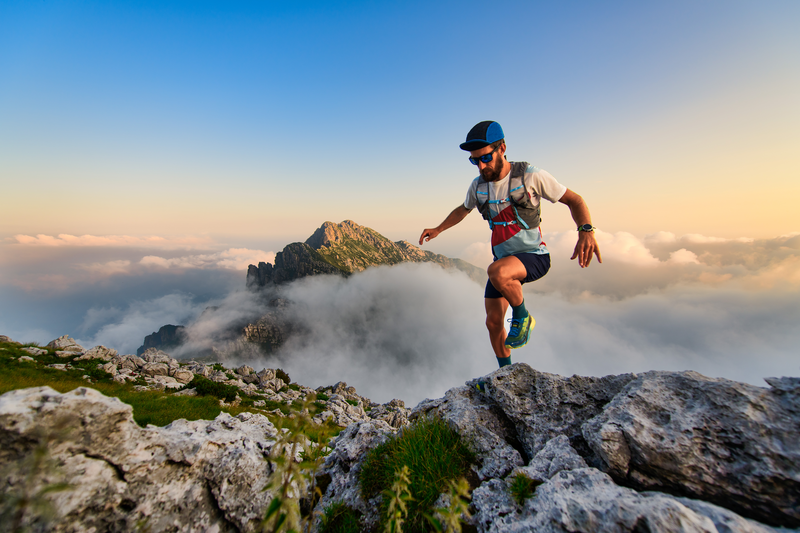
95% of researchers rate our articles as excellent or good
Learn more about the work of our research integrity team to safeguard the quality of each article we publish.
Find out more
REVIEW article
Front. Immunol. , 10 July 2020
Sec. Nutritional Immunology
Volume 11 - 2020 | https://doi.org/10.3389/fimmu.2020.01407
This article is part of the Research Topic Dietary Polyphenols for Improving Gut Health: Volume 1 View all 23 articles
Colorectal cancer (CRC) is one of the most prevalent cancers that threaten people in many countries. It is a multi-factorial chronic disease caused by a combination of genetic and environmental factors, but it is mainly related to lifestyle factors, including diet. Plentiful plant foods and beverages are abundant in polyphenols with antioxidant, anti-atherosclerotic, anti-inflammatory, and anticancer properties. These compounds participate in host nutrition and disease pathology regulation in different ways. Polyphenolic compounds have been used to prevent and inhibit the development and prognosis of cancer, and examples include green tea polyphenol (–)epigallocatechin-3-O-gallate (EGCG), curcumin, and resveratrol. Of course, there are more known and unknown polyphenol compounds that need to be further explored for their anticancer properties. This article focuses on the fact that polyphenols affect the progression of CRC by controlling intestinal inflammation, epigenetics, and the intestinal microbe in the aspects of prevention, treatment, and prognosis.
Colorectal cancer (CRC) is one of the most common malignancies worldwide (1, 2). Like many diseases, the formation of CRC is caused by various genetic and environmental factors (3). Globally in 2018, CRC ranked third in the morbidity of all new cancer cases, with more than 1.8 million new cases, and the second in mortality, with more than 860,000 deaths (4). CRC has a genetic susceptibility syndrome, but this condition accounts for only a small portion of CRC cases (5). According to the analysis of twin and family studies, the heritability of CRC is only 12–35%. The relatively low heritability level of CRC reflects the importance of the environment, that is, the environment plays a greater role in causing sporadic CRC (6). Cancer prevention is one of the most significant priorities in public health (7). Epidemiological studies on the relationship between dietary habits and disease risk have shown that diet has a direct impact on public health (8). Much effort has been made to assess the preventive chemical effects of natural products in the past few decades. Polyphenols have attracted much attention due to their advantages of few side effects, wide availability, and low toxicity (9). Polyphenols are widely found in the plant kingdom. They are considered potentially useful for anti-inflammatory and anti-tumor drugs, which may be one of the good candidate drugs for cancer prevention and treatment on account of affecting the disease process of cancer in many ways (10, 11). Hence, polyphenols are considered as potential molecular sources for the treatment of various malignant tumors.
Polyphenols are phytochemicals existing in plants, widely found in tea, vegetables, soft fruits, and wine, which can regulate intestinal microecological stability and reduce cancer risk (12–14). Although symptoms cannot be described in their absence, in vitro and animal model studies have shown that polyphenols have a wide range of pharmacological and therapeutic properties, including anticancer (13), anti-inflammatory (15), antioxidant (16), and vascular protective properties (17). The evidence revealed that epigallocatechin-3-gallate (EGCG) suppressed the growth of melanoma cells by activating 67-kDa laminin receptor (67LR) signaling pathway. That is, 67LR participates in the upregulation of miRNA let-7b expression induced by EGCG through cAMP/protein kinase A (PKA)/protein phosphatase 2A (PP2A) signaling pathway, while the upregulation of let-7b results in the downregulation of a high mobility group A2 (HMGA2), the target gene related to tumor development (18). Olive oil polyphenols represented the pro-oxidative and proinflammatory effects undergoing the representative mixture of oxysterols, while Caco-2 completely differentiates into intestinal epithelial-like cells. In addition, olive oil polyphenols could directly regulate the phosphorylation of p38 and JNK1/2 and the activation of NF-κB after phosphorylation of IκB and maintain the level of NO with that of the control group by inhibiting iNOS induction (19). Polyphenol-rich plum could prevent weight gain and increase the proportion of high-density lipoprotein cholesterol and total cholesterol in plasma. In addition, it could reduce the level of angiotensin II in plasma and its receptor Agtr1 in heart tissue, which reveals that polyphenols may be a receptor-γ agonist activated by peroxisome proliferators, and these results suggest that polyphenol-rich plum may possess the properties of myocardial protection (20). The study showed that chlorogenic acid stimulates the expression of IFN-γ mRNA and increases the number of IFN- γ+ CD4+ cells in mouse lymphoid aggregation cells, while the number of cells (IFN- γ+ CD4+, IFN-γ+ CD49b+, and IL-12+ CD11b+) in mouse spleen increased significantly, which indicated that polyphenols had a certain stimulating effect on the cellular immune system of mice (21). Table 1 shows the health properties of a variety of polyphenols from different sources. Diet composition and habits are closely related to the occurrence of cancer. The study has shown that green tea polyphenols have a protective effect on female CRC patients, and there is a significant negative correlation between the daily intake of 1 cup of tea and the risk of CRC (30). In addition, the study of F344 rat model treated with n azoxymethane showed that polyphenon E reduced tumor diversity and tumor size while reducing the nuclear expression of β-catenin, inducing apoptosis, and increasing RXRα, β, and γ expression levels in adenocarcinoma (26). Due to the fewer side effects and low toxicity of polyphenols, the biological activity of polyphenols has become a hot topic in many research fields over the years (31).
