- 1Department of Pediatrics, Otto-von-Guericke-University, Magdeburg, Germany
- 2Health Campus Immunology, Infectiology and Inflammation, Otto-von-Guericke-University, Magdeburg, Germany
- 3Department of Otorhinolaryngology, Head and Neck Surgery, Otto-von-Guericke-University, Magdeburg, Germany
- 4Department of Biometry and Medical Informatics, Otto-von-Guericke-University, Magdeburg, Germany
Every sixth child suffers from hypertrophy of the adenoid, a secondary lymphoid organ, at least once in childhood. Little is known about the impact of pathogen-provocation vs. developmental impact on T-cell responses after 1 year of age. Therefore, developmental and infection-driven influences on the formation of T-cell-compartments and -multifunctionality in adenoids were analyzed taking into account patient's history of age and inflammatory processes. Here, we show that in adenoids of 102 infants and children similar frequencies of naïve, effector, and memory T-cells were accumulated, whereby history of suffering from subsequent infection symptoms resulted in lower frequencies of CD4+ and CD8+ T-cells co-expressing several cytokines. While patients suffering from sole nasal obstruction had balanced Th1- and Th17-compartments, Th1 dominated in patients with concomitant upper airway infections. In addition, analysis of cytokine co-expressing CD4+ and CD8+ T-cells showed that children at the age of three or older differed significantly from those being 1- or 2-years old, implicating a developmental switch in T-cell differentiation at that age. Yet, dissecting age and infectious history of the patients revealed that while CD8+ T-cell differentiation seems to be triggered by development, CD4+ T-cell functionality is partly impaired by infections. However, this functionality recovers by the age of 3 years. Thus, 3 years of age seems to be a critical period in an infant's life to develop robust T-cell compartments of higher quality. These findings identify important areas for future research and distinguish an age period in early childhood when to consider adjusting the choice of treatment of infections.
Introduction
Secondary lymphoid organs such as lymph nodes and spleen are important locations for the initiation of T-cell responses against pathogens. Mucosal associated lymphoid tissues that include adenoids are also secondary lymphoid organs and are confronted continuously with pathogenic antigens and function as platforms to generate adaptive immune responses for defense (1, 2). Hypertrophy of the adenoids is common in infants and younger children and predisposes to recurrent infections of the upper airways or otitis media with effusion and impaired hearing (3). Adenoidectomy at an early age often reduces these recurrent infections and restores proper hearing. Yet, even without surgery, children in some cases outgrow the chronic infections and the enlarged adenoids regress spontaneously (3–5). It is well-known that the adaptive immune response of adults and infants differs profoundly, but rather little is known about the impact of pathogen-provocation vs. developmental impact on T-cell responses after 1 year of age (6). T-cell differentiation can be understood as the delineation of T-cells into unique and defined sub-populations with certain functions. Sub-populations of CD4+ T-cells, namely Th1, Th2, Th9, Th17, or of CD8+ T-cells, Tc1, Tc2, and Tc17, are mainly defined by their cytokine production (1, 7). For neonates and infants, a Th2 bias has been suggested, but was not visible upon inflammation and pathogen confrontation (2, 8, 9). In addition, fetal and neonatal T-cells express enhanced the signatory cytokines IL-8 or IL-17, yet also IL-10 has been reported (8, 10, 11). That this signatory cytokine production is not as straight forward in infants and children, as no enhanced IL-8 or IL-17 expression was detectable in a cohort of 1 to 5 year old children at different mucosal sites (12). Although Th17 cells are discussed to be essential in children for mucocutaneous immunity especially against Candida and Staphylococcus in the respiratory tract and on the skin, and Th1 cells for protection against intracellular antigens, a balance of both seems to be optimal (13). In terms of CD8+ T-cell responses, they are suggested to not be generated properly before the age of two (14, 15). Nevertheless, other associated studies suggest that infections, treatment with antibiotics as well as individual variations in immune development during childhood have long-term consequences to the prevalence of many diseases (16–18). However, detailed knowledge of molecular and cellular mechanisms is missing to fully understand T-cell responses early in life.
A strategy for the evaluation of CD4+ and CD8+ T-cell functionality is based on the co-expression of different cytokines by individual T-cells, which provides them with different effector functions as well as cytokine-mediated expansion (19, 20). In general, these multifunctional T-cells are strongly associated with protection against pathogen-threat and fast clearance of infections. Consequently, vaccines are thought to improve the protection against infections mainly through the formation of multifunctional T-cells (21–23). However, the capability of infants to form substantial amounts of multifunctional T-cells is still not clear.
In the present study, adenoids were chosen to analyze the formation of cytokine co-expressing T-cells, which served as an indication for the ability of the organism to generate complex adaptive immune responses. Circumstances of acute and chronic infections as possible driving forces for the formation of T-cell differentiation were compared with developmental changes with age within the general development of children's immune system.
Methods
Study Design
The study was approved by the Clinical Research Ethics Board of the University of Magdeburg (N° 06/11) and all patient parents provided written informed consent at the time of enrollment in accordance with the Declaration of Helsinki. One hundred and two children aged between 1 and 11 years suffering from adenoid hypertrophy who underwent adenoidectomy at the Department of Otorhinolaryngology, Head & Neck Surgery at the University Hospital of Magdeburg were included in the study. Children suffering from immune or genetic diseases were excluded. According to predominant clinical symptoms of the children at the time of surgery (Table 1), three groups were compared, two infection groups with either recurrent infections of the upper airways or concomitant otitis media with effusion and one none-infection group presenting with nasal obstruction only.
Analysis of Immune Competence
Mononuclear cell (MC) suspensions were generated from adenoids in PBS-BSA by mechanical dissociation over a 70-μm cell strainer (BD Biosciences, San Jose, CA) (2). 1–5 x 107 MCs were cryopreserved at −80°C in 1 ml DMSO and FCS in a ratio 1:10. For cell culture MCs were thawed, washed, resuspended in x-vivo-15 media (Lonza, Belgium) and rested overnight at 37°C. 5 x 106 MCs/ml were stimulated with 10 ng/ml PMA and 1 μg/ml Ionomycin in the presence of 5 μg/ml Brefeldin A (Sigma-Aldrich, St. Louis, MO) for 6 hr. Unstimulated cells were used for phenotyping of CD4+ and CD8+ T-cell subsets. For intracellular analysis, cells were fixed in 2% paraformaldehyde (Morphisto) and permeabilized with 0.5% saponin (Sigma) (24). Cytometric data were acquired on a FACS-Canto II (BD) and analyzed with FlowJo (FlowJo LLC) software. The following fluorochrome-conjugated Abs were used for flow cytometry: anti-CD3 (SK7), anti-CD45 (H130), anti-CD4 (RPA-T4), anti-CD8 (RPA-T8), anti-CD31, anti-CD45RO (UCHL1), anti-CD45RA, and anti-CD27, anti-IL-2 (MQ1-17H12), anti-TNFα (Mab11), anti-IL-17 (eBio64DEC17) and anti-IFNγ. The gating strategy for cytokine expression and phenotyping of CD4+ and CD8+ T-cell subsets are shown in the supplemental (Figures S1, S2). T-cell subpopulations showing single, double, triple and quadruple cytokine producers were analyzed by applying Boolean gating on either CD3/CD4 or CD3/CD8 T-cells.
