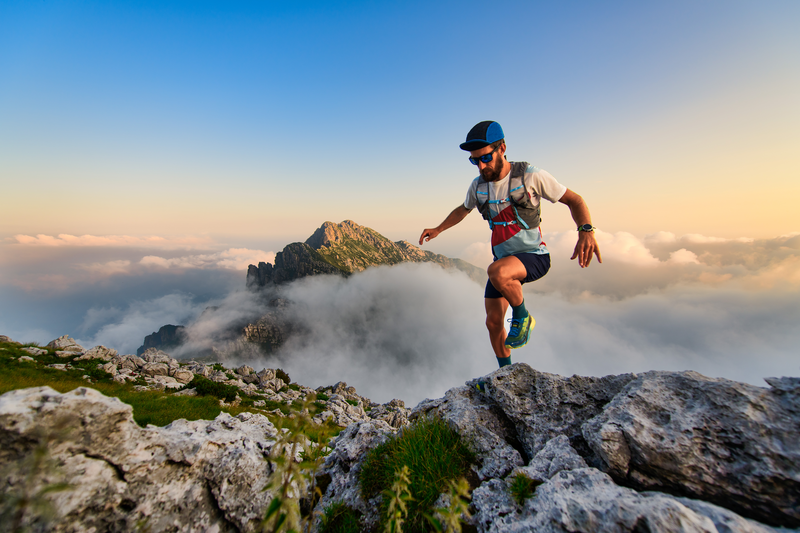
94% of researchers rate our articles as excellent or good
Learn more about the work of our research integrity team to safeguard the quality of each article we publish.
Find out more
MINI REVIEW article
Front. Immunol. , 12 May 2020
Sec. Alloimmunity and Transplantation
Volume 11 - 2020 | https://doi.org/10.3389/fimmu.2020.00833
This article is part of the Research Topic Transplantation of Marginal Organs: Immunological Aspects and Therapeutic Perspectives View all 17 articles
Donor organ shortage, growing waiting lists and substantial organ discard rates are key problems in transplantation. The critical importance of organ quality in determining long-term function is becoming increasingly clear. However, organ quality is difficult to predict. The lack of good measures of organ quality is a serious challenge in terms of acceptance and allocation of an organ. The underlying review summarizes currently available methods used to assess donor organ quality such as histopathology, clinical scores and machine perfusion characteristics with special focus on molecular analyses of kidney quality. The majority of studies testing molecular markers of organ quality focused on identifying organs at risk for delayed graft function, yet without prediction of long-term graft outcome. Recently, interest has emerged in looking for molecular markers associated with biological age to predict organ quality. However, molecular gene sets have not entered the clinical routine or impacted discard rates so far. The current review critically discusses the potential reasons why clinically applicable molecular quality assessment using early kidney biopsies might not have been achieved yet. Besides a critical analysis of the inherent limitations of surrogate markers used for organ quality, i.e., delayed graft function, the intrinsic methodological limitations of studies assessing organ quality will be discussed. These comprise the multitude of unpredictable hits as well as lack of markers of nephron mass, functional reserve and regenerative capacity.
Good organ quality is the basis for successful long-term transplant outcome. The ability to withstand and repair immune and non-immune mediated injury and the number of nephrons to match the increased and persistent metabolic demand to a single kidney characterize optimal kidney organ quality with the potential to best long-term function. Hence, a robust assessment of kidney quality at time of transplantation is needed, in particular in donors with suboptimal conditions, i.e., marginal donors with old age, uncertain medical history, long ischemia time or pre-donation renal failure. In case of doubt clinicians will err on the side of caution and decide on discarding the organ, despite organ shortage and growing waiting lists. This is reflected in the high kidney discard rates in the US despite significant efforts to expand the donor pool. Nearly 20% of kidneys recovered are discarded, mainly based on procurement biopsies as method to assess organ quality (1–4). In Europe, where procurement biopsies are rarely performed, kidney discard rates are significantly lower and this is associated with saved patient life years (4, 5). This difference between US and European allocation practice underscores the need for more reliable and objective methods for organ quality assessment, especially in marginal donors, to decrease the number of discarded organs. So far, no evaluation process has sufficient discriminatory potential to guide the clinician and implanting surgeon team whether to accept or discard an organ. Currently available methods for assessment of organ quality are summarized in Table 1 and discussed in the following paragraphs.