The colon is a part of the digestive system and has a complex three-dimensional structure, and the length of the human colon is about 100–150 cm (32, 33). Its main function is to absorb the water and electrolyte left after the small intestine washes and excretes the feces. In terms of deconstruction, the colon begins with the cecum, followed by the colon, transverse colon, descending colon, sigmoid colon, and rectum. This organ is covered by double outer muscles and smooth muscle cells (34). In the mass, the development of cancer can be divided into three stages: initiation, progress, and promotion (35), as shown in Figure 1. The initial stage of CRC starts from the normal mucosa, and the cell replication is indiscriminately disordered and shows abnormal proliferation with the formation of enlarged crypt clusters (36, 37). If there is no appropriate intervention treatment in the development process, the adenoma will expand into CRC, which can spread in the body through metastasis (36). The development of CRC is a multi-step process that follows an adenoma-cancer sequence and has a background of genomic instability (38). CRC shares a number of common molecular characteristics, including microsatellite instability, chromosomal instability, and epigenetic silencing through the CpG island methylated phenotype (39). According to the unique genetic, pathological, and clinical characteristics of these pathways, these can be used for the molecular classification of CRC and comprehensive analysis of tumors to improve the diagnosis, treatment, and prognosis of the disease according to the unused conditions (39). According to the evidence provided by the World Cancer Research Foundation, processed foods, such as those smoked, pickled, or preserved with chemical preservatives, and red meat will increase the possibility of inducing CRC. In contrast, fiber-rich foods can reduce the risk of CRC (40, 41).
Figure 1. The process of colorectal cancer. The development of cancer includes three stages: initiation, progress, and promotion. Poor dietary habits, such as the long-term consumption of processed meat (smoked meat, picked meat, sausage, etc.) and red meat, may accelerate tumor burden and eventually induce CRC. CRC, colorectal cancer.
Metabolism is a key step in the digestion and absorption of nutrients and further participation in various physiological activities or responses in the host, as well as a key area of interaction between the host and the microbiome (42, 43). Intestinal microbes can metabolize a variety of dietary nutrients, such as indigestible carbohydrates and host metabolites (2). The relationship between dietary polyphenols and the prevention of human malignancies has been a research hotspot in the past few years. One of the reasons for investigating these compounds is that they have a protective effect on CRC (44). In terms of extraction techniques, it can be divided into extractable polyphenols (EPP) and non-extractable polyphenols (NEPP) (45). EPP includes low molecular weight compounds from monomers to decamers, which are soluble in organic solvents, such as methanol, ethanol, and ethyl acetate (46). EPP has a wide range of chemical structures, including hydrolyzed tannins, flavonoids, benzoic acid, hydroxycinnamic acid, stilbene, and others (46). EPP dissolve in the stomach and small intestine, where they can be absorbed at least partially through the small intestinal mucosa, which in turn produces metabolic and systemic effects. For example, dietary extractable proanthocyanidins (EPA) is partially hydrolyzed into epicatechin in the small intestine and absorbed (47, 48). Then a part is widely conjugated in the liver (49), while the other part, together with dietary fiber and indigestible substrates, reaches the colon where the intestinal flora catabolizes to produce metabolites, such as phenylacetic acid, phenylpropionic acid, and phenylbutyric acid (50, 51).
Since NEPP reach the colon almost intact, which is the primary site of their metabolic transformation, it is clear that their primary health trait is gastrointestinal health (52, 53). NEPP are a concentrated tannin and hydrolyzable phenolic high molecular weight compound or polyphenols related to fiber and protein in the diet, which remain in water or organic extracts (54). After being ingested, NEPP are not released from the food matrix through chewing, the acidic environment in the stomach, or the action of digestive enzymes (52). NEPP are not bio-accessible in the small intestine. They reach the colon from the gastrointestinal tract as an insoluble substrate, where they release single polyphenols and different bioavailable metabolites through the workings of the bacterial community in the colon (46). In other words, NEPP can provide absorbable and bioactive metabolites for the intestinal tract once they are fermented by colonic microorganisms. The study showed that 10 ml/kg of proanthocyanidins-rich dietary fiber could induce changes in the expression of tumor suppressor genes and proto-oncogenes genes and affect lipid synthesis, energy metabolism, cell cycle, and apoptosis, which suggested that polyphenols might help reduce the risk of CRC (55).
As a source of biological compounds, natural products have a great potential value because they can reduce/inhibit the risk and development of cancer, which is very useful for medical applications (56). CRC is caused by a series of pathological changes in the transformation of the normal colonic epithelium into invasive carcinoma. The development of CRC spans a multi-step process of 10–15 years, which provides opportunities for its early detection and prevention. Dietary polyphenols have been shown to have anticancer effects by affecting epigenetics (57), inflammation (58), mRNA expression (59–61), and gut microbes (14). The continuous discovery and mining of polyphenols have opened up new directions for the prevention and treatment of cancer. There are now polyphenols and derivatives used in clinical anti-colorectal cancer (62). For instance, silymarin could inhibit Wnt signal transduction in CRC cells to reduce the expression of hydro-catenin and TCF4, thus achieving the apoptosis and proliferation effects on cancer cells. Studies have revealed that EGCG (63), resveratrol (64), and curcumin (65) possess the properties of apoptosis, anti-proliferation, anti-angiogenesis, and cell cycle arrest in the development of CRC.
Traditionally, cancer is regarded as a series of diseases driven by progressive genetic abnormalities, including tumor mutations (suppressor genes and carcinogenic genes), and chromosomal abnormalities (66). However, cancer is also a disease driven by “epigenetic changes,” and they are derived from normal cells, which mediate mechanisms that do not affect the original DNA sequence but regulate heritable changes in gene activity through meiosis and mitosis (67, 68). Epigenetic changes include DNA methylation, histone modification, microRNA, and nucleosome positioning (69). Epigenetic driver genes are thought to be involved in the early stages of the tumor, and DNA methylation changes are a hallmark of CRC (70).
