing and Tissue Regeneration
IL-22 binding with its receptor complex stimulates downstream signaling pathways that activate cell survival genes. Up-regulation of cell proliferation genes supports tissue redevelopment and wound healing [Figure 2; (127)]. IL-22 mediated myofibroblast differentiation and production carry out skin wound healing process in mice (128). Upon skin inflammation, increase in IL-22 expression aids keratinocyte proliferation and migration toward site of injury. Meanwhile, during the process of wound healing, keratinocyte differentiation is repressed by IL-22 (127, 129, 130). Deficiency of IL-22 in injury has shown compromised granulation, ECM production and formation of tissue and wound contraction. Primary dermal fibroblast bears IL-22R1 which, when bind with IL-22, stimulates downstream JAK/STAT pathway. Following dimerization and translocation of STAT molecules into nucleus, results in enhanced production of ECM, fibronectin, and collagen. Therefore, in IL-22 deficient murine model, faulty wound contraction, and ECM production are observed (131). Likewise, in another study, absence of IL-22 resulted in defective recovery from DSS-caused intestinal injury in mice. Alternatively, a gene delivery system increasing the IL-22 expression in intestinal mucosa enhanced intestinal repair (78). A separate study elucidates that IL-22 deficiency can also alter the intestinal microbiota thus worsening disease severity. This study also signifies the importance of IL-22 in maintaining the balance between microbiota of the intestine and intestinal immunity (132).
IL-22 is also recognized to play key role in tissue regeneration after an injury. Hepatocyte proliferation serves as a requisite process in order to undergo tissue regeneration following hepatectomy. In that scenario, post-surgery IL-22 activation helps in orchestrating cell survival and proliferation genes (133, 134). Accumulating evidence through studies on mice suggest that IL-22 mediated liver regeneration is carried out in collaboration with IL-6 and TGF-α signaling cascades (135). Similarly, IL-22 induced STAT3 triggering activates intestinal stem cells to regenerate epithelium (136, 137). Up-regulated IL-22 expression during thymic recovery allows for the renewal and regeneration of thymus (48). In case of allogeneic hematopoietic cell transplant, donor T-cell derived IL-22 not only regenerates insulted thymus, but also reduces chronic graft-versus-host disease (GVHD) likelihood. It is achieved by IL-22 directed enhanced expression of Aire, an autoimmune regulator in murine model (138). Upon influenza virus infection, tracheal epithelial cells underwent proliferation and regeneration in response to IL-22 expressed in wild-type mice (104). Though a regenerative potential of IL-22 in renal tubular injury is well-defined, however, in glomerular disease, redundancy in IL-22 expression was observed (139). In addition, IL-22 has been shown to stimulate β-cells expression of Reg proteins in pancreas. Reg proteins are believed to participate in regenerating islets (140). However, regenerative cell survival signaling of IL-22 has a profound potential to shift toward tumor formation, when over-activated in uncontrolled manner. It was also observed that IL-22R deficient mice had a delay in wound healing. IL-22, along with IL-20 and IL-24 enhances wound healing in type II db/db diabetic mice by inducing genes involved in re-epithelialization, tissue remodeling and innate host defense mechanisms from injured skin (141).
Cellular sources of IL-22 has been observed to vary in different tissues. As per studies, Th-22 cells represent the major source of IL-22 in skin (34). Whereas, NK cells contribute as releasing factor of IL-22 in gut cells at time of inflammation in patients like ankylosing spondylitis (142). Moreover, STAT-3 mediated IL-22 signaling is associated with mucosal wound healing in epithelial cells of intestine (78). Similarly, Lymphoid tissue inducer (LTi) is known to be a source of IL-22 in fetal lymph nodes and spleen for its regenerative functions (143). Higher levels of IL-22 in keloid scars have also been observed, however, its exact role whether as healing or pathological factor is still needed to be explored (144).
IL-22 in Cancers
Despite the well-documented involvement of IL-22 in inflammatory responses leading to wound healing and tissue regeneration, in certain circumstances, where there is a disturbance in the intricate balance between repair and damage, IL-22 can serve in the direction of carcinogenesis. This is due to the participation of pathways favoring cellular proliferation and survival that are common in both wound healing and carcinogenesis. Consequently, IL-22 has the potential of promoting carcinogenesis in organs that are responsive to IL-22 due to the presence of its receptor (Figure 2).
Colorectal Cancer
Chronic intestinal inflammation and prolonged tissue damage are two of the main factors that predispose the colon mucosa to carcinogenesis. This can be attributed to the underlying mechanisms involved in wound healing and their potential to lead toward carcinogenesis in case of a disrupted balance. This can be based on the fact that signaling pathways driving the processes of tissue repair and cancer development share many similarities and several common factors. Therefore, in order to evade the development of cancer, tight control should be in place to balance the factors mediating tissue repair in case of tissue damage. A long term tissue healing response is required in the presence of persistent tissue damage or chronic inflammation. However, it can ultimately result in fibrosis and carcinogenesis. Therefore, development of cancer can be deemed as an outcome of excess and abnormal healing occurring due to failed regulation of signaling (Figure 3).
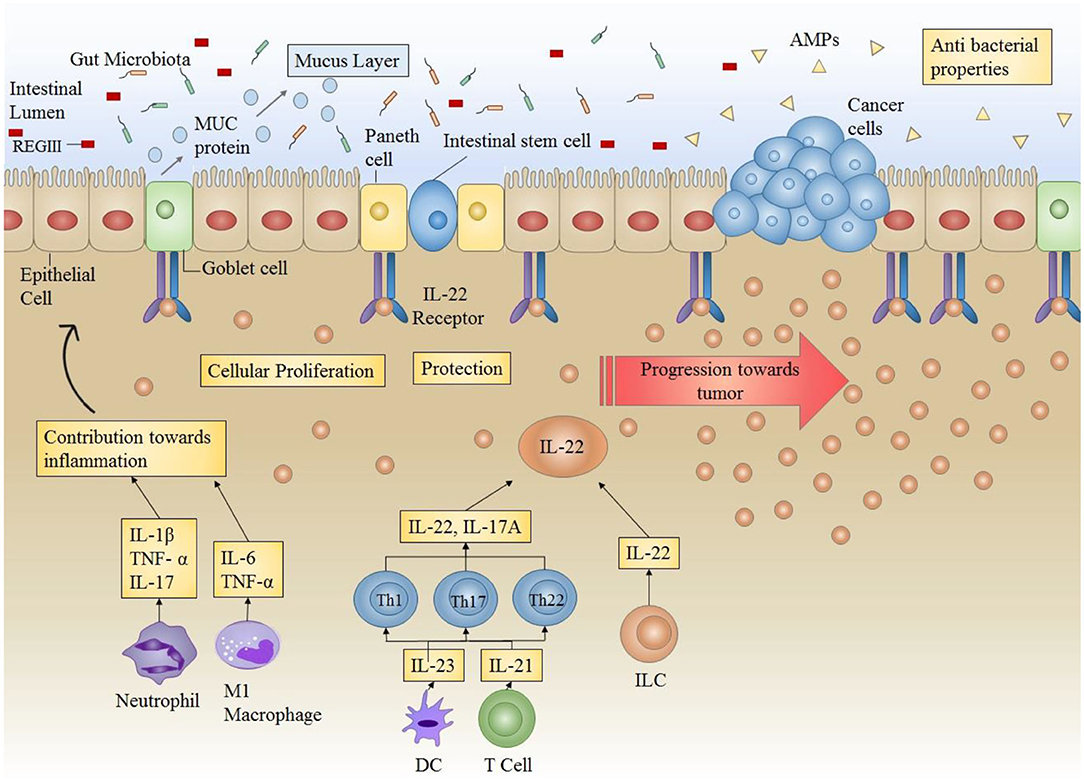
Figure 3. Role of IL-22 in the intestinal microenvironment: Under normal circumstances, IL-22 plays a very important role in providing protection against infections by increasing the production of AMPs (antimicrobial peptides) (including REGIII) thus enhancing antibacterial competence. IL-22 also increases mucus secretion by producing mucins (MUC) in order to ensure protection of epithelial layer and maintenance of epithelial barrier. Furthermore, IL-22 also directly acts on epithelial stem cells to enhance cellular proliferation. IL-22 in conjunction with other cytokines maintains the overall environment for protection against infections and inflammations. However, in case of prolonged or uncontrollable expression (as in colitis), IL-22 can result in progression of tumor formation.
Increasing evidence shows an involvement of IL-22 in progression of colorectal carcinogenesis. Key cellular sources responsible for producing IL-22 in the setting of colon cancer include ILCs and Th22 cells. IL-22 producing CD4+ T cells have been shown to enhance the stemness of colorectal cancer (145). Colonic dendritic cells, irrespective of their maturation level are responsible for secreting high IL-22 levels (146). In murine models, boosted levels of IL-22 reveal an indispensable role of this cytokine in the enhancement of tumor burden and decline in survival rate (147). Furthermore, by augmenting cellular proliferation and up-regulating inflammation, ILCs have proven to be essential in transitioning intestinal inflammation into colorectal cancer (148, 149).
A direct association has been reported between stagings of colorectal cancer with IL-22. IL-22 administration results in the acquisition of chemoresistance in human colorectal cancer cell lines (150). In a murine model of colitis mediated carcinoma, deficit in IL-22 ensured the development of fewer tumors. At the molecular level, IL-22 promotes carcinogenesis of colon cancer cells by activating STAT3, AKT, MAPK, and NFκβ. The enhancement of tumor cell proliferation also occurs as a result of modification of p16 and p21 promoters which are cell cycle checkpoint genes, thus resulting in heightened carcinogenicity (151, 152). IL-22 demonstrates a dual nature in facilitating carcinogenesis. The reduced expression of this cytokine outcomes in hampered tissue repair thus prolonging the process of inflammation and ultimately results in carcinogenesis. On the other hand, enhanced IL-22 expression can extend tissue regeneration process and also stimulate the development of colon cancer (25). In conformity with studies, the removal of ILCs in murine model of inflammation mediated carcinogenesis of colon cells shows a decrease in tumor formation. Similarly, anti-IL-22 antibody administration restored the symptoms associated with colitis along with the reduction of cancer burden (52). Based on these findings, it is concluded that a tight regulation and controlled release of IL-22 is required for effective wound healing that does not progress to carcinogenesis.
