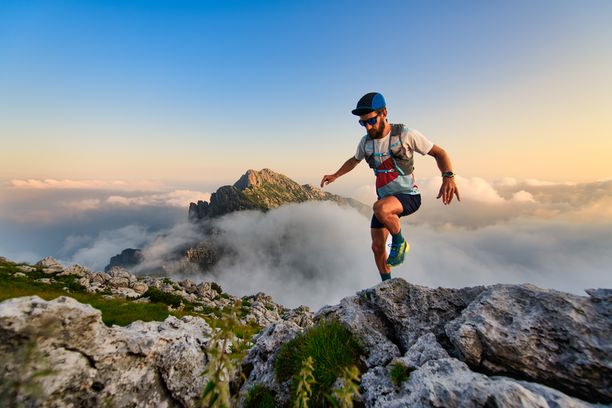
95% of researchers rate our articles as excellent or good
Learn more about the work of our research integrity team to safeguard the quality of each article we publish.
Find out more
ORIGINAL RESEARCH article
Front. Immunol., 20 August 2020
Sec. Comparative Immunology
Volume 11 - 2020 | https://doi.org/10.3389/fimmu.2020.01857
This article is part of the Research TopicGastrointestinal Immunity and Crosstalk with Internal Organs in FishView all 13 articles
Vibriosis is a commonly found bacterial disease identified among fish and shellfish cultured in saline waters. A multitude of Vibrio species have been identified as the causative agents. LamB, a member of outer membrane protein (OMPs) family of these bacteria is conserved among all Vibrio species and has been identified as an efficient vaccine candidate against vibriosis. Rootless duckweed (Wolffia) is a tiny, edible aquatic plant possessing characteristics suitable for the utilization as a bioreactor. Thus, we attempted to express a protective edible vaccine antigen against fish vibriosis in nuclear-transformed Wolffia. We amplified LamB gene from virulent Vibrio alginolyticus and it was modified to maximize the protein expression level and translocate the protein to the endoplasmic reticulum (ER) in plants. It was cloned into binary vector pMYC under the control of CaMV 35S promoter and introduced into Wolffia globosa by Agrobacterium-mediated transformation. Integration and expression of the LamB gene was confirmed by genomic PCR and RT-PCR. Western blot analysis revealed accumulation of the LamB protein in 8 transgenic lines. The cross-protective property of transgenic Wolffia was evaluated by orally vaccinating zebrafish through feeding fresh transgenic Wolffia and subsequently challenging with virulent V. alginolyticus. High relative percent survival (RPS) of the vaccinated fish (63.3%) confirmed that fish immunized with transgenic Wolffia were well-protected from Vibrio infection. These findings suggest that Wolffia expressed LamB could serve as an edible plant-based candidate vaccine model for fish vibriosis and feasibility of utilizing Wolffia as bioreactor to produce edible vaccines.
Vibriosis is a serious disease commonly identified among fish and shellfish aquaculture and has become a major limiting factor in the aquaculture industry worldwide. A group of Gram-negative bacteria inhabiting in saline waters belonging to the genus Vibrio has been identified as the causative agent (1). Massive (mis) use of antibacterials to control vibriosis has resulted in severe environmental as well as health concerns (2). Thus, development of vaccination strategies against vibriosis will be an effective solution for the management of vibriosis in aquaculture. Most vaccines currently used (especially for viral diseases) come from lab cultured pathogens through attenuation or inactivation, bringing potential risk of residual pathogenic activity. In this regard, recombinant proteins expressed in plant bioreactors are safer and more reliable, as they contain specific components of pathogens with the immunological properties of the original pathogen but not its pathogenic properties (3, 4).
Among fish vaccine delivery techniques, oral routes (oral immunization) would be an attractive alternative, as it is simple, cheap, and ideal for mass administration to fish of all sizes without causing a stress. However, oral immunization is a multifaceted process, depending on multiple cellular and molecular mechanisms. As teleost, fish lack Peyer's patches and antigen-transporting M cells, which are important to initiate the gut immune responses but their second segment of the gut has been identified as the main site of antigen uptake (5, 6). For that, many lymphoid cells and macrophages are diffusely present between the epithelial cells and in the lamina propria enterocytes of the hind gut (6, 7). Thus, as teleost fish intestine can easily be exploited for oral vaccination strategies.
Oral vaccination of fish has become less effective due to digestive degradations of antigens in the acidic environment of the foregut, before it reaches the immune responsive areas of the hind gut (8). Thus, while developing edible vaccines, special concern should be given to protect antigen from the harsh gastric environment to ensure antigen uptake in the second gut segment of fish. Protective antigens expressed in transgenic plants are the ideal solution for such issues. Thick, rigid cell walls of the plants encapsulate the antigenic proteins, thus protecting them well from the acidic environment of the stomach. They act as vehicles to orally deliver protective antigens (9) to get through the acidic environment of the foregut without intestinal degradation. Thus, the antigen can reach the second gut in the intestine safely in sufficient quantities and successful oral vaccination can be achieved.
Outer membrane proteins (OMPs) are unique components reside in the outer membrane of Gram-negative bacteria and responsible for maintaining the integrity and selective permeability of the bacterial outer membrane (10). As they are being localized at the bacterial cell surfaces, OMPs of Gram-negative bacteria could be efficiently recognized as foreign substances by immunological defense systems of hosts. Thus, OMPs of Gram-negative bacteria have been identified as highly immunogenic components due to their exposed epitopes as well as being conserved in nature (11). LamB proteins are a family of OMPs identified in vibrios and Lun et al. (12) has reported that it can be used as a broad cross-protective vaccine candidate against vibriosis.