Statistical Analysis
Statistical analyzes were performed using GraphPad Prism6 and SPSS22. Normal distributions of variables were checked with a Kolmogorov-Smirnoff test. For pairwise comparisons, either Student t-test or non-parametric Mann-Whitney test was used depending on Gaussian distribution. For multiple comparisons, Kruskal-Wallis method with post-hoc Dunn multiple comparison or one-way ANOVA with a Bonferroni test were used. Furthermore, two-way-ANOVA multiple comparisons in conjunction with a Tukey HSD (honest-significance-difference) test or Spearman correlations were performed as indicated. Statistical significance was considered for p < 0.05. *p < 0.05, **p < 0.01, ***p < 0.001, ****p < 0.0001. Results are expressed as mean ± standard error of mean (SEM).
Results
Substantial Amounts of Effector and Memory T-Cells Persist in Pediatric Adenoids
To identify characteristics of T-cell differentiation, a cohort of 102 pediatric patients aged 1–11 years undergoing adenoidectomy because of hypertrophy were analyzed as a model for inflammation-driven or developmental driven forces on T-cell functionality. Clinical history of patients was exploited to be able to group patients according to previous suffering from acute or chronic infections, namely concomitant otitis media with effusion (group 3, OME) or recurrent infections of the upper airways (group 2, UAI). Another group suffering only from nasal obstruction was considered to represent the non-infectious group (group 1, NO). An ex vivo analysis of single cell suspensions of adenoids was performed using flow cytometric immunotyping of CD4+ and CD8+ T-cells (Figures 1, 2, and Figures S1, S2). Accumulation of recent thymic emigrants (RTE), considered as CD4+CD45RA+CD31+ and CD8+CD45RA+CD27+ T-cells, were similar in the three patient groups, and thus identified to be independent of infection history (Figure 1A). In terms of antigen-experienced T-cells, similar frequencies of effector (CD4+CD45RO+CD27− and CD8+CD45RA+CD27−) and memory (CD4+CD45RO+CD27+ or CD8+CD45RO+CD27+) T-cells were observed within the three disease groups suggesting that the size of these compartments is independent of inflammation caused by infections. Surprisingly, even in the non-inflammatory group 1, infants and children showed similar frequencies of memory T-cell populations as patients with infections (Figure 1A) (14). Of note, the naïve and effector CD8+ T-cell compartments of all adenoids analyzed were much larger than the one of CD4+ T-cells (Figure 1A). However, the CD4+ T-cell population was comprised of a notably larger memory compartment than that of the CD8+ one (Figure 1A).
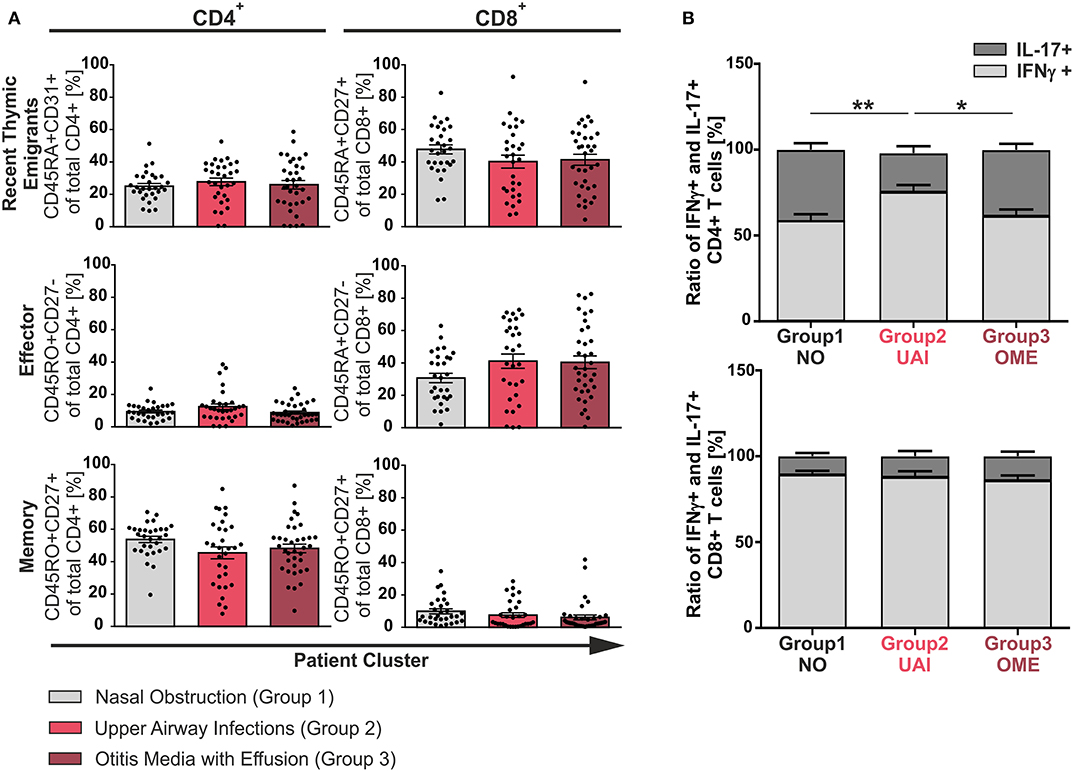
Figure 1. Phenotype of CD4+ and CD8+ T-cells among the three clinical groups. Mononuclear cells (MC) were isolated from adenoids of 102 children suffering from adenoid hypertrophy that underwent adenoidectomy. MCs of children with sole nasal obstruction (group 1), with those suffering from recurrent infections of the upper airways (group 2), or with concomitant otitis media with effusion (group 3) were either left unstimulated for phenotyping or stimulated for analysis of cytokine production with PMA and Ionomycin in the presence of Brefeldin A for 6 h. Surface marker or cytokine expression of CD4+ and CD8+ T-cells was determined by using flow cytometry. (A) Frequencies of naive/recent thymic emigrants, effector, and memory T-cells were compared depending on predominant clinical symptoms, each shown for total CD4+ (left side) and CD8+ (right side) T-cells. Children suffering from sole nasal obstruction (group 1, light gray bars), suffering from recurrent upper airway obstructions (group 2, red bars), or suffering from concomitant otitis media with effusion (group 3, dark red bars) are depicted. Each dot represents an individual patient out of n = 102. Horizontal lines indicate mean ± SEM (one-way ANOVA, Tukey HSD multiple comparisons). (B) The percentages of total IFNγ+ (light gray) or IL-17+ (dark gray) CD4+ (upper panel) and CD8+ (lower panel) T-cells were determined, calculated to hundred percent, and presented in a comparative manner. Kolmogorov-Smirnoff test as well as a Kruskal-Wallis method with post-hoc Dunn multiple comparison were used. Horizontal lines indicate mean ± SEM. *p < 0.05, **p < 0.01.
