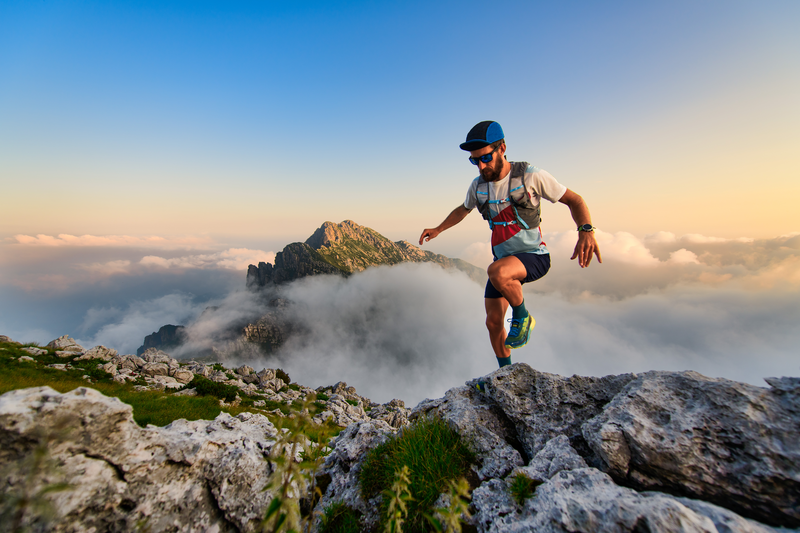
94% of researchers rate our articles as excellent or good
Learn more about the work of our research integrity team to safeguard the quality of each article we publish.
Find out more
REVIEW article
Front. Immunol. , 19 May 2020
Sec. Cytokines and Soluble Mediators in Immunity
Volume 11 - 2020 | https://doi.org/10.3389/fimmu.2020.00868
This article is part of the Research Topic Modulating Cytokines as Treatment for Autoimmune Diseases and Cancer View all 14 articles
We completed clinical trials of rhIL-15 by bolus, subcutaneous, and continuous intravenous infusions (CIV). IL-15 administered by CIV at 2 mcg/kg/day yielded a 38-fold increase in 10- day number of circulating NK cells, a 358-fold increase in CD56bright NK cells and a 5.8-fold increase in CD8 T cells. However, IL-15 preparations administered as monotherapy were ineffective, due to actions of immunological checkpoints and due to the lack of tumor specific targeting by NK cells. To circumvent checkpoints, trials of IL-15 in combination with other anticancer agents were initiated. Tumor-bearing mice receiving IL-15 with antibodies to CTLA-4 and PD-L1 manifested marked prolongation of survival compared to mice receiving IL-15 with either agent alone. In translation, a phase I trial was initiated involving IL-15 (rhIL-15), nivolumab and ipilimumab in patients with malignancy (NCT03388632). In rhesus macaques CIV IL-15 at 20 μg/kg/day for 10 days led to an 80-fold increase in number of circulating effector memory CD8 T cells. However, administration of γc cytokines such as IL-15 led to paralysis/depression of CD4 T-cells that was mediated through transient expression of SOCS3 that inhibited the STAT5 signaling pathway. This lost CD4 helper role could be restored alternatively by CD40 agonists. In the TRAMP-C2 prostate tumor model the combination of IL-15 with agonistic anti-CD40 produced additive effects in terms of numbers of TRAMP-C2 tumor specific Spas/SCNC/9H tetramer positive CD8 T cells expressed and tumor responses. A clinical trial is being initiated for patients with cancer using an intralesional anti-CD40 in combination with CIV rhIL-15. To translate IL-15-mediated increases in NK cells, we investigated combination therapy of IL-15 with anticancer monoclonal antibodies including rituximab in mouse models of EL-4 lymphoma transfected with human CD20 and with alemtuzumab (CAMPATH-1H) in a xenograft model of adult T cell leukemia (ATL). IL-15 enhanced the ADCC and therapeutic efficacy of both antibodies. These results provided the scientific basis for trials of IL-15 combined with alemtuzumab (anti-CD52) for patients with ATL (NCT02689453), with obinutuzumab (anti-CD20) for patients with CLL (NCT03759184), and with avelumab (anti-PD-L1) in patients with T-cell lymphoma (NCT03905135) and renal cancer (NCT04150562). In the first trial, there was elimination of circulating ATL and CLL leukemic cells in select patients.
The goal of immunotherapy is to direct the host immune system to attack patients' cancer (1, 2). Clinical trials initially focused on efforts to enhance immune responses using the stimulatory cytokines IFNα or IL-2 (2). High-dose IL-2 was approved by the FDA for the treatment of patients with metastatic melanoma and metastatic renal carcinoma (3) but caused severe systemic toxicity, including capillary leak syndrome, hypotension, hypoxia, and oliguric renal failure. These problems prompted the investigation transition to IL-15 in an effort to obtain the benefits of IL-2 but with fewer adverse events (AEs) (2).
IL-15 was identified by our group and by Grabstein in culture supernatants from HUT102 and Cv1/EBNA cell lines that stimulated proliferation of the cytokine dependent T-cell line CTLL-2 (4–6). IL-15 is a 14–15 kDa 4 alpha-helix-bundle family cytokine family member that stimulates the generation of NK, NKT, gamma delta, IL/C1, intraepithelial lymphocytes, innate cells expressing CD103+ CD56+ CD44+ and memory CD8 T cells (2, 7–17). Some IL-15 regulation of protein production occurs at the level of transcription; however, most control is at translation (18). Type I and II interferons, CD40 ligation, and Toll-like receptor stimuli stimulate transcription (19). IL-15 translation is impeded by multiple 5′-untranslated region (UTR) AUG sequences, a long signal peptide and a negative regulatory element in the coding sequence C-terminus (19). IL-15 mRNA is expressed by many tissues. However, IL-15 protein is largely limited to dendritic cells, macrophages, and monocytes (20). IL-15 signals through a heterotrimeric receptor that is composed of the common gamma chain (γc) subunit (CD132) shared with IL-2, IL-4, IL-7, IL-9, and IL-21; the beta chain (βc) subunit (IL-2/IL-15R, CD122) shared with the IL-2 receptor and a private IL-15 specific alpha subunit IL-15Rα (CD215) (2, 8, 20, 21). IL-15 binding to the IL-2/IL-15Rβ/γc heterodimeric receptor induces JAK1 activation that phosphorylates STAT3 via the beta chain, and JAK3 activation that phosphorylates STAT5 via the gamma chain (20–25).
