- 1Nephrology Division, Assaf-Harofeh (Shamir) Medical Center, Be'er Ya'akov, Israel
- 2Sackler School of Medicine, Tel-Aviv University, Tel-Aviv, Israel
There is growing evidence concerning the potential use of mesenchymal stromal cells (MSCs) for different tissue injuries. Initially, the intended physiological use of MSCs was due to their ability to differentiate and replace damaged cells. However, MSCs have multiple effects, including being able to significantly modulate immunological responses. MSCs are currently being tested for neurodegenerative diseases, graft vs. host disease, kidney injury, and other chronic unremitting tissue damage. Using MSCs in acute tissue damage is only now being studied. Acute kidney injury (AKI) is a common cause of morbidity and mortality. After the primary insult, overactivation of the immune system culminates in additional secondary potentially permanent kidney damage. MSCs have the potential to ameliorate the secondary damage, and recent studies have shed important light on their mechanisms of action. This article summarizes the basics of MSCs therapy, the newly discovered mechanisms of action, and their potential application in the setting of AKI.
Introduction
Acute kidney injury (AKI) is a syndrome of rapid renal function deterioration over a period of hours or days (1). AKI is a common cause of morbidity and mortality, complicating 20% of hospitalized patients, half of them needing renal replacement therapy (2). This severe form of AKI is related to a 50% increase in mortality among other devastating long-term consequences, including end-stage renal disease (ESRD), and dialysis dependence (2, 3).
The etiologies of AKI are varied and are usually divided into pre-, intra-, and post-renal categories (4). The most common category is prerenal, secondary to hypoperfusion of the kidney. Hypoperfusion of the kidney can be caused by reduced effective blood volume secondary to hemorrhage, cardiac insufficiency, and/or third spacing of fluids (4). Intrarenal AKI is caused by intrinsic injury to the renal tissue, with the most common injury being acute tubular necrosis secondary to ischemia–reperfusion injury (IRI) (5–7). Other intrinsic renal causes include glomerular pathologies such as glomerulonephritis and interstitial and vascular injury (4). Postrenal AKI occurs from obstruction of the ureters, bladder outlet, or urethra (4). Irrespective of the primary cause, an intrarenal inflammatory cascade is activated following the acute kidney injury, which if not quickly controlled, culminates in additional renal damage and irreversible renal fibrosis (detailed below).
In the last decade, cumulative evidence has shown the significant role that overactivated immune responses play in the development of AKI (8). This understanding paved the way to new therapeutic strategies for this relatively common and life-threatening acute kidney condition. Unfortunately, despite the progress in our understanding of AKI biology, treatment options for AKI in the daily clinical setting are still limited (1, 3, 5). While dialysis can be relatively effective in handling the hazardous electrolytes and volume complications as a supportive therapy, there is a need for a treatment that can counteract the pathological cascade that can culminate in irreversible loss of renal tissue (1, 5).
The Immune Response to Acute Kidney Injury
The immune system plays a crucial role in AKI mechanisms with involvement of both the innate and adaptive immune system branches (9). Regarding the innate immune system, cytokines serve as major mediators with both increased production of cytokines and reduced clearance being reported during AKI (10). Interleukin (IL)-6, IL-8, and tumor necrosis factor (TNF)-α are usually elevated and are related to endothelial dysfunction and tubular injury (11). Conversely, IL-10 has an ameliorating effect by promoting immune tolerance (12). In the AKI setting, growth factors also play a role by regulating inflammation and programmed cell death. When administrated early after the acute insult, growth factors such as the epidermal growth factor (EGF), insulin-like growth factor (IGF), and fibroblast growth factor (FGF) can promote renal repair and renal function restoration in animal models (13).
The complement system, a part of the innate immune system, also has an important role in the pathogenesis of renal injury and is involved in glomerular, tubulointerstitial, and vascular kidney injuries (14). The final common pathway of the complement system is the membrane attack complex that induces direct cellular damage and causes activation and migration of neutrophils, which further amplifies the injury (15). Suppressing the complement system in AKI has shown promising results in preclinical studies (16).
The cellular response to AKI includes both pro- and anti-inflammatory characteristics. Dendritic cells, monocytes/macrophages, neutrophils, T lymphocytes, and B lymphocytes are all involved in AKI and can be detected as early as 1 h after the acute insult (17). The involvement of these cells can directly and indirectly induce apoptosis of the renal tubular cells (17). Neutrophils recruited to the injured kidney cause vascular congestion that, together with the secreted cytotoxic compounds, including reactive oxygen species, further exacerbate tissue damage (11). M1 macrophages release chemokines, proinflammatory cytokines, and inducible nitric oxide synthase, which form peroxynitrites. These peroxynitrites have a vasoconstrictive effect, which can aggravate the ischemic and inflammatory damage (11). Lymphocytes enhance AKI by releasing IL-17, a proinflammatory cytokine that also increases vascular permeability (11, 17, 18). In contrast, M2 macrophages and regulatory T cells are essential for suppressing the overactivated inflammatory response and for regenerating damaged renal tissue and are detected while recovering from the acute insult (9).