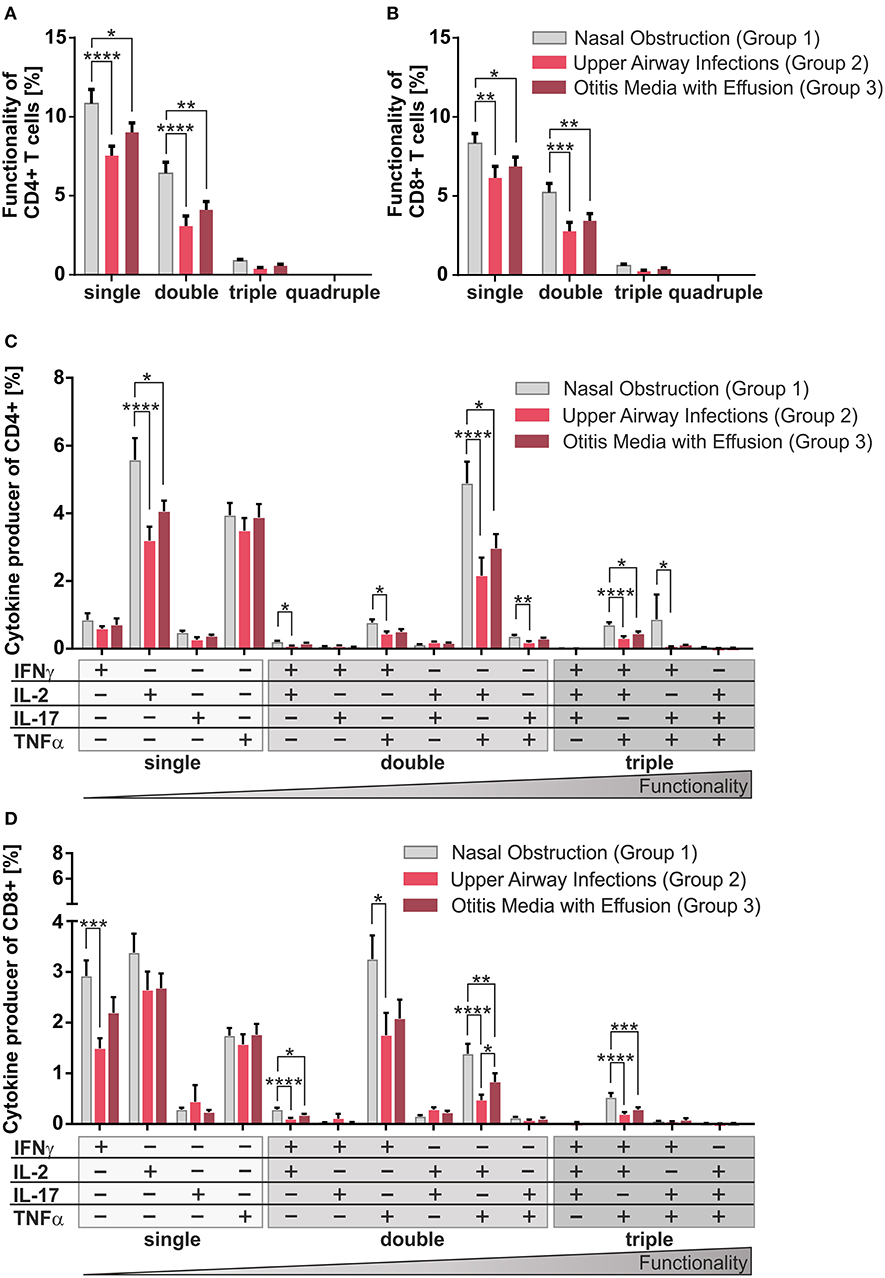
Figure 2. Multifunctionality of CD4+ and CD8+ T-cells among the three clinical groups. MCs of pediatric adenoids were stimulated as described in Figure 1. Intracellular cytokine expression of CD4+ and CD8+ T-cells was determined by using flow cytometry and the Boolean gating strategy. (A,B) Frequency of summarized CD4+ and CD8+ subpopulations (summarized subsets that express just one (single), or simultaneously two (double), three (triple), or four (quadruple) different cytokines) among all CD4+ (A) and CD8+ (B) T-cells. (C,D) Frequencies of individual CD4+ and CD8+ subpopulations (+ indicates the expressed cytokine) among all CD4+ (C) and CD8+ (D) T-cells. Light gray bars represent children suffering from sole upper airway obstruction (group 1), whereas red bars represent children additionally suffering from recurrent upper airway infections (group 2), and dark red bars stand for children with concomitant otitis media with effusion (group 3). The triangle symbolizes that the functionality of the T-cells increases with the number of cytokines produced simultaneously (two-way ANOVA, Tukey HSD multiple comparisons) *p < 0.05, **p < 0.01, ***p < 0.001, ****p < 0.0001.
Reduced Frequencies of Multifunctional T-Cells in Children With Infectious Complications
Having found the size of CD4+ and CD8+ compartments of memory and effector T-cells to be independent of infection history, differentiation was monitored in our model using cytokine production as surrogate marker (Figures 1B, 2). Even though there was a balance of IFNγ and IL-17 producers in the CD4+ compartment as needed to effectively fight infections (Figure 1B, upper panel), frequencies of Th17 cells clearly dropped in half by patients suffering from upper airway inflammations (group 2). In the CD8+ compartment Tc1 cells dominated independent of infections (Figure 1B, lower panel).
Single, double, and triple cytokine producers of CD4+ and CD8+ T-cells were monitored in all disease groups (Figures 2A,B). Single and double producers dominated the response in both compartments and were significantly increased in children with sole nasal obstruction (group 1) compared to the other two groups; quadruple producers were hardly visible. As triple producers of TNFα, IL-2, plus IFNγ are known to be strongly associated with pathogen clearance (21), special attention was payed to them by dissecting triple producers into individual functional T-cell subsets (Figures 2C,D). Children with sole nasal obstruction (group 1) showed significantly more of those Th1-like and Tc1-like triple producers than those in the two infection-biased clinical groups (Figures 2C,D). As Th17 and Tc17 cells are known to show high plasticity to acquire the ability to co-express the Th1-lineage cytokine IFNγ (25), IL-17 producing triple producers were also monitored. Along with the before-mentioned triple-producers, the IFNγ/IL-17 co-expressing population was mainly detected together with TNFα co-expression in CD4+ T-cells of patients without infectious history (Figure 2C).
Overall, similarly for the CD4+ (Figures 2A,C) and the CD8+ compartments (Figures 2B,D), patients from the non-infectious group 1 differed profoundly from the other two groups, showing enhanced frequencies of co-producers, thus functionally better equipped T-cells (21).
Effector and Memory T-Cell Compartments Are Overall Similar in Adenoids of Infants and Children
As multiple infections were not leading to enhanced differentiation/accumulation of multifunctional T-cells (Figure 2), we asked whether the age of the patients could actually be decisive. Therefore, the patients were grouped by age and the T-cell compartments were then analyzed accordingly. Results demonstrated that frequencies of CD8+ RTE and effector T-cells did not show any differences between age groups. In the CD4+ compartment, only the frequency of RTE but neither of effector nor of memory cells showed a significant dependency on age (Figure 3A). This also demonstrates that already 1 year old infants generate substantial amounts of memory CD4+ T-cells. When comparing children of 3 years and older with 2-year olds, the former were seen to have about twice as many CD8+ memory T-cells although at very low frequencies (p < 0.01) (Figure 3A).