Gastric Cancer
IL-22 has been revealed to act as a pro-tumor cytokine in the cancers associated with gastrointestinal tract. The infiltration of cellular sources of IL-22 including Th22 and CD4+ T cells in the intratumoral tissue increases, corresponding to the stage of tumor (153–155). Patients with gastric tumors have also been shown to have increased levels of circulating T cells secreting IL-22 as well as IL-17, thus showing a positive association with tumor progression (154). In gastric cancer patients, IL-22R1 expression is augmented that indicates a positive correspondence with the stage of cancer and lymphatic invasion (156). Chromosomal mutations including single nucleotide polymorphisms and chromosomal gains have also been reported in gastric cancers. A SNP was found in the IL22 locus which was linked to an increase of about 2.5-folds in the risk of development of gastric tumors (157). Chromosomal gains at the IL22RA1 locus 1p36.11 are also observed which suggests that gastric cancer cells show increased responsiveness to IL-22 due to a high copy number of IL-22R1 (158). IL-22 gene polymorphisms were found to increase the susceptibility of developing gastric cancer in a study that was conducted on Asian population (159). Furthermore, IL-22 has also been described to aid metastasis of gastric tumor cells via increasing the expression of matrix metallopeptidases. Elevated levels of matrix metallopeptidases enhance the invasiveness of tumors (160). Various studies have shown boosted levels of IL-22 in chemotherapy resistance patients to FOLFOX4 adjuvant chemotherapy. Moreover, IL-22 mediated drug resistance has also been observed in 5-Fluorouracil and Oxaliplatin treated colon cancer cell lines (150).
Pancreatic Cancer
In humans, the highest expression of IL-22R occurs in the pancreatic acinar cells, therefore pancreatic cells are one of the main targets of IL-22 (49, 50). Many studies reveal that in pancreatic inflammation, IL-22 elicits a protective role (161, 162). This is achieved through up-regulation of anti-apoptotic genes including RegIII, thus ensuring the survival of cells and stimulating tissue repair. Mice deficient in IL-22 exhibit a worsening of tissue damage in case of pancreatitis. And this results in an increase in fibrosis (77). However, in the instance of persistently increased IL-22 expression and signaling dysregulation, this protective role can be easily manipulated into a carcinogenic one (163). In pancreatic cancer cell lines, production and release of various tumor enhancing factors including VEGFα, Interleukin 10 and TGF-β are stimulated by IL-22. IL-22 mediated ductal adenocarcinomas of the pancreas expresses elevated level of IL-22 and IL-22R1, concomitant with enhanced MMP production and invasion of lymph nodes. This illustrates the pro-tumor functions of IL-22 [Figure 4; (164, 165)]. Elated expression of systemic IL-22 positively correlates to poor prognosis of patients undergoing resection for pancreatic ductal adenocarcinomas. Therefore, the increased intra-tumoral infiltration of cellular sources of IL-22 including CD4+ cells and Th22 cells elevated IL-22 levels entail a poor patient survival outcome (119). IL-22 has been shown to increase the tumorigenicity and stemness of pancreatic cancer cells, through JAK/STAT3 signaling (166). IL-22 increases the expression of VEGF, anti-apoptotic gene Bcl-X and some immunosuppressive cytokines (167). Likewise, through STAT-3 dependent up-regulation of MMP9, in vitro IL-22 has been shown to enhance the metastatic potential of pancreatic cancer cell lines (168).
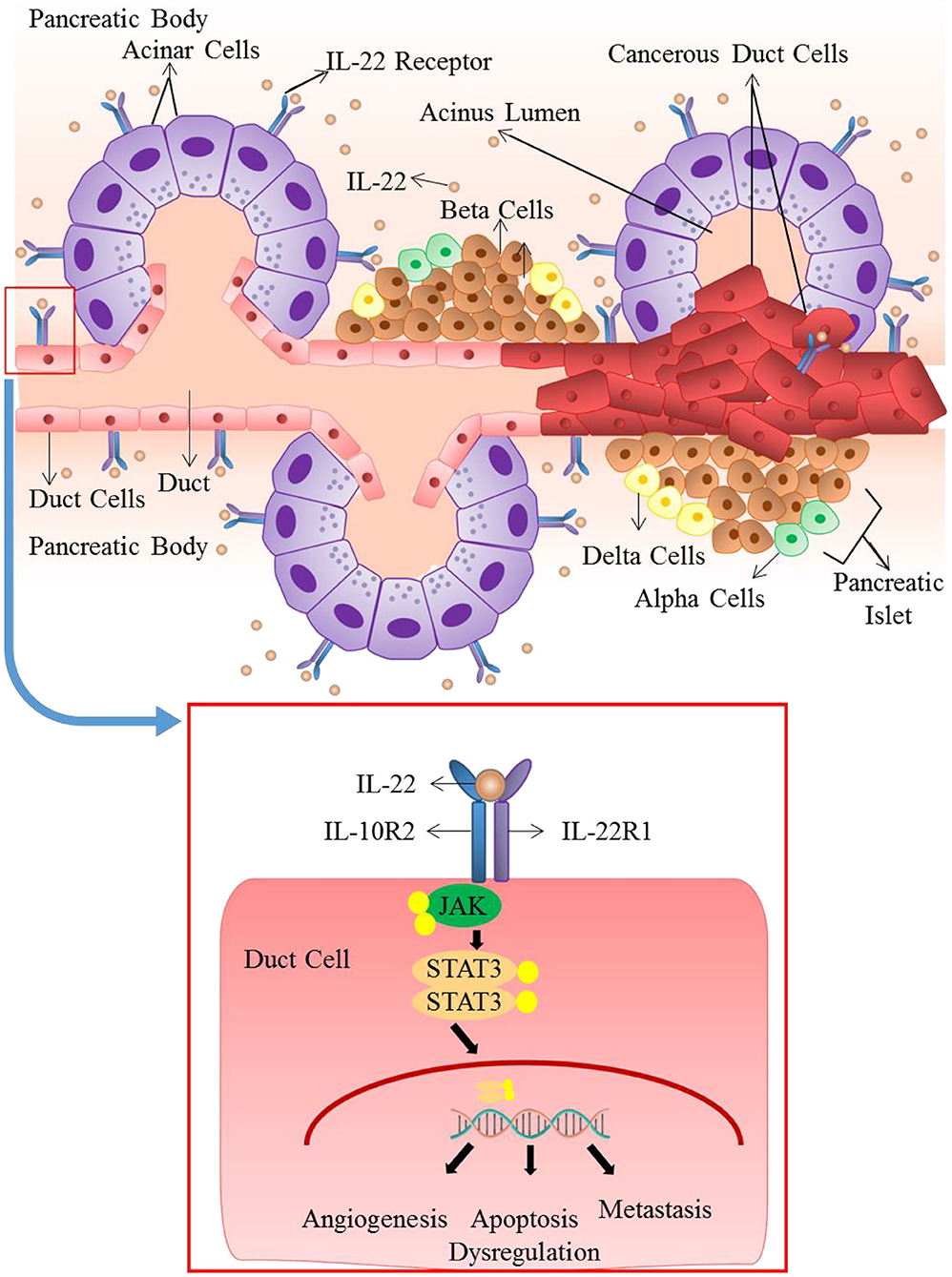
Figure 4. Role of IL-22 in pancreatic ductal adenocarcinoma: IL-22 mediated ductal adenocarcinomas of the pancreas expresses elevated levels of IL-22 and IL-22R1. Dysregulated and persistently elevated levels of IL-22 stimulates the production and release of various tumor enhancing factors through activation of JAK/STAT3 pathway. It results in apoptosis dysregulation, angiogenesis and metastasis that lead to advancement of cancer.
Skin, Lung, and Brain Cancer
In spite of the fact that IL-22 has a significant function in autoimmunity and the inflammatory responses of skin, involvement of IL-22 in skin cancer is rarely documented. One study reports, an increased infiltration of cells secreting IL-22 specifically Th22 cells in squamous cells carcinoma of skin. This infiltration and recruiting of Th22 cells to the site of cancer was partially due to MMP10 and S100A15 (169). A higher number of these cells were also found in basal cell carcinoma. IL-22 increased the proliferative as well as migratory potential of both basal cell carcinoma as well as squamous cell carcinoma of skin through STAT3 pathway and AKT (170). CSA (Cyclosporin) is involved in increasing the Squamous Cell Carcinoma (SCC) by favoring polarization of Th22 which results in increased IL-22 production and thus the blockage of IL22 by using anti-IL-22 antibody could potentially become a viable therapeutic option (31). Keratinocyte's response to IL22 is increased by UVB resulting in skin inflammation (171). Another in vitro study reveals that IL-22 and IL22R1 expression is enhanced in tumorous and peri-tumorous tissues, where cellular proliferation may be encouraged by IL-22 (172). It has also been reported that IL-22 elevated in lung cancer and renders lung cancer cells resistant to chemotherapy (173). In patients with malignant pleural effusions in non-small lung cancer, increased IL-22 levels have been associated with a poorer prognosis and survival rate (173). IL-22 has also been shown to be increased in non-functioning and prolactin-secreting macroadenomas of the pituitary (174). Murine model of glioma illustrates that increased IL-22 levels in brain can worsen the symptoms. This may occur due to enhanced IL-6, TNF, and IL-1β production stimulated by IL-22. Correspondingly, by using anti-IL-22 antibody or in IL-22 knock-out mice, the decreased IL-22 expression exhibited protective roles in the development of glioma (175).
Liver Cancer
IL-22 has an imperative role in liver regeneration and liver tissue healing. IL-22 shows the ability to reverse the damages caused to liver by activating wide range of healing agents and signaling pathways (10, 67, 135, 176–179). However, in the setting of chronic inflammation as in the case of chronic HCV and HBV infections, persistently elevated levels of IL-22 seem to aid the process of carcinogenesis (180). Hepatocellular carcinoma (HCC) tumor-infiltrating lymphocytes (TILs) are reported to be enriched with IL-22 in consort with elevated expression of IL-23 and IL-22BP in cancerous tissue. Enhanced level of IL-22 has been positively correlated with formation and staging of tumors (181). Reportedly, IL-22 stimulates STAT3 phosphorylation which elevates the expression of proto-oncogenes in HCC (168). A study showed that IL-22 liver specific transgenic murine model was more prone to develop diethylnitrosamine-induced tumorigenesis (124). In agreement to this, when IL-22 expressing TILs derived from HCC patients were introduced into lung cancer xenograft murine model, it orchestrated oncogenic signaling pathways in the tissue (181). Overall, IL-22 elevated levels in murine models and liver cancer patients point toward its vital role in liver tumor development and progression.
Manipulation of IL-22 Axis for Therapeutic Purposes
The IL-22—IL-22 subunit (IL-22R1) axis has shown a high potential clinical relevance in inflammatory diseases like psoriasis, ulcerative colitis, liver and pancreatic damage, graft-versus-host disease, certain infections, and tumors.
A direct IL-22-neutralizing antibody, ILV-094, has completed Phase I and II trials for psoriasis and rheumatoid arthritis, respectively. Neutralization of IL-22 may improve disease control and quality of life for late stage disease patients by reducing metastasis, chemoresistance and inflammation associated with cancer (182). Single i.v. dose of recombinant IL-22 (3.5 μg per mouse) resulted in Attenuation of acetaminophen (paracetamol)-induced liver damage (183). In liver, in vivo IL-22 cDNA delivery resulted in protection from ConA-, carbon tetrachloride-, and Fas-induced liver damage (184). These approaches show great potential for future therapeutics aimed at enhancing tissue repair or preventing cancer development.