The relation between the different arms of the immune system can either escalate or downgrade the final injury (11, 15). To steer the cells and factors toward a less devasting rout, new treatments are being investigated including the use of stem-cell therapy.
Mesenchymal Stromal Cells
Mesenchymal stromal cells (MSCs) are fibroblast-like multipotent cells that can differentiate into mesodermal-line cells including adipocytes, chondroblasts, osteoblasts, and renal tubular cells (19, 20). These cells exhibit self-renewal properties, with a potential to replace damaged cells (21). MSCs are defined by three main characteristics: (1) plastic adherent when maintained in standard culture conditions; (2) expression of CD105, CD73, and CD90, with no expression of other CDs that are not mesenchyme related [including CD45, CD34, CD14, or CD11b, CD79-α, or CD19 and human leukocyte antigen (HLA)-DR surface molecules]; and (3) the ability to differentiate into a mature mesoderm related cell line in vitro (22). Unlike embryonic stem cells, MSCs are found in many organs even in adults (20, 22, 23).
In the past two decades, MSCs from different origins are being used in different clinical trial settings (24). For example, bone-marrow-derived MSCs are used in children to treat graft-vs.-host disease, autologous marrow MSCs for heart disease (23), and both bone-marrow and adipose-derived MSCs are used in Crohn's-related enterocutaneous fistular disease (25). In the neurodegenerative field, MSCs are being studied in amyotrophic lateral sclerosis, multiple system atrophy, Parkinson's disease, Alzheimer's disease, and multiple sclerosis. While animal studies have been promising, clinical studies have demonstrated conflicting results (26, 27). The encouraging results obtained in the field of degenerative diseases can be related, among others, to the effect that MSCs have on the immune factors in these disease settings (26, 27).
The Biology of Mesenchymal Stromal Cells
MSCs can affect and be affected by other cells through different immune mediators. Cytokines, chemokines, and transcription factors can influence the differentiation of MSCs. Expression in MSCs of specific transcription factors, including Runx2, Sox9, PPARγ, MyoD, GATA4, and GATA6, promote their differentiation into specific cell lineage (20).
The primary rationale for using MSCs to rejuvenate damaged tissue was initially related to their ability to differentiate into the damaged tissue-related cells. Following IRI, MSCs migrate to the injured site and alleviate the damage (21). Studies have demonstrated that MSCs have beneficial effects even at very early stages after their migration, before any differentiation and proliferation can be expected (28). This observation has led to the understanding that the MSC's early beneficial effects are related to their paracrine activity in the surrounding tissue (29, 30). Recent studies have demonstrated that MSCs can induce both local and remote anti-inflammatory effects (31). The immunomodulatory effects of MSCs are broad and cover much of the innate and adaptive immune systems (19). For example, MSCs can secrete factors such as insulin-like growth factor-1 (IGF-1), vascular endothelial growth factor (VEGF), angiopoietin 1, keratinocyte growth factor, and macrophage inflammatory protein 1α. These broad signaling factors are capable of promoting cell proliferation, angiogenesis, and wound healing (30). Paracrine or extracellular vesicle-delivered growth factors, such as hepatocyte growth factor (HGF) or VEGF, represent additional mechanisms by which MSCs exert therapeutic effects on renal injury (13).
MSCs can present both pro- and anti-inflammatory profiles. These different phenotypes are related to their ability to sense the environment and respond to changes in the tissue. The effect is induced by activation of different macrophage populations (19). Macrophages are divided to two main groups: M1 and M2 macrophages. M1 macrophages are considered proinflammatory cells and secrete proinflammatory cytokines including IL-1, IL-6, TNF-α, and interferon-γ. M2 macrophages are anti-inflammatory cells that secret anti-inflammatory cytokines such as IL-10 and transforming growth factor (TGF)-β1 (19, 32, 33). Thus, MSCs can induce differentiation of monocytes to one of the macrophage phenotype groups according to the inflammatory status of the damaged tissue (19). MSCs can also affect T-cell activation and differentiation toward T-regulatory cells that have anti-inflammatory properties (34). In addition to the paracrine effects on the immune system, MSCs can transfer mitochondria into the damaged cells, enabling better energy utilization, and restoration of the adenosine triphosphate (ATP) supply, thus promoting cellular recuperation (34). MSCs might also assist in preserving tubular mitochondria, thus preserving the functionality of these cells (35). By improving oxygen metabolism and energy utilization, MSCs reduce the oxidative stress and induce antioxidant activity (36).