Wolffia, the rootless duckweed is the smallest member of the duckweed family as well as in the plant kingdom (13). Due to its prominent asexual propagation, rootless characteristics and extremely reduced size, it has become an attractive, highly efficient bio-manufacturing platform for foreign protein production and makes it a more suitable candidate for submerged cultivation in bio-fermenters (14). Thus, high biomass of genetically homogeneous Wolffia populations can be easily obtained within the compact environment on artificial media. As it is an edible plant, the proteins in transgenic Wolffia can be directly consumed without extraction and/or purification of the active constituent. This is one of the main advantages identified in this system and makes Wolffia a more attractive member among the duckweed family, especially in edible vaccine research.
Lack of an efficient gene transformation protocol for Wolffia has limited the utilization of this valuable plant as a bio-manufacturing platform. To fulfill this gap in our previous work, we developed an efficient gene transformation protocol for W. globosa, the Wolffia species available in China (15). As the second step, here we focused on exploring the feasibility to express an edible protective vaccine antigen LamB for fish vibriosis in W. globosa.
LamB outer membrane protein of Vibrio bacteria was selected as the antigenic protein to express in W. globosa. The pathogenic Vibrio alginolyticus ECGY0608 (kindly given by Professor Ai-hua Li, Institute of Hydrobiology, Chinese Academy of Sciences) isolated from diseased fish was used to amplify the LamB gene. Genomic DNA of V. alginolyticus was extracted from overnight cultures in the TSA medium with a TIANamp Bacteria DNA kit (Cat.−DP 302, Tian GEN Biotech Beijin Co. LTD) according to the manufacturer's protocol. DNA extracts obtained were used as templates to amplify the LamB gene using degenerate primers. The primer sequences were lamB-f: 5-ATG AAA AAA GTA AGT SNY ATT GCA G-3 and LamB-r: 5-TTA CCA CCA AGC TTC NRC TTG-3 (12). PCR was carried out in a thermal cycler (Eppendorff, USA) with the following set up: Initial denaturation for 5 min at 95°C, Then 30 cycles were run with conditions: 95°C for 30 s, 48°C for 45 s, and 72°C for 1 min and final cycle of 72°C for 7 min. The PCR product was analyzed by electrophoresis on a 1.2% agarose gel, and purified with Easy Pure PCR purification kit (TRANS Gen Biotech). The amplified DNA fragment was cloned into the pGEM-T-Easy vector (Promega) and sequenced (http://www.tsingke.net).
The identities of the nucleotide sequences were determined by comparing with known sequences in GenBank using the respective BLAST program available at NCBI (http://www.ncbi.nlm.nih.gov/).
The LamB gene obtained was further modified to achieve higher protein expression, accumulation, and isolation. To enhance Agrobacterium tumefaciens-mediated stable gene expression in W. globosa, we designed the construct to express ER targeted LamB fusion protein under the control of cauliflower mosaic virus (CaMV) 35S promoter, a tCUP translational enhancer, PR1b signal peptide, and the nopaline synthase (nos) terminator in the plant expression vector pMYC.
Thus, tobacco cryptic constitutive promoter (tCUP) enhancer and pathogenesis-related protein 1b (PR1b) signal peptide were synthesized (http://www.tsingke.net) and spliced together with the 5′ end of the LamB sequence by overlap extension (16). MYC protein tag and KDEL retention signal peptide was incorporated to the 3′ end of LamB by newly designed primers. Specific restriction sites (Pst1/Ncol1/Sal1/BamHI) were designed from the cloned nucleotide sequence to amplify the encoding sequence for the mature LamB protein, and incorporated into the PCR during LamB gene modification. Primer sequences used to modify the LamB gene are shown in Table 1. Schematic representation of the expression cassette (modified LamB gene) is shown in Figure 1. It was sub-cloned into the pGEM-T-Easy vector (Promega) and the modifications were confirmed by sequencing (http://www.tsingke.net).
Figure 1. Schematic representation of T-DNA region of the expression vector (pMYC-LamB) construct used for W. globosa transformation. Transgene expression fragment (LamB) was placed under the control of the cauliflower mosaic virus 35S promoter, tCUP, translational enhancer from the tobacco cryptic upstream promoter; Pr1b, tobacco pathogenesis related 1b protein secretary signal peptide; C-myc, detection/purification tag; KDEL, endoplasmic reticulum retrieval tetrapeptide; nos, nopaline synthase transcription terminator.
Then the LamB expression cassette was cut out from the pGEM-T-Easy between cut sites Pst1 and BamHI and using the same sites, cloned into pMYC binary vector under the control of the CaMV 35S promoter. Thus, the gene was located downstream from the 35S cauliflower mosaic virus promoter (CaMV35S) and upstream of the nopaline synthase 3′ UTR (NOSt) to give the binary vector pMYC-LamB. We confirmed the successful insertion of the LamB expression cassette into the binary vector pMYC-LamB by restriction enzyme digestion and sequencing (http://www.tsingke.net). The resulting binary vector pMYC-LamB was used for LamB protein expression in W. globosa, following its Agrobacterium-mediated transformation (15).
Thus, the construct (pMYC-LamB) was mobilized into the commercially available disarmed Agrobacterium strain EHA105 (http://www.transgen.com.cn/) by heat shock according to the manufacture's protocol and used for the transformation of W. globosa.
W. globosa fronds were collected from Wuhan Botanical Garden (RDSC Clone W. globosa 5563), Chinese Academy of Sciences (CAS) (30.54° N and 114.42° E) at the city of Wuhan, Hubei province, China. Aseptic populations of W. globosa single clones were obtained in solid SH medium (17) with 2% sucrose and 0.6% agar and used as explants in this study. W. globosa clusters were induced by culturing explants in cluster induction medium for 4 months (18). Clusters obtained were used for the transformation experiments.