Conclusion and Future Directions
T helper cells (including Th1, Th17, and Th22) are the primary producers of IL-22. Innate lymphoid cells are another key producer. IL-22 is an inflammatory cytokine so it is produced as a result of tissue injury and is clearly detectable in plasma following local tissue inflammation. Therefore, in inflammatory conditions, like in tissue injury, or infection, IL-22 level is significantly enhanced. Environmental factors, including cytokines (mentioned in section IL-22 Sources and Targets as the causes for induction of IL-22 production), metabolites, and oxygen play an important role in regulating the time and place of increasing IL-22 expression as well as shutting it down (77). In the case of acute inflammation, IL-22 generally plays a protective role like in skin, liver, colon, pancreas, lungs, and epithelial cells, however, in the case of chronic inflammation, this protective role can be converted into a pathological one. The epithelial cells of many organs, liver cells, fibroblasts, and pancreatic cells are considered to the principal target of interleukin-22 (77). IL-22/IL-22R1 axis alter signaling in target cells. The epithelial cells respond by enhancing the expression levels of many different proteins including anti-bacterial proteins like BD-2, S100A7-9, LCN2 (lipocalin-2) chemokines that attract granulocytes (CXCL 1, 5, and 8) and some MMPs. In the respiratory cells of colon and lungs, IL-22 causes the target cells to generate MUC-1. In skin epithelium, IL-22 has shown to decrease the expression of certain proteins implicated in cellular differentiation of epithelial cells (for example keratin 1 and keratin 10, desmocollin 1, and involucrin). The chief targets of IL-22 are the pancreatic cells and liver cells, where IL-22 upregulates Bcl-2, Mcl-1 (important antiapoptotic proteins), mitogenic proteins (like p21 and RBL2), and Bcl-XL. Anti-bacterial proteins like REG3 are also upregulated (178). Additionally, in liver cells, IL-22 stimulation also enhances the levels of some acute phase proteins, including haptoglobin LPS binding protein and serum amyloid A (135, 185). In rheumatoid arthritis patients, CC chemokine ligand 2 (a chemokine that attracts monocytes), and RANKL (receptor activator of NF-kB ligand), that stimulates monocytes to be differentiated into osteoclasts, are also increased by IL-22 (186). No effect of IL-22 on activated or resting immune cells is considered to be an important property of IL-22. IL-22 also synergizes with other cytokines like IL-17 and interferon gamma to elicit its role Table 1.
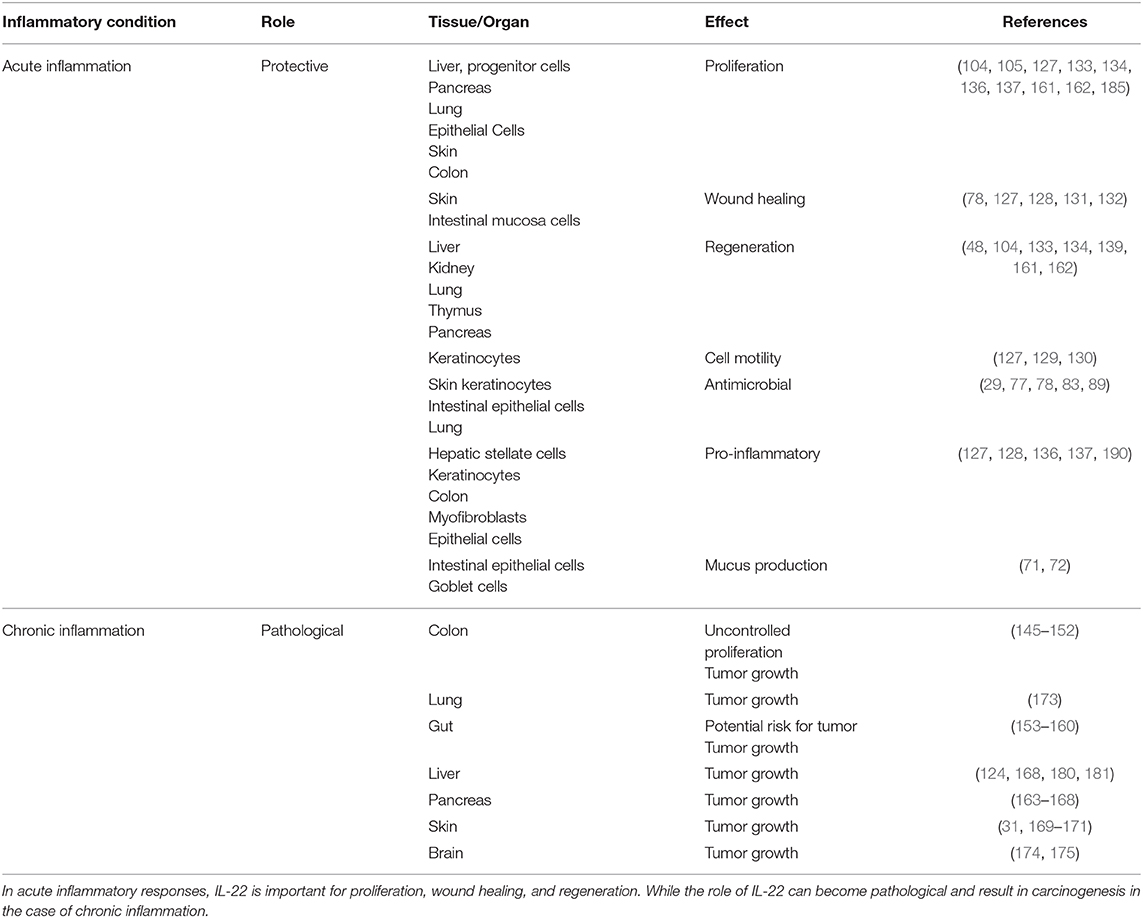
Table 1. Table demonstrates how the role of IL-22 can differ in the setting of acute vs. chronic inflammation and how it can convert the protective role of IL-22 into a pathological one.
However, in some cases, IL-22 signaling differs from reparative conditions and causes pathological conditions like inflammatory diseases and cancers due to dysregulation or increased levels like in chronic inflammation. All of these above mentioned pathways, that are essential for wound healing, regeneration and protection, become the cause of pathological conditions when persistently expressed (as in chronic inflammatory conditions). Furthermore, the levels of IL-22/IL-22R1 axis also differ in early and late cancers which shows its potential to be used as a staging marker in the future. The influence of IL-22 on hyperplasia, adenoma, early carcinoma, and late carcinoma stages of cancer was investigated using IL-22−/−/MMTV-PyMT spontaneous breast cancer mouse model showing that it is necessary for malignant transformation of cancer cells which is the critical stage for metastasis. Inhibition of IL-22 can hinder cancer cell malignancy (187). Similarly the infiltration of cellular sources of IL-22 including Th22 and CD4+ T cells in the intratumoral tissue increases, corresponding to the stage of tumor (153, 155). Patients with gastric tumors have also been shown to have increased levels of circulating T cells secreting IL-22 as well as IL-17, thus showing a positive association with tumor progression (154). In gastric cancer patients, IL-22R1 expression is augmented that indicates a positive correspondence with the stage of cancer and lymphatic invasion (156). Furthermore, IL-22+ immune cell frequency was also higher in HBV-infected patients with liver cirrhosis than in non-cirrhotic patients in positive correlation with cirrhotic stage score (123). In colon cancer, enhanced IL-22 expression is associated with poor prognosis and an enhanced tumorigenesis, with protective role shown by IL-22 binding protein (188). IL-22 and HOXB-AS5 (a long non-coding RNA located in HOX gene clusters) were upregulated in the serum and tissues of Breast Cancer patients and were associated with clinical stages which showed that the IL-22-HOXB-AS5-PI3K/AKT functional axes may serve as potential molecule biomarkers for diagnosis or therapeutic strategy (189).
Future Directions
Further studies are needed to identify more possible sources of IL-22 and the pathways involved in its signaling. Furthermore, role of epigenetic modifications, transcription factors, non-coding RNAs in IL-22 regulation needs to be further studied. Understanding IL-22 biology for the potential development of vaccines needs to be further researched to utilize IL-22 for vaccine development. The factors and conditions that control the protective and pathological role of IL-22 needs to be studied further. Owing to its therapeutic potential in inflammatory diseases like psoriasis, ulcerative colitis, liver and pancreatic damage, graft-versus-host disease, certain infections and tumors, and its association with disease staging, IL-22 can prove to be useful for therapeutic and prognostic purposes in the future.
Author Contributions
SM and VL conceived the idea, designed the lay out, and critically reviewed the manuscript. SM, TA, FM, and RP reviewed the literature and drafted the manuscript. All authors approved the final draft.
Funding
This work was financially supported from European Regional Development Fund—Project, Application of Modern Technologies in Medicine and Industry (No. CZ.02.1.01/0.0/0.0/17_048/0007280) and Charles University Fund—Project—UNCE/MED/006 Project Centrum of clinical and experimental liver surgery.
Conflict of Interest
The authors declare that the research was conducted in the absence of any commercial or financial relationships that could be construed as a potential conflict of interest.
