- 1Department of Preventive Veterinary Medicine, Faculty of Veterinary Medicine and Livestock Sciences, University of Chile, Santiago, Chile
- 2Department of Pathology, Faculty of Veterinary Medicine and Livestock Sciences, University of Chile, Santiago, Chile
- 3Department of Immunology, ICBM, Faculty of Medicine, University of Chile, Santiago, Chile
- 4Department of Medical Microbiology and Immunology, College of Medicine and Life Sciences, University of Toledo, Toledo, OH, United States
Because of its capacity to increase a physiologic inflammatory response, to stimulate phagocytosis, to promote cell lysis and to enhance pathogen immunogenicity, the complement system is a crucial component of both the innate and adaptive immune responses. However, many infectious agents resist the activation of this system by expressing or secreting proteins with a role as complement regulatory, mainly inhibitory, proteins. Trypanosoma cruzi, the causal agent of Chagas disease, a reemerging microbial ailment, possesses several virulence factors with capacity to inhibit complement at different stages of activation. T. cruzi calreticulin (TcCalr) is a highly-conserved, endoplasmic reticulum-resident chaperone that the parasite translocates to the extracellular environment, where it exerts a variety of functions. Among these functions, TcCalr binds C1, MBL and ficolins, thus inhibiting the classical and lectin pathways of complement at their earliest stages of activation. Moreover, the TcCalr/C1 interaction also mediates infectivity by mimicking a strategy used by apoptotic cells for their removal. More recently, it has been determined that these Calr strategies are also used by a variety of other parasites. In addition, as reviewed elsewhere, TcCalr inhibits angiogenesis, promotes wound healing and reduces tumor growth. Complement C1 is also involved in some of these properties. Knowledge on the role of virulence factors, such as TcCalr, and their interactions with complement components in host–parasite interactions, may lead toward the description of new anti-parasite therapies and prophylaxis.
Introduction
The complement system (C), essential in both the innate and adaptive immune responses, increases physiologic inflammation, stimulates microbial phagocytosis and their lysis, and promotes the elimination of a large variety of aggressive microorganisms by enhancing their immunogenicity. Some activated C components and derived molecules, opsonize a variety of microorganisms and apoptotic cells promoting their phagocytosis and destruction inside the phagocyte (1). However, pathogens such as viruses, bacteria, fungi and parasites, utilize some surface proteins and receptors to evade C during its activation (1).
Trypanosoma cruzi calreticulin (TcCalr), similar to calreticulin from other species, including human (HuCALR), is a multifunctional endoplasmic reticulum-resident chaperone, that the parasite translocates to the extracellular environment, where TcCalr participates in C evasion and infection, with important consequences in virulence. Thus, TcCalr is a bona fide virulence factor. Calr from other important parasite species shares several of these properties with TcCalr. These issues are reviewed herein. TcCalr also participates in the control of angiogenesis and tumor growth, as reviewed elsewhere (2).
Complement Activation and Regulation: a Brief Overview
C consists of soluble and membrane-bound molecules that are activated through a stringently regulated proteolytic cascade (3). Activation may occur through the classical (CP), alternative (AP), and lectin (LP) pathways. The CP is initiated by the recognition, by C1, of antibodies aggregated on foreign antigens, or by acute phase proteins identifying danger signals on a microbial aggressor. The LP is activated by mannose-binding lectin (MBL) or by ficolins recognizing a variety of bacterial motifs. Conversely, spontaneous hydrolysis of C3, near cell surfaces, produces a constitutive AP activation, which is tightly controlled by C regulatory proteins present on host cells (Factor I, C4-binding protein, decay-accelerating factor, membrane co-factor protein, C receptor 1) or in plasma (Factor H, C1-inhibitor, S-protein, clusterin, CD59). These proteins limit amplification of the downstream cascade (3, 4).
C activation generates split products with opsonizing, pro-inflammatory and immune-stimulating properties (3). The three activation pathways converge in the generation of C3 convertases that continuously cleave C3 into C3a and C3b, as well as C5 convertases that produce the split products C5a and C5b. C5b, in conjunction with C6–C9, form the membrane attack complex (MAC) and lyse the pathogen (3).
C1 and Calreticulin Interaction Promotes Phagocytosis
In mammals, C1 is a highly complex protein, composed by eighteen polypeptide chains, grouped in six heterotrimeric units, each carrying the products of 3 genes, A, B, C. Each trimer has several functional sites located on both a collagen-like (cC1q) and a globular head (gC1q) regions (5). Each globular head (ghA, ghB, and ghC) has special affinity for the CH2 and CH3 domains of IgG and IgM molecules, respectively, or for other unrelated molecules (5).
Beyond its role as a pattern recognition receptor (PRR), C1 binds to a wide variety of phagocytic cells, resulting in the induction of cell-specific responses such as phagocytosis, cellular activation, release of biological mediators and expression of adhesion molecules, promoting inflammation (6). At least four C1q binding cell surface receptors have been identified: CR1 (CD35), receptor for C3b; C1q-Rp (CD93), a 120 kDa O-sialoglycoprotein; gC1q-R/p33, a 33kDa homotrimeric protein, and cC1q-R/CR, a 60 kDa protein (5, 6). The 33 kDa molecule has high affinity for the globular heads while the 60 kDa molecule, also known as collectin receptor, binds to the collagenous tails and its N-terminal sequence is 100% identical with Calr (5).
Calr is a 46 kDa multifunctional protein, mainly located in the endoplasmic reticulum (ER) and highly conserved in all species, including plants and microorganisms (2, 7, 8). Calr is involved in Ca2+ homeostasis and in other important functions inside and outside the cell, including: cardiogenesis, adipocyte differentiation, cellular stress responses, wound healing and immunity (9). Its structure comprises three main domains: N-terminal globular, flexible proline-rich P intermediate arm-like and C-carboxyl terminal (7, 9).
Both, C1q and MBL bind to apoptotic cells and stimulate phagocytosis by ligation of Calr on the phagocyte surface, which binds to the endocytic receptor protein CD91 (10). Phagocytic cells, monocyte-derived macrophages and dendritic cells express and secrete Calr as a C1q receptor. On the cell, Calr bridges the phagocytic cell and the target (apoptotic cell or an immune complex), promoting removal (6). Activated macrophages secrete Calr, which binds to the surface of viable target cells and marks them for removal by programmed cell phagocytosis (11). Additionally, Calr is found on the surface of apoptotic cells acting as a damage-associated molecular pattern (DAMP), responsible for the immunogenicity of apoptotic cancerous cells (12–14). Binding of C1q to cell-bound Calr results in opsonization (15). Most important, the pro-phagocytic Calr/C1q/C1qR interaction is used by different parasites to promote infectivity.