Agrobacterium-mediated nuclear transformation was performed as previously described (15). Briefly, W. globosa clusters were mixed with glass beads and bacterial suspension of A. tumefaciens (EHA105 harboring the pMYC-LamB construct) and subjected to vigorous shaking for 30 min to injure the clusters. Subsequently, these were blotted onto sterile filter paper, and co-cultivated for 72 h on antibiotic-free liquid SH medium. Then clusters were subjected to 2 week resting period and finally for 1 month selection. Plant selectable marker used in the construct was Hyg-R. Thus, Hygromycin B (Hyg) was used as the selection agent at the concentration of 5.0 mgl−1 to select transgenic explants as well as to obtain pure transgenic lines. All steps of transformation experiments were carried out at 25 ± 1°C under the white light of 85 μmol m−2 s−1, 16: 8 h light: dark photoperiod.
To confirm gene integration within the plant genome we first performed a PCR assay. Total genomic DNA from the putative transgenic and wild-type W. globosa explants was extracted using a plant genome extraction kit, NuClean PlantGen DNA Kit (CWBIO, China) (http://www.cwbiotech.com.cn/) according to the manufacture's protocol. Genomic DNA obtained was used as the template to amplify the modified LamB gene integrated. PCR analysis of putatively transgenic plants was performed using PCR primers, Overlap F—a and Overlap R—a (Table 1). Overlap PCR primers amplify a 1,548-bp fragment comprising the sequence of modified Lam B gene.
Total RNA from wild and transformed W. globosa was extracted using the Trizol reagent (Invitrogen). cDNA was synthesized with 2 μg of total RNA using a Prime Script RT reagent Kit (Takara) according to the manufacture's protocol. RT-PCR for LamB was conducted with newly designed LamB gene specific primers (LamB-RT-F: 5′-GTTTCTTTCGCTTGGGTTCG-3′, LamB-RT-R: 5′-CATTACGCCGTTTTTCGCAT-3′). An Actin gene was used as the internal control. Actin gene was amplified using two degenerate primers, ActF: 5′-GTGYTKGAYTCTGGTGATGGTGT-3′ and ActR: 5′-ACCTTRATCTTCATGCTGCTSGG-3′ (15).
Total soluble protein was extracted from transgenic and wild type Wolffia fronds. The fronds were ground in liquid nitrogen to a fine powder. 0.5 g powder was re-suspended in 4 volumes of extraction buffer [50 mM Tris–HCl, pH 8.0, 10 mM EDTA, pH 8.0, 10% (v/v) glycerol, 1% (w/v) SDS, 30 mM 2-mercaptoethanol, 4 μg/ml aprotinin, and 4 μg/ml leupeptin]. Total proteins were extracted for 20 min at 4°C and then centrifuged at 14,000× g for 10 min at 4°C. Supernatant was taken for further analysis. Protein extracts were quantified with the help of BCA protein assay kit (Beyotime, China, Cat. P0012) according to the manufacturer's instructions.
Protein extracts were subjected to fully automated capillary electrophoresis (CE) based Western blot assay to identify the recombinant LamB protein in transgenic Wolffia. Automated Western blot was performed by the Peggy Sue instrument, Protein Simple (Santa Clara, CA, USA). Biotinylated molecular weight ladder, streptavidin-HRP, DTT, fluorescent standards, luminol-S, peroxide, sample buffer, stacking matrix, separation matrix, running buffers, wash buffer, and the HRP-conjugated secondary antibody, were used according to the manufacture's protocol. Mouse anti-myc (QW–bio, China) was used as the primary antibody. “Virtual blot” electrophoretic images were generated using Compass Software (ProteinSimple). The kit also provides capillaries, antibody diluents, and sample loading plates.
Healthy and V. alginolyticus infection-free four months old wild type zebrafish (AB strain) obtained from single parental stock were purchased from a fish farm/Institute of Hydrobiology (Wuhan, China) (mean weight 0.4 g; length 2–3 cm) and acclimatized for 2 months prior to experiments. During acclimatization, fish were kept in tanks with running water. Water temperature was ~28°C and fish were reared at 12:12 h light: dark cycle. Fish were fed twice a day with commercial feed and wild type W. globosa. The animal studies were approved by the Animal Care and Use Committee of the Institute of Hydrobiology, Chinese Academy of Sciences (approval ID Keshuizhuan Y73Z061).
From the acclimatized fish, 270 apparently healthy (no clinical signs) individuals were randomly divided into three groups at a density of 30 fish/tank. A description of oral immunization and challenge infection is shown in Figure 2. The fresh biomass of wild type and transgenic W. globosa were used for the vaccination experiment. The fish in group 1 were fed with commercial feed twice a day during the experimental period and it was used as the control group. The fish in group 3 were immunized (primary immunization) by feeding with transgenic W. globosa and commercial feed twice a day for the period of 2 months. Then booster vaccination was performed for another month after 1 month interval of primary immunization. The fish in group 2 were fed with wild type untransformed W. globosa and commercial feed according to the schedule described in Figure 2. Oral vaccination experiments were performed in biological triplicates. Blood was collected from vaccinated and unvaccinated fish (from five fish at each time) at 5, 6, 7, 8, 9, and 10 weeks after booster vaccination. Blood (5 μl/fish) was collected from the caudal vein of fish by placing a capillary tube through the caudal fin cut. The blood was allowed to clot by keeping at room temperature for 2 h and then at 4°C overnight. Serum was collected after centrifugation at 750 × g for 10 min and was stored at −80°C for ELISA.