References
1. Lanfranca MP, Lin Y, Fang J, Zou W, Frankel T. Biological and pathological activities of interleukin-22. J Mol Med. (2016) 94:523–34. doi: 10.1007/s00109-016-1391-6
2. Mühl H, Scheiermann P, Bachmann M, Härdle L, Heinrichs A, Pfeilschifter J. IL-22 in tissue-protective therapy. Br J Pharmacol. (2013) 169:761–71. doi: 10.1111/bph.12196
3. Shabgah AG, Navashenaq JG, Shabgah OG, Mohammadi H, Sahebkar A. Interleukin-22 in human inflammatory diseases and viral infections. Autoimmu Rev. (2017) 16:1209–18. doi: 10.1016/j.autrev.2017.10.004
4. Hernandez P, Gronke K, Diefenbach A. A catch-22: interleukin-22 and cancer. Eur J Immunol. (2018) 48:15–31. doi: 10.1002/eji.201747183
5. Dumoutier L, Louahed J, Renauld JC. Cloning and characterization of IL-10-related T cell-derived inducible factor (IL-TIF), a novel cytokine structurally related to IL-10 and inducible by IL-9. J Immunol. (2000) 164:1814–9. doi: 10.4049/jimmunol.164.4.1814
6. Bleicher L, de Moura PR, Watanabe L, Colau D, Dumoutier L, Renauld JC, et al. Crystal structure of the IL-22/IL-22R1 complex and its implications for the IL-22 signaling mechanism. FEBS letters. (2008) 582:2985–92. doi: 10.1016/j.febslet.2008.07.046
7. Logsdon NJ, Jones BC, Allman JC, Izotova L, Schwartz B, Pestka S, et al. The IL-10R2 binding hot spot on IL-22 is located on the N-terminal helix and is dependent on N-linked glycosylation. J Mol Biol. (2004) 342:503–14. doi: 10.1016/j.jmb.2004.07.069
8. Nagem RA, Colau D, Dumoutier L, Renauld JC, Ogata C, Polikarpov I. Crystal structure of recombinant human interleukin-22. Structure. (2002) 10:1051–62. doi: 10.1016/S0969-2126(02)00797-9
9. Ouyang W, Rutz S, Crellin NK, Valdez PA, Hymowitz SG. Regulation and functions of the IL-10 family of cytokines in inflammation and disease. Ann Rev Immunol. (2011) 29:71–109. doi: 10.1146/annurev-immunol-031210-101312
10. Dumoutier L, Van Roost E, Colau D, Renauld J-C. Human interleukin-10-related T cell-derived inducible factor: molecular cloning and functional characterization as an hepatocyte-stimulating factor. Proc Natl Acad Sci USA. (2000) 97:10144–9. doi: 10.1073/pnas.170291697
11. Nagem RA, Lucchesi KW, Colau D, Dumoutier L, Renauld JC, Polikarpov I. Crystallization and synchrotron X-ray diffraction studies of human interleukin-22. Acta Crystallogr Sec D. (2002) 58:529–30. doi: 10.1107/S0907444902001063
12. Logsdon NJ, Jones BC, Josephson K, Cook J, Walter MR. Comparison of interleukin-22 and interleukin-10 soluble receptor complexes. J Interf Cytokine Res. (2002) 22:1099–112. doi: 10.1089/10799900260442520
13. Xie MH, Aggarwal S, Ho WH, Foster J, Zhang Z, Stinson J, et al. Interleukin (IL)-22, a novel human cytokine that signals through the interferon receptor-related proteins CRF2–4 and IL-22R. J Biol Chem. (2000) 275:31335–9. doi: 10.1074/jbc.M005304200
14. Kotenko SV, Izotova LS, Mirochnitchenko OV, Esterova E, Dickensheets H, Donnelly P, et al. Identification of the functional interleukin-22 (IL-22) receptor complex the Il-10r2 chain (Il-10rβ) is a common chain of both the Il-10 And Il-22 (Il-10-related T cell-derived inducible factor, Il-tif) receptor complexes. J Biol Chem. (2001) 276:2725–32. doi: 10.1074/jbc.M007837200
15. Langer JA, Cutrone EC, Kotenko S. The Class II cytokine receptor (CRF2) family: overview and patterns of receptor–ligand interactions. Cytokine Growth Factor Rev. (2004) 15:33–48. doi: 10.1016/j.cytogfr.2003.10.001
16. Kotenko SV, Langer JA. Full house: 12 receptors for 27 cytokines. Int Immunopharmacol. (2004) 4:593–608. doi: 10.1016/j.intimp.2004.01.003
17. Jones BC, Logsdon NJ, Walter MR. Crystallization and preliminary X-ray diffraction analysis of human IL-22 bound to the extracellular IL-22R1 chain. Acta Crystallogr Sec F. (2008) 64:266–9. doi: 10.1107/S1744309108004752
18. Jones BC, Logsdon NJ, Walter MR. Structure of IL-22 bound to its high-affinity IL-22R1 chain. Structure. (2008) 16:1333–44. doi: 10.1016/j.str.2008.06.005
19. Yoon SI, Jones BC, Logsdon NJ, Harris BD, Deshpande A, Radaeva S, et al. Structure and mechanism of receptor sharing by the IL-10R2 common chain. Structure. (2010) 18:638–48. doi: 10.1016/j.str.2010.02.009
20. Lejeune D, Dumoutier L, Constantinescu S, Kruijer W, Schuringa JJ, Renauld J-C. Interleukin-22 (IL-22) activates the JAK/STAT, ERK, JNK, and p38 MAP kinase pathways in a rat hepatoma cell line pathways that are shared with and distinct from IL-10. J Biol Chem. (2002) 277:33676–82. doi: 10.1074/jbc.M204204200
21. Mitra A, Raychaudhuri SK, Raychaudhuri SP. IL-22 induced cell proliferation is regulated by PI3K/Akt/mTOR signaling cascade. Cytokine. (2012) 60:38–42. doi: 10.1016/j.cyto.2012.06.316
22. Dumoutier L, Lejeune D, Colau D, Renauld JC. Cloning and characterization of IL-22 binding protein, a natural antagonist of IL-10-related T cell-derived inducible factor/IL-22. J Immunol. (2001) 166:7090–5. doi: 10.4049/jimmunol.166.12.7090
23. Kotenko SV, Izotova LS, Mirochnitchenko OV, Esterova E, Dickensheets H, Donnelly RP, et al. Identification, cloning, and characterization of a novel soluble receptor that binds IL-22 and neutralizes its activity. J Immunol. (2001) 166:7096–103. doi: 10.4049/jimmunol.166.12.7096
24. de Moura PR, Watanabe L, Bleicher L, Colau D, Dumoutier L, Lemaire MM, et al. Crystal structure of a soluble decoy receptor IL-22BP bound to interleukin-22. FEBS Lett. (2009) 583:1072–7. doi: 10.1016/j.febslet.2009.03.006
25. Martin JC, Bériou G, Heslan M, Bossard C, Jarry A, Abidi A, et al. IL-22BP is produced by eosinophils in human gut and blocks IL-22 protective actions during colitis. Mucosal Immunol. (2016) 9:539–49. doi: 10.1038/mi.2015.83
26. Huber S, Gagliani N, Zenewicz LA, Huber FJ, Bosurgi L, Hu B, et al. IL-22BP is regulated by the inflammasome and modulates tumorigenesis in the intestine. Nature. (2012) 491:259–63. doi: 10.1038/nature11535
27. Luckheeram RV, Zhou R, Verma AD, Xia B. CD4+ T cells: differentiation and functions. Clin Dev Immunol. (2012) 2012:925135. doi: 10.1155/2012/925135
28. Wolk K, Kunz S, Asadullah K, Sabat R. Cutting edge: immune cells as sources and targets of the IL-10 family members? J Immunol. (2002) 168:5397–402. doi: 10.4049/jimmunol.168.11.5397
29. Liang SC, Tan X-Y, Luxenberg DP, Karim R, Dunussi-Joannopoulos K, Collins M, et al. Interleukin (IL)-22 and IL-17 are coexpressed by Th17 cells and cooperatively enhance expression of antimicrobial peptides. J Exp Med. (2006) 203:2271–9. doi: 10.1084/jem.20061308
30. Chung Y, Yang X, Chang SH, Ma L, Tian Q, Dong C. Expression and regulation of IL-22 in the IL-17-producing CD4+ T lymphocytes. Cell Res. (2006) 16:902–7. doi: 10.1038/sj.cr.7310106
31. Abikhair M, Mitsui H, Yanofsky V, Roudiani N, Ovits C, Bryan T, et al. Cyclosporine A immunosuppression drives catastrophic squamous cell carcinoma through IL-22. JCI Insight. (2016) 1:e86434. doi: 10.1172/jci.insight.86434
32. Basu R, O'Quinn DB, Silberger DJ, Schoeb TR, Fouser L, Ouyang W, et al. Th22 cells are an important source of IL-22 for host protection against enteropathogenic bacteria. Immunity. (2012) 37:1061–75. doi: 10.1016/j.immuni.2012.08.024
33. Doulabi H, Rastin M, Shabahangh H, Maddah G, Abdollahi A, Nosratabadi R, et al. Analysis of Th22, Th17 and CD4+ cells co-producing IL-17/IL-22 at different stages of human colon cancer. Biomed Pharmacother. (2018) 103:1101–6. doi: 10.1016/j.biopha.2018.04.147
34. Duhen T, Geiger R, Jarrossay D, Lanzavecchia A, Sallusto F. Production of interleukin 22 but not interleukin 17 by a subset of human skin-homing memory T cells. Nature Immunol. (2009) 10:857–63. doi: 10.1038/ni.1767
35. Eyerich S, Eyerich K, Pennino D, Carbone T, Nasorri F, Pallotta S, et al. Th22 cells represent a distinct human T cell subset involved in epidermal immunity and remodeling. J Clin Investig. (2009) 119:3573–85. doi: 10.1172/JCI40202
36. Yang XY, Wang HY, Zhao XY, Wang LJ, Lv QH, Wang QQ. Th22, but not Th17 might be a good index to predict the tissue involvement of systemic lupus erythematosus. J Clin Immunol. (2013) 33:767–74. doi: 10.1007/s10875-013-9878-1
37. Alam MS, Maekawa Y, Kitamura A, Tanigaki K, Yoshimoto T, Kishihara K, et al. Notch signaling drives IL-22 secretion in CD4+ T cells by stimulating the aryl hydrocarbon receptor. Proc Natl Acad Sci USA. (2010) 107:5943–8. doi: 10.1073/pnas.0911755107
38. Esser C, Rannug A, Stockinger B. The aryl hydrocarbon receptor in immunity. Trends Immunol. (2009) 30:447–54. doi: 10.1016/j.it.2009.06.005