To conclude, MSCs can promote tissue regeneration even before differentiating into the damaged cell line of the injured tissue. This influence is related to their early multifaceted paracrine effects.
Treatment With Mesenchymal Stromal Cells in Acute Kidney Injury
In the setting of AKI, MSCs promote protective effects on the injured kidney and ameliorate tissue damage (34, 36). The beneficial effects of MSCs are noticeable early after their injection and can be attributed to the following paracrine related mechanisms (Table 1 and Figure 1):
• An increase in the M2 macrophage CD68/CD163 population. As discussed, these M2 macrophages have anti-inflammatory and proregenerative properties (28, 32).
• A shift from the proinflammatory cytokines TNF-α, and IL-1β to the anti-inflammatory cytokine IL-10 with a favorable expression of the homing adhesion molecules intracellular adhesion molecules (ICAM-1) and vascular cell adhesion molecule 1 (VCAM-1) (50).
• An inhibitory effect on the complement system's overactivation and the related cellular damage generated by the membrane attack complex (28).
• Release of proangiogenesis growth factors such as VEGF, and proliferative growth factors (IGF, EGF) that promote cellular repair and promote cell regeneration (13, 51).
• Exosomes—one of the most exciting discoveries in intercellular communication. Exosomes are membrane-bound extracellular vesicles that are produced by most eukaryotic cells. Their size is about 30–120 nm in diameter (around the size of lipoproteins) and contain various molecular constituents of their cell of origin, including proteins, mRNA, and miRNA or double-stranded DNA (52, 53). Recent studies have demonstrated that administration of MSCs-derived exosomes can ameliorate the expected renal damage in the setting of AKI (52).
• Epigenetic effects—a shift in gene expression. Xie et al. (54) demonstrated that overexpression of the Klotho gene, which regulates apoptosis, can reinforce the protective effect of MSCs in the setting of AKI. Chen et al. demonstrated that the protective effect of MSCs in the setting of AKI can be related to TNF-inducible gene 6 protein expression. This protein, in addition to its anti-inflammatory effect, can promote renal tubular epithelial cell proliferation (55).
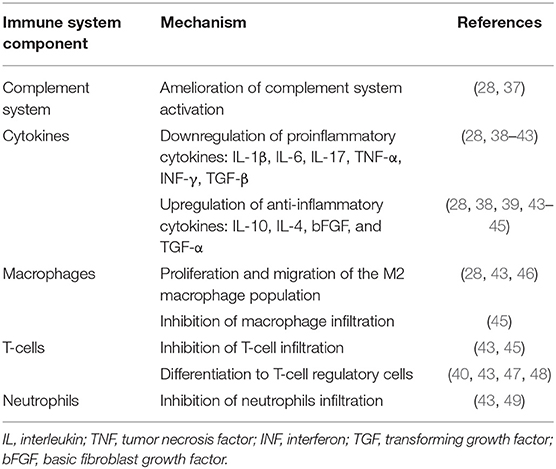
Table 1. Immunomodulatory mechanisms of mesenchymal stromal cells in the setting of acute kidney injury.
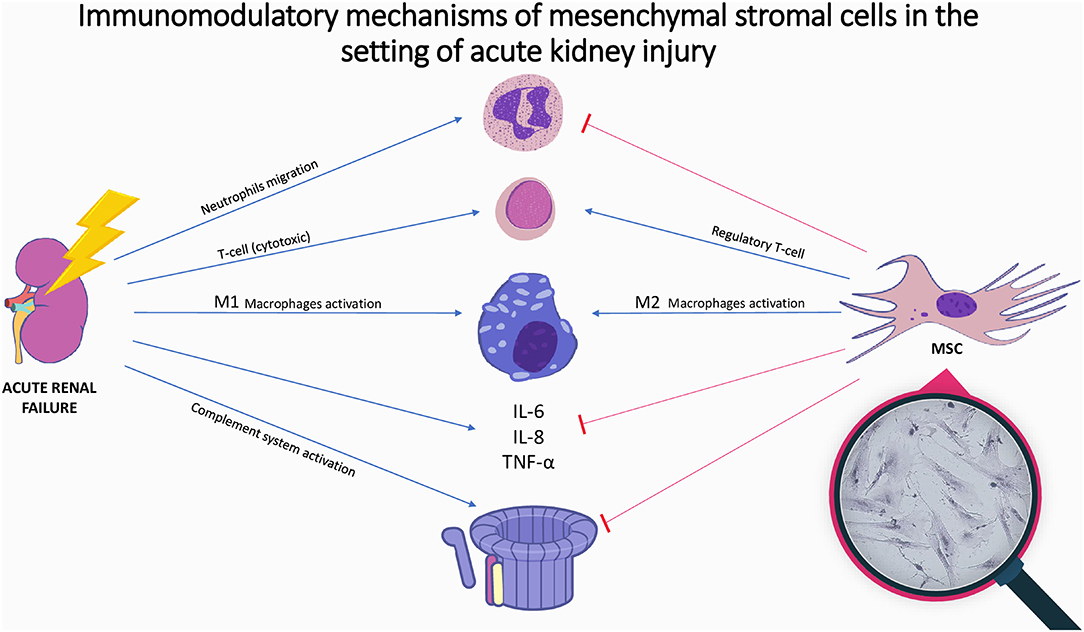
Figure 1. Immunomodulatory mechanisms of mesenchymal stromal cells in the setting of acute kidney injury. Illustration of the immune mechanisms of acute kidney injury and the immunomodulatory effect of mesenchymal stromal cells. Acute kidney injury is accompanied by increase in inflammatory cytokines, complement activation and immune cell activation. Mesenchymal stromal cells inhibit cytokines release, complement system activation, and neutrophils migration, while promoting M2-anti-inflammatory macrophages and regulatory T-cells proliferation; In magnifying glass: microscopic picture of mesenchymal stromal cells-placenta origin; MSC, mesenchymal stromal cell; IL, Interleukin; TNF, tumor necrosis factor.