Trypanosoma Cruzi Evades the Complement System: the Role of Calreticulin
Chagas disease, is a zoonotic and chronic parasitic illness affecting 7–8 million people worldwide, that may be symptomatic in about 30% of those infected, leading to incapacitating situations in some of them. The disease is currently endemic in 21 Latin-American countries and, due to migration of chronically infected individuals, is now a global concern (16). Its causal agent, the flagellated protozoan T. cruzi, is an obligatory intracellular infectious agent transmitted by triatomine vectors, but also by congenital route, blood transfusions, organ transplantation or by ingesting contaminated food and beverages (17). In all these routes of infection, once trypomastigotes (infective form in mammalian host) reach the bloodstream, the parasite, using different proteins and mechanisms, bypasses C-mediated lysis (18), and disseminates to many tissues during the acute phase (19). There are a variety of molecules involved in C immune evasion in T. cruzi. Among them, TcCalr plays an important role.
Bloodstream trypomastigotes, amastigotes (intracellular replicative stage in host cells) and metacyclic trypomastigotes (infective forms present in vector dejections) are resistant to the C-mediated lysis (20). Instead, epimastigotes, the replicative and non-infective form of the parasite, are highly susceptible (21–23). The ability to resist C differs among the parasite developmental stage (24) and strains (21).
Several molecules present on the parasite have been identified as resistance mediators, at different levels of the C cascade. Moreover, trypomastigotes capture inhibitory host components, which are used to inhibit the C activation on the parasite surface, such as: plasma-membrane derived-vesicles (PMV) (25, 26), T. cruzi trypomastigotes-decay accelerating factor (T-DAF) (27, 28), T. cruzi C regulatory protein (CRP) (29–32); Factor H (FH) (33), gp58/68 (34), and C2 receptor inhibitor trispanning (CRIT) (21, 35) (Table 1). The molecular inhibitory mechanisms of these proteins are only partially known. Some of these molecules play a central role in the inhibition of C3 and/or C5 convertases. The inhibition of these key enzymes may have important biological consequences, such as: (i) inhibition of C-mediated lysis, (ii) a decrease in the C3a and C5a (anaphylotoxins) generation (these small C fragments are essential in the recruitment of blood cells to the infection site), and (iii) a decreased opsonization, which mediates phagocytosis of pathogens during infection (25).
TcCalr, similar to its human counterpart, resides in the ER, where it modulates Ca2+ homeostasis and participates as a chaperone protein (39). However, TcCalr is also located in the Golgi, reservosomes, flagellar pocket, cell surface, cytosol, nucleus and kinetoplast. Large quantities of TcCalr accumulate in the kinetoplast, apparently as a previous step to its translocation to the parasite exterior (36, 39, 40). This parasitic protein shares 50% of homology with HuCALR (41) and with its three domains (42): N, P, and C. Within the N and P domains, TcCalr has an S-domain (aa 159–281) that specifically interacts with C1 (43, 44) (Figure 1).
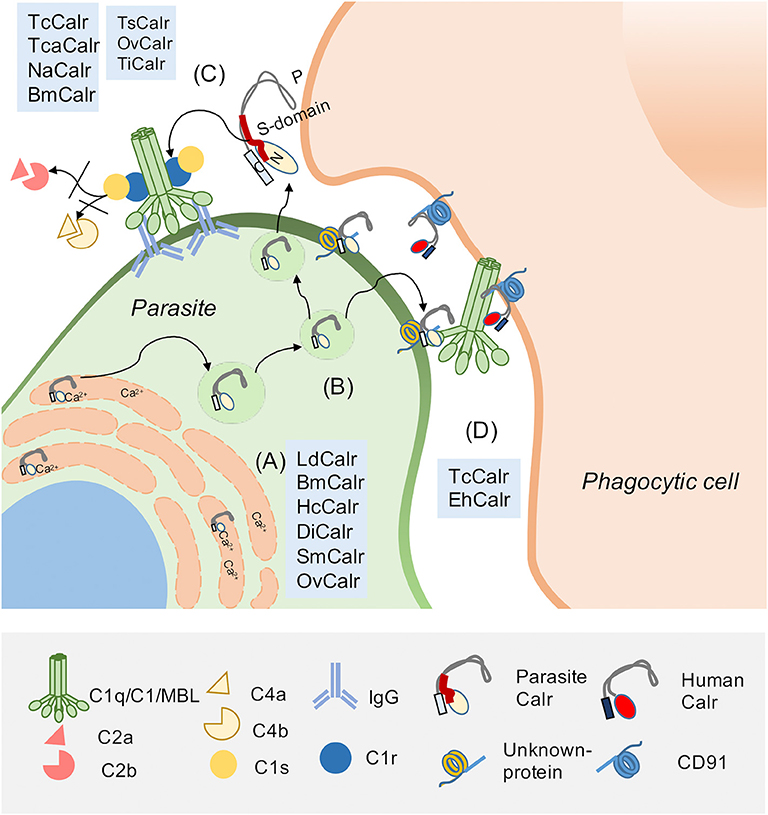
Figure 1. Parasite Calreticulin participates in complement (C) evasion and in infectivity. Calreticulin (Calr), highly conserved and pleiotropic, is mainly found in the endoplasmic reticulum (ER), but also in the extracellular environment. (A) In the ER, Calr is a chaperone and Ca2+ binding protein. These properties are also described for Calrs from other parasites, such as: L. donovani (LdCalr), B. malayi (BmCalr), H. contortus (HcCalr), D. immitis (DiCalr), S. mansoni (SmCalr) and O. viverrini (OvCalr). (B) Extracellularly, the Calr S-domain (a fragment located between N and P domains) interacts with C system molecules such as (C) C1q, C1 complex and MBL, inhibiting C activation. This property has been described for human Calr (HuCALR) and parasitic Calrs such as T. cruzi calreticulin (TcCalr), T. carassii (TcaCalr), N. americanum (NaCalr), BmCalr, T. spiralis (TsCalr), OvCalr, and T. infestans (TiCalr). Additionally, the C1/Calr interaction on the parasite is used by T. cruzi and E. histolytica to promote infectivity. (D) TcCalr and E. histolytica Calr (EhCalr) on the parasite surface bind C1q. This interaction is recognized by HuCALR present on phagocytes as an “eat me” signal. This Calr/C1q/HuCALR interaction, that promotes phagocytosis, was previously described as a mechanism to promote the clearance of apoptotic cells, which overexpress Calr as a damage-associated molecular pattern (DAMP) on their surface. This Calr on apoptotic cells is recognized by the pattern-recognition receptor (PRR) C1q, which is recognized by a C1q receptor on the phagocytic cells, identified as HuCALR. Calr, which does not have a transmembrane tail, binds to CD91 on the phagocyte.