Six weeks post-vaccination, 30 fish from each group (group 1, 2, and 3) were anesthetized by immersing in tricaine methane sulfonate (MS-222) solution and challenged by intraperitoneal inoculation with 10 μl of 3.25 × 108 cfu/ml cell suspension. Thirty fish were challenged by intraperitoneal inoculation with 10 μl PBS as control. Bacterial suspension was prepared as follows: virulent Vibrio alginolyticus was cultured at 28°C in TSB medium for 18 h, harvested by centrifugation at 4,000 g for 10 min and washed with PBS three times. Bacteria were suspended in 0.01 M PBS (pH 7.4) and adjusted to the concentration of 3.25 × 1010 cells/ml. In a preliminary assay, the median lethal dose (LD50) was determined to be 6.5 × 107 CFU per fish. Fish from vaccinated and control groups were challenged using five times their lethal dose (LD50) (12). Fish mortality was monitored daily for 14 days, and dead fish were removed on a daily basis. The relative percent survival (RPS) was calculated by the following formula: RPS = [1− (% mortality of vaccinated fish/% mortality of control fish)] × 100%.
To analyze the protein by a more sensitive method for the detection of immunogenic epitopes of expressed protein, antibody titer was determined from the immunized fish by sandwich ELISA. Briefly, the sera of zebrafish were serially diluted in carbonate/bicarbonate buffer, pH 7.4 (1:1, v/v) and added in triplicate to wells of the plates. The plates were incubated overnight at 4°C. The remaining binding sites were blocked 1 h at 37°C with PBS-TS. Then 100 μl purified LamB (0.5 μg/well) was added to the plates. After incubation at 37°C for 2 h and washing three times with PBS-T, rabbit anti-LamB sera (1:1000 diluted in PBS-TS) were added to the plates. The plates were incubated and washed as above. HRP conjugated with goat-anti rabbit IgG (1:1000 diluted in PBS-TS) was added and incubated for another 1 h at 37°C and was then reacted with o-Phenylenediamine (OPD) substrate (Beyotime) for 10 min. Finally, the reaction was blocked by 2M H2SO4, and the absorbance of each and every well was measured at 492 nm with the help of Bio-Rad iMark micro-plate reader. The student's t-test was used to conduct significant tests.
From the genomic DNA of pathogenic V. alginolyticus, we successfully amplified the 1,200 bp full-length ORF of antigenic LamB gene. The deduced amino acid sequence of amplified gene consists of 447 amino acid residues. Gene was amplified using degenerate LamB primers as described by Lun et al. (12). According to Lun et al. (12), the annealing temperature of the PCR reaction was 58°C. However, though we were unable to amplify the LamB gene at this temperature, we were able to successfully amplify it at an annealing temperature of 48°C. The gene sequence was subjected to the homology search BLASTn in NCBI (http://www.ncbi.nlm.nih.gov/). Results showed 99% similarity with the LamB gene of V. alginolyticus (Accession no. EU625279). With this finding, the gene amplified was confirmed as the LamB gene of V. alginolyticus and it was used to construct the expression vector (pMYC-LamB) to transform antigenic LamB gene into Wolffia plant for antigenic protein expression trials.
We obtained hygromycin-resistant transgenic Wolffia fronds after 7–8 weeks of cultivation on SH medium in the presence of 5.0 mg l−1 hygromycin (Hyg). In total, 8 independent hygromycin-resistant transgenic Wolffia lines with LamB gene construct were identified and those were confirmed by PCR conducted with LamB gene specific primers (Figure 3A). To confirm that the LamB gene was correctly transcribed, the transgenic Wolffia were characterized in more detail by RT-PCR with LamB gene specific primers. PCR products of the expected size corresponding to the specific primers were detected in the transgenic Wolffia, whereas no amplified PCR product was detected in non-transformed wild-type Wolffia (Figure 3B). The Actin gene was used as an internal control. All transgenic Wolffia lines we tested were positive for the LamB transcript (Figure 3B).
Figure 3. Screening transgenic Wolffia and confirmation of the modified LamB gene integration. (A) PCR amplification of modified LamB gene from Wolffia transformant. L: 2,000 bp ladder, Lane 1–3: contained 3 independent LamB integrated Wolffia lines. Lane 4: (Marked as +ve) contains plasmid extract containing LamB construct and it was used as the positive control. Lane 5: (marked –ve) contains genomic DNA from wild plants. (B) RT-PCR for the LamB in Wolffia transformant. An Actin gene was used as the internal control. Lanes 1–3: Putative Wolffia transformants, Lanes 4: Wild type plants.
CE based western blot assay was conducted with the total soluble protein extracted from the transgenic Wolffia to confirm the expression of recombinant LamB protein. Probing blots with mouse anti-myc primary antibody revealed full-length 52-kDa protein (Figure 4A). This product is consistent with the predicted size of fully intact recombinant LamB protein. The total soluble protein from wild-type plants did not show any band, indicating that mouse anti-myc antibody did not cross-react with any plant proteins in the crude extract. This confirmed the production of recombinant LamB antigenic protein in transformed W. globosa. The signal intensity (area) of CE based Western blot assay corresponding to the expression level of recombinant protein varied between three transgenic lines, indicating the different levels of protein expression in transgenic Wolffia. Figure 4B shows the different expression levels (signal intensity/area) obtained for three transgenic lines. The highest level of signals was detected in the S2 transgenic line and signal from S1 was comparatively weak according to the CE based western blot.