While there is a growing body of knowledge in preclinical studies, the available clinical data on MSCs in AKI is still scarce. A recent study using MSCs in postcardiac surgery patients did not show beneficial effects regarding postsurgery AKI (56). This unfortunate result can be attributed to time of MSCs administration. The optimal results are obtained when MSCs are administered closest to the initiation of IRI (50). The detection of AKI, based on commonly used blood markers in humans (serum creatinine and urea), is usually late, after AKI and tubular necrosis are well-established (7). In this scenario, when the damage is already well-established, the potential immunological benefits of MSCs are probably negligible. In addition, the MSCs themselves might be injured by an overactivated complement system (57).
In addition to AKI, there is growing evidence of MSC benefits in the setting of chronic kidney disease (CKD). Even though the clinical studies done so far included relatively small numbers of patients, the evidence looks promising regarding the ability of MSCs to prevent the expected kidney function deterioration over time (58–60). In patients suffering from chronic diabetic nephropathy, allogeneic transplantation of MSCs demonstrated renal function improvements compared to placebo (60). The effect can be attributed to the paracrine secretion of VEGF and IGF-1 needed for angiogensis and tissue regeneration, and to the anti-inflammatory effect that controls the overactivated immune response that accompanies most CKD before significant sclerosis develops (59, 61).
One of the relevant clinical settings where MSCs potentially have beneficial effects is in postrenal transplantation patients. In the immediate posttransplantation period, IRI is one of the main reasons for AKI (62). Thanks to the above-discussed immunomodulating effects of MSCs, there are promising results in preclinical trials, and clinical studies are currently ongoing (63).
Current Available MSC Safety Data
Several safety concerns are related to the use of MSCs in clinical settings. The first concern is related to the administration technique. When the cells are administered intravenously (IV), most of the cells are found within the lungs (28, 64). When the lung capillaries are blocked with these cells, ventilation, and respiratory difficulties ensue. Therefore, higher dosage with high concentration of MSCs should be avoided. The second concern is related to exposing the immune system to foreign cells when administering donor cells. Luckily, MSCs do not stimulate an intense immune response, since they only express the HLA-DR but lack other HLA typings (29). In a CKD trial, none of the patients developed persistent donor-specific anti-HLA antibodies (60). In particular, fetal MSCs have very low immunogenicity by nature and can be used to overcome this potential barrier (36). The last concern is related to the proliferation and differentiation of pluripotent cells injected to a living body, with their potential of being transformed into malignant cells. This concern is probably irrelevant, since stromal cells need a special environment and signaling factors to act as stem cells and differentiate, and usually do not survive after administration (20, 64). In any case, to address this scenario, more research with long-term follow-up is needed.
Even though clinical trials with long-term follow-up are still lacking, some preclinical trials have addressed the safety issues. Till now, no serious adverse effects were reported in either preclinical (65, 66) or clinical studies (21, 56, 60, 67).
Summary and Future Perspectives
The ongoing cumulative data on the beneficial physiological effects of MSCs open new treatment opportunities for diseases that are currently being managed with only supportively therapy. While other types of stem cells, such as hematopoietic stem cells, are used in the clinical practice, the clinical data on MSCs is still scarce. In the setting of AKI, MSCs by way of their paracrine effects, can modulate the hazardous results of an overactivated inflammatory response. MSCs hold hope for future novel therapies, and a better understanding of the immune-biological effects of these cells will enable development of new treatment strategies.
Author Contributions
SZ-I and SE conducted a review of articles and wrote the manuscript. Both authors contributed to the article and approved the submitted version.