Table 1. Comparison of different assessment tools to evaluate organ quality in kidney transplantation.
In 1995, the seminal paper on procurement biopsies by Gaber et al. presented a significantly increased rate of delayed graft function (DGF) and graft loss with glomerulosclerosis of >20% (6). However, accumulating data in the last 25 years questions the utility of procurement biopsies for evaluating donor kidneys (3, 7, 8). A systematic review by Wang et al. reported that all 47 published studies on kidney biopsies were retrospective, poor in design, and the results were heterogeneous. The percent glomerulosclerosis was most often examined and failed to predict graft failure in 7 out of 14 studies (7).
Analyzing biopsy findings it is necessary to distinguish between pre-implantation biopsies, performed immediately before implantation, and procurement biopsies, taken at time of donor kidney retrieval (9). Histology, in contrast to molecular changes, is expected to be similar in pre-implantation and procurement biopsies. For allocation purposes, focus lies on the procurement biopsy. As time is an important factor in the allocation process, these biopsies are evaluated on frozen sections stained with hematoxylin-eosin and not in paraffin-embedded tissues stained with periodic-acid-schiff, masson trichrome, and methenamine silver. Also, evaluation is done by on-call pathologists often not by an experienced renal pathologist. Furthermore, no consensus exists regarding use of wedge biopsies or core needle biopsies. All these factors pose problems. Hence, classification of histological lesions might differ when evaluated on frozen vs. paraffin embedded sections and interpretation might vary between on-call pathologists vs. experienced nephro pathologists contributing to the poor quality with missing information, lack of concordance and reproducibility (8–12). Even the agreement between expert renal transplant pathologists were only moderate to poor at Banff Histopathological Consensus meeting for preimplantation kidney biopsies with most interclass correlations less than 0.5 (12). In addition, intrinsic differences between wedge biopsies, that preferentially evaluate the subcapsular zone overestimating glomerulosclerosis, and core needle biopsies, that preferentially represent the cortex, further impact comparisons between various practices of procurement biopsies (9).
Finally, no consensus exists regarding the grading system to be used for interpretation of procurement biopsies. Besides the Banff grading system scoring individual lesions (12), several composite histological scoring systems have been described (7, 9). Yet, most histological composite scores lack validation in independent cohorts as well as testing of their predictive power in multivariate analyses including donor age and organ function and hence they might erroneously appear as independent predictors of graft failure. These facts underline the difficulty to predict long-term graft outcome based on histological evaluation of procurement biopsies (13). All these limitations translate into high discordance between two biopsies obtained of the same kidney (8) and also contribute to the high discrepancy in discard rates between centers (2, 3).
Graft survival rates of unilaterally discarded kidneys might indeed still be acceptable for some patients (2, 8). One-year death censored graft survival rate of recipients from unilaterally discarded kidneys due to donor factors (in particular biopsy findings) has been reported to be over 90% and five-year death censored graft survival was >85% (2). This underscores the fact that the currently available scores for organ assessment using histology inaccurately capture organ quality and the gain of life years for the individual patient.
The first clinical parameter found to be negatively associated with graft survival was age (13, 14). Besides age, established cardiovascular co-morbidities also associate with graft survival (15). Hence, common variables used in all scoring systems include donor age, history of hypertension and serum creatinine, altogether being surrogate markers of reduced nephron mass and extent of established injury and repair capacity, key donor factors contributing to long-term graft outcome (13, 16). However, these clinical markers lack robustness and standardization as organ quality metrics.