Figure 4. Capillary electrophoresis (CE) based Western blot assay conducted for detection of recombinant LamB protein in protein extracts of transgenic Wolffia. (A) Gel-like image viewed from Western blot assay. Left to right: BTL: Biotinylated ladder WT: Un-transformed wild type plant, S1–S3: Transformed plants. Anti- c- myc mouse monoclonal antibody was used as a primary antibody and the HRP-conjugated mouse anti-mouse IgG antibody used as a secondary antibody. (B) Signal intensity (area) shown in Capillary electrophoresis (CE) based Western blot assay for detection of recombinant LamB protein in protein extracts from the fronds of wild type and transformed Wolffia. WT - Wild type Wolffia, S1–S3 transgenic Wolffia lines.
Zebrafish were orally immunized with transgenic Wolffia and subsequently challenged with the pathogenic V. alginolyticus to evaluate the cross-protective potential of LamB recombinant protein produced in transgenic Wolffia. Fish mortality rate and sandwich ELISA were determined to evaluate the efficacy of this oral vaccine. Cumulative mortality of challenged zebrafish is shown in Table 2. Mortality rate of LamB vaccinated zebrafish was significantly lower compared to the control group (P < 0.05). The RPS of LamB vaccinated group was 63.3%. These results confirmed the protective capacity of LamB vaccinated zebrafish against V. alginolyticus. However, the antibodies against LamB protein in the sera of vaccinated fish were not detected by sandwich ELISA.
Table 2. Cumulative mortality of challenged zebrafish after vaccination by V. alginolyticus ECGY0608.
Among members of the duckweed family, Wolffia has been identified as a valuable bioreactor for foreign protein production (19). Lack of an efficient gene transformation protocol has limited the utilization of this valuable plant as a bio-manufacturing platform. To fulfill this gap in our previous work, we developed an efficient gene transformation protocol for W. globosa, the Wolffia species available in China (15). Use of antibiotics to control vibriosis in aquaculture causes serious health and environmental issues (20). Immunoprophylaxis would be a promising tool for effective control of this disease in a more effective and economical manner. Thus, as the second phase of our study, we attempted to demonstrate bioencapsulation of vaccinogenic outer membrane protein of vibrio in Wolffia for oral delivery against fish vibriosis.
With the morphological and functional differences investigated within the fish intestine, its second segment was identified as the main place of antigen uptake (5, 6). Studies conducted by Rombout et al. (21) and Firdaus (22) using fish with formalin killed V. anguillarum and feed-based adjuvant vaccine of Streptococcus agalactiae have proven that oral vaccination in fish could elicit certain levels of humoral and mucosal immunities following the development of gut-associated lymphoid tissue (GALT) (23). Thus, oral vaccines will be effective immune stimulators only if the antigenic substances reach the correct inductive sites in the fish gut. Accordingly, to achieve highly efficient edible vaccines, special techniques need to be developed to prevent antigenic substances from biodegradation in the proteolytic environment of the fish foregut till it reaches the local immune system.
Encapsulating vaccinogenic components in edible plants has become popular due to a number of advantages such as low production cost, direct administration by feeding without a needle and syringe and possible long-term storage without special storage facilities. Until now, many successful oral vaccines by expressing recombinant proteins in edible plants with higher expression level have been published for human diseases but for the fish diseases it is limited. In these studies, producing vaccine antigens in edible plants, including tomato, potato, lettuce, or rice has been identified as a viable approach for expressing mucosal vaccines (23–26). In particular, Shina et al. (27) has reported expression of a vaccinogenic recombinant major capsid protein (rMCP) of rock bream iridovirus in transgenic rice callus against iridovirus of fish. In this study oral immunization of Rock bream with rMCP in lyophilized rice callus powder has elicit the intestinal mucosal immunity for protection against iridovirus infection. This study suggests that oral administration of plant expressed vaccine antigen as a useful method to implement a vaccine program against fish diseases.
Recently duckweeds have been identified as a more efficient bio-manufacturing platform to express heterologous proteins. However few reports on utilizing duckweeds for protein expression can be seen (28–30) and Lemna was the commonly used species. Single attempt on utilizing Wolffia to express human granulocyte colony-stimulating factor (hG-CSF) by nuclear-transformation at the level of 0.002–0.2% has been reported (31). But expressing vaccine antigens has not been reported.
Outer membrane proteins (OMP) of gram negative bacteria are identified as a type of vaccine candidate and used to formulate vaccine against bacterial pathogens. OMPs of bacteria are being localized at the exteriors of the cell surface and are targets for bactericidal and protective antibodies (32, 33). Thus, we focused on LamB OMP of Vibrio to be expressed in W. globosa, which has already been identified as a broad cross protective vaccine antigen against fish vibriosis (12). We constructed LamB expression vector and introduced LamB gene into the nuclear genome of Wolffia as an alternative approach to prevent fish vibriosis.
Development of effective plant based edible vaccines deals with the level of foreign protein expressed in transgenic plants. Higher protein expression is necessary to induce protective immune responses of host via oral routes of delivery. Tobacco cryptic constitutive promoter (tCUP) is a translational enhancer sequence used for the expression vectors and it is being effective across a wide range of plant species, including monocots, dicots, or gymnosperms and a wide range of other organisms such as yeast (34). It can be combined with other promoters, such as CaMV 35S promoter, to elevate their activity further (34, 35). Thus, for our LamB expression construct, we used tCUP translational enhancer sequence to achieve higher expression of heterologous LamB protein in Wolffia. Moreover, signal peptides and endoplasmic reticulum (ER) retention signals are often used in expression vectors for correct compartmentalization and retention of the heterologous protein in plant ER (36–39). This minimizes the modifications happening to the glycoproteins in Golgi apparatus (40). Thus, we used PR1b signal peptide sequence and ER retention signal KDEL for our construct to direct and keep the homologous LamB protein in ER.