Conflict of Interest
The authors declare that the research was conducted in the absence of any commercial or financial relationships that could be construed as a potential conflict of interest.
Acknowledgments
We thank Dr. Mechael Kanovsky for his help in professionally editing the manuscript.
References
1. Kellum JA, Lameire N, Group KAGW. Diagnosis, evaluation, and management of acute kidney injury: a KDIGO summary (Part 1). Crit Care. (2013) 17:204. doi: 10.1186/cc11454
2. Levey AS, James MT. Acute Kidney Injury. Ann Intern Med. (2017) 167:ITC66-ITC80. doi: 10.7326/AITC201711070
3. Chawla LS, Kimmel PL. Acute kidney injury and chronic kidney disease: an integrated clinical syndrome. Kidney Int. (2012) 82:516–24. doi: 10.1038/ki.2012.208
4. Yu A, Chertow G, Luyckx V, Marsden P, Skorecki K, Taal M. Pathophysiology of acute kidney injury. In: Luyckx VA, editor. Brenner and Rector's The Kidney. Amsterdam: Elsevier (2019). p. 906–39.
5. Bellomo R, Kellum JA, Ronco C. Acute kidney injury. Lancet. (2012) 380:756–66. doi: 10.1016/S0140-6736(11)61454-2
6. Liano F, Pascual J. Epidemiology of acute renal failure: a prospective, multicenter, community-based study. Madrid acute renal failure study group. Kidney Int. (1996) 50:811–8. doi: 10.1038/ki.1996.380
7. Johnson RJ, Feehally J, Floege J, Tonelli M. Comprehensive Clinical Nephrology. Amsterdam: Elsevier (2019).
8. Ortiz A, Sanchez-Nino MD, Izquierdo MC, Martin-Cleary C, Garcia-Bermejo L, Moreno JA, et al. Translational value of animal models of kidney failure. Eur J Pharmacol. (2015) 759:205–20. doi: 10.1016/j.ejphar.2015.03.026
9. Bonavia A, Singbartl K. A review of the role of immune cells in acute kidney injury. Pediatr Nephrol. (2018) 33:1629–39. doi: 10.1007/s00467-017-3774-5
10. Singbartl K, Joannidis M. Short-term effects of acute kidney injury. Crit Care Clin. (2015) 31:751–62. doi: 10.1016/j.ccc.2015.06.010
11. Singbartl K, Formeck CL, Kellum JA. Kidney-immune system crosstalk in AKI. Semin Nephrol. (2019) 39:96–106. doi: 10.1016/j.semnephrol.2018.10.007
12. Sakai K, Nozaki Y, Murao Y, Yano T, Ri J, Niki K, et al. Protective effect and mechanism of IL-10 on renal ischemia-reperfusion injury. Lab Invest. (2019) 99:671–83. doi: 10.1038/s41374-018-0162-0
13. Gao L, Zhong X, Jin J, Li J, Meng XM. Potential targeted therapy and diagnosis based on novel insight into growth factors, receptors, and downstream effectors in acute kidney injury and acute kidney injury-chronic kidney disease progression. Signal Transduct Target Ther. (2020) 5:9. doi: 10.1038/s41392-020-0106-1
14. McCullough JW, Renner B, Thurman JM. The role of the complement system in acute kidney injury. Semin Nephrol. (2013) 33:543–56. doi: 10.1016/j.semnephrol.2013.08.005
15. Chan RK, Ibrahim SI, Verna N, Carroll M, Moore FD Jr, Hechtman HB, et al. Ischaemia-reperfusion is an event triggered by immune complexes and complement. Br J Surg. (2003) 90:1470–8. doi: 10.1002/bjs.4408
16. Zilberman-Itskovich S, Abu-Hamad R, Stark M, Efrati S. Effect of anti-C5 antibody on recuperation from ischemia/reperfusion-induced acute kidney injury. Ren Fail. (2019) 41:967–75. doi: 10.1080/0886022X.2019.1677248
17. Kinsey GR, Okusa MD. Expanding role of T cells in acute kidney injury. Curr Opin Nephrol Hypertens. (2014) 23:9–16. doi: 10.1097/01.mnh.0000436695.29173.de
18. Radi ZA. Immunopathogenesis of acute kidney injury. Toxicol Pathol. (2018) 46:930–43. doi: 10.1177/0192623318799976
19. Bernardo ME, Fibbe WE. Mesenchymal stromal cells: sensors and switchers of inflammation. Cell Stem Cell. (2013) 13:392–402. doi: 10.1016/j.stem.2013.09.006
20. Almalki SG, Agrawal DK. Key transcription factors in the differentiation of mesenchymal stem cells. Differentiation. (2016) 92:41–51. doi: 10.1016/j.diff.2016.02.005