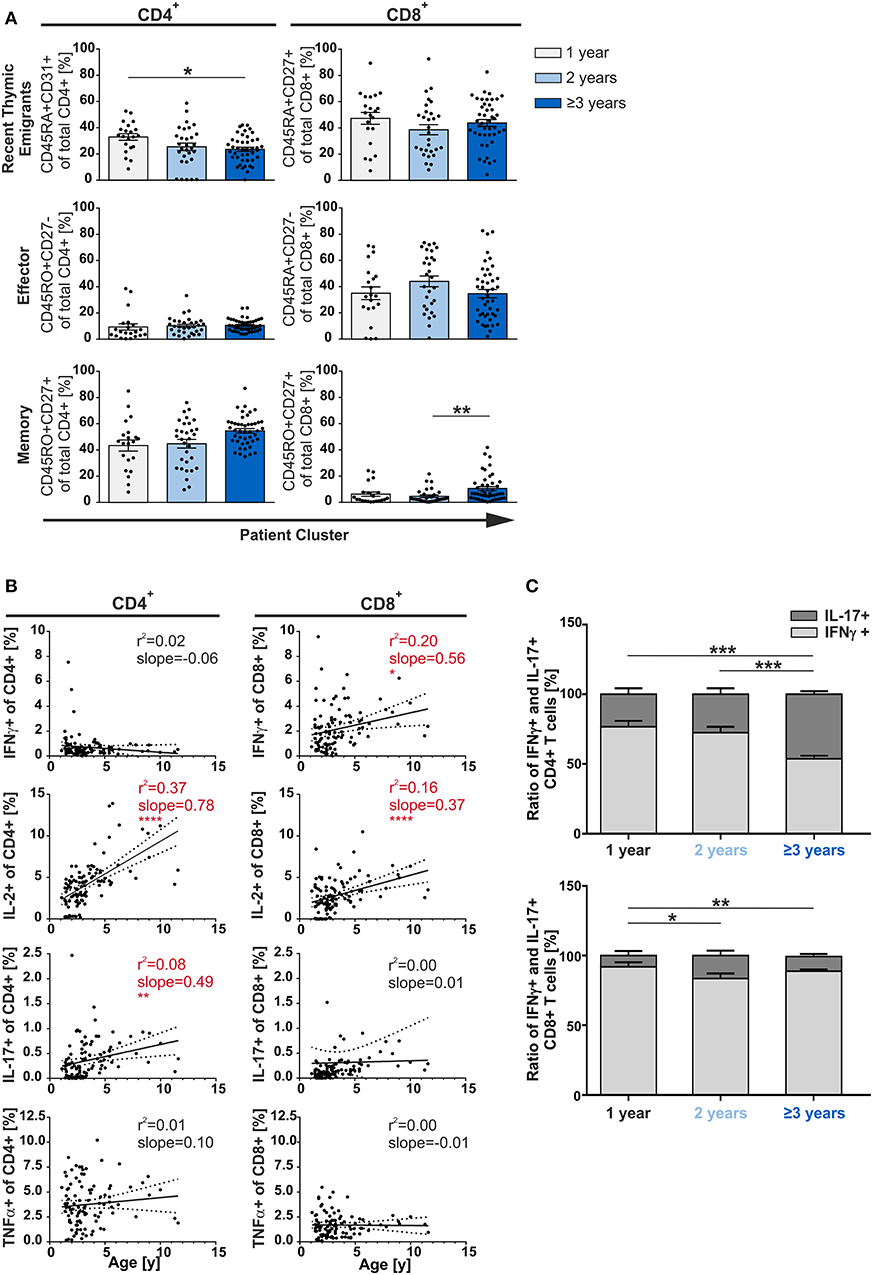
Figure 3. Phenotype of CD4+ and CD8+ T-cells depending on age. MCs of adenoids of children being 1, 2, or 3 years and older with adenoidectomy were stimulated and analyzed as in Figure 1. (A) Frequencies of naive/recent thymic emigrants, effector, and memory T-cells are compared depending on age at the time of surgery, each shown for total CD4+ (left side) and CD8+ (right side) T-cells. One-year-old (light gray bars), 2-year-old (light blue bars), and 3-year-old and older (dark blue bars) infants and children are depicted. Each dot represents an individual patient from n = 102 infants and children included in the study. Horizontal lines indicate mean ± SEM (one-way ANOVA, Tukey HSD multiple comparison). *p < 0.05, **p < 0.01. (B) Line graphs represent linear regression with 95% confidence intervals among CD4+ and CD8+ T-cells. Functional populations for single producers for each individual cytokine analyzed within participants by advancing age (1–11 years). Line graph statistics were analyzed by Spearman r correlation (*p < 0.05, **p < 0.01, ****p < 0.0001). (C) The percentages of total IFNγ+ (light gray) or IL-17+ (dark gray) CD4+ (upper panel) and CD8+ (lower panel) T-cells were determined, calculated to hundred percent, and presented in a comparative manner. Kolmogorov-Smirnoff test as well as a Kruskal-Wallis method with post-hoc Dunn multiple comparison were used. Horizontal lines indicate mean ± SEM. *p < 0.05, **p < 0.01, ***p < 0.001.
Next, we analyzed the single cytokine producing cells in terms of aging during early childhood. Among all CD4+ and CD8+ T-cells, single-producers increase steadily and significantly (slopeCD4 1.44; slopeCD8 0.56; data not shown). This increase was mainly due to the CD4+ IL-2 and IL-17 and the CD8+ IFNγ and IL-2 single producers (Figure 3B). Besides age differences, CD4+ single TNFα and single IFNγ producers as well as CD8+ single IL-17 and single TNFα producers were generated at equal frequencies.
As IL-17 was described as a signatory cytokine in fetal and neonatal T-cells (26), we further focused on Th17 cells. Unexpectedly, Th1-like cells were found to be more abundant than Th17-like cells in younger infants (1- and 2-year-olds) while frequencies of Th17 cells significantly rose with age in adenoids of 1 to 11-year olds (Figures 3B,C). Indeed, older children (≥3 years) showed 50% (p < 0.001) more IL-17 producing CD4+ T-cells than those being 1 or 2-years old, leading to a Th1:Th17 balance in the CD4+ compartment (Figure 3C). The CD8+ compartment was always dominated by Tc1 when compared to Tc17 cells, with a significant doubling of the frequency of Tc17 cells from the age of 2 years—albeit in low frequency (Figure 3C).
Frequencies of Multifunctional CD4+ and CD8+ T-Cells Are Increased From the Age of 3 Years Onward
Next, to investigate the age-dependency on functionality of CD4+ and CD8+ T-cells in adenoids, we analyzed individual cytokine co-expressing T-cells in 1, 2, and 3 years and older children (Figure 4). Summarizing the cytokine producers within the CD4+ and CD8+ compartments as single, double-, triple-, and quadruple-producers, respectively, a significant increase of single- and double-producers was observed in the oldest group compared to the 1- and 2-year-old infants (Figures 4A,B). These data show a clear difference becoming visible in the functionality of T-cells during and after the third year of life.