Finally using the pMYC-LamB construct and the Agrobacterium-mediated stable nuclear transformation protocol we developed, we successfully expressed the homologous LamB antigenic gene in Wolffia, and regeneration of transgenic Wolffia occurred at a satisfactory level. The PCR and transcription analysis conducted with the transgenic Wolffia indicated that the foreign genes have been transcribed correctly. All transgenic Wolffia lines were phenotypically indistinguishable from wild type control fronds. The development and growth rate of these transgenic Wolffia did not differ from the corresponding characteristics of the non-transformed control fronds. This indicates that the expression of the foreign protein has no effect on the growth and development of transgenic Wolffia. Thus, we successfully utilized this less/under exploited plant species, W. globosa as a novel vaccine expression platform to express the antigenic LamB against vibriosis. As an edible aquatic plant, this antigenic protein expressed transgenic Wolffia can be used to vaccinate the herbivorous fish through direct feeding without extraction and/or purification of the active constituent. To vaccinate the carnivorous fish, transgenic Wolffia can be fed with meat or commercial feeds.
The signal intensity (area) of CE based western blot assay corresponding to the expression level of recombinant LamB protein varied between three independent transgenic Wolffia lines. This indicates that the protein expression in the three transgenic lines is variable. This variation may be due to differences in the location/integration site of the newly introduced gene, the copy number of the T-DNA integrated and/or T-DNA organization (41, 42), as well as epigenetic effects (43). However, in our study we were unable to quantify the LamB protein accumulation in transgenic Wolffia due to the unavailability of protein standards.
The biological activity of recombinant LamB protein was assessed through bacterial challenge followed with an immunological assay. According to our findings the protective capacity of recombinant LamB against pathogenic V. alginolyticus in immunized fish were significant. This result confirmed the protective capacity of recombinant LamB against V. alginolyticus in fish. Even though the challenge test clearly indicates the protective capacity of transgenic Wolffia, with the ELISA we were unable to detect the antibodies against LamB in the sera of vaccinated fish. Some identified reasons for this type of erroneous results are competitive inhibition of test antibodies by relevant antibodies present in animal serum, denaturation of enzymes conjugated to detection antibodies, change in antibody specificity due to antigenic drift, sampling time and condition of the fish (44, 45).
In conclusion, we have successfully expressed antigenic LamB outer membrane protein of Vibrio in transgenic Wolffia against fish vibriosis and the successful nuclear transformation of LamB fusion protein with their functional properties is further demonstrated. Confirmation on its immunogenic property needs to be verified further by immunological studies to accomplish its immunostimulant property. However, our study opens the way to utilize the smallest flowering plant, Wolffia, for the development of low cost, easily deliverable and effective subunit vaccines.
All datasets generated for this study are included in the article/supplementary material.
The animal study was reviewed and approved by Animal Care and Use Committee of the Institute of Hydrobiology, Chinese Academy of Sciences (approval ID Keshuizhuan Y73Z061).
HH and PH planned and designed the research. PH, ZS, JY, and XZ performed experiments and data analysis. PH, ZS, and HH wrote the manuscript. All authors contributed to manuscript revision and read and approved the submitted version.
This work was supported by National Key R & D Program (2018YFD0900801), the Major Project of Technology Innovation Program of Hubei, China (2017ABA135), Open Project of Guangdong Provincial Key Laboratory of Applied Botany, South China Botanical Garden, Chinese Academy of Sciences (AB2018020), and Hubei Province Postdoctoral Science Foundation.
The authors declare that the research was conducted in the absence of any commercial or financial relationships that could be construed as a potential conflict of interest.
The authors are thankful to the University of Chinese Academy of Sciences (UCAS) scholarship program.
1. Austin B, Austin DA. Bacterial Fish Pathogens: Disease of Farmed and Wild Fish. Chichester: Springer Praxis Publishing Ltd (2007). p. 61–150.
2. Heuer OE, Kruse H, Grave K, Collignon P, Karunasagar I, Angulo FJ. Human health consequences of use of antimicrobial agents in aquaculture. Clin Infect Dis. (2009) 49:1248–53. doi: 10.1086/605667
3. Marsian J, Lomonossoff GP. Molecular pharming - VLPs made in plants. Curr Opin Biotech. (2016) 37:201–6. doi: 10.1016/j.copbio.2015.12.007
4. Giddings G. Transgenic plants as protein factories. Curr Opin Biotech. (2001) 12:450–4. doi: 10.1016/S0958-1669(00)00244-5
5. Georgopoulou U, Sire MF, Vernier JM. Immunological demonstration of intestinal absorption and digestion of protein macromolecules in the trout (Salmo gairdneri). Cell Tissue Res. (1986) 245:387–95. doi: 10.1007/BF00213946
6. Rombout JHWM, Van den Berg AA, Van den Berg CTGA, Witte P, Egberts E. Immunological importance of the second gut segment of carp. III. systemic and/or mucosal immune responses after immunization with soluble or particulate antigen. Fish Biol. (1989) 36:179–86. doi: 10.1111/j.1095-8649.1989.tb02967.x
7. Georgopoulou U, Vernier JM. Local immunological response in the posterior intestinal segment of the rainbow trout after oral administration of macromolecules. Dev Comp Immunol. (1986) 10:529–37. doi: 10.1016/0145-305X(86)90174-6
8. Stefaan V, Frans O, Renaat K, Armand M. Mucosal response in African catfish after administration of Vibrio anguillarum O2 antigens via different routes. Fish Shellfish Immun. (2005) 18:125–33. doi: 10.1016/j.fsi.2004.06.004
9. Rigano MM, Walmsley AM. Expression systems and developments in plant-made vaccines. Immunol Cell Biol. (2005) 83:271–7. doi: 10.1111/j.1440-1711.2005.01336.x
10. Nikaido H. Molecular basis of bacterial outer membrane permeability revisited. Microbiol Mol Biol R. (2003) 67:593–656. doi: 10.1128/MMBR.67.4.593-656.2003
11. Khushiramani R, Girisha SK, Karunasagar I, Karunasagar I. Cloning and expression of an outer membrane protein ompTS of Aeromonas hydrophila and study of immunogenicity in fish. Protein Expr Purif. (2007) 51:303–7. doi: 10.1016/j.pep.2006.07.021
12. Lun J, Xia C, Yuan C, Zhang Y, Zhong M, Huang T, et al. The outer membrane protein, LamB (maltoporin), is a versatile vaccine candidate among the Vibrio species. Vaccine. (2014) 32:809–15. doi: 10.1016/j.vaccine.2013.12.035
13. Landolt E. The family of Lemnaceae - a monographic study Vol 1. In: Landolt E, editor. Veroffentlichungen des Geobotanischen Institutes der Eidgenossischen Technischen Hochschule, Zurich: Geobotanical Institute of the ETH, Stiftung Rubel (1986).