39. Budda SA, Girton A, Henderson JG, Zenewicz LA. Transcription factor HIF-1α controls expression of the cytokine IL-22 in CD4 T cells. J Immunol. (2016) 197:2646–52. doi: 10.4049/jimmunol.1600250
40. Witte E, Witte K, Warszawska K, Sabat R, Wolk K. Interleukin-22: a cytokine produced by T, NK and NKT cell subsets, with importance in the innate immune defense and tissue protection. Cytokine Growth Factor Rev. (2010) 21:365–79. doi: 10.1016/j.cytogfr.2010.08.002
41. Satoh-Takayama N, Vosshenrich CA, Lesjean-Pottier S, Sawa S, Lochner M, Rattis F, et al. Microbial flora drives interleukin 22 production in intestinal NKp46+ cells that provide innate mucosal immune defense. Immunity. (2008) 29:958–70. doi: 10.1016/j.immuni.2008.11.001
42. Lee JS, Cella M, McDonald KG, Garlanda C, Kennedy GD, Nukaya M, et al. AHR drives the development of gut ILC22 cells and postnatal lymphoid tissues via pathways dependent on and independent of Notch. Nat Immunol. (2012) 13:144–51. doi: 10.1038/ni.2187
43. Qiu J, Heller JJ, Guo X, Zong-ming EC, Fish K, Fu YX, et al. The aryl hydrocarbon receptor regulates gut immunity through modulation of innate lymphoid cells. Immunity. (2012) 36:92–104. doi: 10.1016/j.immuni.2011.11.011
44. Wolk K, Kunz S, Witte E, Friedrich M, Asadullah K, Sabat R. IL-22 increases the innate immunity of tissues. Immunity. (2004) 21:241–54. doi: 10.1016/j.immuni.2004.07.007
45. Whittington HA, Armstrong L, Uppington KM, Millar AB. Interleukin-22: a potential immunomodulatory molecule in the lung. Am J Respir Cell Mol Biol. (2004) 31:220–6. doi: 10.1165/rcmb.2003-0285OC
46. Sugimoto K, Ogawa A, Mizoguchi E, Shimomura Y, Andoh A, Bhan AK, et al. IL-22 ameliorates intestinal inflammation in a mouse model of ulcerative colitis. J Clin Investig. (2008) 118:534–44. doi: 10.1172/JCI33194
47. Wolk K, Haugen HS, Xu W, Witte E, Waggie K, Anderson M, et al. IL-22 and IL-20 are key mediators of the epidermal alterations in psoriasis while IL-17 and IFN-γ are not. J Mol Med. (2009) 87:523–36. doi: 10.1007/s00109-009-0457-0
48. Dudakov JA, Hanash AM, Jenq RR, Young LF, Ghosh A, Singer NV, et al. Interleukin-22 drives endogenous thymic regeneration in mice. Science. (2012) 336:91–5. doi: 10.1126/science.1218004
49. Aggarwal S, Xie MH, Maruoka M, Foster J, Gurney AL. Acinar cells of the pancreas are a target of interleukin-22. J Interferon Cytokine Res. (2001) 21:1047–53. doi: 10.1089/107999001317205178
50. Shioya M, Andoh A, Kakinoki S, Nishida A, Fujiyama Y. Interleukin 22 receptor 1 expression in pancreas islets. Pancreas. (2008) 36:197–9. doi: 10.1097/MPA.0b013e3181594258
51. Kulkarni OP, Hartter I, Mulay SR, Hagemann J, Darisipudi MN, VR SK, et al. Toll-like receptor 4–induced IL-22 accelerates kidney regeneration. J Am Soc Nephrol. (2014) 25:978–89. doi: 10.1681/ASN.2013050528
52. Zhang W, Dang E, Shi X, Jin L, Feng Z, Hu L, et al. The pro-inflammatory cytokine IL-22 up-regulates keratin 17 expression in keratinocytes via STAT3 and ERK1/2. PLoS ONE. (2012) 7:e40797. doi: 10.1371/journal.pone.0040797
53. Leung JM, Davenport M, Wolff MJ, Wiens KE, Abidi WM, Poles MA, et al. IL-22-producing CD4+ cells are depleted in actively inflamed colitis tissue. Mucosal Immunol. (2014) 7:124–33. doi: 10.1038/mi.2013.31
54. Murano T, Okamoto R, Ito G, Nakata T, Hibiya S, Shimizu H, et al. Hes1 promotes the IL-22-mediated antimicrobial response by enhancing STAT3-dependent transcription in human intestinal epithelial cells. Biochem Biophys Res Commun. (2014) 443:840–6. doi: 10.1016/j.bbrc.2013.12.061
55. Nagalakshmi ML, Rascle A, Zurawski S, Menon S, de Waal Malefyt R. Interleukin-22 activates STAT3 and induces IL-10 by colon epithelial cells. Int Immunopharmacol. (2004) 4:679–91. doi: 10.1016/j.intimp.2004.01.008
56. Sa SM, Valdez PA, Wu J, Jung K, Zhong F, Hall L, et al. The effects of IL-20 subfamily cytokines on reconstituted human epidermis suggest potential roles in cutaneous innate defense and pathogenic adaptive immunity in psoriasis. J Immunol. (2007) 178:2229–40. doi: 10.4049/jimmunol.178.4.2229
57. Kong X, Feng D, Wang H, Hong F, Bertola A, Wang FS, et al. Interleukin-22 induces hepatic stellate cell senescence and restricts liver fibrosis in mice. Hepatology. (2012) 56:1150–9. doi: 10.1002/hep.25744
58. Andoh A, Zhang Z, Inatomi O, Fujino S, Deguchi Y, Araki Y, et al. Interleukin-22, a member of the IL-10 subfamily, induces inflammatory responses in colonic subepithelial myofibroblasts. Gastroenterology. (2005) 129:969–84. doi: 10.1053/j.gastro.2005.06.071
59. Cho K-A, Suh JW, Lee KH, Kang JL, Woo S-Y. IL-17 and IL-22 enhance skin inflammation by stimulating the secretion of IL-1β by keratinocytes via the ROS-NLRP3-caspase-1 pathway. Int Immunol. (2011) 24:147–58. doi: 10.1093/intimm/dxr110
60. Bansal G, Das D, Hsieh C-Y, Wang Y-H, Gilmore BA, Wong C-M, et al. IL-22 activates oxidant signaling in pulmonary vascular smooth muscle cells. Cell Signal. (2013) 25:2727–33. doi: 10.1016/j.cellsig.2013.09.001
61. Guilloteau K, Paris I, Pedretti N, Boniface K, Juchaux F, Huguier V, et al. Skin inflammation induced by the synergistic action of IL-17A, IL-22, oncostatin M, IL-1α, and TNF-α recapitulates some features of psoriasis. J Immunol. (2010) 184:5263–70. doi: 10.4049/jimmunol.0902464
62. Nograles KE, Zaba LC, Shemer A, Fuentes-Duculan J, Cardinale I, Kikuchi T, et al. IL-22–producing “T22” T cells account for upregulated IL-22 in atopic dermatitis despite reduced IL-17–producing TH17 T cells. J Allergy Clin Immunol. (2009) 123:1244–52.e2. doi: 10.1016/j.jaci.2009.03.041
63. Leyva-Castillo JM, Yoon J, Geha RS. IL-22 promotes allergic airway inflammation in epicutaneously sensitized mice. J Allergy Clin Immunol. (2019) 143:619–30. e7. doi: 10.1016/j.jaci.2018.05.032
64. Fukaya T, Fukui T, Uto T, Takagi H, Nasu J, Miyanaga N, et al. Pivotal role of IL-22 binding protein in the epithelial autoregulation of interleukin-22 signaling in the control of skin inflammation. Front Immunol. (2018) 9:1418. doi: 10.3389/fimmu.2018.01418
65. Ito T, Hirose K, Saku A, Kono K, Takatori H, Tamachi T, et al. IL-22 induces Reg3γ and inhibits allergic inflammation in house dust mite–induced asthma models. J Exp Med. (2017) 214:3037–50. doi: 10.1084/jem.20162108
66. Hsueh Y-H, Chang Y-N, Loh C-E, Gershwin ME, Chuang Y-H. AAV-IL-22 modifies liver chemokine activity and ameliorates portal inflammation in murine autoimmune cholangitis. J Autoimmun. (2016) 66:89–97. doi: 10.1016/j.jaut.2015.10.005
67. Zenewicz LA, Yancopoulos GD, Valenzuela DM, Murphy AJ, Karow M, Flavell RA. Interleukin-22 but not interleukin-17 provides protection to hepatocytes during acute liver inflammation. Immunity. (2007) 27:647–59. doi: 10.1016/j.immuni.2007.07.023
68. Zenewicz LA, Yancopoulos GD, Valenzuela DM, Murphy AJ, Stevens S, Flavell RA. Innate and adaptive interleukin-22 protects mice from inflammatory bowel disease. Immunity. (2008) 29:947–57. doi: 10.1016/j.immuni.2008.11.003
69. Wolk K, Warszawska K, Hoeflich C, Witte E, Schneider-Burrus S, Witte K, et al. Deficiency of IL-22 contributes to a chronic inflammatory disease: pathogenetic mechanisms in acne inversa. J Immunol. (2011) 186:1228–39. doi: 10.4049/jimmunol.0903907
70. Alabbas SY, Begun J, Florin TH, Oancea I. The role of IL-22 in the resolution of sterile and nonsterile inflammation. Clin Transl Immunol. (2018) 7:e1017. doi: 10.1002/cti2.1017
71. Gallo RL, Hooper LV. Epithelial antimicrobial defence of the skin and intestine. Nature Rev Immunol. (2012) 12:503. doi: 10.1038/nri3228
72. Aujla SJ, Chan YR, Zheng M, Fei M, Askew DJ, Pociask DA, et al. IL-22 mediates mucosal host defense against Gram-negative bacterial pneumonia. Nat Med. (2008) 14:275. doi: 10.1038/nm1710
73. Kim C, Nazli A, Rojas O, Chege D, Alidina Z, Huibner S, et al. A role for mucosal IL-22 production and Th22 cells in HIV-associated mucosal immunopathogenesis. Mucosal Immunol. (2012) 5:670. doi: 10.1038/mi.2012.72
74. Thibodeau V, Fourcade L, Labbé A-C, Alary M, Guédou F, Poudrier J, et al. Highly-exposed HIV-1 seronegative female commercial sex workers sustain in their genital mucosa increased frequencies of tolerogenic myeloid and regulatory T-cells. Sci Rep. (2017) 7:43857. doi: 10.1038/srep43857
75. Budda SA, Zenewicz LA. IL-22 deficiency increases CD4 T cell responses to mucosal immunization. Vaccine. (2018) 36:3694–700. doi: 10.1016/j.vaccine.2018.05.011
76. Ngo VL, Abo H, Maxim E, Harusato A, Geem D, Medina-Contreras O, et al. A cytokine network involving IL-36γ, IL-23, and IL-22 promotes antimicrobial defense and recovery from intestinal barrier damage. Proc Natl Acad SciUSA. (2018) 115:E5076–85. doi: 10.1073/pnas.1718902115