IL-15, like IL-2, stimulated proliferation of T cells, induced generation of cytotoxic lymphocytes and memory phenotype CD8 T cells, and stimulated proliferation and maintenance of natural killer (NK) cells (2, 8, 20). In contrast to IL-2, IL-15 did not mediate activation-induced cell death (AICD), did not consistently activate Tregs and caused less capillary leak syndrome (2, 8, 26). IL-2 is a promiscuously secreted molecule, whereas IL-15 is locally secreted in small quantities where membrane-bound IL-15 induces signals at an immunological synapse (27–32). IL-15 and IL-15Rα co-expressed by monocytes and DCs become associated on cell surfaces where IL-15 is presented in trans to NK and CD8 memory T-cells (27–32). In addition, IL-15 cis presentation is required for optimal NK-cell activation in lipopolysaccharide-mediated inflammatory conditions (33).
Although IL-2 stimulates immune responses directed at cancer cells, it also suppresses immune responses by maintenance of CD25+ Foxp3 T-regulatory cells and by participation in AICD (34–37).
Efficacy was observed with IL-15 in multiple murine immunotherapy trials including the syngeneic TRAMP (transgene adenocarcinoma mouse prostate) -C2 prostatic cancer, Pme1-1, B16 melanoma, MC38 and CT26 colon carcinoma models suggesting that IL-15 might be more effective than IL-2 in cancer therapy (38–40). Ten-day 20 mcg/kg/day administration of IL-15 to rhesus macaques by continuous infusion (CIV) was associated with an 80–100 fold increase in the number of circulating effector memory CD8 T cells (41, 42). To translate the observation of the effect of IL-15 on NK cells and CD8 cells, we have completed first-in-human trials of rhIL-15 by bolus, subcutaneous and continuous intravenous infusions (CIV) (2, 43–45). However, IL-15 administered as monotherapy was ineffective, likely due to the actions of immunological checkpoints (2). To circumvent such checkpoints, trials of IL-15 in combination with other anticancer agents have been initiated and are a major focus of this review.
We initiated a first-in-human phase I trial of recombinant Escherichia coli produced IL-15 administered by IV bolus daily for 12 days to patients with metastatic malignancy (2, 43) (Table 1). The initial dose of 3 μg/kg/day was too toxic with patients developing grade 3 thrombocytopenia and hypotension, and doses of 1.0 and 0.3 μg/kg/day were added (2, 43). All patients at the 0.3 μg/kg dose level received 12 doses without dose-limiting toxicity (DLT). With the 3 μg/kg dose level as assessed by flow cytometry there was a 10-fold increase in the circulating NK numbers, a 3-fold increase in the number of CD4 cells and an 8-fold increase in the number of CD8 T cells. Stable disease was the best response. Inflammatory cytokines IL-6 and IFN-γ were markedly elevated (50-fold), a phenomenon which coincided with acute clinical toxicities of fever, chills and blood pressure changes. To reduce toxicity by reducing Cmax excess, mediated cytokine release, and macrophage activation syndrome, two additional clinical trials were initiated, one by subcutaneous, and another by continuous intravenous infusion (2, 44, 45).
In the subcutaneous rhIL-15 trial in refractory solid tumor cancer patients' therapy consisted of daily (Monday–Friday) subcutaneous injections of rhIL-15 for 2 consecutive weeks (44). Nineteen patients were treated with rhIL-15. Among 19 patients treated there were two serious events: grade 2 pancreatitis, grade 3 cardiac chest pain, hypotension, and elevated troponin. No objective responses were observed. Treatment induced a 3-fold increase in the number of circulating CD8 T cells, a 10.8-fold expansion of circulating NK cells, and a 39.7-fold increase in CD56bright cells.
In an additional trial 27 patients were treated for 10 days by continuous intravenous infusion with rhIL-15; with 2.0 μg/kg/day identified as the MTD (45). There were eight serious adverse events including: papilledema, uveitis, pneumonitis, duodenal erosions, two bleeding events, and two deaths, one likely due to drug-related gastrointestinal ischemia (2, 45). Limited reduction in tumor volume was observed in several patients, but stable disease per RECIST 1.1 criteria was the best response noted (45). In this trial, the IL-15 Cmax was at 48 h, followed by a decline of serum IL-15 concentrations during the infusions to 8% of the maximum level by days 8–10 of infusion. This decline may reflect IL-15-mediated induction of the number of IL-15 receptor-bearing cells with an increase in the number of IL-2/IL-15Rβ (CD122) receptors per cell acting as a sink binding the infused rhIL-15. There was a mean 5.8-fold increase in the number of circulating CD8 T cells, a 38-fold increase in the total of NK cells and a 358-fold increase in CD56bright NK cells (2, 45).
Studying purified NK cells in vitro Felices et al. (48) suggested that continuous treatment with IL-15 exhausts purified NK cells resulting in decreased viability and a cell cycle arrest gene expression pattern. Furthermore, they propose that their findings should inform IL-15 dosing strategies (2, 48). Our studies with IL-15 in vivo by CIV to humans do not support these conclusions. The proliferation rates of different subsets of NK cells 2 days after the termination of 10-day IL-15 CIV assayed by Ki-67 were over 90% (45, 49). The cytolytic capacities were very effective for both CD56dim and CD56bright NK subsets. At the maximum NK level 2 days following the termination of IL-15 CIV the antibody-dependent cellular cytotoxicity (ADCC) assayed with CD20 antibody-coated Raji cells, natural cytotoxicity to K-562 cells mediated by NKp30, NKp46, and MICA/NKG2D mediated cytotoxicity was exceptionally effective (2, 49). These observations on the effects of IL-15 on NK subsets do not support the hypothesis that such strategies would be associated with NK-cell exhaustion but rather support the view that after 10-day rhIL-15 CIV NK cells remain effective.