The TcCalr/C1 interaction promotes important functions in the host-parasite interplay. TcCalr competes with the (C1r-C1s)2 tetrameric complex for binding to the collagenous tails of C1q, interfering with the C1s-mediated cleavage of C4 and C2 and thus CP activation (36). TcCalr binds both serine-proteases, C1r and C1s, but only TcCalr-C1r binding inhibits the C4-activating function (37). Additionally, TcCalr competes with the serine proteases, but does not displace them from preformed C1 (37). This fact may be explained by the strong interaction between the enzymatic tetramer (C1r2, C1s2) and C1q (45). TcCalr inhibits C more efficiently than HuCALR and these functional differences may be explained, at least in part, by comparative crystallographic studies that have identified conformational rearrangements in TcCalr and HuCALR (46) and some aminoacidic substitutions that confer differences in polarity and spatial stability (47). TcCalr not only inhibits the CP; it also binds MBL and Ficolins, inhibiting the LP (38). L-, but not H-Ficolin binds to TcCalr, but this binding does not interfere with lipoteichoic acid binding to L-Ficolin and its activation. Moreover, L-Ficolin binds preferentially to trypomastigotes, rather than to epimastigotes, which translocate significantly lower amounts of TcCalr to their surfaces (38). All these facts have been corroborated in vivo by using genetically modified parasites carrying a monoallelic deletion of the TcCRT gene or a transgenic version, expressing an extra copy of the gene. The parasites expressing less TcCalr are significantly more susceptible to C-mediated lysis and those overexpressing TcCRT are significantly more resistant to both CP and LP-mediated lysis (48, 49).
TcCALR/C1 Interaction: Role in Promoting Infectivity
Another important role of the TcCalr/C1 interaction is to promote infectivity. TcCalr is expressed mainly on the trypomastigote flagellum emergence area, where it recruits C1q/C1 (36). This interaction correlates with a TcCalr mRNA level increase in the early infection steps (50). This is corroborated since anti-TcCalr F(ab')2 antibody fragments (unable to bind C1 because they lack their Fc domains) inhibit the TcCalr/C1 interaction, thus decreasing infectivity in vitro and in vivo (50). Non-infective epimastigotes express less TcCalr on their surface (36, 51), which may contribute to their high sensitivity to C and lack of infectivity. In agreement with this notion, when exogenous TcCalr is added, epimastigotes are internalized by fibroblasts, in a C1q-dependent manner (52).
As mentioned, the capacity of the TcCalr/C1q interaction to mediate phagocytosis was originally described for apoptotic cells. C1q and MBL bind to these cells, exposing HuCALR, and stimulating their ingestion by ligation on the phagocyte surface in a HuCALR/C1q-mediated manner (10). We have proposed that the TcCalr/C1q complex is recognized as an “eat me” signal on the parasite by host Calr on phagocytes and other cellular types, thus promoting infectivity (Figure 1). In agreement with these findings, Calr-deficient fibroblasts are unable to internalize these parasites (52). Moreover, in mice inoculated with trypomastigotes, carrying a monoallelic TcCalr deletion, no parasitemia, nor anti-T. cruzi IgG levels are detected, demonstrating that these mutants have a potent restriction in their capacity to infect host cells, due to insufficient Calr expression and consequent reduced resistance to C (49).
Calr is also important in infectivity, as determined in an ex vivo model using human placenta explants, which express high HuCALR levels (53–55). In these explants, the TcCalr/C1q/HuCALR synapsis mediates the first stages of T. cruzi infection (56). This fact is particularly relevant due to the current high impact of congenital Chagas disease transmission.
TcCalr also binds MBL and Ficolins (36, 37), but the role of TcCalr/MBL or TcCalr/Ficolins interactions in T. cruzi infectivity processes has not yet been demonstrated. However, in C-resistant T. cruzi strains, MBL seems to participate in the infectivity process while the parasite deactivates the LP (57). However, the ligand for MBL on the parasite surface remains unknown.
Calreticulin in Other Parasitic Infections
Several functions are shared and conserved, to differing extents, by Calr from different species (41, 58). Calr, is a surprisingly pleiotropic protein, present in all nucleated cells in different organisms including parasites, where it was first described in Schistosoma mansoni (59, 60), Dirofilaria immitis (61) and Necator americanus (62). More recently, Calr has been characterized in Entamoeba histolytica (63, 64), Leishmania donovani (65), Trypanosoma carassii (66), and in the helminths Brugia malayi (67), Haemonchus contortus (68), Opisthorchis viverrini (69, 70), and Trichinella spiralis (71).
Structurally, Calr from different species possesses a broad spectrum of sequence conservations and differences (46). L. donovani Calr (LdCalr) binds Ca2+and RNA sequences (65) and its P-domain is implicated in ER chaperone functions, since a modulation of its expression affects the targeting of proteins associated with virulence, during their trafficking through the parasite secretory pathway (72). Additionally, proteomic approaches indicate LdCalr is an immunostimulatory protein (73, 74). However, its specific role in host-parasite interactions is still unknown.
In E. histolytica, a protozoan parasite that causes amebiasis, Calr (EhCalr) participates in several roles related to the host-immune modulation. Quantitative proteomic analysis and an ex vivo modeling indicates that EhCalr is an abundant membrane protein expressed in virulent variants (75, 76). EhCalr, from pathogenic and non-pathogenic species, binds C1 and inhibits the CP activation (63). Additionally, EhCalr is exported from the ER to the phagolysosome, where it favors phagocytosis in a C1q-dependent manner (64, 77). EhCalr also interferes in the pathogenesis and host immune response modulation. Thus, in vitro, EhCalr acts as an immunogen for the specific activation of peripheral blood mononuclear cells, inducing a Th2 cytokine profile, during the acute phase, and a Th1 profile in the resolution phase (78).
T. carassii, a flagellated bloodstream parasite of cyprinid fish, produces anemia during peak parasitemias and it is highly resistant to C-mediated lysis (79, 80). Its Calr (TcaCalr) is a surface protein that binds C1 and inhibits the CP activation (66), but its role in infectivity has not yet been elucidated. Trypanosoma congolense Calr (TcoCalr) is an immunogen in mice, delaying parasitemia and increasing survival in challenged animals (81).
In nematodes Calr is also important in immune evasion (2). Necator americanus Calr (NaCalr) was first described as a hookworm allergen in infected patients (62). NaCalr does not bind Ca2+, but interacts with C1 and inhibits the CP (82). Calr from B. malayi (BmCalr), a parasite causing lymphatic filariasis, binds Ca2+ and Zinc (67) and interacts with host C1, inhibiting the CP (83). Haemonchus contortus is a gastrointestinal parasite of small ruminants that feeds on blood. The N-domain of HcCalr mediates Ca2+ binding and blood clotting inhibition. It also binds C1 (68) and C-reactive protein (84), thus inhibiting the CP. The C1 binding sites reside in two sequences present in its N-domain (85). Trichinella spiralis activates C in infective larvae, adults and newborn larvae. However, C is primarily activated by the AP (86) and none of these stages bind C1 (87). T. spiralis expresses two proteins that bind C1 and inhibit the CP: paramyosin (88) and Calr (TsCalr) (71). Additionally, TsCalr/C1q binding inhibits the C1-induced non-C activation of macrophages (71). In the nematode Dirofilaria immitis, a Ca2+ binding protein, similar to Calr, was isolated and shown to be immunogenic in chronically-infected microfilaremic dogs (61).