21. Perico N, Casiraghi F, Remuzzi G. Clinical translation of mesenchymal stromal cell therapies in nephrology. J Am Soc Nephrol. (2018) 29:362–75. doi: 10.1681/ASN.2017070781
22. Dominici M, Le Blanc K, Mueller I, Slaper-Cortenbach I, Marini F, Krause D, et al. Minimal criteria for defining multipotent mesenchymal stromal cells. The International Society for Cellular Therapy position statement. Cytotherapy. (2006) 8:315–7. doi: 10.1080/14653240600855905
23. Galipeau J, Sensebe L. Mesenchymal stromal cells: clinical challenges and therapeutic opportunities. Cell Stem Cell. (2018) 22:824–33. doi: 10.1016/j.stem.2018.05.004
24. Dulak J, Szade K, Szade A, Nowak W, Jozkowicz A. Adult stem cells: hopes and hypes of regenerative medicine. Acta Biochim Pol. (2015) 62:329–37. doi: 10.18388/abp.2015_1023
25. Carvello M, Lightner A, Yamamoto T, Kotze PG, Spinelli A. Mesenchymal stem cells for perianal Crohn;s disease. Cells. (2019) 8:764. doi: 10.3390/cells8070764
26. Xie C, Liu YQ, Guan YT, Zhang GX. Induced stem cells as a novel multiple sclerosis therapy. Curr Stem Cell Res Ther. (2016) 11:313–20. doi: 10.2174/1574888X10666150302110013
27. Staff NP, Jones DT, Singer W. Mesenchymal stromal cell therapies for neurodegenerative diseases. Mayo Clin Proc. (2019) 94:892–905. doi: 10.1016/j.mayocp.2019.01.001
28. Zilberman-Itskovich S, Abu-Hamad R, Zarura R, Sova M, Hachmo Y, Stark M, et al. Human mesenchymal stromal cells ameliorate complement induced inflammatory cascade and improve renal functions in a rat model of ischemia-reperfusion induced acute kidney injury. PLoS One. (2019) 14:e0222354. doi: 10.1371/journal.pone.0222354
29. Ullah I, Subbarao RB, Rho GJ. Human mesenchymal stem cells - current trends and future prospective. Biosci Rep. (2015) 35:e00191. doi: 10.1042/BSR20150025
30. Chen L, Tredget EE, Wu PY, Wu Y. Paracrine factors of mesenchymal stem cells recruit macrophages and endothelial lineage cells and enhance wound healing. PLoS One. (2008) 3:e1886. doi: 10.1371/journal.pone.0001886
31. Shi Y, Wang Y, Li Q, Liu K, Hou J, Shao C, et al. Immunoregulatory mechanisms of mesenchymal stem and stromal cells in inflammatory diseases. Nat Rev Nephrol. (2018) 14:493–507. doi: 10.1038/s41581-018-0023-5
32. Tang PM, Nikolic-Paterson DJ, Lan HY. Macrophages: versatile players in renal inflammation and fibrosis. Nat Rev Nephrol. (2019) 15:144–58. doi: 10.1038/s41581-019-0110-2
33. Hamidzadeh K, Christensen SM, Dalby E, Chandrasekaran P, Mosser DM. Macrophages and the recovery from acute and chronic inflammation. Annu Rev Physiol. (2017) 79:567–92. doi: 10.1146/annurev-physiol-022516-034348
34. Erpicum P, Detry O, Weekers L, Bonvoisin C, Lechanteur C, Briquet A, et al. Mesenchymal stromal cell therapy in conditions of renal ischaemia/reperfusion. Nephrol Dial Transplant. (2014) 29:1487–93. doi: 10.1093/ndt/gft538
35. Perico L, Morigi M, Rota C, Breno M, Mele C, Noris M, et al. Human mesenchymal stromal cells transplanted into mice stimulate renal tubular cells and enhance mitochondrial function. Nat Commun. (2017) 8:983. doi: 10.1038/s41467-017-00937-2
36. Missoum A. Recent updates on mesenchymal stem cell based therapy for acute renal failure. Curr Urol. (2020) 13:189–99. doi: 10.1159/000499272
37. Tang M, Zhang K, Li Y, He QH, Li GQ, Zheng QY, et al. Mesenchymal stem cells alleviate acute kidney injury by down-regulating C5a/C5aR pathway activation. Int Urol Nephrol. (2018) 50:1545–53. doi: 10.1007/s11255-018-1844-7
38. Togel F, Hu Z, Weiss K, Isaac J, Lange C, Westenfelder C. Administered mesenchymal stem cells protect against ischemic acute renal failure through differentiation-independent mechanisms. Am J Physiol Renal Physiol. (2005) 289:F31–42. doi: 10.1152/ajprenal.00007.2005