A major challenge with rhIL-15 is that it has a short in vivo survival. Therefore, an array of alternative IL-15 agents associated with IL-15Rα were introduced clinically (50–61) (Figure 1). These included an IL-15N72D mutein with a 4-5-fold increase in biological activity, heterodimeric mammalian IL-15/IL-15Rα (hetIL-15) (51–54) a heterodimer consisting of IL-15 and IL-15Rα (51–54), the RLI, a fusion protein consisting of IL-15 linked to the cytokine binding (sushi) domain of IL-15Rα (59, 60), RLI-anti-CD20 and RLI-CD20 which are RLI linked to anti-CD20 or GD2 (55, 61), ALT-803 a mutated (N72D) IL-15 linked to the sushi domain of IL-15Rα that is fused to an IgG-Fc fragment to increase in vivo survival (55–57), the ALT-803 scaffold fused to 4-single chains of rituximab, a tumor-targeting monoclonal antibody (2, 58). ALT-803 was administered to 33 patients with hematological malignancies via IV or SC once weekly for 4 doses and pharmacokinetic analysis showed prolonged serum concentrations following SC compared to IV infusion (46). There were 2 deaths–one due to sepsis, and one intracranial hemorrhage purported to be unrelated to ALT-803. Administrations of hetIL-15 or ALT-803 by subcutaneous injection produced concentric injection site reactions up to 30 cm in diameter erythematous plaques that were associated with infiltration of CD56+ NKp46− γδ T-cells. This and other systemic AEs with ALT-803 and hetIL-15 precluded further increases in doses of IL-15 bearing agents. When the maximum fold increases in the number of circulating NK cells with different agents and dosing schedules were compared, rhIL-15 administered by bolus infusion at the MTD (0.3 mcg/kg/day) yielded a 2–3 fold increase in NK cells (43) (Table 1). rhIL-15 administered subcutaneously at the expansion dose of 2 mcg/kg/day on days 1–5, and 8–12 was associated with a 10.8-fold increase in total circulating NK cells and a 39.7-fold increase in CD56bright NK cells (44). The ALT-803 mutant at 10 mcg/kg/week elicited an 8-fold increase in NK cells (46). rhIL-15 by CIV at 2 mcg/kg/day for 10 days resulted in the greatest increase with a 38-fold increase in circulating total NK cells and a 358-fold increase in CD56bright NK cells (45).
Figure 1. IL-15 agonists used in immunotherapy. IL-15 preparations in clinical use include rhIL-15 produced in Escherichia coli (43–45), an IL-15N72D mutein (50), heterodimeric mammalian IL-15 (hetIL-15) (51–54), RLI, a fusion protein consisting of IL-15 linked to the cytokine-binding (sushi) domain of IL-15R alpha (59). Anti-CD-20-RLI and anti-GD2-RLI are fusion proteins consisting of RLI linked to anti-CD20 or anti-GD2, respectively (55, 61). ALT-803 (Altor Pharmaceutical) represents a mutated N72D) IL-15 (asparagine replacing aspartic residue) linked to the sushi domain of IL-15R that is fused to an IgG-Fc fragment to increase in vivo survival (56, 57) and ALT-803 scaffold has been fused to 4 single- chains of the tumor-targeting monoclonal antibody rituximab (58).
Novel approaches with IL-15 are being developed to yield the desired pharmacokinetics of IL-15 plus IL-15Rα with one dosing per week along with maximal increases in NK and CD8 T cells provided by CIV rhIL-15. PEGylated IL-15 to prolong survival is being developed. Furthermore, a long-acting rhIL-15 depo for enhanced cancer immunotherapy is being developed, with IL-15 mixed with an aqueous solution of PLGA-PEG, a copolymer that is in solution at room temperature but transitions into a hydrogel at body temperature (Tan and Waldmann unpublished observations).
Although rhIL-15 by CIV yielded dramatic augmentation in the number of circulating NK cells, it will have to be used in combination with other anticancer agents due to the inhibitory actions of immunological checkpoints and the lack of tumor specific targeting by NK cells (Tables 2, 3). A major challenge in IL-15 immunotherapy is finding a combination of drugs with new mechanisms of action that improve the outcome achieved with the existing standard of care and simultaneously result in fewer toxic effects for patients. Forty-two patients with refractory acute myeloid leukemia received intravenous (IV) (NCT01385423) or subcutaneous (SC) (NCT02395822) recombinant human IL-15 (rhIL-15) after lymphodepleting chemotherapy and haploidentical NK cell infusions (75). Escalating doses of rhIL-15 (0.3–1.0 mcg/kg) were given on 12 consecutive days in a phase I trial to 26 patients. Subcutaneous IL-15 at 2.0 mcg/kg was administered in a phase II trial to 16 patients. With the IV dosing and dose level 3 (1 mcg/kg) dose-limiting toxicity consisting of grade 4 pulmonary toxicity (diffuse alveolar hemorrhage) in one patient and prolonged neutropenia (beyond 42 days) in 2 of 4 patients at this dose were observed. In the subsequent phase II trial using 2 mcg/kg SC of rhIL-15 there was a previously unreported cytokine release syndrome (CRS) observed in 56% of patients with concurrent neurological toxicity in 5 of 9 patients that was responsive to steroid and tocilizumab treatment. Eight of 25 evaluable patients receiving IV IL-15 had a response with 6 CRs and 2 CRis. The overall response to SC IL-15 was 6 of 15 patients with a CR in one and CRi in 5 (75). Thus, haploidentical NK cell infusions given with rhIL-15 achieved remissions in about 35% of patients with refractory acute myeloid leukemia.
In rhesus macaques IL-15 by CIV at 20 mcg/kg/day for 10 days led to 80 to 100-fold increases in circulating effector memory CD8 T cells (41). Furthermore, rhIL-15 by CIV to patients with metastatic malignancy led to a mean 5.8-fold increase in the number of circulating CD8+, MHC class II+ cells (2, 45). However, this effect was not associated with evidence that the CD8 T cells manifested antitumor activity nor did it provide anticancer efficacy. In terms of CD8 T-cell function, γc cytokines such as IL-15 induced immunoregulatory SOCS checkpoint agents. IL-15 increased the expression of CIS, a checkpoint of NK cell mediated tumor immunity as well as SOCS1 that attenuates IL-15 receptor signaling by CD8+ CD44hi memory T lymphocytes (77, 78). Furthermore, Sckisel et al. demonstrated that administration of gamma cytokines such as IL-2 and IL-15 led to paralytic depression of CD4 T cell that was mediated through transient expression of SOCS3 that inhibited STAT5B signaling (2, 79). This paralysis of CD4 helper T-cell activity inhibited the generation of tumor-specific CD8 T cells. It was demonstrated that CD4 helper cells' role could be provided by CD40 agonists (67, 68, 80–82). We showed in the TRAMP-C2 murine syngeneic tumor model that treatment with either an agonistic anti-CD40 antibody alone or IL-15 prolonged animal survival, however the combination of agonistic anti-CD40 with IL-15 produced markedly additive effects when compared with either agent alone (68). Neither agonistic anti-CD40 nor IL-15 augmented the number of tumor-specific CD8 T cells, whereas administration of the combination of IL-15 with an agonistic anti-CD40 antibody was associated with a 10-fold increase in the number of SPAS/SCNC 9H tetramer positive anti-TRAMP-C2 tumor specific CD8 T cells (68). Examination of this tumor system was extended by evaluating TRAMP-C2 administered on each flank with anti-CD40 administered intratumorally in one flank tumor and IL-15 administered systemically. In this model there was an abscopal effect obtained with reduction in the size of the tumor not injected with anti-CD40 beyond that mediated by IL-15 alone. A clinical trial is being initiated that utilizes an optimized intralesional anti-CD40 antibody in combination with CIV rhIL-15 (83, 84).