77. Wolk K, Witte E, Wallace E, Döcke WD, Kunz S, Asadullah K, et al. IL-22 regulates the expression of genes responsible for antimicrobial defense, cellular differentiation, and mobility in keratinocytes: a potential role in psoriasis. Eur J Immunol. (2006) 36:1309–23. doi: 10.1002/eji.200535503
78. Pickert G, Neufert C, Leppkes M, Zheng Y, Wittkopf N, Warntjen M, et al. STAT3 links IL-22 signaling in intestinal epithelial cells to mucosal wound healing. J Exp Med. (2009) 206:1465–72. doi: 10.1084/jem.20082683
79. Vaishnava S, Yamamoto M, Severson KM, Ruhn KA, Yu X, Koren O, et al. The antibacterial lectin RegIIIγ promotes the spatial segregation of microbiota and host in the intestine. Science. (2011) 334:255–8. doi: 10.1126/science.1209791
80. Brandl K, Plitas G, Schnabl B, DeMatteo RP, Pamer EG. MyD88-mediated signals induce the bactericidal lectin RegIIIγ and protect mice against intestinal Listeria monocytogenes infection. J Exp Med. (2007) 204:1891–900. doi: 10.1084/jem.20070563
81. Li Y, Wang J, Li Y, Wu H, Zhao S, Yu Q. Protecting intestinal epithelial cells against deoxynivalenol and E. coli damage by recombinant porcine IL-22. Vet Microbiol. (2019) 231:154–9. doi: 10.1016/j.vetmic.2019.02.027
82. Sonnenberg GF, Monticelli LA, Alenghat T, Fung TC, Hutnick NA, Kunisawa J, et al. Innate lymphoid cells promote anatomical containment of lymphoid-resident commensal bacteria. Science. (2012) 336:1321–5. doi: 10.1126/science.1222551
83. Van Maele L, Carnoy C, Cayet D, Ivanov S, Porte R, Deruy E, et al. Activation of Type 3 innate lymphoid cells and interleukin 22 secretion in the lungs during Streptococcus pneumoniae infection. J Infect Dis. (2014) 210:493–503. doi: 10.1093/infdis/jiu106
84. Trevejo-Nunez G, Chen K, Kolls JK. C34 mechanisms of host defense in bacterial and fungal infections: role of the Il-22 binding protein during acute and chronic lung infection. Am Jo Respi Crit Care Med. (2017) 195:A5263. doi: 10.1164/ajrccm-conference.2017.C34
85. Dhiman R, Indramohan M, Barnes PF, Nayak RC, Paidipally P, Rao LV, et al. IL-22 produced by human NK cells inhibits growth of Mycobacterium tuberculosis by enhancing phagolysosomal fusion. J Immunol. (2009) 183:6639–45. doi: 10.4049/jimmunol.0902587
86. Treerat P, Prince O, Cruz-Lagunas A, Muñoz-Torrico M, Salazar-Lezama M, Selman M, et al. Novel role for IL-22 in protection during chronic Mycobacterium tuberculosis HN878 infection. Mucosal Immunol. (2017) 10:1069. doi: 10.1038/mi.2017.15
87. Dhiman R, Venkatasubramanian S, Paidipally P, Barnes PF, Tvinnereim A, Vankayalapati R. Interleukin 22 inhibits intracellular growth of Mycobacterium tuberculosis by enhancing calgranulin A expression. J Infect Dis. (2014) 209:578–87. doi: 10.1093/infdis/jit495
88. Schulz SM, Köhler G, Schütze N, Knauer J, Straubinger RK, Chackerian AA, et al. Protective immunity to systemic infection with attenuated Salmonella enterica serovar enteritidis in the absence of IL-12 is associated with IL-23-dependent IL-22, but not IL-17. J Immunol. (2008) 181:7891–901. doi: 10.4049/jimmunol.181.11.7891
89. Forbester JL, Lees EA, Goulding D, Forrest S, Yeung A, Speak A, et al. Interleukin-22 promotes phagolysosomal fusion to induce protection against Salmonella enterica Typhimurium in human epithelial cells. Proc Natl Acad Sci USA. (2018) 115:10118–23. doi: 10.1073/pnas.1811866115
90. Siegemund S, Schütze N, Schulz S, Wolk K, Nasilowska K, Straubinger RK, et al. Differential IL-23 requirement for IL-22 and IL-17A production during innate immunity against Salmonella enterica serovar Enteritidis. Int Immunol. (2009) 21:555–65. doi: 10.1093/intimm/dxp025
91. Wilson MS, Feng CG, Barber DL, Yarovinsky F, Cheever AW, Sher A, et al. Redundant and pathogenic roles for IL-22 in mycobacterial, protozoan, and helminth infections. J Immunol. (2010) 184:4378–90. doi: 10.4049/jimmunol.0903416
92. Graham AC, Carr KD, Sieve AN, Indramohan M, Break TJ, Berg RE. IL-22 production is regulated by IL-23 during Listeria monocytogenes infection but is not required for bacterial clearance or tissue protection. PLoS ONE. (2011) 6:e17171. doi: 10.1371/journal.pone.0017171
93. Weber GF, Schlautkötter S, Kaiser-Moore S, Altmayr F, Holzmann B, Weighardt H. Inhibition of interleukin-22 attenuates bacterial load and organ failure during acute polymicrobial sepsis. Infect Immun. (2007) 75:1690–7. doi: 10.1128/IAI.01564-06
94. Gessner MA, Werner JL, Lilly LM, Nelson MP, Metz AE, Dunaway CW, et al. Dectin-1 dependent IL-22 contributes to early innate lung defense against Aspergillus fumigatus. Infect Immun. (2011) 80:410–7. doi: 10.1128/IAI.05939-11
95. Reeder KM, Mackel JJ, Godwin MS, Dunaway CW, Blackburn JP, Patel RP, et al. Role of common γ-chain cytokines in lung interleukin-22 regulation after acute exposure to Aspergillus fumigatus. Infect Immunity. (2018) 86:e00157–18. doi: 10.1128/IAI.00157-18
96. Liu Y, Yang B, Zhou M, Li L, Zhou H, Zhang J, et al. Memory IL-22-producing CD4+ T cells specific for Candida albicans are present in humans. Eur J Immunol. (2009) 39:1472–9. doi: 10.1002/eji.200838811
97. De Luca A, Zelante T, D'angelo C, Zagarella S, Fallarino F, Spreca A, et al. IL-22 defines a novel immune pathway of antifungal resistance. Mucosal Immunol. (2010) 3:361–73. doi: 10.1038/mi.2010.22
98. Kagami S, Rizzo HL, Kurtz SE, Miller LS, Blauvelt A. IL-23 and IL-17A, but not IL-12 and IL-22, are required for optimal skin host defense against Candida albicans. J Immunol. (2010) 185:5453–62. doi: 10.4049/jimmunol.1001153
99. Conti HR, Shen F, Nayyar N, Stocum E, Sun JN, Lindemann MJ, et al. Th17 cells and IL-17 receptor signaling are essential for mucosal host defense against oral candidiasis. J Expe Med. (2009) 206:299–311. doi: 10.1084/jem.20081463
100. Reeder KM, Dunaway CW, Blackburn JP, Yu Z, Matalon S, Hastie AT, et al. The common γ-chain cytokine IL-7 promotes immunopathogenesis during fungal asthma. Mucosal Immunol. (2018) 11:1352–62. doi: 10.1038/s41385-018-0028-1
101. Lilly LM, Gessner MA, Dunaway CW, Metz AE, Schwiebert L, Weaver CT, et al. The β-glucan receptor dectin-1 promotes lung immunopathology during fungal allergy via IL-22. J Immunol. (2012) 189:3653–60. doi: 10.4049/jimmunol.1201797
102. Yi P, Liang Y, Yuan DM, Jie Z, Kwota Z, Chen Y, et al. A tightly regulated IL-22 response maintains immune functions and homeostasis in systemic viral infection. Sci Rep. (2017) 7:3857. doi: 10.1038/s41598-017-04260-0
103. Paget C, Ivanov S, Fontaine J, Renneson J, Blanc F, Pichavant M, et al. Interleukin-22 is produced by invariant natural killer T lymphocytes during influenza a virus infection potential role in protection against lung epithelial damages. J Biol Chem. (2012) 287:8816–29. doi: 10.1074/jbc.M111.304758
104. Kumar P, Thakar MS, Ouyang W, Malarkannan S. IL-22 from conventional NK cells is epithelial regenerative and inflammation protective during influenza infection. Mucosal Immunol. (2013) 6:69–82. doi: 10.1038/mi.2012.49
105. Pociask DA, Scheller EV, Mandalapu S, McHugh KJ, Enelow RI, Fattman CL, et al. IL-22 is essential for lung epithelial repair following influenza infection. Am J Pathol. (2013) 182:1286–96. doi: 10.1016/j.ajpath.2012.12.007
106. Ivanov S, Renneson J, Fontaine J, Barthelemy A, Paget C, Fernandez EM, et al. Interleukin-22 reduces lung inflammation during influenza A virus infection and protects against secondary bacterial infection. J Virol. (2013) 87:6911–24. doi: 10.1128/JVI.02943-12
107. Barthelemy A, Sencio V, Soulard D, Deruyter L, Faveeuw C, Le Goffic R, et al. Interleukin-22 immunotherapy during severe influenza enhances lung tissue integrity and reduces secondary bacterial systemic invasion. Infect Immun. (2018) 86:e00706-17. doi: 10.1128/IAI.00706-17
108. Klatt NR, Estes JD, Sun X, Ortiz AM, Barber JS, Harris LD, et al. Loss of mucosal CD103+ DCs and IL-17+ and IL-22+ lymphocytes is associated with mucosal damage in SIV infection. Mucosal Immunol. (2012) 5:646–57. doi: 10.1038/mi.2012.38
109. Chebli K, Papon L, Paul C, Garcel A, Campos N, Scherrer D, et al. The anti-HIV candidate ABX464 dampens intestinal inflammation by triggering IL-22 production in activated macrophages. Sci Rep. (2017) 7:4860. doi: 10.1038/s41598-017-04071-3
110. Zhang B, Chassaing B, Shi Z, Uchiyama R, Zhang Z, Denning TL, et al. Prevention and cure of rotavirus infection via TLR5/NLRC4–mediated production of IL-22 and IL-18. Science. (2014) 346:861–5. doi: 10.1126/science.1256999