Calrs from the trematodes Schistosoma mansoni (SmCalr) and Schistosoma japonicum (SjCalr) have been characterized (59, 60, 89, 90). SmCalr is a Ca2+ binding protein, mainly present in miracidia and genital organs (59), that participates as T and B cell antigen (60). Both C1 (91) and MBL (92) bind to S. mansoni, but the role of SmCalr in this binding or C evasion is unknown. SjCalr participates as an immunomodulatory protein, activating dendritic cells and inducing a Th1 immune response (89). Calr from Opisthorchis viverrini (OvCalr), a trematode parasite affecting humans, with carcinogenic effects, is mainly expressed in the reproductive system and its C-domain binds Ca2+ (70). OvCalr also binds C1 and inhibits the CP and, additionally, OvCalr is released from the parasite, interfering with cell proliferation, cell migration and sprouting, and stimulates specific antibody production (69).
Hematophagous arthropods also use these Calr-mediated mechanisms to evade C. Thus, Calr from Triatoma infestans (TiCalr), the principal vector of Chagas disease (93), also binds C1 and inhibits the CP. Most likely TiCalr in saliva helps to control the activation of host C, present in the blood meal and consequent digestive tract tissue damage (93). Another example is the tick Amblyomma americanum, which secretes Calr (AaCalr) while feeding (94). AaCalr also binds C1, but this interaction does not inhibit C activation (94). In ticks, such as Boophilus micropus, Calr (BmCalr) is present in saliva, is immunogenic in tick-infested bovines (95) and, similar to Haemaphysalis qinghaiensis Calr (HqCalr), it is secreted in their host during blood sucking, promoting a humoral response (96).
Other TcCALR Functions in the Host-Parasite Interplay
As reviewed elsewhere (2), in addition to their roles in C evasion and infectivity, TcCalr and its N-terminal domain are anti-angiogenic in several experimental set ups (97–99). The anti-tumor effect of T. cruzi infection has been fully reproduced by exogenously administrated rTcCalr (97) and reverted by polyclonal anti-rTcCalr F(ab')2 antibodies (51). Native endogenous TcCalr, in the context of the parasite, also has an anti-tumor effect on T. cruzi infection, since mice inoculated with TA3-MTXR tumor cells, infected with T. cruzi trypomastigotes and treated with anti-TcCalr antibodies neutralize the anti-tumor effect of the infection (51). Most recently, we have proposed that TcCalr binds to canine transmissible venereal tumor (CTVT) cells and to a canine mammary carcinoma cell line, improving the immunogenicity of both tumors. These cells can be engulfed by macrophages and dendritic cells co-cultured with rTcCalr, accelerating its maturation and activating T cells (100). Similar to its human counterpart, TcCalr promotes wound healing in rats (101); however, whether this property correlates with the known anti-complement capacity of the parasite chaperone, is unknown.
Summary
Calr is a multifunctional chaperone, resident in the ER, where it controls Ca+2 homeostasis. However, Calr has important roles outside the cells, because it is also secreted (58). Calr is highly conserved among plants and mammals and some of its functions are significant in host-pathogen interactions (2). Thus, an important function of Calr in microorganisms is its capacity to bind C1, with consequent inhibition of the CP of C (4) and promotion of infectivity (1, 4). More recently, these two important effects have also been described for Calr from a variety of protozoan and metazoan parasites (2). C1 binding allows the parasite to evade the C system and to promote engulfment of the parasite by mimicking a strategy used by apoptotic cells (12). TcCalr also has important functions related with the inhibition of angiogenesis and tumor growth, as revised elsewhere (51, 97, 98). Progress in the knowledge of Calr functions in different parasitic infections may be useful in the design of new therapies and/or vaccines.
Author Contributions
GR-T, VF, and AF contributed equally to the generation of this review. GR-T prepared the figure. GR-T, VF, and AF edited the text. All authors contributed substantially to the writing and with published previous research included herein. All authors contributed to the article and approved the submitted version.
Funding
The University of Toledo Biomedical Research Innovation Program (VF), Toledo, Ohio, USA; VID, University of Chile (AF); FONDECYT-Chile 1130099 (AF), and CONICYT-REDES 170126 (GR-T).
Conflict of Interest
The authors declare that the research was conducted in the absence of any commercial or financial relationships that could be construed as a potential conflict of interest.
References
1. Lukácsi S, Mácsik-Valent B, Nagy-Baló Z, Kovács KG, Kliment K, Bajtay Z, et al. Utilization of complement receptors in immune cell–microbe interaction. FEBS Lett. (2020) doi: 10.1002/1873-3468.13743. [Epub ahead of print].
2. Ramírez-Toloza G, Sosoniuk-Roche E, Valck C, Aguilar-Guzmán L, Ferreira VP, Ferreira A. Trypanosoma cruzi calreticulin: immune evasion, infectivity, and tumorigenesis. Trends Parasitol. (2020) 36:368–381. doi: 10.1016/j.pt.2020.01.007
3. Andrighetto S, Leventhal J, Zaza G, Cravedi P. Complement and complement targeting therapies in glomerular diseases. Int J Mol Sci. (2019) 20:6336. doi: 10.3390/ijms20246336
4. Ramírez-Toloza G, Ferreira A. Trypanosoma cruzi evades the complement system as an efficient strategy to survive in the mammalian host: the specific roles of host/parasite molecules and Trypanosoma cruzi calreticulin. Front Microbiol. (2017) 8:1667. doi: 10.3389/fmicb.2017.01667
5. Ghebrehiwet B, Geisbrecht BV, Xu X, Savitt AG, Peerschke EI. The C1q Receptors: Focus on gC1qR/p33 (C1qBP, p32, HABP-1). Semin Immunol. (2019) 5:101338. doi: 10.1016/j.smim.2019.101338
6. Ghebrehiwet B, Peerschke EI. cC1q-R (calreticulin) and gC1q-R/p33: ubiquitously expressed multi-ligand binding cellular proteins involved in inflammation and infection. Mol Immunol. (2004) 41:173–83. doi: 10.1016/j.molimm.2004.03.014
7. Schcolnik-Cabrera A, Oldak B, Juárez M, Cruz-Rivera M, Flisser A, Mendlovic F. Calreticulin in phagocytosis and cancer: opposite roles in immune response outcomes. Apoptosis. (2019) 24:245–55. doi: 10.1007/s10495-019-01532-0