IL-15 augments the expression of immune checkpoints TIGIT, TIM3, IL-10, as well as the expression of PD-1 on CD8 T cells (85, 86). Furthermore, IL-15 is required for the expression of a negative regulatory lymphocyte population that expresses CD122+ CD8+ (87). The combination of anti-PD-L1 with ALT-803 yielded additivity in murine tumor models (71–73, 88). Furthermore, trans-signaling with the RLI human IL-15 linked to the human IL-15Rα sushi domain augmented effector memory CD8 T-cell responses and enhanced antitumor activity of the PD-1 agonist (72). ALT-803 in combination with nivolumab in individuals with non-small cell lung cancer was associated with an objective response in 6 of 21 patients (74). To address checkpoints, we administered IL-15 in combination with antibodies to PD-L1 and cytotoxic lymphocyte antigen-4 (CTLA-4) in the CT26 and MC38 colon carcinoma and TRAMP-C2 prostatic cancer syngeneic tumor models (2, 69, 70). In these models IL-15 alone provided modest antitumor activity. The addition of either PD-L1 or CTLA-4 in association with IL-15 did not augment its efficacy. However, tumor-bearing mice receiving the combination of both anti-checkpoint antibodies with IL-15 manifested a significant prolongation of survival. In translation of this observation, a phase I trial in patients with refractory cancers has been initiated that involves rhIL-15 in combination with nivolumab and ipilimumab (NCT03388632).
As noted above, rhIL-15 administration led to dramatic increases in the number of activated NK cells, however such increases alone were not sufficient to produce antitumor efficacy probably because most tumors express self MHC class I molecules that interact with KIRs or NKG2A/CD94 that inhibit NK-effector function (2). Furthermore, there is lack of tumor cell identification and specific targeting by NK cells. The combination of IL-15 with tumor specific monoclonal antibodies has shown efficacy with a number of anticancer antibodies (Tables 2, 3) (55, 56, 58, 62, 64, 72). In preclinical trials IL-15 preparations have been reported to be of value in combination with in vivo administered anticancer monoclonal antibodies. In particular, IL-15 increased ADCC and antitumor activity when administered with anti-gp75, with B16F10 tumors (56) and with anti-CD20 with B-cell lymphomas (65). The combination of IL-15 with anti-PD1 or anti-PD-L-1 was more effective than with the individual agents alone (62, 69, 72, 75). In addition, an engineered fusion protein involving a soluble form of human IL-15Rα sushi with an antibody demonstrated antitumor responses (58). Furthermore, there was an enhanced ADCC and anti-breast cancer efficacy of cetuximab with a chimeric protein encompassing human IL-15 (62). We also investigated a combination therapy that involves IL-15 with rituximab in a syngeneic mouse model of EL4 transfected with human CD20 and with alemtuzumab (CAMPATH-1H) in a xenograft model of human adult T-cell leukemia (ATL) (69). IL-15 enhanced the therapeutic efficacy of both antibodies. This efficacy was dramatically reduced in FcRγ−/− mice suggesting that IL-15 increased the ADCC of the anticancer monoclonal antibodies. Both NK cells and macrophages were critical elements of interacting effectors involved in the augmented ADCC and augmented therapeutic responses (71). Following interaction with macrophages there was induction of expression of FcRγIV critical for ADCC by the NK cells (2, 74). These results provided the scientific basis for a phase I trial of IL-15 combined with alemtuzumab (anti-CD52) for patients with ATL (NCT02689453) (2). Trials have also been initiated in patients with chronic lymphocytic leukemia with obinutuzumab in combination with rhL-15 (NCT03759184) and IL-15 with avelumab (anti-PD-L1) in patients with mature T-cell lymphoma (NCT03905135) and renal cell cancer (NCT04150562), and IL-15 with mogamulizumab (anti-CCR4, NCT04185220) in patients with ATL and cutaneous T-cell lymphoma.
Despite their dramatic augmentation of NK cells and CD8 T cells, all IL-15 preparations administered as monotherapy in solid tumor patients with cancer have been ineffective probably due to counter-regulatory immunologic processes. In particular, there was inhibition of NK action by interaction of KIRs and NKG2A with self-class I MHC. There was parallel inhibition of CD8 T cells stimulated by IL-15 due to the induction of SOCS3 in CD4 helper T cells, thereby yielding “helpless” CD8 T cells (79). Furthermore, IL-15 induced checkpoints TIGIT, TIM3, IL-10, and PD-1 on CD8 T cells (85, 86). To circumvent these checkpoints combination trials that involve IL-15 with multiple anticancer agents are being performed. Our combination therapeutic trials include IL-15 with intralesional agonistic anti-CD40 to yield tumor specific CD8 T cells, IL-15 with the checkpoint inhibitors, anti-CTLA-4 and anti-PD-L1, and especially IL-15 with cancer directed monoclonal antibodies to increase their ADCC and anticancer efficacy. It is hoped with the use of these combination therapies that IL-15 will take a prominent role in the treatment of patients with metastatic malignancy.
TW designed, wrote, and edited the manuscript. SD, MM, and KC provided critical comments, concepts, and insights. All authors read and approved the manuscript prior to submission.
This paper was supported in part by the Intramural Program of the Center for Cancer Research, National Cancer Institute, NIH.
The authors declare that the research was conducted in the absence of any commercial or financial relationships that could be construed as a potential conflict of interest.
This study was supported by the Intramural Research Program of the National Cancer Institute, NIH. This study was performed under the conditions of the World Medical Association's Declaration of Helsinki. All patients signed a written informed consent for participation in clinical studies. Clinical studies were approved by the Intramural Review Board of the National Cancer Institute. Animal studies were approved by the NCI Animal Care and Use Committee.