111. Hernández PP, Mahlakõiv T, Yang I, Schwierzeck V, Nguyen N, Guendel F, et al. Interferon-λ and interleukin 22 act synergistically for the induction of interferon-stimulated genes and control of rotavirus infection. Nat Immunol. (2015) 16:698–707. doi: 10.1038/ni.3180
112. Su L, Liao Q, Wu Y, Chen X. Kaposi's sarcoma-associated herpesvirus-encoded LANA down-regulates IL-22R1 expression through a cis-acting element within the promoter region. PLoS ONE. (2011) 6:e19106. doi: 10.1371/journal.pone.0019106
113. Wang P, Bai F, Zenewicz LA, Dai J, Gate D, Cheng G, et al. IL-22 signaling contributes to West Nile encephalitis pathogenesis. PLoS ONE. (2012) 7:e44153. doi: 10.1371/journal.pone.0044153
114. Yi P, Liang Y, Chen J, Hood B, Azar S, Rossi S, et al. IL-22 signaling contributes to modulate the pathogenesis of Zika virus infection. J Immunol. (2017) 198(Suppl. 1):158.8. Available online at: https://www.jimmunol.org/content/198/1_Supplement/158.8.abstract
115. Xiang X, Gui H, King NJ, Cole L, Wang H, Xie Q, et al. IL-22 and non-ELR-CXC chemokine expression in chronic hepatitis B virus-infected liver. Immunol Cell Biol. (2012) 90:611–9. doi: 10.1038/icb.2011.79
116. Foster RG, Golden-Mason L, Rutebemberwa A, Rosen HR. Interleukin (IL)-17/IL-22-producing T cells enriched within the liver of patients with chronic hepatitis C viral (HCV) infection. Digest Dis Sci. (2012) 57:381–9. doi: 10.1007/s10620-011-1997-z
117. Yi P, Liang Y, Yuan D, Jie Z, Kwota Z, Cong Y, et al. PI3K/mTOR-dependent IL-22 production modulates polyfunctional T cell responses in viral hepatitis. J Immunol. (2016) 196 (Suppl. 1):196.12. Available online at: https://www.jimmunol.org/content/196/1_Supplement/196.12.short
118. Cobleigh MA, Robek MD. Protective and pathological properties of IL-22 in liver disease: implications for viral hepatitis. Am J Pathol. (2013) 182:21–8. doi: 10.1016/j.ajpath.2012.08.043
119. Feng D, Kong X, Weng H, Park O, Wang H, Dooley S, et al. Interleukin-22 promotes proliferation of liver stem/progenitor cells in mice and patients with chronic hepatitis B virus infection. Gastroenterology. (2012) 143:188–98. doi: 10.1053/j.gastro.2012.03.044
120. Zhang Y, Cobleigh MA, Lian JQ, Huang CX, Booth CJ, Bai XF, et al. A proinflammatory role for interleukin-22 in the immune response to hepatitis B virus. Gastroenterology. (2011) 141:1897–906. doi: 10.1053/j.gastro.2011.06.051
121. Jie Z, Liang Y, Yi P, Tang H, Soong L, Cong Y, et al. Retinoic acid regulates immune responses by promoting IL-22 and modulating S100 proteins in viral hepatitis. J Immunol. (2017) 198:3448–60. doi: 10.4049/jimmunol.1601891
122. Mo R, Wang P, Lai R, Li F, Liu Y, Jiang S, et al. Persistently elevated circulating Th22 reversely correlates with prognosis in HBV-related acute-on-chronic liver failure. J Gastroenterol Hepatol. (2017) 32:677–86. doi: 10.1111/jgh.13537
123. Zhao J, Zhang Z, Luan Y, Zou Z, Sun Y, Li Y, et al. Pathological functions of interleukin-22 in chronic liver inflammation and fibrosis with hepatitis B virus infection by promoting T helper 17 cell recruitment. Hepatology. (2014) 59:1331–42. doi: 10.1002/hep.26916
124. Park O, Wang H, Weng H, Feigenbaum L, Li H, Yin S, et al. In vivo consequences of liver-specific interleukin-22 expression in mice: implications for human liver disease progression. Hepatology. (2011) 54:252–61. doi: 10.1002/hep.24339
125. Dambacher J, Beigel F, Zitzmann K, Heeg MH, Göke B, Diepolder HM, et al. The role of interleukin-22 in hepatitis C virus infection. Cytokine. (2008) 41:209–16. doi: 10.1016/j.cyto.2007.11.016
126. Hennig BJ, Frodsham AJ, Hellier S, Knapp S, Yee LJ, Wright M, et al. Influence of IL-10RA and IL-22 polymorphisms on outcome of hepatitis C virus infection. Liver Inter. (2007) 27:1134–43. doi: 10.1111/j.1478-3231.2007.01518.x
127. Avitabile S, Odorisio T, Madonna S, Eyerich S, Guerra L, Eyerich K, et al. Interleukin-22 promotes wound repair in diabetes by improving keratinocyte pro-healing functions. J Investig Dermatol. (2015) 135:2862–70. doi: 10.1038/jid.2015.278
128. McGee HM, Schmidt BA, Booth CJ, Yancopoulos GD, Valenzuela DM, Murphy AJ, et al. IL-22 promotes fibroblast-mediated wound repair in the skin. J Investig Dermatol. (2013) 133:1321–9. doi: 10.1038/jid.2012.463
129. Boniface K, Bernard FX, Garcia M, Gurney AL, Lecron JC, Morel F. IL-22 inhibits epidermal differentiation and induces proinflammatory gene expression and migration of human keratinocytes. J Immunol. (2005) 174:3695–702. doi: 10.4049/jimmunol.174.6.3695
130. Zheng Y, Danilenko DM, Valdez P, Kasman I, Eastham-Anderson J, Wu J, et al. Interleukin-22, a TH 17 cytokine, mediates IL-23-induced dermal inflammation and acanthosis. Nature. (2007) 445:648–51. doi: 10.1038/nature05505
131. Brockmann L, Giannou AD, Gagliani N, Huber S. Regulation of TH17 cells and associated cytokines in wound healing, tissue regeneration, and carcinogenesis. Int J Mol Sci. (2017) 18:1033. doi: 10.3390/ijms18051033
132. Zenewicz LA, Yin X, Wang G, Elinav E, Hao L, Zhao L, et al. IL-22 deficiency alters colonic microbiota to be transmissible and colitogenic. J Immunol. (2013) 190:5306–12. doi: 10.4049/jimmunol.1300016
133. Zhou H, Xie G, Mao Y, Zhou K, Ren R, Zhao Q, et al. Enhanced regeneration and hepatoprotective effects of interleukin 22 fusion protein on a predamaged liver undergoing partial hepatectomy. J Immunol Res. (2018) 2018:5241526. doi: 10.1155/2018/5241526
134. Brand S, Dambacher J, Beigel F, Zitzmann K, Heeg MH, Weiss TS, et al. IL-22-mediated liver cell regeneration is abrogated by SOCS-1/3 overexpression in vitro. Am J Physiol Gastrointest Liver Physiol. (2007) 292:G1019–28. doi: 10.1152/ajpgi.00239.2006
135. Ren X, Hu B, Colletti LM. IL-22 is involved in liver regeneration after hepatectomy. Am J Physiol Gastrointest Liver Physiol. (2010) 298:G74–80. doi: 10.1152/ajpgi.00075.2009
136. Lindemans CA, Calafiore M, Mertelsmann AM, O'connor MH, Dudakov JA, Jenq RR, et al. Interleukin-22 promotes intestinal-stem-cell-mediated epithelial regeneration. Nature. (2015) 528:560–4. doi: 10.1038/nature16460
137. Lindemans CA, Calafiore M, O'Connor M, Mertelsmann A, van den Brink MR, Hanash AM. Interleukin-22 treatment promotes intestinal stem cell-mediated epithelial regeneration via Stat3 activation and reduces GI Gvhd. Biol Blood Marrow Transpl. (2016) 22:S56–7. doi: 10.1016/j.bbmt.2015.11.345
138. Pan B, Zhang F, Lu Z, Li L, Shang L, Xia F, et al. Donor T-cell-derived interleukin-22 promotes thymus regeneration and alleviates chronic graft-versus-host disease in murine allogeneic hematopoietic cell transplant. Int Immunopharmacol. (2019) 67:194–201. doi: 10.1016/j.intimp.2018.12.023
139. Gnirck AC, Wunderlich M, Becker M, Xiong T, Weinert E, Meyer-Schwesinger C, et al. Endogenous IL-22 is dispensable for experimental glomerulonephritis. Am J Physiol Renal Physiol. (2019) 316:F712–22. doi: 10.1152/ajprenal.00303.2018
140. Hill T, Krougly O, Nikoopour E, Bellemore S, Lee-Chan E, Fouser LA, et al. The involvement of interleukin-22 in the expression of pancreatic beta cell regenerative Reg genes. Cell Regener. (2013) 2:2. doi: 10.1186/2045-9769-2-2
141. Kolumam G, Wu X, Lee WP, Hackney JA, Zavala-Solorio J, Gandham V, et al. IL-22R ligands IL-20, IL-22, and IL-24 promote wound healing in diabetic db/db mice. PLoS ONE. (2017) 12:e0170639. doi: 10.1371/journal.pone.0170639
142. Ciccia F, Accardo-Palumbo A, Alessandro R, Rizzo A, Principe S, Peralta S, et al. Interleukin-22 and interleukin-22–producing NKp44+ natural killer cells in subclinical gut inflammation in ankylosing spondylitis. Arthr Rheum. (2012) 64:1869–78. doi: 10.1002/art.34355
143. Zenewicz LA, Flavell RA. Recent advances in IL-22 biology. Int Immunol. (2011) 23:159–63. doi: 10.1093/intimm/dxr001
144. Tiveron LR, da Silva IR, da Silva MV, Peixoto AB, Rodrigues DB, Rodrigues Jr V. High in situ mRNA levels of IL-22, TFG-β, and ARG-1 in keloid scars. Immunobiology. (2018) 223:812–7. doi: 10.1016/j.imbio.2018.08.010
145. Kryczek I, Lin Y, Nagarsheth N, Peng D, Zhao L, Zhao E, et al. IL-22+ CD4+ T cells promote colorectal cancer stemness via STAT3 transcription factor activation and induction of the methyltransferase DOT1L. Immunity. (2014) 40:772–84. doi: 10.1016/j.immuni.2014.03.010
146. Mizoguchi A, Yano A, Himuro H, Ezaki Y, Sadanaga T, Mizoguchi E. Clinical importance of IL-22 cascade in IBD. J Gastroenterol. (2018) 53:465–74. doi: 10.1007/s00535-017-1401-7
147. Yuksel ES, Topal F, Gür Ö, Topal F, Karahanlý C, Uran B. IL-17 and IL-22 in colitis associated colorectal cancer in mice. Medical Science. 23:375–380.