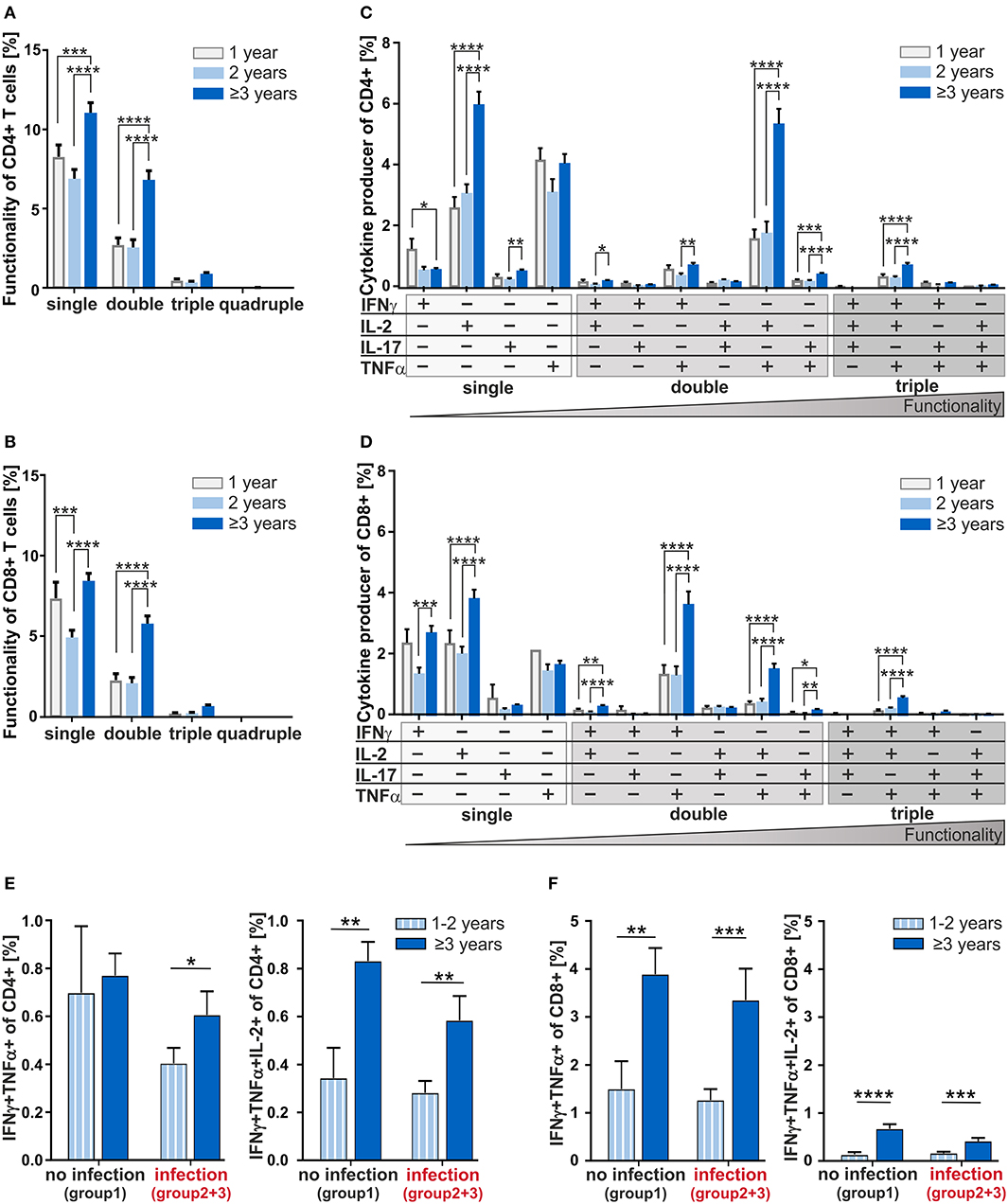
Figure 4. Multifunctionality of CD4+ and CD8+ T-cells depending on age and cytokine co-expression profiles depending on the infection status. MCs of adenoids from children being 1, 2, or 3 years and older with adenoidectomy were stimulated as in Figure 1 and analyzed as in Figure 2. (A,B) Frequencies of summarized CD4+ and CD8+ subpopulations (summarized subsets that express just one (single), or simultaneously two (double), three (triple), or four (quadruple) different cytokines) among all CD4+ (A) and CD8+ (B) T-cells. (C,D) Frequencies of individual CD4+ and CD8+ subpopulations (+ indicates the expressed cytokine) among all CD4+ (C) and CD8+ (D) T-cells. Children being 1 year old (light gray bars) at the time of surgery, children being 2 years old (light blue bars), and children being 3 years and older (dark blue bars) are depicted. The triangle symbolizes that the functionality of the T-cells increases with the number of cytokines produced simultaneously (two-way ANOVA, Tukey HSD multiple comparisons). *p < 0.05, **p < 0.01, ***p < 0.001, ****p < 0.0001. (E,F) Frequencies of IFNγ and TNFα double positive CD4+ (E) and CD8+ (F) T-cells in children being 1 and 2 years of age or 3 years and older either with (summarized group 2 and 3) or without (group 1) infections are shown on the left, while frequencies of IFNγ, TNFα, and IL-2 triple positive CD4+ (E) and CD8+ (F) T-cells are shown on the right. White and light blue bars represent children being 1 and 2 years of age whereas dark blue bars represent children of 3 years and older at the time of surgery. Horizontal lines indicate mean ± SEM (Kolmogorov-Smirnoff test; Kruskal-Wallis method with post-hoc Dunn multiple comparison). *p < 0.05, **p < 0.01, ***p < 0.001, ****p < 0.0001.
Analyzing CD4+ double producers, IL-2+TNFα+ co-producers accounted for the largest subpopulation and children being 3 years and older accounted for 70% more IL-2+TNFα+ co-producers than 1- and 2-year-olds (Figure 4C). Indeed, differences could be seen between children of 3 and those being 2 years of age for most of the double producers analyzed, i.e., IFNγ co-producers with IL-2+or TNFα+ as well as for IL-17+TNFα+ co-producers.
Overall, the functionality of CD4+ T-cells determined by co-expression of IFNγ, IL-2, IL-17, and TNFα increased significantly with age (Figures 4A,C). For most of the individual subpopulations according to cytokine expression, functional capacity of T-cells in 1- and 2-year-old children were hardly distinguishable. The IFNγ+IL-2+TNFα+ co-expressing CD4+ and CD8+ T-cells which are known to correlate with pathogen clearance (21), were shown at a significantly higher frequency in children being 3 years and older compared to those of one and 2 years of age (Figures 4C,D). Concerning these triple-positive T-cells in the CD8+ compartment, children of at least 3 years of age accounted for 77% more (p < 0.0001) than children of one and 65% more (p < 0.0001) than children of 2 years of age (Figure 4D). Thus, 3-years- and older children who display significantly higher frequencies of double- and triple-cytokine co-producing T-cells show increased T-cell functionality.
Taken together, with increasing age, children can be described to harbor functionally more complex CD4+ and CD8+ T-cells such as double- and triple-producers. For younger children, a higher amount of functionally less equipped T-cells dominate.
Developmental Impact on the Functionality of CD4+ and CD8+ T-Cells
At first glance, the ability to co-express cytokines simultaneously seemed to be based on a non-inflammatory and development-specific process. In order to separate the influence of development and infection on the generation of cytokine co-expressing T-cells, we next analyzed the age dependency of the generation of these co-expressing CD4+ and CD8+ T-cells taking into consideration the infection history (Figures 4E,F). In the none-infection group of infants and children, the CD4+IFNγ+TNFα+ double producers were identified to be similar across different ages (Figure 4E, left). However, focusing on children with infection history, 1- and 2-year-olds display dramatically reduced frequencies of these double producers. Data also show that children from the age of three show an increase in the double producers despite infections. Having a look at the CD4+IFNγ+TNFa+IL-2+ triple producers, a drastic increase with age was visible independent of infection history (Figure 4E, right). Clearly, CD8+IFNγ+TNFα+ double producers and albeit in lower frequencies CD8+IFNγ+TNFa+IL-2+ triple producers increased considerably with 3 years of age, regardless of any acute or chronic infection in earlier life (Figure 4F). Consequently, while infections seem to be responsible for reduced frequencies of IFNγ+TNFα+ co-expressing CD4+ T-cells in the first 2 years of life, an increase of triple producers is merely due to development. This contrasts with the CD8+ T-cells, whose increase of any kind of cytokine co-producers is determined exclusively by age—thus, purely developmental.