1. Restifo NP, Dudley ME, Rosenberg SA. Adoptive immunotherapy for cancer: harnessing the T cell response. Nat Rev Immunol. (2012) 12:269–81. doi: 10.1038/nri3191
2. Waldmann TA, Miljkovic MD, Conlon KC. IL-15 (dys) regulation of lymphoid homeostasis: implications for therapy of autoimmunity and cancer. J Exp Med. (2020) 217:e20191062. doi: 10.1084/jem.20191062
3. Rosenberg SA. IL-2: the first effective immunotherapy for human cancer. J Immunol. (2014) 192:5451–8. doi: 10.4049/jimmunol.1490019
4. Bamford RN, Grant AJ, Burton JD, Peters C, Kurys G, Goldman CK, et al. The interleukin (IL) 2 receptor beta chain is shared by IL-2 and a cytokine, provisionally designated IL-T, that stimulates T-cell proliferation and the induction of lymphokine-activated killer cells. Proc Natl Acad Sci USA. (1994) 91:4940–4. doi: 10.1073/pnas.91.11.4940
5. Burton JD, Bamford RN, Peters C, Grant AJ, Kurys G, Goldman CK, et al. A lymphokine, provisionally designed interleukin T and produced by a human adult T-cell leukemia line, stimulates T-cell proliferation and the induction of lymphokine-activated killer cells. Proc Natl Acad Sci USA. (1994) 91:4935–9. doi: 10.1073/pnas.91.11.4935
6. Grabstein KH, Eisenman J, Shanebeck K, Rauch C, Srinivasan S, Fung V, et al. Cloning of a T cell growth factor that interacts with the beta chain of the interleukin-2 receptor. Science. (1994) 264:965–8. doi: 10.1126/science.8178155
7. Waldmann TA, Tagaya Y. IL-15 cytokine. In Oppenheim JJ, Feldmann M, editors. The Cytokine Reference. A Compendium of Cytokines and Other Mediators of Host Defense. London: Academic Press. (2000). p. 213–24.
8. Fehniger TA, Caligiuri MA. Interleukin 15: biology and relevance to human disease. Blood. (2001) 297:14–32. doi: 10.1182/blood.V97.1.14
9. Boyman O, Letourneau S, Krieg C, Spreng J. Homeostatic proliferation and survival of naïve and memory T cells. Eur J Immunol. (2009) 39:2088–94. doi: 10.1002/eji.200939444
10. Schluns KS, Lefrancois L. Cytokine control of memory T-cell development and survival. Nat Rev Immunol. (2003) 3:269–79. doi: 10.1038/nri1052
11. Mackay LK, Rahimpour A, Ma JZ, Collins N, Stock AT, Hafon ML, et al. The developmental pathway for CD103+CD8+ tissue-resident memory T cells of skin. Nat Immunol. (2013) 14:1294–301. doi: 10.1038/ni.2744
12. Mayassi T, Jabri B. Human intraepithelial lymphocytes. Mucosal Immunol. (2018) 11:1281–9. doi: 10.1038/s41385-018-0016-5
13. Kwong B, Lazarevic V. T-bet orchestrates CD8αα IEL differentiation. Immunity. (2014) 41:169–71. doi: 10.1016/j.immuni.2014.08.003
14. Klose CS, Blatz K, d'Hargues Y, Hernandez PP, Kofoed-Nielsen M, Ripka, et al. The transcription factor T-bet is induced by IL-15 and thymic agonist selection and controls CD8αα(+) intraepithelial lymphocyte development. Immunity. (2014) 41:230–43. doi: 10.1016/j.immuni.2014.06.018
15. Robinette ML, Bando JK, Song W, Ulland TK, Gilfillan S, Colonna M. IL-15 sustains IL-7R-independent ILC2 and ILC3 development. Nat Commun. (2017) 8:14601. doi: 10.1038/ncomms14601
16. Fuchs A, Vermi W, Lee JS, Lonardi S, Gilfillan S, Newberry RD, et al. Intraepithelial type 1 innate lymphoid cells are a unique subset of IL-12-and IL-15 responsive IFN-γ-producing cells. Immunity. (2013) 38:769–81. doi: 10.1016/j.immuni.2013.02.010
17. Sciume G, Le MT, Gadina M. HiJAKing innate lymphoid cells? Front Immunol. (2017) 8:438. doi: 10.3389/fimmu.2017.00438
18. Bamford RN, Battiata AP, Waldmann TA. IL-15: the role of translational regulation in their expression. J Leukoc Biol. (1996) 59:476–80. doi: 10.1002/jlb.59.4.476
19. Bamford RN, Battiata AP, Burton JD, Sharma H, Waldmann TA. Interleukin (IL)-15/IL-T production by the adult T-cell leukemia line HuT-102 is associated with a human T-cell lymphotrophic virus type 1 region/IL-15 fusion message that lacks many upstream AUGs that normally attenuate IL-15 mRNA translation. Proc Natl Acad Sci USA. (1996) 93:2897–902. doi: 10.1073/pnas.93.7.2897
20. Waldmann TA. The biology of interleukin-2 and interleukin-15: implications for cancer therapy and vaccine design. Nat Rev Immunol. (2006) 6:595–601. doi: 10.1038/nri1901
21. Mishra A, Sullivan L, Caligiuri MA. Molecular pathways: interleukin-15 signaling in health and in cancer. Clin Cancer Res. (2014) 20:2044–50. doi: 10.1158/1078-0432.CCR-12-3603
22. Carson WE, Fehniger TA, Haldar S, Eckhert K, Lindemann MJ, Lai CF, et al. A potential role for interleukin-15 in the regulation of human natural killer cell survival. J Clin Invest. (1997) 99:937–43. doi: 10.1172/JCI119258
23. Geginat J, Sallusto F, Lanzavecchia A. Cytokine-driven proliferation and differentiation of human naïve, central memory and effector memory CD4+ T cells. Pathol Biol (Paris). (2003) 51:64–6. doi: 10.1016/S0369-8114(03)00098-1
24. Farag SS, Caligiuri MA. Human natural killer cell development and biology. Blood Rev. (2006) 20:123–37. doi: 10.1016/j.blre.2005.10.001
25. Huntington ND. The unconventional expression of IL-15 and its role in NK cell homeostasis. Immunol Cell Biol. (2014) 92:210–3. doi: 10.1038/icb.2014.1
26. Marks-Konczalik J, Dubois S, Losi JM, Sabzevari H, Yamada H, Feigenbaum L, et al. IL-2 induced activation-induced cell death is inhibited in IL-15 transgenic mice. Proc Natl Acad Sci USA. (2000) 97:11445–50. doi: 10.1073/pnas.200363097
27. Dubois S, Mariner J, Waldmann TA, Tagaya Y. IL-15R alpha recycles and presents IL-15 in trans to neighboring cells. Immunity. (2002) 17:537–47. doi: 10.1016/S1074-7613(02)00429-6
28. Ma A, Koka R, Burkett P. Diverse functions of IL-2, IL-15 and IL-7 in lymphoid homeostasis. Annu Rev Immunol. (2006) 24:657–79. doi: 10.1146/annurev.immunol.24.021605.090727
29. Kobayashi H, Dubois S, Sato N, Sabzevari H, Sakai Y, Waldmann TA, et al. Role of trans-cellular IL-15 presentation in the activation of NK cell-mediated killing, which leads to enhanced tumor immunosurveillance. Blood. (2005) 105:721–7. doi: 10.1182/blood-2003-12-4187