Abnormal DNA methylation is often detected in gastrointestinal tumors and may therefore be used for the screening, diagnosis, prognosis, and prediction of colorectal cancer (71). DNA methylation is a eukaryotic genomic modification event that occurs at the fifth carbon site of the cytosine residue in CpG dinucleotide, which is essential for mammalian development. Polyphenols in the diet may reduce the risk of colon cancer by altering DNA methylation. EGCG, one of the major polyphenols in green tea, has been shown to inhibit DNA methyltransferase (DNMT) activity and to reactivate methylation-silencing genes in cancer cells. In addition, EGCG can activate methylation-silencing genes in a variety of cancer cells, such as HT-29, KYSE 150, and PC3, indicating that EGCG may prevent cancer by reversing the silencing of related genes (72). The gallic acid portion of the D ring in EGCG can interact with the cytosine active site on the DNMT enzyme, which may be one of the reasons EGCG can be an effective DNMT inhibitor (57). Moreover, the combined application of EGCG and sodium butyrate promoted colorectal cancer cell apoptosis and induced cell cycle arrest and DNA damage (73). Curcumin-induced DNA methylation in CRC cell lines (HCT116, HT29, and RKO) was time-dependent, and curcumin treatment results in methylation changes at partial methylation sites rather than CpG sites that are completely methylated (74). Polyphenols inhibit DNA methylation and DNMT activity in two ways: reducing intracellular S-adenosylmethionine (SAM) concentration and non-competitively inhibiting DNMT activity or inserting DNMT binding vesicles to form a competitive inhibition (75).
MiRNAs are a new class of small molecule ncRNAs that can be combined by miRNA targets of various signal pathways, usually as a modulator of gene expression (76). MiRNA is dysregulated in cancer cells through the epigenetic mechanism in cancer cells/colonic tumor tissue—overexpressed and underexpressed miRNA (77, 78), suggesting that miRNAs are associated with cancer and may have a vital role in diagnosis, prediction, and as therapeutic targets for CRC (79). There is increasing evidence that polyphenols play an anti-tumor role by regulating miRNA and its target protein in different cancer cells. The study demonstrated that resveratrol had protective properties on colon cancer SW480. The results showed that resveratrol treatment reduced the key effector transforming growth factor β (TGF-β) signal pathway, tumor suppressor PTEN and PDCD4, and Dicer-1, which were responsible for the enzyme process of transforming pre-miRNA into mature miRNA, while enhancing the expression of miR-663, a tumor suppressor microRNA targeting TGF β 1 transcript (80). Moreover, the combination of resveratrol and quercetin (RQ) reduced the production of ROS and improved the antioxidant effect of in the HT-29 cell line. In addition, RQ reduced the expression of Sp1, Sp3, and Sp4 mRNA and reduced microRNA-27a (miR-27a) and the induced Sp-inhibitor zinc finger protein ZBTB10, which indicated that the interaction between RQ and miR-27a- ZBTB10 axis played a role in Sp down-regulation. These indicated that polyphenols could be used as natural anticancer agents (81).
Intestinal microbes play a key role in the integration of environmental factors with host physiology and metabolism, which may affect the occurrence and development of CRC through the changes of immune and metabolic signals mediated by metabolism, including the balance of intestinal cell proliferation and death, and the changes of host metabolic activity (82, 83). CRC is closely related to microbial changes near the mucosa where the tumor is located, and part of this ecological disorder is characterized by the expansion of bacterial taxa, while the dominant species in the development of CRC is still unclear (84, 85). Although the available epidemiological evidence is limited, relatively consistent research data indicates that the number of butyrate-producing bacteria in CRC has decreased while Fusobacterium nucleatum (Fn) and Bacteroides fragilis have increased (86, 87). Moreover, a retrospective analysis of 13,096 adult bacteremia without a history of cancer indicates that the late diagnosis of CRC may be related to Bacteroides fragilis and Streptococcus gallolyticus, which may be related to intestinal disorders and barrier dysfunction that cause these bacteria to enter the bloodstream (88). As mentioned above, NEPP have bioavailability in the colon, where polyphenols and gut microbes form a two-way interaction in which polyphenols regulate the composition and diversity of intestinal microbes. At the same time, intestinal microorganisms decompose the ingested polyphenols and then release more active and easily absorbed metabolites than natural polyphenols, which may provide more possibilities for preventing CRC (89, 90). The correlation analysis of the gram-negative anaerobic commensal bacteria Fn and human CRC genome showed that in 99 CRC patients, fluorescence in situ hybridization was used to connect CRC and Fn amplification. It was found that the Clostridium sequence was associated with lymph node metastasis (91, 92). Tea polyphenols have different degrees of antibacterial activity, which may hinder the bacterial cell membrane and the chelation of iron. The study showed that EGCG and theaflavin could reduce the expression of two virulence factors (hemolysis and hydrogen sulfide) expressed by FN and inhibit the adhesion of FN to oral epithelial cells and matrix proteins (93). Mucus-associated Escherichia coli (E. coli) is more common in CRC tissues, and their abundance is associated with tumor stage and prognosis (94). Pathogenic E. coli producing pathogenic colibactin is more common in terminal illness, and the CRC-related E. coli could significantly increase the number of polyps in ApcMin/+ mice, indicating that E. coli may promote the occurrence of tumors (95). Red wine polyphenols could be dose-dependent to prevent intestinal cytotoxicity to HT-29 caused by E. coli 270 (96). How microbial diversity suppresses tumorigenesis and which species or their interactions are associated with CRC development remain to be further explored. In addition, it is challenging to explore the effect of the abundance of specific strains in commensal bacteria on the development of CRC experimentally (87).
The intestine is a multi-functional organ whose functions include digestion/absorption, barrier function, and recognition of external stimuli and signal transduction (97). Chronic inflammation occurs when the gut is constantly damaged by external stress (such as food, bacteria, and environmental chemicals). Inflammation is a non-specific immune response that protects the body from myriad biological and chemical threats in the surrounding environment, relying on the dense cellular and molecular control mechanisms of the intestinal mucosa (98). Polyphenols may play an anticancer role by regulating inflammatory pathways through key signal transduction pathways and thus affect the course of the disease. The key signaling pathways include NFκB and signal transducer and activator of transcription (STAT3), as well as phosphatidylinositol 3-kinase (PI3K) and cyclo-oxygenase (COX) (99). Curcumin mediates its chemotherapy by regulating NFκB transcription factor expression, inhibiting NFκB-regulated gene products, such as COX-2, adhesion molecule, matrix metalloproteinases (MMPs), iNOS, Bcl-2, and TNF, and regulating cyclin expression (cyclin D1 and p21) (100). The upregulation of STAT3 can attract chemokines from immune and inflammatory cells to induce tumor-related inflammation (101). EGCG could inhibit the phosphorylation of STAT3 (102), while resveratrol inhibits IL-6-induced intercellular cell adhesion molecule-1 (ICAM-1) gene expression by interfering with STAT3 phosphorylation (103). The PI3K signaling pathway plays a vital role in tumorigenesis, which includes inhibiting apoptosis, increasing cell proliferation and growth, and reducing cell cycle arrest (104, 105). The study has shown that curcumin inhibited the expression of oncogene MDM2 through the PI3K signaling pathway, which is related to cell survival and proliferation (106). Moreover, cyclo-oxygenase-2 (COX2) is an inducer of prostaglandin synthesis, and its tumor-promoting effect is mediated by its main end product, prostaglandin PGE2 (107). COX2 has displayed overexpression during the development of CRCs. The study of pomegranate extract could significantly downregulate the expression of COX-2 protein and the constitutive expression and phosphorylation of NF-kB p65 in HT-29 cells (108).