Discussion
To better understand the functionality of pediatric T-cell responses, T-cell functionality was studied in 102 children suffering from adenoid hypertrophy, either in combination with acute or with chronic infections or none.
Here, we show that in a secondary lymphoid organ, the adenoid, a consistently similar frequency of naïve, effector, and memory T-cells is maintained in patients aged 1–11 years, with no general impact of infection history or age (Figures 1A, 3A). In blood, a steady increase in circulating memory T-cells and decrease in naïve T cells is discussed (6, 27, 28). However, the consistency of T-cell compartment sizes in adenoids suggests functional significance. Herein, memory CD4+ T-cells were the predominant population in adenoids starting at 1 year of age, followed by recent thymic emigrants, while the latter comprised the largest proportion of CD8+ T-cells. For other tissues and blood in infants it was reported that local T-cell compositions are mainly made up of naive T-cells (12, 29). The local accumulation of a high proportion of CD4+ memory T-cells especially in adenoids early in life likely reflects the requirement of immediate defense to the recurrent exposure to pathogens. The CD8+ compartment in the data consistently displayed low frequencies of memory cells in comparison to effector and naïve cells. This is in line with previous findings, which report that CD8+ T-cells do not easily generate memory and the same may apply to at least this pediatric relevant period or site (30).
Direct killing of infected cells as part of the protection against pathogens might explain the large proportion of CD8+ effector T-cells compared to CD8+ memory T-cells in the adenoids. Mechanistically, either the challenging environment or cell-intrinsic mechanisms support a preferred differentiation in effector T-cells rather than into memory T-cells. Effector T-cells are advantageous because they react faster than memory CD8+ T-cells (31). However, if the enhanced proportion of CD8+ effector T-cells in children is caused by the hypothesized layered immune system at birth, meaning that the T-cell differentiation based on different progenitor cells still exists in parallel, this needs to be further elaborated (30).
Although frequencies of effector and memory T-cells do not change dramatically in adenoids, a continuous change in the TCR repertoire can be expected, as colonization of mucosal sites increases diversity within the first 3 years of life when the phylogenetic composition of the bacterial communities evolve toward an adult-like configuration (12, 32).
Even though patients with sole nasal obstruction could be expected to suffer from infections for organic and steric reasons alone, our results would not support such a scenario. As especially those patients in general generate higher frequencies of cytokine co-producers than those in the other two groups with acute and chronic infectious complications, and they can be interpreted as having a better disease-control (Figure 2). This is in line with many studies showing that these multifunctional T-cells are needed to clear infections and are superior to single-producers (20, 33–36). Indeed, this T-cell profile of higher quality seems to be protective against infectious complications in children with enlarged adenoids (Figure 2). These results are also supported by vaccination studies showing that vaccines eliciting multifunctional CD4+ or CD8+ T-cells ensure better protection against viral infections, e.g., a significant increase in multifunctional T-cells following repeated annual influenza vaccination was recently reported (21–23). Furthermore, HPV-vaccines were illustrated to enhance the control of HPV-associated tumors by activation of multifunctional CD8+ T-cells (37). This further strengthens our data that multifunctionality of T-cells enhances protection also in pediatric patients and leads to a better control of pathogen-provocation.
While CD4+ T-cells of patients with upper airway infections showed a Th1 dominance, the group with only nasal obstruction showed an optimal Th1/Th17 ratio of one (Figure 1B). This is in agreement with the report that a balanced ratio of Th1 and Th17 cells in adenoids is advantageous (13). Nevertheless, patients with acute infection (Figure 1B, group 3), also show a balance of Th1/Th17 ratio and it is tempting to speculate that it may still be beneficial to prevent chronicity. Overall, the reported IL-17-bias of fetal and neonatal cells does not account for T-cells in early childhood (2, 38). The disappearance of the fetal/neonatal-monitored Th17-bias could be explained by the high plasticity of Th17 cells to convert to Th1-like cells (25), or by a different origin of the responding cells (30). Indeed, absence of Th17 dominance points toward a replacement of the suggested neonatal precursor cells postpartum rather than to the co-existence of a layered immune system (13).
Age strongly appears to influence the magnitude and quality of the T-cell responses and being 3 years or older seems to mark a turning point in regard to T-cell quality. Knowledge about the effect of age on multifunctionality of T-cells in secondary lymphoid organs is scarce (2) and to our knowledge, so far, no ex vivo data from secondary lymphoid organs are available from multifunctionality of T-cells in a pediatric cohort as young as ours. However, age effects on T-cell multifunctionality in older children (11–17 years) was suggested in a small study using circulating T-cells (34). In adulthood, frequency of cytokine co-expressing T-cells is stable (39, 40).
In the CD8+ compartment, the generation of multifunctionality demonstrates especially a clear-cut increase from 3 years of age with a highly significant impact of age, but not of infections (Figure 4F). However, a switch from low frequencies of multifunctional as well as memory CD8+ T-cells early in life could point toward missing differentiation-supporting structures within the secondary lymphoid structure. Indeed, also other subpopulations of lymphocytes that are dependent on secondary lymphoid structures such as mature marginal zone B cells, start to accumulate in substantial amounts not before 2 years of age (41, 42). Thus, even after 1 year of life, functionality analysis of T-cells in adenoids demonstrates that the development of the immune system still follows a developmental trajectory (43). Hence, the influence of developmental processes on immune functioning throughout life span could be far greater than that is apparent at first glance (44, 45).
Importantly, in comparison to the CD8+ compartment the CD4+ compartment behaves differently in the way that age and infections impact the formation of cytokine co-producers (Figure 4E). Whereas, IFNγ+TNFα+IL-2+ triple producers are similar in infectious and non-infectious groups before the age of three, IFNγ+TNFα+ double producers differ profoundly. This implicates that filling the compartment of triple producers during infections might happen on the expense of double producers. As these triple producers are more prone to enter the memory pool, this might be of ontogenetic significance (21). Therefore, the ability to generate more complex T-cell-responses seems to be an internal mechanism within the development of a child's immune system.
In conclusion, the comparison of these young age groups according to cytokine co-expression and clinical symptoms might help physicians designing conditioning protocols adapted to age, taking into account the better control of infections and a fully equipped immune system already after 3 years of age. These findings also outline from an immunological point of view, that it is important to consider age and acute or chronic infection history when deciding upon clinical procedures, thus, the importance of adapting indications and protocols according to expected immune fitness.
Data Availability Statement
All datasets presented in this study are included in the article/Supplementary Material.
Ethics Statement
The studies involving human participants were reviewed and approved by Clinical Research Ethics Board of the University of Magdeburg (No. 06/11). Written informed consent to participate in this study was provided by the participants' legal guardian/next of kin. Written informed consent was obtained from the minor(s)' legal guardian/next of kin for the publication of any potentially identifiable images or data included in this article.