30. Lucas M, Schachterle W, Oberle K, Aichele P, Diefenbach A. Dendritic cells prime natural killer cells by trans-presenting interleukin 15. Immunity. (2007) 26:503–17. doi: 10.1016/j.immuni.2007.03.006
31. Huntington ND, Legrand N, Alves NL, Jaron B, Weijer K, Plet A, et al. IL-15 trans-presentation promotes human NK cell development and differentiation in vivo. J Exp Med. (2009) 206:25–34. doi: 10.1084/jem.20082013
32. Castillo EF, Schluns KS. Regulating the immune system via IL-15 transpresentation. Cytokine. (2012) 59:479–90. doi: 10.1016/j.cyto.2012.06.017
33. Zanoni I, Spreafico R, Bodio C, Di Gioia M, Cigni C, Broggi A, et al. IL-15 cis presentation is required for optimal NK cell activation in lipopolysaccharide-mediated inflammatory conditions. Cell Rep. (2013) 4:1235–49. doi: 10.1016/j.celrep.2013.08.021
34. Sakaguchi S. Regulatory T cells: history and perspective. Methods Mol Biol. (2011) 707:3–17. doi: 10.1007/978-1-61737-979-6_1
35. Lenardo M, Chan KM, Hornung F, McFarland H, Siegel R, Wang J, et al. Mature T lymphocyte apoptosis—immune regulation in a dynamic and unpredictable antigenic environment. Annu Rev Immunol. (1999) 17:221–53. doi: 10.1146/annurev.immunol.17.1.221
36. Snow AL, Pandiyan P, Zheng L, Krummey SM, Lenardo MJ. The power and the promise of restimulation-induced cell death in human immune diseases. Immunol Rev. (2010) 236:68–82. doi: 10.1111/j.1600-065X.2010.00917.x
37. Brentjens RJ, Latouche JB, Santos E, Marti F, Going MC, Lyddane C, et al. Eradication of systemic B-cell tumors by genetically targeted human T lymphocytes co-stimulated by CD80 and interleukin-15. Nat Med. (2003) 9:279–86. doi: 10.1038/nm827
38. Munger W, DeJoy SQ, Jeyaseelan R Sr, Torley LW, Grabstein KH, Eisenmann J, et al. Studies evaluating the antitumor activity and toxicity of interleukin-15, a new T cell growth-factor: comparison with interleukin-2. Cell Immunol. (1995) 165:289–93. doi: 10.1006/cimm.1995.1216
39. Klebanoff CA, Finkelstein SE, Surman DR, Lichtman MK, Gattinoni L, Theoret MR, et al. IL-15 enhances the in vivo antitumor activity of tumor-reactive CD8(+) T cells. Proc Natl Acad Sci USA. (2004) 101:1969–74. doi: 10.1073/pnas.0307298101
40. Teague RM, Sather BD, Sacks JA, Huang MZ, Dossett ML, Morimoto J, et al. Interleukin-15 rescues tolerant CD8(+) T cells for use in adoptive immunotherapy of established tumors. Nat Med. (2006) 12:335–41. doi: 10.1038/nm1359
41. Sneller MC, Kopp WC, Engelke KJ, Yovandich JL, Creekmore SP, Waldmann TA, et al. IL-15 administered by continuous infusion to rhesus macaques induces massive expansion of CD8+ T effector memory population in peripheral blood. Blood. (2011) 118:6845–8. doi: 10.1182/blood-2011-09-377804
42. Waldmann TA, Lugli E, Roederer M, Perera LP, Smedley JV, Macallister RP, et al. Safety (toxicity), pharmacokinetics, immunogenicity, and impact on elements of the normal immune system of recombinant human IL-15 in rhesus macaques. Blood. (2011) 117:4787–95. doi: 10.1182/blood-2010-10-311456
43. Conlon KC, Lugli E, Welles HC, Rosenberg SA, Fojo AT, Morris JC, et al. Redistribution, hyperproliferation, activation of natural killer cells and CD8 T cells, and cytokine production during first-in-human clinical trial of recombinant human interleukin-15 in patients with cancer. J. Clin. Oncol. (2015) 33:74–82. doi: 10.1200/JCO.2014.57.3329
44. Miller JS, Morishima C, McNeel DG, Patel MR, Kohrt HEK, Thompson JA, et al. A first-in-human phase I study of subcutaneous outpatient recombinant human IL15 (rhIL15) in adults with advanced solid tumors. Clin Cancer Res. (2018) 24:1525–35. doi: 10.1158/1078-0432.CCR-17-2451
45. Conlon KC, Potter EL, Pittaluga S, Lee CCR, Miljkovic MD, Fleisher TA, et al. IL-15 by continuous intravenous infusion to adult patients with solid tumors in a Phase I trial induced dramatic NK-cell subset expansion. Clin Cancer Res. (2019) 25:4945–54. doi: 10.1158/1078-0432.CCR-18-3468
46. Romee R, Cooley S, Berrien-Elliott MM, Westervelt P, Verneris MR, Wagner JE, et al. First-in-human phase 1 clinical study of the IL-15 superagonist complex ALT-803 to treat relapse after transplantation. Blood. (2018) 131:2515–27. doi: 10.1182/blood-2017-12-823757
47. Margolin K, Morishima C, Velcheti V, Miller JS, Lee SM, Silk AW, et al. Phase I trial of ALT-803, a novel recombinant IL-15 complex, in patients with advanced solid tumors. Clin Cancer Res. (2018) 24:5552–61. doi: 10.1158/1078-0432.ccr-18-0945
48. Felices M, Lenvik AJ, McElmurry R, Chu S, Hinderlie P, Bendzick L, et al. Continuous treatment with IL-15 exhausts human NK cells via a metabolic defect. JCI Insight. (2018) 3:3. doi: 10.1172/jci.insight.96219
49. Dubois S, Conlon KC, Muller JR, Hsu-Albert J, Beltran N, Bryant BR, et al. IL15 infusion of cancer patients expands the subpopulation of cytotoxic CD56bright NK cells and increases NK-cell cytokine release capabilities. Cancer Immunol Res. (2017) 5:929–38. doi: 10.1158/2326-6066.CIR-17-0279
50. Zhu X, Marcus WD, Xu W, Lee HI, Han K, Egan JO, et al. Novel human interleukin-15 agonists. J Immunol. (2009) 183:3598–607. doi: 10.4049/jimmunol.0901244
51. Chertova E, Bergamaschi C, Chertov O, Sowder R, Bear J, Roser JD, et al. Characterization and favorable in vivo properties of heterodimeric soluble IL-15-IL-15Rα cytokine compared to IL-15 monomer. J Biol Chem. (2013) 288:18093–103. doi: 10.1074/jbc.M113.461756
52. Bergamaschi C, Rosati M, Jalah R, Valentin A, Kulkarni V, Alicea C, et al. Intracellular interaction of interleukin-15 with its receptor alpha during production leads to mutual stabilization and increased bioactivity. J Biol Chem. (2008) 283:4189–99. doi: 10.1074/jbc.M705725200
53. Bergamaschi C, Bear J, Rosati M, Beach RK, Alicea C, Sowder R, et al. Circulating IL-15 exists as heterodimeric complex with soluble IL-15Rα in human and mouse serum. Blood. (2012) 120:e1–8. doi: 10.1182/blood-2011-10-384362
54. Bergamaschi C, Watson DC, Valentin A, Bear J, Peer CJ, Figg WD Sr, et al. Optimized administration of hetIL-15 expands lymphocytes and minimizes toxicity in rhesus macaques. Cytokine. (2018) 108:213–24. doi: 10.1016/j.cyto.2018.01.011