39. Semedo P, Wang PM, Andreucci TH, Cenedeze MA, Teixeira VP, Reis MA, et al. Mesenchymal stem cells ameliorate tissue damages triggered by renal ischemia and reperfusion injury. Transplant Proc. (2007) 39:421–3. doi: 10.1016/j.transproceed.2007.01.036
40. Semedo P, Palasio CG, Oliveira CD, Feitoza CQ, Goncalves GM, Cenedeze MA, et al. Early modulation of inflammation by mesenchymal stem cell after acute kidney injury. Int Immunopharmacol. (2009) 9:677–82. doi: 10.1016/j.intimp.2008.12.008
41. Cao H, Qian H, Xu W, Zhu W, Zhang X, Chen Y, et al. Mesenchymal stem cells derived from human umbilical cord ameliorate ischemia/reperfusion-induced acute renal failure in rats. Biotechnol Lett. (2010) 32:725–32. doi: 10.1007/s10529-010-0207-y
42. Furuichi K, Shintani H, Sakai Y, Ochiya T, Matsushima K, Kaneko S, et al. Effects of adipose-derived mesenchymal cells on ischemia-reperfusion injury in kidney. Clin Exp Nephrol. (2012) 16:679–89. doi: 10.1007/s10157-012-0614-6
43. Sun P, Liu J, Li W, Xu X, Gu X, Li H, et al. Human endometrial regenerative cells attenuate renal ischemia reperfusion injury in mice. J Transl Med. (2016) 14:28. doi: 10.1186/s12967-016-0782-3
44. Luo J, Zhao X, Tan Z, Su Z, Meng F, Zhang M. Mesenchymal-like progenitors derived from human embryonic stem cells promote recovery from acute kidney injury via paracrine actions. Cytotherapy. (2013) 15:649–62. doi: 10.1016/j.jcyt.2013.01.009
45. Tsuda H, Yamahara K, Otani K, Okumi M, Yazawa K, Kaimori JY, et al. Transplantation of allogenic fetal membrane-derived mesenchymal stem cells protects against ischemia/reperfusion-induced acute kidney injury. Cell Transplant. (2014) 23:889–99. doi: 10.3727/096368913X665594
46. Geng Y, Zhang L, Fu B, Zhang J, Hong Q, Hu J, et al. Mesenchymal stem cells ameliorate rhabdomyolysis-induced acute kidney injury via the activation of M2 macrophages. Stem Cell Res Ther. (2014) 5:80. doi: 10.1186/scrt469
47. Kilpinen L, Impola U, Sankkila L, Ritamo I, Aatonen M, Kilpinen S, et al. Extracellular membrane vesicles from umbilical cord blood-derived MSC protect against ischemic acute kidney injury, a feature that is lost after inflammatory conditioning. J Extracell Vesicles. (2013) 2:21927. doi: 10.3402/jev.v2i0.21927
48. Hu J, Zhang L, Wang N, Ding R, Cui S, Zhu F, et al. Mesenchymal stem cells attenuate ischemic acute kidney injury by inducing regulatory T cells through splenocyte interactions. Kidney Int. (2013) 84:521–31. doi: 10.1038/ki.2013.114
49. Tian H, Lu Y, Shah SP, Wang Q, Hong S. 14S,21R-dihydroxy-docosahexaenoic acid treatment enhances mesenchymal stem cell amelioration of renal ischemia/reperfusion injury. Stem Cells Dev. (2012) 21:1187–99. doi: 10.1089/scd.2011.0220
50. Liu X, Cai J, Jiao X, Yu X, Ding X. Therapeutic potential of mesenchymal stem cells in acute kidney injury is affected by administration timing. Acta Biochim Biophys Sin (Shanghai). (2017) 49:338–48. doi: 10.1093/abbs/gmx016
51. Fleig SV, Humphreys BD. Rationale of mesenchymal stem cell therapy in kidney injury. Nephron Clin Pract. (2014) 127:75–80. doi: 10.1159/000363680
52. Aghajani Nargesi A, Lerman LO, Eirin A. Mesenchymal stem cell-derived extracellular vesicles for kidney repair: current status and looming challenges. Stem Cell Res Ther. (2017) 8:273. doi: 10.1186/s13287-017-0727-7
53. Altanerova U, Jakubechova J, Repiska V, Altaner C. Exosomes of human mesenchymal stem/stromal/medicinal signaling cells. Neoplasma. (2017) 64:809–15. doi: 10.4149/neo_2017_601
54. Xie LB, Chen X, Chen B, Wang XD, Jiang R, Lu YP. Protective effect of bone marrow mesenchymal stem cells modified with klotho on renal ischemia-reperfusion injury. Ren Fail. (2019) 41:175–82. doi: 10.1080/0886022X.2019.1588131
55. Chen Y, Tang X, Li P, Zhou Y, Xue T, Liu J, et al. Bone marrow derived mesenchymal stromal cells ameliorate ischemia/reperfusion injury-induced acute kidney injury in rats via secreting tumor necrosis factor-inducible gene 6 protein. Biomed Res Int. (2019) 2019:9845709. doi: 10.1155/2019/9845709