8. Joshi R, Paul M, Kumar A, Pandey D. Role of calreticulin in biotic and abiotic stress signalling and tolerance mechanisms in plants. Gene. (2019) 714:144004. doi: 10.1016/j.gene.2019.144004
9. Wang W-A, Groenendyk J, Michalak M. Calreticulin signaling in health and disease. Int J Biochem Cell Biol. (2012) 44:842–6. doi: 10.1016/j.biocel.2012.02.009
10. Ogden CA, deCathelineau A, Hoffmann PR, Bratton D, Ghebrehiwet B, Fadok VA, et al. C1q and mannose binding lectin engagement of cell surface calreticulin and CD91 initiates macropinocytosis and uptake of apoptotic cells. J Exp Med. (2001) 194:781–96. doi: 10.1084/jem.194.6.781
11. Feng M, Marjon KD, Zhu F, Weissman-Tsukamoto R, Levett A, Sullivan K, et al. Programmed cell removal by calreticulin in tissue homeostasis and cancer. Nat Commun. (2018) 9:3194. doi: 10.1038/s41467-018-05211-7
12. Galluzzi L, Buqué A, Kepp O, Zitvogel L, Kroemer G. Immunogenic cell death in cancer and infectious disease. Nat Rev Immunol. (2017) 17:97–111. doi: 10.1038/nri.2016.107
13. Obeid M, Tesniere A, Ghiringhelli F, Fimia GM, Apetoh L, Perfettini J-L, et al. Calreticulin exposure dictates the immunogenicity of cancer cell death. Nat Med. (2007) 13:54–61. doi: 10.1038/nm1523
14. Krysko DV, Garg AD, Kaczmarek A, Krysko O, Agostinis P, Vandenabeele P. Immunogenic cell death and DAMPs in cancer therapy. Nat Rev Cancer. (2012) 12:860–75. doi: 10.1038/nrc3380
15. Verneret M, Tacnet-Delorme P, Osman R, Awad R, Grichine A, Kleman J-P, et al. Relative contribution of c1q and apoptotic cell-surface calreticulin to macrophage phagocytosis. J Innate Immun. (2014) 6:426–34. doi: 10.1159/000358834
16. Bivona AE, Alberti AS, Cerny N, Trinitario SN, Malchiodi EL. Chagas disease vaccine design: the search for an efficient trypanosoma cruzi immune-mediated control. Biochim Biophys Acta Mol Basis Dis. (2020) 1866:165658. doi: 10.1016/j.bbadis.2019.165658
17. Vos T, Barber RM, Bell B, Bertozzi-Villa A, Biryukov S, Bolliger I, et al. Global, regional, and national incidence, prevalence, and years lived with disability for 301 acute and chronic diseases and injuries in 188 countries, 1990–2013: a systematic analysis for the global burden of disease study 2013. Lancet. (2015) 386:743–800. doi: 10.1016/S0140-6736(15)60692-4
18. Pangburn MK. Host recognition and target differentiation by factor H, a regulator of the alternative pathway of complement. Immunopharmacology. (2000) 49:149–57. doi: 10.1016/S0162-3109(00)80300-8
19. Cardoso MS, Reis-Cunha JL, Bartholomeu DC. Evasion of the immune response by trypanosoma cruzi during acute infection. Front Immunol. (2015) 6:659. doi: 10.3389/fimmu.2015.00659
20. Iida K, Whitlow MB, Nussenzweig V. Amastigotes of trypanosoma cruzi escape destruction by the terminal complement components. J Exp Med. (1989) 169:881–91. doi: 10.1084/jem.169.3.881
21. Cestari Idos S, Evans-Osses I, Freitas JC, Inal JM, Ramirez MI. Complement C2 receptor inhibitor trispanning confers an increased ability to resist complement-mediated lysis in trypanosoma cruzi. J Infect Dis. (2008) 198:1276–83. doi: 10.1086/592167
22. Muniz J, Borriello A. Etudo sobre a agao htica de diferentes soros sobre as formas de cultura e sanguicolas do schizotrypanum cruzi en crithidias. Rev Brasil Med. (1945) 2:994–8.
23. Rubio M. Actividad lítica de sueros normales sobre formas de cultivo y sanguíneas de Trypanosoma cruzi. Bol Chil Parasitol. (1956) 11:62–9.
24. Anziano DF, Dalmasso AP, Lelchuk R, Vasquez C. Role of complement in immune lysis of trypanosoma cruzi. Infect Immun. (1972) 6:860–4. doi: 10.1128/IAI.6.5.860-864.1972
25. Cestari I, Ansa-Addo E, Deolindo P, Inal JM, Ramirez MI. Trypanosoma cruzi immune evasion mediated by host cell-derived microvesicles. J Immunol (Baltimore, Md: 1950). (2012) 188:1942–52. doi: 10.4049/jimmunol.1102053
26. Ramirez MI, Deolindo P, de Messias-Reason IJ, Arigi EA, Choi H, Almeida IC, et al. Dynamic flux of microvesicles modulate parasite-host cell interaction of trypanosoma cruzi in eukaryotic cells. Cell Microbiol. (2017) 19:e12672. doi: 10.1111/cmi.12672
27. Kipnis TL, Joiner KA, da Silva WD, Rimoldi MT, Hammer CH, Sher A. Identification of membrane components of trypanosoma cruzi modulators of complement system. Mem Inst Oswaldo Cruz. (1988) 83(Suppl. 1):571–5. doi: 10.1590/S0074-02761988000500069
28. Tambourgi DV, Kipnis TL, da Silva WD, Joiner KA, Sher A, Heath S, et al. A partial cDNA clone of trypomastigote decay-accelerating factor (T-DAF), a developmentally regulated complement inhibitor of trypanosoma cruzi, has genetic and functional similarities to the human complement inhibitor DAF. Infect Immun. (1993) 61:3656–63. doi: 10.1128/IAI.61.9.3656-3663.1993
29. Norris KA, Bradt B, Cooper NR, So M. Characterization of a trypanosoma cruzi C3 binding protein with functional and genetic similarities to the human complement regulatory protein, decay-accelerating factor. J Immunol (Baltimore, Md: 1950). (1991) 147:2240–7.
30. Norris KA, Schrimpf JE. Biochemical analysis of the membrane and soluble forms of the complement regulatory protein of Trypanosoma cruzi. Infect Immun. (1994) 62:236–43. doi: 10.1128/IAI.62.1.236-243.1994
31. Beucher M, Meira WS, Zegarra V, Galvao LM, Chiari E, Norris KA. Expression and purification of functional, recombinant trypanosoma cruzi complement regulatory protein. Protein Expr Purif. (2003) 27:19–26. doi: 10.1016/S1046-5928(02)00562-4
32. Norris KA. Stable transfection of trypanosoma cruzi epimastigotes with the trypomastigote-specific complement regulatory protein cDNA confers complement resistance. Infect Immun. (1998) 66:2460–5. doi: 10.1128/IAI.66.6.2460-2465.1998
33. Schenkman S, Guther ML, Yoshida N. Mechanism of resistance to lysis by the alternative complement pathway in trypanosoma cruzi trypomastigotes: effect of specific monoclonal antibody. J Immunol (Baltimore, Md: 1950). (1986) 137:1623–8.
34. Fischer E, Ouaissi MA, Velge P, Cornette J, Kazatchkine MD. gp 58/68, a parasite component that contributes to the escape of the trypomastigote form of T. cruzi from damage by the human alternative complement pathway. Immunology. (1988) 65:299–303.