55. Vincent M, Teppaz G, Lajoie L, Sole V, Bessard A, Maillasson M, et al. Highly potent anti-CD20-RLI immunocytokine targeting established human B lymphoma in SCID mouse. MAbs. (2014) 6:1026–37. doi: 10.4161/mabs.28699
56. Chen X, Liu B, Han K, Kong L, Noel T, Jeng EK, et al. Combination therapy of an IL-15 superagonist complex, ALT-803, and a tumor targeting monoclonal antibody promotes direct antitumor activity and protective vaccinal effect in a syngenic mouse melanoma model. J Immunother Cancer. (2015) 3(Suppl 2):P347. doi: 10.1186/2051-1426-3-S2-P347
57. Furuya H, Chan OTM, Pagano I, Zhu C, Kim N, Peres R, et al. Effectiveness of two different dose administration regimens of an IL-15 superagonist complex (ALT-803) in an orthotopic bladder cancer mouse model. J Transl Med. (2019) 17:29. doi: 10.1186/s12967-019-1778-6
58. Schmohl JU, Felices M, Taras E, Miller JS, Vallera DA. Enhanced ADCC and NK cell activation of an anticarcinoma bispecific antibody by genetic insertion of a modified IL-15 cross-linker. Mol Ther. (2016) 24:1312–22. doi: 10.1038/mt.2016.88
59. Mortier E, Quéméner A, Vusio P, Lorenzen I, Boublik Y, Grötzinger J, et al. Soluble interleukin-15 receptor alpha (IL-15R alpha)-sushi as a selective and potent agonist of IL-15 action through IL-15R beta/gamma. Hyperagonist IL-15 X IL-15R alpha fusion proteins. J Biol Chem. (2006) 281:1612–9. doi: 10.1074/jbc.M508624200
60. Bessard A, Solé V, Bouchard G, Quéméner A, Jacques Y. High antitumor activity of RLI, an interleukin-15 (IL-15)-IL-15 receptor alpha fusion protein, in metastatic melanoma and colorectal cancer. Mol Cancer Ther. (2009) 8:2736–45. doi: 10.1158/1535-7163.MCT-09-0275
61. Vincent M, Bessard A, Cochonneau D, Teppaz G, Solé V, Maillasson M, et al. Tumor targeting of the IL-15 superagonist RLI by an anti-GD2 antibody strongly enhances its antitumor potency. Int J Cancer. (2013) 133:757–65. doi: 10.1002/ijc.28059
62. Zhang M, Wen B, Anton OM, Yao Z, Dubois S, Ju W, et al. IL-15 enhanced antibody-dependent cellular cytotoxicity mediated by NK cells and macrophages. Proc Natl Acad Sci USA. (2018) 115:E10915–24. doi: 10.1073/pnas.1811615115
63. Roberti MP, Barrio MM, Bravo AI, Rocca YS, Arriaga JM, Bianchini M, et al. IL-15 and IL-2 increase cetuximab-mediated cellular cytotoxicity against triple negative breast cancer cell lines expressing EGFR. Breast Cancer Res Treat. (2011) 130:465–75. doi: 10.1007/s10549-011-1360-2
64. Rosario M, Liu B, Kong L, Collins LI, Schneider SE, Chen X, et al. The IL-15-based ALT-803 complex enhances Fc gamma RIIIα-triggered NK cell responses and in vivo clearance of B cell lymphomas. Clin Cancer Res. (2016) 22:596–608. doi: 10.1158/1078-0432.CCR-15-1419
65. Moga E, Cantó E, Vidal S, Juarez C, Sierra J, Briones J. Interleukin-15 enhances rituximab-dependent cytotoxicity against chronic lymphocytic leukemia cells and overcomes transforming growth factor beta-mediated immunosuppression. Exp Hematol. (2011) 39:1064–71. doi: 10.1016/j.exphem.2011.08.006
66. Liu B, Kong L, Han K, Hong H, Marcus WD, et al. A novel fusion of ALT-803 (interleukin (IL)-15 superagonist) with an antibody demonstrates antigen-specific antitumor responses. J Biol Chem. (2016) 291:23869–81. doi: 10.1074/jbc.M116.733600
67. Zhang M, Yao Z, Dubois S, Ju W, Muller JR, Waldmann TA. Interleukin-15 combined with an anti-CD40 antibody provides enhanced therapeutic efficacy for murine models of colon cancer. Proc Natl Acad Sci USA. (2009) 106:7513–8. doi: 10.1073/pnas.0902637106
68. Zhang ML, Ju W, Yao Z, Yu P, Wei BR, Simpson RM, et al. Augmented IL-15Rα expression by CD40 activation is critical in synergistic CD8 T cell-mediated antitumor activity of anti-CD40 antibody with IL-15 in TRAMP-C2 tumors in mice. J Immunol. (2012) 188:6156–64. doi: 10.4049/jimmunol.1102604
69. Yu P, Steel JC, Zhang M, Morris JC, Waitz R, Fasso M, et al. Simultaneous inhibition of two regulatory T-cell subsets enhanced Interleukin-15 efficacy in a prostate tumor model. Proc Natl Acad Sci USA. (2012) 109:6187–92. doi: 10.1073/pnas.1203479109
70. Yu P, Steel JC, Zhang M, Morris JC, Waldmann TA. Simultaneous blockage of multiple immune system inhibitory checkpoints enhances antitumor activity mediated by interleukin-15 in a murine metastatic colon carcinoma model. Clin Cancer Res. (2010) 16:6019–28. doi: 10.1158/1078-0432.CCR-10-1966
71. Zhao M, Luo M, Xie Y, Jiang H, Cagliero C, Li N, et al. Development of a recombinant human IL-15. SIL-15Rα/Fc superagonist with improved half-life and its antitumor activity alone or in combination with PD-1 blockade in mouse model. Biomed Pharmacother. (2019) 112:10867. doi: 10.1016/j.biopha.2019.108677
72. Kowalsky SJ, Liu Z, Feist M, Berkey SE, Ma C, Ravindranathan R, et al. Superagonist IL-15-armed oncolytic virus elicits potent antitumor immunity and therapy that are enhanced with PD-1 blockade. Mol Ther. (2018) 26:2476–86. doi: 10.1016/j.ymthe.2018.07.013
73. Knudson KM, Hicks KC, Alter S, Schlom J, Gameiro SR. Mechanisms involved in IL-15 superagonist enhancement of anti-PD-L1 therapy. J Immunother Cancer. (2019) 7:82. doi: 10.1186/s40425-019-0551-y
74. Wrangle JM, Velcheti V, Patel MR, Garrett-Mayer E, Hill EG, Ravenel JG, et al. ALT-803, an IL-15 superagonist, in combination with nivolumab in patients with metastatic non-small cell lung cancer: a non-randomised, open-label, phase 1b trial. Lancet Oncol. (2018) 19:694–704. doi: 10.1016/S1470-2045(18)30148-7
75. Cooley S, He F, Bachanova V, Vercellotti GM, DeFor TE, Curtsinger JM, et al. First-in-human trial of rhIL-15 and haploidentical natural killer cell therapy for advanced acute myeloid leukemia. Blood Adv. (2019) 3:1970–80. doi: 10.1182/bloodadvances.2018028332
76. Miljkovic MD, et al. Interleukin-15 (IL-15) and alemtuzumab for relapsed/refractory mature T-cell malignancies [abstract]. In: Proceedings of the 2020 T-cell Lymphoma Forum. La Jolla, CA (2018).