Due to uncontrolled cell division in cancer, the development of cancer is related to many factors, including diagnosis, drug-induced cytotoxicity, chemotherapy resistance, and prognosis. The development cycle is long, affecting a large number of people. Inhibiting DNMT activity and regulating mRNA changes and intestinal changes through natural compounds may be powerful approaches to cancer prevention by regulating multiple cell functions to destroy multi-stage cancer diseases, thereby mediating multiple anticancer pathways. Polyphenols are widely found in food and beverages, and the combination of polyphenols and traditional drugs can improve therapeutic effects, reduce the drug resistance of tumor cells, and reduce the toxicity of chemotherapy drugs. The influence of polyphenols on the intestinal microbiota has been widely recognized, but their changes and mechanism of action in the progress of CRC need a lot of research and supplement. Substantial evidence proves that polyphenols are among the best choices for the prevention and treatment of CRC. On the concentration and bioavailability of polyphenols, whether a moderate-to-high dose will have a beneficial effect on health without affecting gut barrier integrity and gut microbe remains unclear. Whether the rich and complex polyphenols in the daily diet will affect the therapeutic effect of polyphenols is also unclear. That may be further elucidated with the development of techniques and research in the field of omics.
SD and SX: writing—original draft preparation. JF and HJ: writing—review and editing. All authors contributed to manuscript revision, read, and approved the submitted version.
This study was supported by National Natural Science Foundation of China (No. 31672457), Ministry of Agricultural of the People's Republic of China (2015-Z64 and 2016-X47), and Hunan Provincial Science and Technology Department (2016NK2101, 2017NK2322, 2016WK2008, and 2016TP2005).
The authors declare that the research was conducted in the absence of any commercial or financial relationships that could be construed as a potential conflict of interest.
1. Priego S, Feddi F, Ferrer P, Mena S, Benlloch M, Ortega A, et al. Natural polyphenols facilitate elimination of HT-29 colorectal cancer xenografts by chemoradiotherapy: a Bcl-2- and superoxide dismutase 2-dependent mechanism. Mol Cancer Ther. (2008) 7:3330–42. doi: 10.1158/1535-7163.MCT-08-0363
2. Wong SH, Yu J. Gut microbiota in colorectal cancer: mechanisms of action and clinical applications. Nat Rev Gastroenterol Hepatol. (2019) 16:690–704. doi: 10.1038/s41575-019-0209-8
3. Lichtenstein P, Holm NV, Verkasalo PK, Iliadou A, Kaprio J, Koskenvuo M, et al. Environmental and heritable factors in the causation of cancer–analyses of cohorts of twins from Sweden, Denmark, and Finland. N Engl J Med. (2000) 343:78–85. doi: 10.1056/NEJM200007133430201
4. Bray F, Ferlay J, Soerjomataram I, Siegel RL, Torre LA, Jemal A. Global cancer statistics 2018: GLOBOCAN estimates of incidence and mortality worldwide for 36 cancers in 185 countries. CA Cancer J Clin. (2018) 68:394–424. doi: 10.3322/caac.21492
5. Foulkes WD. Inherited susceptibility to common cancers. N Engl J Med. (2008) 359:2143–53. doi: 10.1056/NEJMra0802968
6. Czene K, Lichtenstein P, Hemminki K. Environmental and heritable causes of cancer among 9.6 million individuals in the Swedish family-cancer database. Int J Cancer. (2002) 99:260–6. doi: 10.1002/ijc.10332
7. Hostanska K, Jurgenliemk G, Abel G, Nahrstedt A, Saller R. Willow bark extract (BNO1455) and its fractions suppress growth and induce apoptosis in human colon and lung cancer cells. Cancer Detect Prev. (2007) 31:129–39. doi: 10.1016/j.cdp.2007.03.001
8. Espin JC, Garcia-Conesa MT, Tomas-Barberan FA. Nutraceuticals: facts and fiction. Phytochemistry. (2007) 68:2986–3008. doi: 10.1016/j.phytochem.2007.09.014
9. D'Archivio M, Santangelo C, Scazzocchio B, Varì R, Filesi C, Masella R, et al. Modulatory effects of polyphenols on apoptosis induction: relevance for cancer prevention. Int J Mol Sci. (2008) 9:213–28. doi: 10.3390/ijms9030213
10. Yang CS, Wang X, Lu G, Picinich SC. Cancer prevention by tea: animal studies, molecular mechanisms and human relevance. Nat Rev Cancer. (2009) 9:429–39. doi: 10.1038/nrc2641
11. Elshaer M, Chen Y, Wang XJ, Tang X. Resveratrol: an overview of its anti-cancer mechanisms. Life Sci. (2018) 207:340–9. doi: 10.1016/j.lfs.2018.06.028
12. Duthie SJ. Berry phytochemicals, genomic stability and cancer: evidence for chemoprotection at several stages in the carcinogenic process. Mol Nutr Food Res. (2007) 51:665–74. doi: 10.1002/mnfr.200600257
13. Han Y, Huang M, Li L, Cai X, Gao Z, Li F, et al. Non-extractable polyphenols from cranberries: potential anti-inflammation and anti-colon-cancer agents. Food Func. (2019) 10:7714–23. doi: 10.1039/C9FO01536A
14. Naumovski N, Panagiotakos DB, D'Cunha NM. Untangling the 2-way relationship between red wine polyphenols and gut microbiota. Gastroenterology. (2020) 158:48–51. doi: 10.1053/j.gastro.2019.10.015
15. Yahfoufi N, Alsadi N, Jambi M, Matar C. The immunomodulatory and anti-inflammatory role of polyphenols. Nutrients. (2018) 10:1618. doi: 10.3390/nu10111618