35. Cestari Idos S, Krarup A, Sim RB, Inal JM, Ramirez MI. Role of early lectin pathway activation in the complement-mediated killing of trypanosoma cruzi. Mol Immunol. (2009) 47:426–37. doi: 10.1016/j.molimm.2009.08.030
36. Ferreira V, Valck C, Sanchez G, Gingras A, Tzima S, Molina MC, et al. The classical activation pathway of the human complement system is specifically inhibited by calreticulin from trypanosoma cruzi. J Immunol (Baltimore, Md: 1950). (2004) 172:3042–50. doi: 10.4049/jimmunol.172.5.3042
37. Valck C, Ramirez G, Lopez N, Ribeiro CH, Maldonado I, Sanchez G, et al. Molecular mechanisms involved in the inactivation of the first component of human complement by trypanosoma cruzi calreticulin. Mol Immunol. (2010) 47:1516–21. doi: 10.1016/j.molimm.2010.01.019
38. Sosoniuk E, Vallejos G, Kenawy H, Gaboriaud C, Thielens N, Fujita T, et al. Trypanosoma cruzi calreticulin inhibits the complement lectin pathway activation by direct interaction with L-Ficolin. Mol Immunol. (2014) 60:80–5. doi: 10.1016/j.molimm.2014.03.014
39. Gonzalez A, Valck C, Sanchez G, Hartel S, Mansilla J, Ramirez G, et al. Trypanosoma cruzi calreticulin topographical variations in parasites infecting murine macrophages. Am J Trop Med Hygiene. (2015) 92:887–97. doi: 10.4269/ajtmh.14-0497
40. Souto-Padron T, Labriola CA, de Souza W. Immunocytochemical localisation of calreticulin in trypanosoma cruzi. Histochem Cell Biol. (2004) 122:563–9. doi: 10.1007/s00418-004-0724-7
41. Ramirez G, Valck C, Ferreira VP, Lopez N, Ferreira A. Extracellular trypanosoma cruzi calreticulin in the host-parasite interplay. Trends Parasitol. (2011) 27:115–22. doi: 10.1016/j.pt.2010.12.007
42. Michalak M, Groenendyk J, Szabo E, Gold LI, Opas M. Calreticulin, a multi-process calcium-buffering chaperone of the endoplasmic reticulum. Biochem J. (2009) 417:651–66. doi: 10.1042/BJ20081847
43. Stuart GR, Lynch NJ, Lu J, Geick A, Moffatt BE, Sim RB, et al. Localisation of the C1q binding site within C1q receptor/calreticulin. FEBS Lett. (1996) 397:245–9. doi: 10.1016/S0014-5793(96)01156-8
44. Stuart GR, Lynch NJ, Day AJ, Schwaeble WJ, Sim RB. The C1q and collectin binding site within C1q receptor (cell surface calreticulin). Immunopharmacology. (1997) 38:73–80. doi: 10.1016/S0162-3109(97)00076-3
45. Wallis R, Mitchell DA, Schmid R, Schwaeble WJ, Keeble AH. Paths reunited: Initiation of the classical and lectin pathways of complement activation. Immunobiology. (2010) 215:1–11. doi: 10.1016/j.imbio.2009.08.006
46. Moreau C, Cioci G, Iannello M, Laffly E, Chouquet A, Ferreira A, et al. Structures of parasite calreticulins provide insights into their flexibility and dual carbohydrate/peptide-binding properties. IUCrJ. (2016) 3(Pt 6):408–19. doi: 10.1107/S2052252516012847
47. Álvarez JP, Teneb J, Maldonado I, Weinberger K, Rosas C, Lemus D, et al. Structural bases that underline trypanosoma cruzi calreticulin proinfective, antiangiogenic and antitumor properties. Immunobiology. (2020) 225:151863. doi: 10.1016/j.imbio.2019.10.012
48. Sanchez Valdez FJ, Perez Brandan C, Zago MP, Labriola C, Ferreira A, Basombrio MA. Trypanosoma cruzi carrying a monoallelic deletion of the calreticulin (TcCRT) gene are susceptible to complement mediated killing and defective in their metacyclogenesis. Mol Immunol. (2013) 53:198–205. doi: 10.1016/j.molimm.2012.08.001
49. Sanchez-Valdez FJ, Perez Brandan C, Ramirez G, Uncos AD, Zago MP, Cimino RO, et al. A monoallelic deletion of the TcCRT gene increases the attenuation of a cultured trypanosoma cruzi strain, protecting against an in vivo virulent challenge. PLoS Neglect Trop Dis. (2014) 8:e2696. doi: 10.1371/journal.pntd.0002696
50. Ramirez G, Valck C, Molina MC, Ribeiro CH, Lopez N, Sanchez G, et al. Trypanosoma cruzi calreticulin: a novel virulence factor that binds complement C1 on the parasite surface and promotes infectivity. Immunobiology. (2011) 216:265–73. doi: 10.1016/j.imbio.2010.04.001
51. Abello-Caceres P, Pizarro-Bauerle J, Rosas C, Maldonado I, Aguilar-Guzman L, Gonzalez C, et al. Does native trypanosoma cruzi calreticulin mediate growth inhibition of a mammary tumor during infection? BMC Cancer. (2016) 16:731. doi: 10.1186/s12885-016-2764-5
52. Sosoniuk-Roche E, Vallejos G, Aguilar-Guzmán L, Pizarro-Bäuerle J, Weinberger K, Rosas C, et al. Exogenous calreticulin, incorporated onto non-infective trypanosoma cruzi epimastigotes, promotes their internalization into mammal host cells. Immunobiology. (2017) 222:529–35. doi: 10.1016/j.imbio.2016.10.020
53. Houen G, Koch C. Human placental calreticulin: purification, characterization and association with other proteins. Acta Chem Scan. (1994) 48:905–11. doi: 10.3891/acta.chem.scand.48-0905
54. Crawford K, Kalionis B, Stevenson J, Brennecke S, Gude N. Calreticulin has opposing effects on the migration of human trophoblast and myometrial endothelial cells. Placenta. (2012) 33:416–23. doi: 10.1016/j.placenta.2012.02.003
55. Gu V, Wong M, Stevenson J, Crawford K, Brennecke S, Gude N. Calreticulin in human pregnancy and pre-eclampsia. Mol Hum Reprod. (2008) 14:309–15. doi: 10.1093/molehr/gan017
56. Castillo C, Ramirez G, Valck C, Aguilar L, Maldonado I, Rosas C, et al. The interaction of classical complement component C1 with parasite and host calreticulin mediates trypanosoma cruzi infection of human placenta. PLoS Neglect Trop Dis. (2013) 7:e2376. doi: 10.1371/journal.pntd.0002376
57. Evans-Osses I, Mojoli A, Beltrame MH, da Costa DE, DaRocha WD, Velavan TP, et al. Differential ability to resist to complement lysis and invade host cells mediated by MBL in R4 and 860 strains of trypanosoma cruzi. FEBS Lett. (2014) 588:956–61. doi: 10.1016/j.febslet.2014.01.054
58. Eggleton P, Bremer E, Dudek E, Michalak M. Calreticulin, a therapeutic target? Expert Opin Ther Targets. (2016) 20:1137–47. doi: 10.1517/14728222.2016.1164695