Author Contributions
MB-W and GJ designed the study. MB-W, KL, GJ, and CA participated in the interpretation of the findings, read, and critically revised the manuscript. JK contributed to the design of the work, performed experiments, interpreted the findings, and wrote the manuscript together with MB-W and MP. KH participated in data analysis and revised the manuscript. MP participated in data analysis and data interpretation and wrote the manuscript. SK has made the statistic. All co-authors read the manuscript and have provided important intellectual input to the manuscript. The corresponding author had full access to the data and had final responsibility for the decision to submit for publication.
Funding
This work was supported by DFG Br 1860/12-1 and SFB854 B14.
Conflict of Interest
The authors declare that the research was conducted in the absence of any commercial or financial relationships that could be construed as a potential conflict of interest.
Acknowledgments
We thank K. Kramer and B. Kramer for excellent technical assistance and Aditya Arra for critically reading of the manuscript.
Supplementary Material
The Supplementary Material for this article can be found online at: https://www.frontiersin.org/articles/10.3389/fimmu.2020.01640/full#supplementary-material
References
1. Moulton VR, Farber DL. Committed to memory: lineage choices for activated T cells. Trends Immunol. (2006) 27:261–7. doi: 10.1016/j.it.2006.04.006
2. Vogel K, Pierau M, Arra A, Lampe K, Schlueter D, Arens C, et al. Developmental induction of human T-cell responses against Candida albicans and Aspergillus fumigatus. Sci Rep. (2018) 8:16904. doi: 10.1038/s41598-018-35161-5
3. van den Aardweg MT, Schilder AG, Herkert E, Boonacker CW, Rovers MM. Adenoidectomy for recurrent or chronic nasal symptoms in children. Cochrane Database Syst Rev. (2010) 328:487. doi: 10.1002/14651858.CD008282
4. van den Aardweg MT, Schilder AG, Herkert E, Boonacker CW, Rovers MM. Adenoidectomy for otitis media in children. Cochrane Database Syst Rev. (2010) 300:1551. doi: 10.1002/14651858.CD007810.pub2
5. Ingram DG, Friedman NR. Toward adenotonsillectomy in children: a review for the general pediatrician. JAMA Pediatr. (2015) 169:1155–61. doi: 10.1001/jamapediatrics.2015.2016
6. Schmiedeberg K, Krause H, Röhl F-W, Hartig R, Jorch G, Brunner-Weinzierl MC. T cells of infants are mature, but hyporeactive due to limited Ca2+ influx. PLoS ONE. (2016) 11:e0166633. doi: 10.1371/journal.pone.0166633
7. Wu C-Y, Kirman JR, Rotte MJ, Davey DF, Perfetto SP, Rhee EG, et al. Distinct lineages of T(H)1 cells have differential capacities for memory cell generation in vivo. Nat Immunol. (2002) 3:852–8. doi: 10.1038/ni832
8. Hebel K, Rudolph M, Kosak B, Chang H-D, Butzmann J, Brunner-Weinzierl MC. IL-1β and TGF-β act antagonistically in induction and differentially in propagation of human proinflammatory precursor CD4+ T cells. J Immunol. (2011) 187:5627–35. doi: 10.4049/jimmunol.1003998
9. Halonen M, Lohman IC, Stern DA, Spangenberg A, Anderson D, Mobley S, et al. Th1/Th2 patterns and balance in cytokine production in the parents and infants of a large birth cohort. J Immunol. (2009) 182:3285–93. doi: 10.4049/jimmunol.0711996
10. Gibbons D, Fleming P, Virasami A, Michel M-L, Sebire NJ, Costeloe K, et al. Interleukin-8 (CXCL8) production is a signatory T cell effector function of human newborn infants. Nat Med. (2014) 20:1206–10. doi: 10.1038/nm.3670
11. Vanden Eijnden S, Goriely S, Wit D de, Willems F, Goldman M. IL-23 up-regulates IL-10 and induces IL-17 synthesis by polyclonally activated naive T cells in human. Eur J Immunol. (2005) 35:469–75. doi: 10.1002/eji.200425677
12. Thome JJ, Bickham KL, Ohmura Y, Kubota M, Matsuoka N, Gordon C, et al. Early-life compartmentalization of human T cell differentiation and regulatory function in mucosal and lymphoid tissues. Nat Med. (2016) 22:72–7. doi: 10.1038/nm.4008
13. Lévy R, Okada S, Béziat V, Moriya K, Liu C, Chai LY, et al. Genetic, immunological, and clinical features of patients with bacterial and fungal infections due to inherited IL-17RA deficiency. Proc Natl Acad Sci USA. (2016) 113:E8277–85. doi: 10.1073/pnas.1618300114
14. Galindo-Albarrán AO, López-Portales OH, Gutiérrez-Reyna DY, Rodríguez-Jorge O, Sánchez-Villanueva JA, Ramírez-Pliego O, et al. CD8+ T cells from human neonates are biased toward an innate immune response. Cell Rep. (2016) 17:2151–60. doi: 10.1016/j.celrep.2016.10.056
15. Fike AJ, Kumova OK, Carey AJ. Dissecting the defects in the neonatal CD8+ T-cell response. J Leukoc Biol. (2019) 106:1051–61. doi: 10.1002/JLB.5RU0319-105R
16. Daley D. The evolution of the hygiene hypothesis: the role of early-life exposures to viruses and microbes and their relationship to asthma and allergic diseases. Curr Opin Allergy Clin Immunol. (2014) 14:390–6. doi: 10.1097/ACI.0000000000000101
17. Stiemsma LT, Reynolds LA, Turvey SE, Finlay BB. The hygiene hypothesis: current perspectives and future therapies. Immunotargets Ther. (2015) 4:143–57. doi: 10.2147/ITT.S61528
18. Bailey LC, Forrest CB, Zhang P, Richards TM, Livshits A, DeRusso PA. Association of antibiotics in infancy with early childhood obesity. JAMA Pediatr. (2014) 168:1063–9. doi: 10.1001/jamapediatrics.2014.1539
19. Betts MR, Nason MC, West SM, de Rosa SC, Migueles SA, Abraham J, et al. HIV nonprogressors preferentially maintain highly functional HIV-specific CD8+ T cells. Blood. (2006) 107:4781–9. doi: 10.1182/blood-2005-12-4818
20. Kannanganat S, Kapogiannis BG, Ibegbu C, Chennareddi L, Goepfert P, Robinson HL, et al. Human immunodeficiency virus type 1 controllers but not noncontrollers maintain CD4 T cells coexpressing three cytokines. J Virol. (2007) 81:12071–6. doi: 10.1128/JVI.01261-07
21. Seder RA, Darrah PA, Roederer M. T-cell quality in memory and protection: implications for vaccine design. Nat Rev Immunol. (2008) 8:247–58. doi: 10.1038/nri2274
22. de Rosa SC, Lu FX, Yu J, Perfetto SP, Falloon J, Moser S, et al. Vaccination in humans generates broad T cell cytokine responses. J Immunol. (2004) 173:5372–80. doi: 10.4049/jimmunol.173.9.5372