16. Goni I, Hernandez-Galiot A. Intake of nutrient and non-nutrient dietary antioxidants. Contribution of macromolecular antioxidant polyphenols in an elderly mediterranean population. Nutrients. (2019) 11:2165. doi: 10.3390/nu11092165
17. Adriouch S, Lampure A, Nechba A, Baudry J, Assmann K, Kesse-Guyot E, et al. Prospective association between total and specific dietary polyphenol intakes and cardiovascular disease risk in the Nutrinet-Santé French cohort. Nutrients. (2018) 10:1587. doi: 10.3390/nu10111587
18. Yamada S, Tsukamoto S, Huang Y, Makio A, Kumazoe M, Yamashita S, et al. Epigallocatechin-3-O-gallate up-regulates microRNA-let-7b expression by activating 67-kDa laminin receptor signaling in melanoma cells. Sci Rep. (2016) 6:19225. doi: 10.1038/srep19225
19. Serra G, Incani A, Serreli G, Porru L, Melis MP, Tuberoso CIG, et al. Olive oil polyphenols reduce oxysterols -induced redox imbalance and pro-inflammatory response in intestinal cells. Redox Biol. (2018) 17:348–54. doi: 10.1016/j.redox.2018.05.006
20. Noratto G, Martino HS, Simbo S, Byrne D, Mertens-Talcott SU. Consumption of polyphenol-rich peach and plum juice prevents risk factors for obesity-related metabolic disorders and cardiovascular disease in zucker rats. J Nutr Biochem. (2015) 26:633–41. doi: 10.1016/j.jnutbio.2014.12.014
21. Karasawa K, Uzuhashi Y, Hirota M, Otani H. A matured fruit extract of date palm tree (Phoenix dactylifera L.) stimulates the cellular immune system in mice. J Agric Food Chem. (2011) 59:11287–93. doi: 10.1021/jf2029225
22. Li XW, Chen HP, He YY, Chen WL, Chen JW, Gao L, et al. Effects of rich-polyphenols extract of dendrobium loddigesii on anti-diabetic, anti-inflammatory, anti-oxidant, and gut microbiota modulation in db/db mice. Molecules. (2018) 23:3245. doi: 10.3390/molecules23123245
23. Nakajima VM, Moala T, Caria C, Moura CS, Amaya-Farfan J, Gambero A, et al. Biotransformed citrus extract as a source of anti-inflammatory polyphenols: effects in macrophages and adipocytes. Food Res Int. (2017) 97:37–44. doi: 10.1016/j.foodres.2017.03.034
24. Li Y, Zhang T. Targeting cancer stem cells by curcumin and clinical applications. Cancer Lett. (2014) 346:197–205. doi: 10.1016/j.canlet.2014.01.012
25. Aravindan S, Ramraj SK, Somasundaram ST, Herman TS, Aravindan N. Polyphenols from marine brown algae target radiotherapy-coordinated EMT and stemness-maintenance in residual pancreatic cancer. Stem Cell Res Ther. (2015) 6:182. doi: 10.1186/s13287-015-0173-3
26. Hao X, Xiao H, Ju J, Lee MJ, Lambert JD, Yang CS. Green tea polyphenols inhibit colorectal tumorigenesis in azoxymethane-treated F344 rats. Nutr Cancer. (2017) 69:623–31. doi: 10.1080/01635581.2017.1295088
27. Lu Y, Shan S, Li H, Shi J, Zhang X, Li Z. Reversal effects of bound polyphenol from foxtail millet bran on multidrug resistance in human HCT-8/Fu colorectal cancer cell. J Agric Food Chem. (2018) 66:5190–9. doi: 10.1021/acs.jafc.8b01659
28. Gavrilas LI, Cruceriu D, Ionescu C, Miere D, Balacescu O. Pro-apoptotic genes as new targets for single and combinatorial treatments with resveratrol and curcumin in colorectal cancer. Food Func. (2019) 10:3717–26. doi: 10.1039/C9FO01014A
29. Jin H, Tan X, Liu X, Ding Y. The study of effect of tea polyphenols on microsatellite instability colorectal cancer and its molecular mechanism. Int J Colorectal Dis. (2010) 25:1407–15. doi: 10.1007/s00384-010-1047-x
30. Chen Y, Wu Y, Du M, Chu H, Zhu L, Tong N, et al. An inverse association between tea consumption and colorectal cancer risk. Oncotarget. (2017) 8:37367–76. doi: 10.18632/oncotarget.16959
31. Li D, Chen Y, Huang Y, Zhang L, Yang J, Xu X, et al. Study on the anti-tumor ability of niaowangzhong green tea. J Food Biochem. (2017) 41:e12305. doi: 10.1111/jfbc.12305
32. Ellis H, Mahadevan V. Anatomy of the caecum, appendix and colon. Surgery. (2014) 32:155–8. doi: 10.1016/j.mpsur.2014.02.001
33. Ponz de Leon M, Di Gregorio C. Pathology of colorectal cancer. Dig Liver Dis. (2001) 33:372–88. doi: 10.1016/S1590-8658(01)80095-5
34. Santos IS Ponte BM Boonme P Silva AM Souto EB. Nanoencapsulation of polyphenols for protective effect against colon-rectal cancer. Biotechnol Adv. (2013) 31:514–23. doi: 10.1016/j.biotechadv.2012.08.005
35. Shehzad A, Wahid F, Lee YS. Curcumin in cancer chemoprevention: molecular targets, pharmacokinetics, bioavailability, and clinical trials. Arch Pharm. (2010) 343:489–99. doi: 10.1002/ardp.200900319
36. Boghossian S, Hawash A. Chemoprevention in colorectal cancer–where we stand and what we have learned from twenty year's experience. Surgeon. (2012) 10:43–52. doi: 10.1016/j.surge.2011.07.003
37. Sharma RA, Manson MM, Gescher A, Steward WP. Colorectal cancer chemoprevention: biochemical targets and clinical development of promising agents. Eur J Cancer. (2001) 37:12–22. doi: 10.1016/S0959-8049(00)00326-9
38. Collins D, Hogan AM, Winter DC. Microbial and viral pathogens in colorectal cancer. Lancet Oncol. (2011) 12:504–12. doi: 10.1016/S1470-2045(10)70186-8
39. Soreide K, Nedrebo BS, Knapp JC, Glomsaker TB, Soreide JA, Korner H. Evolving molecular classification by genomic and proteomic biomarkers in colorectal cancer: potential implications for the surgical oncologist. Surg Oncol. (2009) 18:31–50. doi: 10.1016/j.suronc.2008.06.006
40. Research WCRFAIfC. Continuous Update Project Report. Food, Nutrition, Physical Activity, and the Prevention of Colorectal Cancer. (2011). Available online at: https://www.wcrf.org/sites/default/files/Colorectal-Cancer-2011-Report.pdf