59. Khalife J, Liu J, Pierce R, Porchet E, Godin C, Capron A. Characterization and localization of schistosoma mansoni calreticulin Sm58. Parasitology. (1994) 108:527–32. doi: 10.1017/S0031182000077398
60. El Gengehi N, El Ridi R, Tawab NA, El Demellawy M, Mangold BL. A schistosoma mansoni 62-kDa band is identified as an irradiated vaccine T-cell antigen and characterized as calreticulin. J Parasitol. (2000) 86:993–1000. doi: 10.1645/0022-3395 (2000) 086[0993:ASMKBI]2.0.CO;2
61. Tsuji N, Morales T, Ozols V, Carmody A, Chandrashekar R. Molecular characterization of a calcium-binding protein from the filarial parasite dirofilaria immitis. Mol Biochem Parasitol. (1998) 97:69–79. doi: 10.1016/S0166-6851(98)00131-5
62. Brown A, Kasper G, Mcelroy P, Loukas A, Hewitt C, Berry C, et al. A hookworm allergen which strongly resembles calreticulin. Parasite Immunol. (1999) 21:439–50. doi: 10.1046/j.1365-3024.1999.00238.x
63. Ximénez C, González E, Nieves ME, Silva-Olivares A, Shibayama M, Galindo-Gómez S, et al. Entamoeba histolytica and E. dispar calreticulin: inhibition of classical complement pathway and differences in the level of expression in amoebic liver abscess. BioMed Res Int. (2014) 2014:127453. doi: 10.1155/2014/127453
64. González E, de Leon MdCG, Meza I, Ocadiz-Delgado R, Gariglio P, Silva-Olivares A, et al. Entamoeba histolytica calreticulin: an endoplasmic reticulum protein expressed by trophozoites into experimentally induced amoebic liver abscesses. Parasitol Res. (2011) 108:439–49. doi: 10.1007/s00436-010-2085-6
65. Joshi M, Pogue GP, Duncan RC, Lee NS, Singh NK, Atreya CD, et al. Isolation and characterization of leishmania donovani calreticulin gene and its conservation of the RNA binding activity. Mol Biochem Parasitol. (1996) 81:53–64. doi: 10.1016/0166-6851(96)02676-X
66. Oladiran A, Belosevic M. Trypanosoma carassii calreticulin binds host complement component C1q and inhibits classical complement pathway-mediated lysis. Dev Comp Immunol. (2010) 34:396–405. doi: 10.1016/j.dci.2009.11.005
67. Yadav S, Prakash J, Saxena JK. Metal binding study of calreticulin: an immunomodulatory protein of human filarial parasite brugia malayi. Int J Biol Macromol. (2018) 117:1157–68. doi: 10.1016/j.ijbiomac.2018.06.011
68. Suchitra S, Joshi P. Characterization of haemonchus contortus calreticulin suggests its role in feeding and immune evasion by the parasite. Biochim Biophys Acta Gen Subjects. (2005) 1722:293–303. doi: 10.1016/j.bbagen.2004.12.020
69. Chaibangyang W, Geadkaew-Krenc A, Smooker PM, Tesana S, Grams R. Evaluation of opisthorchis viverrini calreticulin for potential host modulation. Acta Trop. (2018) 187:175–81. doi: 10.1016/j.actatropica.2018.08.009
70. Chaibangyang W, Geadkaew-Krenc A, Vichasri-Grams S, Tesana S, Grams R. Molecular and biochemical characterization of opisthorchis viverrini calreticulin. Korean J Parasitol. (2017) 55:643. doi: 10.3347/kjp.2017.55.6.643
71. Zhao L, Shao S, Chen Y, Sun X, Sun R, Huang J, et al. Trichinella spiralis calreticulin binds human complement C1q as an immune evasion strategy. Front Immunol. (2017) 8:636. doi: 10.3389/fimmu.2017.00636
72. Debrabant A, Lee N, Pogue GP, Dwyer DM, Nakhasi HL. Expression of calreticulin P-domain results in impairment of secretory pathway in leishmania donovani and reduced parasite survival in macrophages. Int J Parasitol. (2002) 32:1423–34. doi: 10.1016/S0020-7519(02)00134-0
73. Gupta SK, Sisodia BS, Sinha S, Hajela K, Naik S, Shasany AK, et al. Proteomic approach for identification and characterization of novel immunostimulatory proteins from soluble antigens of leishmania donovani promastigotes. Proteomics. (2007) 7:816–23. doi: 10.1002/pmic.200600725
74. Kumari S, Samant M, Misra P, Khare P, Sisodia B, Shasany AK, et al. Th1-stimulatory polyproteins of soluble Leishmania donovani promastigotes ranging from 89.9 to 97.1 kDa offers long-lasting protection against experimental visceral leishmaniasis. Vaccine. (2008) 26:5700–11. doi: 10.1016/j.vaccine.2008.08.021
75. Ng YL, Olivos-García A, Lim TK, Noordin R, Lin Q, Othman N. Entamoeba histolytica: quantitative proteomics analysis reveals putative virulence-associated differentially abundant membrane proteins. Am J Trop Med Hygiene. (2018) 99:1518–29. doi: 10.4269/ajtmh.18-0415
76. Ximénez C, González E, Nieves M, Magaña U, Morán P, Gudiño-Zayas M, et al. Differential expression of pathogenic genes of entamoeba histolytica vs E. dispar in a model of infection using human liver tissue explants. PLoS ONE. (2017) 12:e0181962. doi: 10.1371/journal.pone.0181962
77. Vaithilingam A, Teixeira JE, Miller PJ, Heron BT, Huston CD. Entamoeba histolytica cell surface calreticulin binds human c1q and functions in amebic phagocytosis of host cells. Infect Immun. (2012) 80:2008–18. doi: 10.1128/IAI.06287-11
78. Gonzalez Rivas E, Ximenez C, Nieves-Ramirez ME, Moran Silva P, Partida-Rodríguez O, Hernandez EH, et al. Entamoeba histolytica calreticulin induces the expression of cytokines in peripheral blood mononuclear cells isolated from patients with amebic liver abscess. Front Cell Infect Microbiol. (2018) 8:358. doi: 10.3389/fcimb.2018.00358
79. McAllister M, Phillips N, Belosevic M. Trypanosoma carassii infection in goldfish (Carassius auratus L.): changes in the expression of erythropoiesis and anemia regulatory genes. Parasitol Res. (2019) 118:1147–58. doi: 10.1007/s00436-019-06246-5
80. Plouffe D, Belosevic M. Enzyme treatment of trypanosoma danilewskyi (Laveran & Mesnil) increases its susceptibility to lysis by the alternative complement pathway of goldfish, Carassius auratus (L.). J Fish Dis. (2004) 27:277–85. doi: 10.1111/j.1365-2761.2004.00541.x
81. Bossard G, Grébaut P, Thévenon S, Séveno M, Berthier D, Holzmuller P. Cloning, expression, molecular characterization and preliminary studies on immunomodulating properties of recombinant Trypanosoma congolense calreticulin. Infect Genet Evol. (2016) 45:320–31. doi: 10.1016/j.meegid.2016.09.020