148. Atreya I, Kindermann M, Wirtz S. Innate lymphoid cells in intestinal cancer development. Semin Immunol. (2019) 41:101267. doi: 10.1016/j.smim.2019.02.001
149. Kirchberger S, Royston DJ, Boulard O, Thornton E, Franchini F, Szabady RL, et al. Innate lymphoid cells sustain colon cancer through production of interleukin-22 in a mouse model. J Exp Med. (2013) 210:917–31. doi: 10.1084/jem.20122308
150. Wu T, Cui L, Liang Z, Liu C, Liu Y, Li J. Elevated serum IL-22 levels correlate with chemoresistant condition of colorectal cancer. Clin Immunol. (2013) 147:38. doi: 10.1016/j.clim.2013.02.007
151. Fukui H, Sekikawa A, Tanaka H, Fujimori Y, Katake Y, Fujii S, et al. DMBT1 is a novel gene induced by IL-22 in ulcerative colitis. Inflammatory Bowel Dis. (2011) 17:1177–88. doi: 10.1002/ibd.21473
152. Sun D, Lin Y, Hong J, Chen H, Nagarsheth N, Peng D, et al. Th22 cells control colon tumorigenesis through STAT3 and polycomb repression complex 2 signaling. Oncoimmunology. (2016) 5:e1082704. doi: 10.1080/2162402X.2015.1082704
153. Zhuang Y, Peng LS, Zhao YL, Shi Y, Mao XH, Guo G, et al. Increased intratumoral IL-22-producing CD4+ T cells and Th22 cells correlate with gastric cancer progression and predict poor patient survival. Cancer Immunol Immunother. (2012) 61:1965–75. doi: 10.1007/s00262-012-1241-5
154. Liu T, Peng L, Yu P, Zhao Y, Shi Y, Mao X, et al. Increased circulating Th22 and Th17 cells are associated with tumor progression and patient survival in human gastric cancer. J Clin Immunol. (2012) 32:1332–9. doi: 10.1007/s10875-012-9718-8
155. Chen X, Wang Y, Wang J, Wen J, Jia X, Wang X, et al. Accumulation of T-helper 22 cells, interleukin-22 and myeloid-derived suppressor cells promotes gastric cancer progression in elderly patients. Oncol Lett. (2018) 16:253–61. doi: 10.3892/ol.2018.8612
156. Fukui H, Zhang X, Sun C, Hara K, Kikuchi S, Yamasaki T, et al. IL-22 produced by cancer-associated fibroblasts promotes gastric cancer cell invasion via STAT3 and ERK signaling. Br J Cancer. (2014) 111:763–71. doi: 10.1038/bjc.2014.336
157. Qin SY, Yang XW, Luo W, Chen M, Liu ZL, Su SB, et al. Association of interleukin 22 polymorphisms with gastric cancer risk. Tumor Biol. (2015) 36:2033–9. doi: 10.1007/s13277-014-2810-3
158. Vauhkonen H, Vauhkonen M, Sajantila A, Sipponen P, Knuutila S. Characterizing genetically stable and unstable gastric cancers by microsatellites and array comparative genomic hybridization. Cancer Genet Cytogenet. (2006) 170:133–9. doi: 10.1016/j.cancergencyto.2006.06.001
159. Wang YM, Li ZX, Tang FB, Zhang Y, Zhou T, Zhang L, et al. Association of genetic polymorphisms of interleukins with gastric cancer and precancerous gastric lesions in a high-risk Chinese population. Tumor Biol. (2016) 37:2233–42. doi: 10.1007/s13277-015-4022-x
160. Ji Y, Yang X, Li J, Lu Z, Li X, Yu J, et al. IL-22 promotes the migration and invasion of gastric cancer cells via IL-22R1/AKT/MMP-9 signaling. Int J Clin Exp Pathol. (2014) 7:3694. Available online at: https://pdfs.semanticscholar.org/45bc/2db52b66988d41be97227b759efb9716b387.pdf
161. Sah RP, Dawra RK, Saluja AK. New insights into the pathogenesis of pancreatitis. Curr Opin Gastroenterol. (2013) 29:523. doi: 10.1097/MOG.0b013e328363e399
162. Nikoopour E, Bellemore SM, Singh B. IL-22, cell regeneration and autoimmunity. Cytokine. (2015) 74:35–42. doi: 10.1016/j.cyto.2014.09.007
163. Xuan X, Tian Z, Zhang M, Zhou J, Gao W, Zhang Y, et al. Diverse effects of interleukin-22 on pancreatic diseases. Pancreatology. (2018) 18:231–7. doi: 10.1016/j.pan.2018.02.014
164. Curd LM, Favors SE, Gregg RK. Pro-tumour activity of interleukin-22 in HPAFII human pancreatic cancer cells. Clin Exp Immunol. (2012) 168:192–9. doi: 10.1111/j.1365-2249.2012.04570.x
165. Wen Z, Liao Q, Zhao J, Hu YA, You L, Lu Z, et al. High expression of interleukin-22 and its receptor predicts poor prognosis in pancreatic ductal adenocarcinoma. Ann Surg Oncol. (2014) 21:125–32. doi: 10.1245/s10434-013-3322-x
166. He W, Wu J, Shi J, Huo YM, Dai W, Geng J, et al. IL22RA1/STAT3 signaling promotes stemness and tumorigenicity in pancreatic cancer. Cancer Res. (2018) 78:3293–305. doi: 10.1158/0008-5472.CAN-17-3131
167. Ye ZJ, Zhou Q, Yin W, Yuan ML, Yang WB, Xiang F, et al. Interleukin 22-producing CD4+ T cells in malignant pleural effusion. Cancer Lett. (2012) 326:23–32. doi: 10.1016/j.canlet.2012.07.013
168. Zhao D, Long XD, Lu TF, Wang T, Zhang WW, Liu YX, et al. Metformin decreases IL-22 secretion to suppress tumor growth in an orthotopic mouse model of hepatocellular carcinoma. Int J Cancer. (2015) 136:2556–65. doi: 10.1002/ijc.29305
169. Briso EM, Guinea-Viniegra J, Bakiri L, Rogon Z, Petzelbauer P, Eils R, et al. Inflammation-mediated skin tumorigenesis induced by epidermal c-Fos. Genes Develop. (2013) 27:1959–73. doi: 10.1101/gad.223339.113
170. Nardinocchi L, Sonego G, Passarelli F, Avitabile S, Scarponi C, Failla CM, et al. Interleukin-17 and interleukin-22 promote tumor progression in human nonmelanoma skin cancer. Eur J Immunol. (2015) 45:922–31. doi: 10.1002/eji.201445052
171. Kim Y, Lee J, Kim J, Choi CW, Hwang YI, Kang JS, et al. The pathogenic role of interleukin-22 and its receptor during UVB-induced skin inflammation. PLoS ONE. (2017) 12:e0178567. doi: 10.1371/journal.pone.0178567
172. Zhang S, Fujita H, Mitsui H, Yanofsky VR, Fuentes-Duculan J, Pettersen JS, et al. Increased Tc22 and Treg/CD8 ratio contribute to aggressive growth of transplant associated squamous cell carcinoma. PLoS ONE. (2013) 8:e62154. doi: 10.1371/journal.pone.0062154
173. Zhang W, Chen Y, Wei H, Zheng C, Sun R, Zhang J, et al. Antiapoptotic activity of autocrine interleukin-22 and therapeutic effects of interleukin-22-small interfering RNA on human lung cancer xenografts. Clin Cancer Res. (2008) 14:6432–9. doi: 10.1158/1078-0432.CCR-07-4401
174. Cannavo S, Ferrau F, Cotta OR, Saitta S, Barresi V, Cristani MT. Increased serum interleukin-22 levels in patients with PRL-secreting and non-functioning pituitary macroadenomas. Pituitary. (2014) 17:76–80. doi: 10.1007/s11102-013-0468-2
175. Liu X, Yang J, Deng W. The inflammatory cytokine IL-22 promotes murine gliomas via proliferation. Exp Ther Med. (2017) 13:1087–92. doi: 10.3892/etm.2017.4059
176. Kong X, Feng D, Mathews S, Gao B. Hepatoprotective and anti-fibrotic functions of interleukin-22: therapeutic potential for the treatment of alcoholic liver disease. J Gastroenterol Hepatol. (2013) 28:56–60. doi: 10.1111/jgh.12032
177. Lu DH, Guo XY, Qin SY, Luo W, Huang XL, Chen M, et al. Interleukin-22 ameliorates liver fibrogenesis by attenuating hepatic stellate cell activation and downregulating the levels of inflammatory cytokines. World J Gastroenterol. (2015) 21:1531. doi: 10.3748/wjg.v21.i5.1531
178. Radaeva S, Sun R, Pan HN, Hong F, Gao B. Interleukin 22 (IL-22) plays a protective role in T cell-mediated murine hepatitis: IL-22 is a survival factor for hepatocytes via STAT3 activation. Hepatology. (2004) 39:1332–42. doi: 10.1002/hep.20184
179. Xing WW, Zou MJ, Liu S, Xu T, Gao J, Wang JX, et al. Hepatoprotective effects of IL-22 on fulminant hepatic failure induced by d-galactosamine and lipopolysaccharide in mice. Cytokine. (2011) 56:174–9. doi: 10.1016/j.cyto.2011.07.022
180. Qin S, Ma S, Huang X, Lu D, Zhou Y, Jiang H. Th22 cells are associated with hepatocellular carcinoma development and progression. Chin J Cancer Res. (2014) 26:135–41. doi: 10.3978/j.issn.1000-9604.2014.02.14
181. Jiang R, Tan Z, Deng L, Chen Y, Xia Y, Gao Y, et al. Interleukin-22 promotes human hepatocellular carcinoma by activation of STAT3. Hepatology. (2011) 54:900–9. doi: 10.1002/hep.24486
182. Lim C, Savan R. The role of the IL-22/IL-22R1 axis in cancer. Cytokine Growth Factor Rev. (2014) 25:257–71. doi: 10.1016/j.cytogfr.2014.04.005
183. Scheiermann P, Bachmann M, Goren I, Zwissler B, Pfeilschifter J, Mühl H. Application of interleukin-22 mediates protection in experimental acetaminophen-induced acute liver injury. Am J Pathol. (2013) 182:1107–13. doi: 10.1016/j.ajpath.2012.12.010
184. Pan H, Hong F, Radaeva S, Gao B. Hydrodynamic gene delivery of interleukin-22 protects the mouse liver from concanavalin A-, carbon tetrachloride-, and Fas ligand-induced injury via activation of STAT3. Cell Mol Immunol. (2004) 1:43–9. Available online at: http://citeseerx.ist.psu.edu/viewdoc/download?doi=10.1.1.408.5569&rep=rep1&type=pdf
185. Mizoguchi A. Healing of intestinal inflammation by IL-22. Inflamm Bowel Dis. (2012) 18:1777–84. doi: 10.1002/ibd.22929
186. De Simone V, Franze E, Ronchetti G, Colantoni A, Fantini MC, Di Fusco D, et al. Th17-type cytokines, IL-6 and TNF-α synergistically activate STAT3 and NF-kB to promote colorectal cancer cell growth. Oncogene. (2015) 34:3493–503. doi: 10.1038/onc.2014.286
187. Katara GK, Kulshrestha A, Schneiderman S, Ibrahim S, Bilal M, Riehl VE, et al. IL-22 is specifically required for malignancy in breast cancer: a potential target to control cancer metastasis. Mol Oncol. (2020) 14:211–24. doi: 10.1158/1538-7445.SABCS18-4600
188. Jiang R, Wang H, Deng L, Hou J, Shi R, Yao M, et al. IL-22 is related to development of human colon cancer by activation of STAT3. BMC cancer. (2013) 13:59. doi: 10.1186/1471-2407-13-59
189. Rui J, Chunming Z, Binbin G, Na S, Shengxi W, Wei S. IL-22 promotes the progression of breast cancer through regulating HOXB-AS5. Oncotarget. (2017) 8:103601. doi: 10.18632/oncotarget.22063
Keywords: IL22-producing cells, wound healing—physiopathology, tissue regeneration, carcinogenesis and metastasis, innate immune response and inflammation
Citation: Arshad T, Mansur F, Palek R, Manzoor S and Liska V (2020) A Double Edged Sword Role of Interleukin-22 in Wound Healing and Tissue Regeneration. Front. Immunol. 11:2148. doi: 10.3389/fimmu.2020.02148
Received: 11 May 2020; Accepted: 07 August 2020;
Published: 17 September 2020.
Edited by:
Zlatko Kopecki, University of South Australia, AustraliaReviewed by:
Lyn Wise, University of Otago, New ZealandGaurav K. Gupta, University of Rochester, United States
Copyright © 2020 Arshad, Mansur, Palek, Manzoor and Liska. This is an open-access article distributed under the terms of the Creative Commons Attribution License (CC BY). The use, distribution or reproduction in other forums is permitted, provided the original author(s) and the copyright owner(s) are credited and that the original publication in this journal is cited, in accordance with accepted academic practice. No use, distribution or reproduction is permitted which does not comply with these terms.
*Correspondence: Vaclav Liska, vena.liska@skaut.cz; Sobia Manzoor, sobia.manzoor@lfp.cuni.cz; dr.sobiamanzoor@asab.nust.edu.pk
†These authors have contributed equally to this work