14. Thompson BG. The maximization of the productivity of aquatic plants for use in controlled ecological life support systems (CELSS). Acta Astronaut. (1989) 19:269–73. doi: 10.1016/0094-5765(89)90039-8
15. Heenatigala PPM, Yang J, Bishopp A, Sun Z, Li G, Kumar S, et al. Development of efficient protocols for stable and transient gene transformation for Wolffia Globosa using Agrobacterium. Front. Chem. (2018) 6:227. doi: 10.3389/fchem.2018.00227
16. Horton RM, Hunt HD, Ho SN, Pullen JK, Pease LR. Engineering hybrid genes without the use of restriction enzymes: gene splicing by overlap extension. Genes. (1989) 77:61–8. doi: 10.1016/0378-1119(89)90359-4
17. Schenk RU, Hildebrandt AC. Medium and techniques for induction and growth of monocotyledonous and dicotyledonous plant cell cultures. Can J Bot. (1972) 50:199–204. doi: 10.1139/b72-026
18. Khvatkov P, Chernobrovkina M, Okuneva A, Shvedova A, Chaban I, Dolgov S. Callus induction and regeneration in Wolffia arrhiza (L.) Horkel ex Wimm. Plant Cell Tis Org. (2015) 120:263–73. doi: 10.1007/s11240-014-0603-4
19. Les DH, Crawford DJ, Landolt TE, Gabel JD, Kimball RT, Rettig JH. Phylogeny and systematics of lemnaceae, the duckweed family. Syst Bot. (2002) 27:221–40. doi: 10.1043/0363-6445-27.2.221
20. Subasinghe RP, Barg U, Tacon A. Chemicals in asian aquaculture: need, usage, issues and challenges. In: Proceeding of the Meeting on the Use of Chemicals in Aquaculture in Asia. Tigbauna, Iloilo, Philippines. (1996). p. 1–7. Available online at: http://hdl.handle.net/10862/612
21. Rombout JW, Blok LJ, Lamers CH, Egbert E. Immunization of carp (Cyprinus carpio) with a Vibrio anguillarum bacterin: Indications for a common mucosal immune system. Dev Comparative Immunol. (1986) 10:341–51. doi: 10.1016/0145-305X(86)90024-8
22. Firdaus NM. Immune responses to Streptococcus agalactiae in red tilapia, Oreochromis spp. following vaccination with non-adjuvanted and adjuvanted vaccine (FAV) incorporated feed pellets (Masters thesis), Universiti Putra Malaysia, Serdang Seri Kembangan, Malaysia (2011). Available online at: http://psasir.upm.edu.my/id/eprint/77356.
23. Embregts CW, Forlenza M. Oral vaccination of fish: Lessons from humans and veterinary species. Dev Comp Immunol. (2016) 64:118–37. doi: 10.1016/j.dci.2016.03.024
24. Fernandez-San Millan A, Ortigosa SM, Hervas-Stubbs S, Corral-Martinez P, Segui-Simarro JM, Gaetan J, et al. Human papillomavirus L1 protein expressed in tobacco chloroplasts self-assembles into virus-like particles that are highly immunogenic. Plant Biotechnol J. (2008) 6:427–41. doi: 10.1111/j.1467-7652.2008.00338.x
25. Koya V, Moayeri M, Leppla SH, Daniell H. Plant-based vaccine: mice immunized with chloroplast-derived anthrax protective antigen survive anthrax lethal toxin challenge. Infect Immun. (2005) 73:8266–74. doi: 10.1128/IAI.73.12.8266-8274.2005
26. Scotti N, Alagna F, Ferraiolo E, Formisano G, Sannino L, Buonaguro L, et al. High-level expression of the HIV-1 Pr55gag polyprotein in transgenic tobacco chloroplasts. Planta. (2009) 229:1109–22. doi: 10.1007/s00425-009-0898-2
27. Shina YJ, Kwona TH, Seob JY, Kimb TJ. Oral immunization of fish against iridovirus infection using recombinant antigen produced from rice callus. Vaccine. (2013) 31:5210–5. doi: 10.1016/j.vaccine.2013.08.085
28. Ko SM, Sun HJ, Oh MJ, Song IJ, Kim MJ, Sin HS, et al. Expression of the protective antigen for PEDV in transgenic duckweed, Lemna minor. Hortic Environ Biote. (2011) 52:511–5. doi: 10.1007/s13580-011-0007-x
29. Firsov A, Tarasenko I, Mitiouchkina T, Ismailova N, Shaloiko L, Vainstein A, et al. High-yield expression of M2e peptide of avian influenza virus H5N1 in transgenic duckweed plants. Mol Biotechnol. (2015) 57:653–61. doi: 10.1007/s12033-015-9855-4
30. Firsov A, Tarasenko I, Mitiouchkina T, Shaloiko L, Kozlov O, Vinokurov L, et al. Expression and immunogenicity of M2e peptide of avian influenza virus H5N1 fused to ricin toxin B chain produced in duckweed plants. Front Chem. (2018) 6:22. doi: 10.3389/fchem.2018.00022
31. Khvatkov P, Firsov A, Shvedova A, Shaloiko L, Kozlov O, Chernobrovkina M, et al. Development of Wolffia arrhiza as a producer for recombinant human granulocyte colony-stimulating factor. Front Chem. (2018) 6:304. doi: 10.3389/fchem.2018.00304