56. Swaminathan M, Stafford-Smith M, Chertow GM, Warnock DG, Paragamian V, Brenner RM, et al. Allogeneic mesenchymal stem cells for treatment of AKI after cardiac surgery. J Am Soc Nephrol. (2018) 29:260–7. doi: 10.1681/ASN.2016101150
57. Li Y, Lin F. Mesenchymal stem cells are injured by complement after their contact with serum. Blood. (2012) 120:3436–43. doi: 10.1182/blood-2012-03-420612
58. El-Ansary M, Saadi G, Abd El-Hamid SM. Mesenchymal stem cells are a rescue approach for recovery of deteriorating kidney function. Nephrology (Carlton). (2012) 17:650–7. doi: 10.1111/j.1440-1797.2012.01622.x
59. Saadi G, El Ansary M, Hassaballa M, Roshdy M, El-Aziz E, Bishai I, et al. Vascular endothelial growth factor and insulin growth factor as an underlying paracrine action of mesenchymal stem cells transfused for the regeneration of stage II and III chronic kidney disease. J Egypt Soc Nephrol Transpl. (2016) 16:3–9. doi: 10.4103/1110-9165.179198
60. Packham DK, Fraser IR, Kerr PG, Segal KR. Allogeneic mesenchymal precursor cells (MPC) in diabetic nephropathy: a randomized, placebo-controlled, dose escalation study. EBioMedicine. (2016) 12:263–9. doi: 10.1016/j.ebiom.2016.09.011
61. Sato Y, Yanagita M. Immune cells and inflammation in AKI to CKD progression. Am J Physiol Renal Physiol. (2018) 315:F1501–F12. doi: 10.1152/ajprenal.00195.2018
62. Chen CC, Chapman WC, Hanto DW. Ischemia-reperfusion injury in kidney transplantation. Front Biosci (Elite Ed). (2015) 7:117–34. doi: 10.2741/e722
63. Casiraghi F, Perico N, Cortinovis M, Remuzzi G. Mesenchymal stromal cells in renal transplantation: opportunities and challenges. Nat Rev Nephrol. (2016) 12:241–53. doi: 10.1038/nrneph.2016.7
64. Eggenhofer E, Benseler V, Kroemer A, Popp FC, Geissler EK, Schlitt HJ, et al. Mesenchymal stem cells are short-lived and do not migrate beyond the lungs after intravenous infusion. Front Immunol. (2012) 3:297. doi: 10.3389/fimmu.2012.00297
65. Park SE, Lee NK, Lee J, Hwang JW, Choi SJ, Hwang H, et al. Distribution of human umbilical cord blood-derived mesenchymal stem cells in the Alzheimer's disease transgenic mouse after a single intravenous injection. Neuroreport. (2016) 27:235–41. doi: 10.1097/WNR.0000000000000526
66. Cai J, Yu X, Xu R, Fang Y, Qian X, Liu S, et al. Maximum efficacy of mesenchymal stem cells in rat model of renal ischemia-reperfusion injury: renal artery administration with optimal numbers. PLoS One. (2014) 9:e92347. doi: 10.1371/journal.pone.0092347
Keywords: acute renal failure, AKI, mesenchymal stem cells, MSC, immune response
Citation: Zilberman-Itskovich S and Efrati S (2020) Mesenchymal Stromal Cell Uses for Acute Kidney Injury—Current Available Data and Future Perspectives: A Mini-Review. Front. Immunol. 11:1369. doi: 10.3389/fimmu.2020.01369
Received: 27 March 2020; Accepted: 28 May 2020;
Published: 21 July 2020.
Edited by:
Giuseppe Castellano, University of Bari Aldo Moro, ItalyReviewed by:
Maryna Skok, Palladin Institute of Biochemistry (NAS Ukraine), UkraineRui Li, Perelman School of Medicine at the University of Pennsylvania, United States
Copyright © 2020 Zilberman-Itskovich and Efrati. This is an open-access article distributed under the terms of the Creative Commons Attribution License (CC BY). The use, distribution or reproduction in other forums is permitted, provided the original author(s) and the copyright owner(s) are credited and that the original publication in this journal is cited, in accordance with accepted academic practice. No use, distribution or reproduction is permitted which does not comply with these terms.
*Correspondence: Shani Zilberman-Itskovich, c2hhbmkuemlsYmVybWFuQG1haWwuaHVqaS5hYy5pbA==