41. Sobiecki JG. Vegetarianism and colorectal cancer risk in a low-selenium environment: effect modification by selenium status? A possible factor contributing to the null results in British vegetarians. Eur J Nutr. (2017) 56:1819–32. doi: 10.1007/s00394-016-1364-0
42. Doll R, Peto R. The causes of cancer: quantitative estimates of avoidable risks of cancer in the United States today. J Natl Cancer Inst. (1981) 66:1191–308. doi: 10.1093/jnci/66.6.1192
43. Zhang FF, Cudhea F, Shan Z, Michaud DS, Imamura F, Eom H, et al. Preventable cancer burden associated with poor diet in the United States. JNCI Cancer Spectrum. (2019) 3:pkz034. doi: 10.1093/jncics/pkz034
44. Araujo JR, Goncalves P, Martel F. Chemopreventive effect of dietary polyphenols in colorectal cancer cell lines. Nutr Res. (2011) 31:77–87. doi: 10.1016/j.nutres.2011.01.006
45. Macagnan FT, da Silva LP, Hecktheuer LH. Dietary fibre: the scientific search for an ideal definition and methodology of analysis, and its physiological importance as a carrier of bioactive compounds. Food Res Int. (2016) 85:144–54. doi: 10.1016/j.foodres.2016.04.032
46. Saura-Calixto F. Concept and health-related properties of nonextractable polyphenols: the missing dietary polyphenols. J Agric Food Chem. (2012) 60:11195–200. doi: 10.1021/jf303758j
47. Baba S, Osakabe N, Natsume M, Terao J. Absorption and urinary excretion of procyanidin B2 [epicatechin-(4beta-8)-epicatechin] in rats. Free Radical Biol Med. (2002) 33:142–8. doi: 10.1016/S0891-5849(02)00871-7
48. Deprez S, Mila I, Huneau JF, Tome D, Scalbert A. Transport of proanthocyanidin dimer, trimer, and polymer across monolayers of human intestinal epithelial Caco-2 cells. Antioxid Redox Signal. (2001) 3:957–67. doi: 10.1089/152308601317203503
49. Scalbert A, Morand C, Manach C, Remesy C. Absorption and metabolism of polyphenols in the gut and impact on health. Biomed Pharmacother. (2002) 56:276–82. doi: 10.1016/S0753-3322(02)00205-6
50. Gonthier MP, Donovan JL, Texier O, Felgines C, Remesy C, Scalbert A. Metabolism of dietary procyanidins in rats. Free Radical Biol Med. (2003) 35:837–44. doi: 10.1016/S0891-5849(03)00394-0
51. Saura-Calixto F, Perez-Jimenez J, Tourino S, Serrano J, Fuguet E, Torres JL, et al. Proanthocyanidin metabolites associated with dietary fibre from in vitro colonic fermentation and proanthocyanidin metabolites in human plasma. Mol Nutr Food Res. (2010) 54:939–46. doi: 10.1002/mnfr.200900276
52. Perez-Jimenez J, Diaz-Rubio ME, Saura-Calixto F. Non-extractable polyphenols, a major dietary antioxidant: occurrence, metabolic fate and health effects. Nutr Res Rev. (2013) 26:118–29. doi: 10.1017/S0954422413000097
53. Larrosa M, Luceri C, Vivoli E, Pagliuca C, Lodovici M, Moneti G, et al. Polyphenol metabolites from colonic microbiota exert anti-inflammatory activity on different inflammation models. Mol Nutr Food Res. (2009) 53:1044–54. doi: 10.1002/mnfr.200800446
54. Perez-Jimenez J, Saura-Calixto F. Literature data may underestimate the actual antioxidant capacity of cereals. J Agric Food Chem. (2005) 53:5036–40. doi: 10.1021/jf050049u
55. Lizarraga D, Vinardell MP, Noe V, van Delft JH, Alcarraz-Vizan G, van Breda SG, et al. A lyophilized red grape pomace containing proanthocyanidin-rich dietary fiber induces genetic and metabolic alterations in colon mucosa of female C57BL/6J mice. J Nutr. (2011) 141:1597–604. doi: 10.3945/jn.110.133199
56. Nair HB, Sung B, Yadav VR, Kannappan R, Chaturvedi MM, Aggarwal BB. Delivery of antiinflammatory nutraceuticals by nanoparticles for the prevention and treatment of cancer. Biochem Pharmacol. (2010) 80:1833–43. doi: 10.1016/j.bcp.2010.07.021
57. Duthie SJ. Epigenetic modifications and human pathologies: cancer and CVD. Proc Nutr Soc. (2011) 70:47–56. doi: 10.1017/S0029665110003952
58. Marzocchella L, Fantini M, Benvenuto M, Masuelli L, Tresoldi I, Modesti A, et al. Dietary flavonoids: molecular mechanisms of action as anti- inflammatory agents. Recent Patents Inflam Allergy Drug Discov. (2011) 5:200–20. doi: 10.2174/187221311797264937
59. Yi J, Li S, Wang C, Cao N, Qu H, Cheng C, et al. Potential applications of polyphenols on main ncRNAs regulations as novel therapeutic strategy for cancer. Biomed Pharmacothe. (2019) 113:108703. doi: 10.1016/j.biopha.2019.108703
60. Li W, Yang W, Liu Y, Chen S, Chin S, Qi X, et al. MicroRNA-378 enhances inhibitory effect of curcumin on glioblastoma. Oncotarget. (2017) 8:73938–46. doi: 10.18632/oncotarget.17881
61. Bi Y, Shen W, Min M, Liu Y. MicroRNA-7 functions as a tumor-suppressor gene by regulating ILF2 in pancreatic carcinoma. Int J Mol Med. (2017) 39:900–6. doi: 10.3892/ijmm.2017.2894
62. Huang XM, Yang ZJ, Xie Q, Zhang ZK, Zhang H, Ma JY. Natural products for treating colorectal cancer: a mechanistic review. Biomed Pharmacother. (2019) 117:109142. doi: 10.1016/j.biopha.2019.109142
63. Stingl JC, Ettrich T, Muche R, Wiedom M, Brockmoller J, Seeringer A, et al. Protocol for minimizing the risk of metachronous adenomas of the colorectum with green tea extract (MIRACLE): a randomised controlled trial of green tea extract versus placebo for nutriprevention of metachronous colon adenomas in the elderly population. BMC Cancer. (2011) 11:360. doi: 10.1186/1471-2407-11-360