82. Kasper G, Brown A, Eberl M, Vallar L, Kieffer N, Berry C, et al. A calreticulin-like molecule from the human hookworm necator americanus interacts with C1q and the cytoplasmic signalling domains of some integrins. Parasite Immunol. (2001) 23:141–52. doi: 10.1046/j.1365-3024.2001.00366.x
83. Yadav S, Gupta S, Selvaraj C, Doharey PK, Verma A, Singh SK, et al. In silico and in vitro studies on the protein-protein interactions between Brugia malayi immunomodulatory protein calreticulin and human C1q. PLoS ONE. (2014) 9:e106413. doi: 10.1371/journal.pone.0106413
84. Suchitra S, Anbu K, Rathore D, Mahawar M, Singh B, Joshi P. Haemonchus contortus calreticulin binds to C-reactive protein of its host, a novel survival strategy of the parasite. Parasite Immunol. (2008) 30:371–4. doi: 10.1111/j.1365-3024.2008.01028.x
85. Naresha S, Suryawanshi A, Agarwal M, Singh B, Joshi P. Mapping the complement C1q binding site in haemonchus contortus calreticulin. Mol Biochem Parasitol. (2009) 166:42–6. doi: 10.1016/j.molbiopara.2009.02.007
86. Hong Y, Kim CW, Ghebrehiwet B. Trichinella spiralis: activation of complement by infective larvae, adults, and newborn larvae. Exp Parasitol. (1992) 74:290–9. doi: 10.1016/0014-4894(92)90152-Z
87. Näreaho A, Saari S, Meri S, Sukura A. Complement membrane attack complex formation and infectivity of trichinella spiralis and T. nativa in rats. Vet Parasitol. (2009) 159:263–7. doi: 10.1016/j.vetpar.2008.10.037
88. Sun R, Zhao X, Wang Z, Yang J, Zhao L, Zhan B, et al. Trichinella spiralis paramyosin binds human complement C1q and inhibits classical complement activation. PLoS Neglect Trop Dis. (2015) 9:e004310. doi: 10.1371/journal.pntd.0004310
89. Ma L, Li D, Yuan C, Zhang X, Ta N, Zhao X, et al. SjCRT, a recombinant schistosoma japonicum calreticulin, induces maturation of dendritic cells and a Th1-polarized immune response in mice. Parasites Vectors. (2017) 10:570. doi: 10.1186/s13071-017-2516-7
90. Huggins MC, Gibbs J, Moloney NA. Cloning of a schistosoma japonicum gene encoding an antigen with homology to calreticulin. Mol Biochem Parasitol. (1995) 71:81–7. doi: 10.1016/0166-6851(95)00038-3
91. Santoro F, Ouaissi MA, Pestel J, Capron A. Interaction between schistosoma mansoni and the complement system: binding of C1q to schistosomula. J Immunol. (1980) 124:2886–91.
92. Klabunde J, Berger J, Jensenius JC, Klinkert M-Q, Zelck UE, Kremsner PG, et al. Schistosoma mansoni: adhesion of mannan-binding lectin to surface glycoproteins of cercariae and adult worms. Exp Parasitol. (2000) 95:231–9. doi: 10.1006/expr.2000.4539
93. Weinberger K, Collazo N, Aguillón JC, Molina MC, Rosas C, Peña J, et al. Triatoma infestans calreticulin: gene cloning and expression of a main domain that interacts with the host complement system. Am J Trop Med Hygiene. (2017) 96:295–303. doi: 10.4269/ajtmh.16-0642
94. Kim TK, Ibelli AMG, Mulenga A. Amblyomma americanum tick calreticulin binds C1q but does not inhibit activation of the classical complement cascade. Ticks Tick-Borne Dis. (2015) 6:91–101. doi: 10.1016/j.ttbdis.2014.10.002
95. Ferreira CAS, Vaz IDS Jr, da Silva SS, Haag KL, Valenzuela JG, Masuda A. Cloning and partial characterization of a boophilus microplus (acari: ixodidae) calreticulin. Exp Parasitol. (2002) 101:25–34. doi: 10.1016/S0014-4894(02)00032-2
96. Gao J, Luo J, Fan R, Fingerle V, Guan G, Liu Z, et al. Cloning and characterization of a cDNA clone encoding calreticulin from haemaphysalis qinghaiensis (acari: ixodidae). Parasitol Res. (2008) 102:737–46. doi: 10.1007/s00436-007-0826-y
97. Lopez NC, Valck C, Ramirez G, Rodriguez M, Ribeiro C, Orellana J, et al. Antiangiogenic and antitumor effects of trypanosoma cruzi calreticulin. PLoS Neglect Trop Dis. (2010) 4:e730. doi: 10.1371/journal.pntd.0000730
98. Molina MC, Ferreira V, Valck C, Aguilar L, Orellana J, Rojas A, et al. An in vivo role for trypanosoma cruzi calreticulin in antiangiogenesis. Mol Biochem Parasitol. (2005) 140:133–40. doi: 10.1016/j.molbiopara.2004.12.014
99. Toledo V, Ramírez G, Valck C, López N, Ribeiro CH, Maldonado I, et al. Comparative in vivo antiangiogenic effects of calreticulin from trypanosoma cruzi and Homo sapiens. Biol Res. (2010) 43:287–9. doi: 10.4067/S0716-97602010000300004
100. Cruz P, Sosoniuk-Roche E, Maldonado I, Torres C, Ferreira A. Trypanosoma cruzi calreticulin: in vitro modulation of key immunogenic markers of both canine tumors and relevant immune competent cells. Immunobiology. (2019) 225:151892. doi: 10.1016/j.imbio.2019.12.001
Keywords: Trypanosoma cruzi, complement, calreticulin, C1q, host-parasite interaction, host immune evasion
Citation: Ramírez-Toloza G, Aguilar-Guzmán L, Valck C, Ferreira VP and Ferreira A (2020) The Interactions of Parasite Calreticulin With Initial Complement Components: Consequences in Immunity and Virulence. Front. Immunol. 11:1561. doi: 10.3389/fimmu.2020.01561
Received: 29 April 2020; Accepted: 12 June 2020;
Published: 23 July 2020.
Edited by:
Heribert Stoiber, Innsbruck Medical University, AustriaReviewed by:
Marcel I. Ramirez, Oswaldo Cruz Foundation (Fiocruz), BrazilDebora Decote-Ricardo, Universidade Federal Rural do Rio de Janeiro, Brazil
Copyright © 2020 Ramírez-Toloza, Aguilar-Guzmán, Valck, Ferreira and Ferreira. This is an open-access article distributed under the terms of the Creative Commons Attribution License (CC BY). The use, distribution or reproduction in other forums is permitted, provided the original author(s) and the copyright owner(s) are credited and that the original publication in this journal is cited, in accordance with accepted academic practice. No use, distribution or reproduction is permitted which does not comply with these terms.
*Correspondence: Galia Ramírez-Toloza, galiaram@uchile.cl; Viviana P. Ferreira, viviana.ferreira@utoledo.edu; Arturo Ferreira, aferreir@med.uchile.cl