77. Delconte RB, Kolesnik TB, Dagley LF, Rautela J, Shi W, Putz EM, et al. CIS is a potent checkpoint in NK cell-mediated tumor immunity. Nat Immunol. (2016) 17:816–24. doi: 10.1038/ni.3470
78. Bottino C, Dondero A, Moretta A, Castriconi R. CIS is a negative regulator of IL-15-mediated signals in NK cells. Transl Cancer Res. (2016) 5:(Suppl 4):S875–7. doi: 10.21037/tcr.2016.10.79
79. Sckisel GD, Bouchlaka MN, Monjazeb AM, Crittenden M, Curti BD, Wilkins DE, et al. Out-of-sequence signal 3 paralyzes primary CD4(+) T-cell-dependent immunity. Immunity. (2015) 43:240–50. doi: 10.1016/j.immuni.2015.06.023
80. Bennett SR, Carbone FR, Karamalis F, Flavell RA, Miller JF, Health WR. Help for cytotoxic-T-cell responses is mediated by CD40 signalling. Nature. (1998) 393:478–80. doi: 10.1038/30996
81. Ridge JP, Di Rosa F, Matzinger P. A conditioned dendritic cell can be a temporal bridge between a CD4+ T-helper and a T-killer cell. Nature. (1998) 393:474–8. doi: 10.1038/30989
82. Schoenberger SP, Toes RE, van der Voort EI, Offringa R, Melief CJ. T-cell help for cytotoxic T lymphocytes is mediated by CD40-CD40L interactions. Nature. (1998) 393:480–3. doi: 10.1038/31002
83. Li F, Ravetch JV. Inhibitory Fcγ receptor engagement drives adjuvant and anti-tumor activities of agonistic CD40 antibodies. Science. (2011) 333:1030–4. doi: 10.1126/science.1206954
84. Dahan R, Barnhart BC, Li F, Yamnjuk AP, Korman AJ, Ravetch JV. Therapeutic activity of agonistic, human anti-CD40 monoclonal antibodies requires selective FcγR engagement. Cancer Cell. (2016) 29:820–31. doi: 10.1016/j.ccell.2016.05.001
85. Mujib S, Jones RB, Lo C, Aidarus N, Clayton K, Sakhdari A, et al. Antigen-independent induction of Tim-3 expression on human T cells by the common γ-chain cytokines IL-2, IL-7, IL-15 and IL-21 is associated with proliferation and is dependent on the phosphoinositide 3-kinase pathway. J Immunol. (2012) 188:3745–56. doi: 10.4049/jimmunol.1102609
86. Chew GM, Fujita T, Webb GM, Burwitz BJ, Wu HL, Reed JS, et al. TIGIT marks exhausted T cells, correlates with disease progression, and serves as a target for immune restoration in HIV and SIV infection. PLoS Pathog. (2016) 12:e1005349. doi: 10.1371/journal.ppat.1005349
87. Rifa'i M, Shi Z, Zhang SY, Lee YH, Shiku H, Isobe KI, et al. CD8+CD122+ regulatory T cells recognize activated T cells via conventional MHC class I-alphabeta TCR interaction and become IL-10-producing active regulatory cells. Int Immunol. (2008) 20:937–47. doi: 10.1093/intimm/dxn052
Keywords: interleukin-15, natural killer cells, CD8 T cells, immunotherapy of cancer, immunological checkpoints
Citation: Waldmann TA, Dubois S, Miljkovic MD and Conlon KC (2020) IL-15 in the Combination Immunotherapy of Cancer. Front. Immunol. 11:868. doi: 10.3389/fimmu.2020.00868
Received: 10 January 2020; Accepted: 15 April 2020;
Published: 19 May 2020.
Edited by:
Erwan Mortier, INSERM U1232 Centre de Recherche en Cancérologie et Immunologie Nantes Angers (CRCINA), FranceReviewed by:
Piergiuseppe De Berardinis, Istituto di biochimica delle proteine (IBP), ItalyCopyright © 2020 Waldmann, Dubois, Miljkovic and Conlon. This is an open-access article distributed under the terms of the Creative Commons Attribution License (CC BY). The use, distribution or reproduction in other forums is permitted, provided the original author(s) and the copyright owner(s) are credited and that the original publication in this journal is cited, in accordance with accepted academic practice. No use, distribution or reproduction is permitted which does not comply with these terms.
*Correspondence: Thomas A. Waldmann, dGF3YWxkQG1haWwubmloLmdvdg==
Disclaimer: All claims expressed in this article are solely those of the authors and do not necessarily represent those of their affiliated organizations, or those of the publisher, the editors and the reviewers. Any product that may be evaluated in this article or claim that may be made by its manufacturer is not guaranteed or endorsed by the publisher.
Research integrity at Frontiers
Learn more about the work of our research integrity team to safeguard the quality of each article we publish.