64. Fuggetta MP, Lanzilli G, Tricarico M, Cottarelli A, Falchetti R, Ravagnan G, et al. Effect of resveratrol on proliferation and telomerase activity of human colon cancer cells in vitro. J Exp Clin Cancer Res. (2006) 25:189–93. doi: 10.3892/ijo.28.3.641
65. Li YH, Niu YB, Sun Y, Zhang F, Liu CX, Fan L, et al. Role of phytochemicals in colorectal cancer prevention. World J Gastroenterol. (2015) 21:9262–72. doi: 10.3748/wjg.v21.i31.9262
66. Kluth M, Jung S, Habib O, Eshagzaiy M, Heinl A, Amschler N, et al. Deletion lengthening at chromosomes 6q and 16q targets multiple tumor suppressor genes and is associated with an increasingly poor prognosis in prostate cancer. Oncotarget. (2017) 8:108923–35. doi: 10.18632/oncotarget.22408
67. Feinberg AP, Ohlsson R, Henikoff S. The epigenetic progenitor origin of human cancer. Nat Rev Genet. (2006) 7:21–33. doi: 10.1038/nrg1748
68. Choong MK, Tsafnat G. Genetic and epigenetic biomarkers of colorectal cancer. Clin Gastroenterol Hepatol. (2012) 10:9–15. doi: 10.1016/j.cgh.2011.04.020
69. Cai L. Cadmium and its epigenetic effects. Curr Med Chem. (2012) 19:2611–20. doi: 10.2174/092986712800492913
70. Weisenberger DJ, Liang G, Lenz HJ. DNA methylation aberrancies delineate clinically distinct subsets of colorectal cancer and provide novel targets for epigenetic therapies. Oncogene. (2018) 37:566–77. doi: 10.1038/onc.2017.374
71. Hashimoto Y, Zumwalt TJ, Goel A. DNA methylation patterns as noninvasive biomarkers and targets of epigenetic therapies in colorectal cancer. Epigenomics. (2016) 8:685–703. doi: 10.2217/epi-2015-0013
72. Fang MZ, Wang Y, Ai N, Hou Z, Sun Y, Lu H, et al. Tea polyphenol (-)-epigallocatechin-3-gallate inhibits DNA methyltransferase and reactivates methylation-silenced genes in cancer cell lines. Cancer Res. (2003) 63:7563–70.
73. Saldanha SN, Kala R, Tollefsbol TO. Molecular mechanisms for inhibition of colon cancer cells by combined epigenetic-modulating epigallocatechin gallate and sodium butyrate. Exp Cell Res. (2014) 324:40–53. doi: 10.1016/j.yexcr.2014.01.024
74. Link A, Balaguer F, Shen Y, Lozano JJ, Leung HC, Boland CR, et al. Curcumin modulates DNA methylation in colorectal cancer cells. PLoS ONE. (2013) 8:e57709. doi: 10.1371/journal.pone.0057709
75. Fang M, Chen D, Yang CS. Dietary polyphenols may affect DNA methylation. J Nutr. (2007) 137(Suppl. 1):223–8s. doi: 10.1093/jn/137.1.223S
76. Jafri MA, Al-Qahtani MH, Shay JW. Role of miRNAs in human cancer metastasis: Implications for therapeutic intervention. Semin Cancer Biol. (2017) 44:117–31. doi: 10.1016/j.semcancer.2017.02.004
77. Schetter AJ, Leung SY, Sohn JJ, Zanetti KA, Bowman ED, Yanaihara N, et al. MicroRNA expression profiles associated with prognosis and therapeutic outcome in colon adenocarcinoma. JAMA. (2008) 299:425–36. doi: 10.1001/jama.299.4.425
78. Slaby O, Svoboda M, Fabian P, Smerdova T, Knoflickova D, Bednarikova M, et al. Altered expression of miR-21, miR-31, miR-143 and miR-145 is related to clinicopathologic features of colorectal cancer. Oncology. (2007) 72:397–402. doi: 10.1159/000113489
79. Gu HF, Mao XY, Du M. Prevention of breast cancer by dietary polyphenols-role of cancer stem cells. Crit Rev Food Sci Nutr. (2020) 60:810–25. doi: 10.1080/10408398.2018.1551778
80. Tili E, Michaille JJ, Alder H, Volinia S, Delmas D, Latruffe N, et al. Resveratrol modulates the levels of microRNAs targeting genes encoding tumor-suppressors and effectors of TGFbeta signaling pathway in SW480 cells. Biochem Pharmacol. (2010) 80:2057–65. doi: 10.1016/j.bcp.2010.07.003
81. Del Follo-Martinez A, Banerjee N, Li X, Safe S, Mertens-Talcott S. Resveratrol and quercetin in combination have anticancer activity in colon cancer cells and repress oncogenic microRNA-27a. Nutr Cancer. (2013) 65:494–504. doi: 10.1080/01635581.2012.725194
82. Kau AL, Ahern PP, Griffin NW, Goodman AL, Gordon JI. Human nutrition, the gut microbiome and the immune system. Nature. (2011) 474:327–36. doi: 10.1038/nature10213
84. Nakatsu G, Li X, Zhou H, Sheng J, Wong SH, Wu WK, et al. Gut mucosal microbiome across stages of colorectal carcinogenesis. Nat Commun. (2015) 6:8727. doi: 10.1038/ncomms9727
85. Yazici C, Wolf PG, Kim H, Cross TL, Vermillion K, Carroll T, et al. Race-dependent association of sulfidogenic bacteria with colorectal cancer. Gut. (2017) 66:1983–94. doi: 10.1136/gutjnl-2016-313321
86. Sears CL, Garrett WS. Microbes, microbiota, and colon cancer. Cell Host Microbe. (2014) 15:317–28. doi: 10.1016/j.chom.2014.02.007
87. Tilg H, Adolph TE, Gerner RR, Moschen AR. The intestinal microbiota in colorectal cancer. Cancer Cell. (2018) 33:954–64. doi: 10.1016/j.ccell.2018.03.004
88. Kwong TNY, Wang X, Nakatsu G, Chow TC, Tipoe T, Dai RZW, et al. Association between bacteremia from specific microbes and subsequent diagnosis of colorectal cancer. Gastroenterology. (2018) 155:383–90.e8. doi: 10.1053/j.gastro.2018.04.028
89. Cueva C, Sanchez-Patan F, Monagas M, Walton GE, Gibson GR, Martin-Alvarez PJ, et al. In vitro fermentation of grape seed flavan-3-ol fractions by human faecal microbiota: changes in microbial groups and phenolic metabolites. FEMS Microbiol Ecol. (2013) 83:792–805. doi: 10.1111/1574-6941.12037