32. Li H, Xiong XP, Peng B, Xu CX, Ye MZ, Yang TC, et al. Identification of broad cross-protective immunogens using heterogeneous antiserum-based immunoproteomic approach. J Proteome Res. (2009) 8:4342–9. doi: 10.1021/pr900439j
33. Li H, Ye MZ, Peng B, Wu HK, Xu CX, Xiong XP, et al. Immunoproteomic identification of polyvalent vaccine candidates from Vibrio parahaemolyticus outer membrane proteins. J Proteome Res. (2010) 9:2573–83. doi: 10.1021/pr1000219
34. Malik K, Wu K, Li XQ, Martin-Heller T, Hu M, Foster E, et al. A constitutive gene expression system derived from the tCUP cryptic promoter elements. Theor Appl Genet. (2002) 105:505–14. doi: 10.1007/s00122-002-0926-0
35. Tian L, Wu K, Levasseur C, Ouellet T, Foster E, Latoszek-Green M, et al. Activity of elements from the tobacco cryptic promoter, tCUP, in conifer tissues. In Vitro Cell. Dev. Biol. Plant. (2003) 39:193–202. doi: 10.1079/IVP2002365
36. Haq TA, Mason HS, Clements JD, Arntzen CJ. Oral immunization with a recombinant bacterial antigen produced in transgenic plants. Science. (1995) 268:714–6. doi: 10.1126/science.7732379
37. Tabe LM, Wardley-Richardson T, Ceriotti A, Aryan A, McNabb W, Moore A, et al. A biotechnological approach to improving the nutritive value of alfalfa. J Anim Sci. (1995) 73:2752–9. doi: 10.2527/1995.7392752x
38. Ko K, Tekoah Y, Rudd PM, Harvey DJ, Dwek RA, Spitsin S, et al. Function and glycosylation of plant-derived antiviral monoclonal antibody. Proc Natl Acad Sci USA. (2003) 100:8013–8. doi: 10.1073/pnas.0832472100
39. Park M, Kim SJ, Vitale A, Hwang I. Identification of the protein storage vacuole and protein targeting to the vacuole in leaf cells of three plant species. Plant Physiol. (2004) 134:625–39. doi: 10.1104/pp.103.030635
40. Fujiyama K, Misaki R, Sakai Y, Omasa T, Seki T. Change in glycosylation pattern with extension of endoplasmic reticulum retention signal sequence of mouse antibody produced by suspension-cultured tobacco BY2 cells. J Biosci Bioeng. (2009) 107:165–72. doi: 10.1016/j.jbiosc.2008.09.016
41. Breyne P, Gheysen G, Jacobs A, Van Montagu M, DePicker A. Effect of T-DNA configuration on transgene expression. Mol Gen Genet. (1992) 235:389–96. doi: 10.1007/BF00279385
42. Hobbs SLA, Warkentin TD, De Long CMO. Transgene copy number can be positively or negatively associated with transgene activity. Plant Mol Biol. (1993) 21:17–26. doi: 10.1007/BF00039614
43. Matzke AJ, Matzke MA. Position effects and epigenetic silencing of plant transgenes. Curr Opin Plant Biol. (1998) 1:142–8. doi: 10.1016/S1369-5266(98)80016-2
44. Schrijver RS, Kramps JA. Critical factors affecting the diagnostic reliability of enzyme-linked immunosorbent assay formats. Rev Sci Tech Off Int Epiz. (1998) 17:550–61. doi: 10.20506/rst.17.2.1117
Keywords: vibriosis, Wolffia globosa, LamB, edible vaccine, recombinant protein, oral immunization
Citation: Heenatigala PPM, Sun Z, Yang J, Zhao X and Hou H (2020) Expression of LamB Vaccine Antigen in Wolffia globosa (Duck Weed) Against Fish Vibriosis. Front. Immunol. 11:1857. doi: 10.3389/fimmu.2020.01857
Received: 06 April 2020; Accepted: 10 July 2020;
Published: 20 August 2020.
Edited by:
Wei-Dan Jiang, Sichuan Agricultural University, ChinaReviewed by:
Qihui Yang, Guangdong Ocean University, ChinaCopyright © 2020 Heenatigala, Sun, Yang, Zhao and Hou. This is an open-access article distributed under the terms of the Creative Commons Attribution License (CC BY). The use, distribution or reproduction in other forums is permitted, provided the original author(s) and the copyright owner(s) are credited and that the original publication in this journal is cited, in accordance with accepted academic practice. No use, distribution or reproduction is permitted which does not comply with these terms.
*Correspondence: Hongwei Hou, aG91aHdAaWhiLmFjLmNu
†These authors have contributed equally to this work
Disclaimer: All claims expressed in this article are solely those of the authors and do not necessarily represent those of their affiliated organizations, or those of the publisher, the editors and the reviewers. Any product that may be evaluated in this article or claim that may be made by its manufacturer is not guaranteed or endorsed by the publisher.
Research integrity at Frontiers
Learn more about the work of our research integrity team to safeguard the quality of each article we publish.