23. Trieu M-C, Zhou F, Lartey SL, Sridhar S, Mjaaland S, Cox RJ. Augmented CD4+ T-cell and humoral responses after repeated annual influenza vaccination with the same vaccine component A/H1N1pdm09 over 5 years. NPJ Vaccines. (2018) 3:37. doi: 10.1038/s41541-018-0069-1
24. Pierau M, Lingel H, Vogel K, Arra A, Brunner-Weinzierl MC. CTLA-4-competent conventional T cells back up regulatory T cells to restrain memory T-helper type 2 cell responses. Allergy. (2019) 75:684–7. doi: 10.1111/all.14042
25. Arra A, Lingel H, Kuropka B, Pick J, Schnoeder T, Fischer T, et al. The differentiation and plasticity of Tc17 cells are regulated by CTLA-4-mediated effects on STATs. Oncoimmunology. (2017) 6:e1273300. doi: 10.1080/2162402X.2016.1273300
26. Lawrence SM, Ruoss JL, Wynn JL. IL-17 in neonatal health and disease. Am J Reprod Immunol. (2018) 79:e12800. doi: 10.1111/aji.12800
27. Saule P, Trauet J, Dutriez V, Lekeux V, Dessaint J-P, Labalette M. Accumulation of memory T cells from childhood to old age: central and effector memory cells in CD4(+) versus effector memory and terminally differentiated memory cells in CD8(+) compartment. Mech Ageing Dev. (2006) 127:274–81. doi: 10.1016/j.mad.2005.11.001
28. Kumar BV, Connors TJ, Farber DL. Human T cell development, localization, and function throughout life. Immunity. (2018) 48:202–13. doi: 10.1016/j.immuni.2018.01.007
29. Thome JJ, Yudanin N, Ohmura Y, Kubota M, Grinshpun B, Sathaliyawala T, et al. Spatial map of human T cell compartmentalization and maintenance over decades of life. Cell. (2014) 159:814–28. doi: 10.1016/j.cell.2014.10.026
30. Mold JE, Venkatasubrahmanyam S, Burt TD, Michaëlsson J, Rivera JM, Galkina SA, et al. Fetal and adult hematopoietic stem cells give rise to distinct T cell lineages in humans. Science. (2010) 330:1695–9. doi: 10.1126/science.1196509
31. Youngblood B, Hale JS, Kissick HT, Ahn E, Xu X, Wieland A, et al. Effector CD8 T cells dedifferentiate into long-lived memory cells. Nature. (2017) 552:404–9. doi: 10.1038/nature25144
32. Yatsunenko T, Rey FE, Manary MJ, Trehan I, Dominguez-Bello MG, Contreras M, et al. Human gut microbiome viewed across age and geography. Nature. (2012) 486:222–7. doi: 10.1038/nature11053
33. Hernández DM, Valderrama S, Gualtero S, Hernández C, López M, Herrera MV, et al. Loss of T-cell multifunctionality and TCR-Vβ repertoire against epstein-barr virus is associated with worse prognosis and clinical parameters in HIV+ patients. Front Immunol. (2018) 9:2291. doi: 10.3389/fimmu.2018.02291
34. Kannanganat S, Ibegbu C, Chennareddi L, Robinson HL, Amara RR. Multiple-cytokine-producing antiviral CD4 T cells are functionally superior to single-cytokine-producing cells. J Virol. (2007) 81:8468–76. doi: 10.1128/JVI.00228-07
35. Ferreira VH, Kumar D, Humar A. Deep profiling of the CD8+ T-cell compartment identifies activated cell subsets and multifunctional responses associated with control of cytomegalovirus viremia. Transplantation. (2019) 103:613–21. doi: 10.1097/TP.0000000000002373
36. Westerhof LM, McGuire K, MacLellan L, Flynn A, Gray JI, Thomas M, et al. Multifunctional cytokine production reveals functional superiority of memory CD4 T cells. Eur J Immunol. (2019) 49:2019–29. doi: 10.1002/eji.201848026
37. Sales NS, Silva JR, Aps LR, Silva MO, Porchia BF, Ferreira LC, et al. In vivo electroporation enhances vaccine-mediated therapeutic control of human papilloma virus-associated tumors by the activation of multifunctional and effector memory CD8+ T cells. Vaccine. (2017) 35:7240–9. doi: 10.1016/j.vaccine.2017.11.011
38. Black A, Bhaumik S, Kirkman RL, Weaver CT, Randolph DA. Developmental regulation of Th17-cell capacity in human neonates. Eur J Immunol. (2012) 42:311–9. doi: 10.1002/eji.201141847
39. Rudolph ME, McArthur MA, Barnes RS, Magder LS, Chen WH, Sztein MB. Differences between pediatric and adult t cell responses to in vitro staphylococcal enterotoxin B stimulation. Front Immunol. (2018) 9:498. doi: 10.3389/fimmu.2018.00498
40. Lelic A, Verschoor CP, Ventresca M, Parsons R, Evelegh C, Bowdish D, et al. The polyfunctionality of human memory CD8+ T cells elicited by acute and chronic virus infections is not influenced by age. PLoS Pathog. (2012) 8:e1003076. doi: 10.1371/journal.ppat.1003076
41. MacLennan IC, Bazin H, Chassoux D, Gray D, Lortan J. Comparative analysis of the development of B cells in marginal zones and follicles. Adv Exp Med Biol. (1985) 186:139–44. doi: 10.1007/978-1-4613-2463-8_17
42. Mond J. T cell independent antigens. Curr Opin Immunol. (1995) 7:349–54. doi: 10.1016/0952-7915(95)80109-X
43. Lee AH, Shannon CP, Amenyogbe N, Bennike TB, Diray-Arce J, Idoko OT, et al. Dynamic molecular changes during the first week of human life follow a robust developmental trajectory. Nat Commun. (2019) 10:1092. doi: 10.1038/s41467-019-08794-x
44. Chen G, Lustig A, Weng N-P. T cell aging: a review of the transcriptional changes determined from genome-wide analysis. Front Immunol. (2013) 4:121. doi: 10.3389/fimmu.2013.00121
Keywords: infant, children, pediatric immunology, development, multifunctional T-cells, immune system, T-cell differentiation, adenoid
Citation: Knolle J, Pierau M, Hebel K, Lampe K, Jorch G, Kropf S, Arens C and Brunner-Weinzierl MC (2020) Children From the Age of Three Show a Developmental Switch in T-Cell Differentiation. Front. Immunol. 11:1640. doi: 10.3389/fimmu.2020.01640
Received: 24 March 2020; Accepted: 18 June 2020;
Published: 28 July 2020.
Edited by:
Ramon Arens, Leiden University Medical Center, NetherlandsReviewed by:
Karl Kai McKinstry, University of Central Florida, United StatesSara Hamilton, University of Minnesota Twin Cities, United States
Copyright © 2020 Knolle, Pierau, Hebel, Lampe, Jorch, Kropf, Arens and Brunner-Weinzierl. This is an open-access article distributed under the terms of the Creative Commons Attribution License (CC BY). The use, distribution or reproduction in other forums is permitted, provided the original author(s) and the copyright owner(s) are credited and that the original publication in this journal is cited, in accordance with accepted academic practice. No use, distribution or reproduction is permitted which does not comply with these terms.
*Correspondence: Monika C. Brunner-Weinzierl, bW9uaWthLmJydW5uZXItd2VpbnppZXJsQG1lZC5vdmd1LmRl
†These authors share first authorship