90. Munoz-Gonzalez I, Jimenez-Giron A, Martin-Alvarez PJ, Bartolome B, Moreno-Arribas MV. Profiling of microbial-derived phenolic metabolites in human feces after moderate red wine intake. J Agric Food Chem. (2013) 61:9470–9. doi: 10.1021/jf4025135
91. Castellarin M, Warren RL, Freeman JD, Dreolini L, Krzywinski M, Strauss J, et al. Fusobacterium nucleatum infection is prevalent in human colorectal carcinoma. Genome Res. (2012) 22:299–306. doi: 10.1101/gr.126516.111
92. Kostic AD, Gevers D, Pedamallu CS, Michaud M, Duke F, Earl AM, et al. Genomic analysis identifies association of Fusobacterium with colorectal carcinoma. Genome Res. (2012) 22:292–8. doi: 10.1101/gr.126573.111
93. Ben Lagha A, Haas B, Grenier D. Tea polyphenols inhibit the growth and virulence properties of Fusobacterium nucleatum. Sci Rep. (2017) 7:44815. doi: 10.1038/srep44815
94. Cougnoux A, Dalmasso G, Martinez R, Buc E, Delmas J, Gibold L, et al. Bacterial genotoxin colibactin promotes colon tumour growth by inducing a senescence-associated secretory phenotype. Gut. (2014) 63:1932–42. doi: 10.1136/gutjnl-2013-305257
95. Veziant J, Gagniere J, Jouberton E, Bonnin V, Sauvanet P, Pezet D, et al. Association of colorectal cancer with pathogenic Escherichia coli: focus on mechanisms using optical imaging. World J Clin Oncol. (2016) 7:293–301. doi: 10.5306/wjco.v7.i3.293
96. Nunes C, Figueiredo R, Laranjinha J, da Silva GJ. Intestinal cytotoxicity induced by Escherichia coli is fully prevented by red wine polyphenol extract: mechanistic insights in epithelial cells. Chem Biol Interact. (2019) 310:108711. doi: 10.1016/j.cbi.2019.06.024
97. Martin DA, Bolling BW. A review of the efficacy of dietary polyphenols in experimental models of inflammatory bowel diseases. Food Func. (2015) 6:1773–86. doi: 10.1039/C5FO00202H
98. Fiocchi C. More answers and more questions in inflammatory bowel disease. Curr Opin Gastroenterol. (2003) 19:325–6. doi: 10.1097/00001574-200307000-00001
99. Little CH, Combet E, McMillan DC, Horgan PG, Roxburgh CS. The role of dietary polyphenols in the moderation of the inflammatory response in early stage colorectal cancer. Crit Rev Food Sci Nutr. (2017) 57:2310–20. doi: 10.1080/10408398.2014.997866
100. Shishodia S, Sethi G, Aggarwal BB. Curcumin: getting back to the roots. Ann N Y Acad Sci. (2005) 1056:206–17. doi: 10.1196/annals.1352.010
101. Yu H, Pardoll D, Jove R. STATs in cancer inflammation and immunity: a leading role for STAT3. Nat Rev Cancer. (2009) 9:798–809. doi: 10.1038/nrc2734
102. Masuda M, Suzui M, Weinstein IB. Effects of epigallocatechin-3-gallate on growth, epidermal growth factor receptor signaling pathways, gene expression, and chemosensitivity in human head and neck squamous cell carcinoma cell lines. Clin Cancer Res. (2001) 7:4220–9.
103. Wung BS, Hsu MC, Wu CC, Hsieh CW. Resveratrol suppresses IL-6-induced ICAM-1 gene expression in endothelial cells: effects on the inhibition of STAT3 phosphorylation. Life Sci. (2005) 78:389–97. doi: 10.1016/j.lfs.2005.04.052
104. Samuels Y, Wang Z, Bardelli A, Silliman N, Ptak J, Szabo S, et al. High frequency of mutations of the PIK3CA gene in human cancers. Science. (2004) 304:554. doi: 10.1126/science.1096502
105. Le NH, Franken P, Fodde R. Tumour-stroma interactions in colorectal cancer: converging on beta-catenin activation and cancer stemness. Br J Cancer. (2008) 98:1886–93. doi: 10.1038/sj.bjc.6604401
106. Li M, Zhang Z, Hill DL, Wang H, Zhang R. Curcumin, a dietary component, has anticancer, chemosensitization, and radiosensitization effects by down-regulating the MDM2 oncogene through the PI3K/mTOR/ETS2 pathway. Cancer Res. (2007) 67:1988–96. doi: 10.1158/0008-5472.CAN-06-3066
107. Hull M, Langman M. Differential expression of cyclooxygenase 2 in human colorectal cancer. Gut. (2000) 47:154. doi: 10.1136/gut.47.1.154
Keywords: polyphenols, colorectal cancer, intestinal inflammation, epigenetics, microbe
Citation: Ding S, Xu S, Fang J and Jiang H (2020) The Protective Effect of Polyphenols for Colorectal Cancer. Front. Immunol. 11:1407. doi: 10.3389/fimmu.2020.01407
Received: 26 April 2020; Accepted: 01 June 2020;
Published: 10 July 2020.
Edited by:
Guan Yang, University of Florida, United StatesReviewed by:
Shihai Zhang, South China Agricultural University, ChinaCopyright © 2020 Ding, Xu, Fang and Jiang. This is an open-access article distributed under the terms of the Creative Commons Attribution License (CC BY). The use, distribution or reproduction in other forums is permitted, provided the original author(s) and the copyright owner(s) are credited and that the original publication in this journal is cited, in accordance with accepted academic practice. No use, distribution or reproduction is permitted which does not comply with these terms.
*Correspondence: Jun Fang, ZmFuZ2p1bjE5NzNAaHVuYXUuZWR1LmNu; Hongmei Jiang, amhtbmRjbkBodW5hdS5lZHUuY24=
Disclaimer: All claims expressed in this article are solely those of the authors and do not necessarily represent those of their affiliated organizations, or those of the publisher, the editors and the reviewers. Any product that may be evaluated in this article or claim that may be made by its manufacturer is not guaranteed or endorsed by the publisher.
Research integrity at Frontiers
Learn more about the work of our research integrity team to safeguard the quality of each article we publish.