The recently introduced KDRI score (resp. kidney donor percentile index KDPI) reflects the rate of graft failure relative to a healthy 40-year old donor. This score was originally based on 14 donor characteristics (donor age, race, history of hypertension, history of diabetes, serum creatinine, cerebrovascular cause of death, height, weight, donation after cardiac death, hepatitis C virus status, HLA-B and -DR mismatch, cold ischemia time, en-bloc kidney transplant, dual kidney transplant) (17–19) and later reduced to 10 variables, as some information may be missing at time of transplantation. This is a far more granular tool for physicians to evaluate the offer and assess generic donor quality and outcome than the previously used dichotomous extended criteria donor (ECD) vs. non-ECD classification. Yet, despite introduction of this more detailed risk index, discard rates in the US remained unchanged at roughly 18–20% (20).
The differences in discard policies and application of the KDRI scores are highlighted in a recently published analysis by Aubert et al. (4). The probability of organ discard for the same KDRI is significantly higher in the United States compared to France and the interpolation of a similar organ use strategy in the United States would generate additional 132’445 allograft life-years over a ten-year observation period with greatest gain of life years through reduced discard of the organs with highest KDRI. These differences in applying the KDRI for accepting organ offers also reflect its limited predictive power. A recent study showed no significant difference in 5-year death-censored graft survival between DCD KDPI 61–81 and DCD KDPI ≥ 85 when used for donation after cardiac death (DCD) kidneys (18). In line with the limited discriminative power regarding graft failure very high KDPI kidneys may reveal acceptable outcomes (21–24). Another group showed 5-year graft survival of 91% using kidneys with KDPI score of 97% as dual transplants, highlighting that besides KDRI, nephron mass plays a major role with respect to graft survival (14, 25). A further a critical issue when using KDRI/KDPI is Hepatitis C virus (HCV) status having the largest contribution to KDPI (KDRI b coefficient 1/4.24; “Xb” component 1/4.24). However, HCV + kidneys are mostly young donors and at current era with available excellent antiviral treatment for HCV, clinical outcomes are excellent in HCV negative patients receiving HCV + deceased-donors (26). The other important critical component of KDPI is the pre-donation serum creatinine level, which might be “falsely” high due to acute kidney injury from acute tubular necrosis. In a multicenter deceased donor study of 2,430 kidneys transplanted from 1,298 deceased donors 585 (24%) were from donors with AKI. The analysis did not show any significant difference in graft survival at 4 years by donor AKI stage (27).
All these articles question the utility of KDRI/KDPI as single decision tool with respect to kidney discard policies. Even though KDRI/KDPI has repeatedly been shown to associate with graft failure, a high KDRI/KDPI is not synonymous with graft failure and underscores its limited discriminative power as single decision tool.
Research and applications of machine-based organ preservation have experienced a significant revival with the goals to reduce peri-transplant ischemia reperfusion injury, to facilitate assessment of organ quality and directed organ therapies, and to decrease the number of marginal organs to be discarded. First described as early as 1935 by Carrel and Lindbergh (28), interest in organ perfusion has re-emerged with the landmark trial published by Moers et al. (29). Machine perfusion for organ preservation was associated with a reduction of DGF compared to cold storage and its application has led to reduced discard rates of organs (30). However, these positive effects on short-term function did so far not translate into a marked improvement in long-term outcomes (31, 32). However, more sophisticated perfusion methods and cell-based therapies are investigated. Currently hypothermic machine perfusion is the most widely used technique, in recent years normothermic machine perfusion is gaining interest (33).
In addition to positively impacting reperfusion injury and organ preservation, machine perfusion also offers the opportunity for organ quality assessment based on perfusate analysis or measurement of perfusion dynamics such as intravascular renal resistance. The largest randomized controlled trial prospectively assessing renal intravascular resistive indexes on hypothermic machine perfusion and its association with graft outcome by Jochmans et al. showed that renal resistance at the end of hypothermic machine perfusion is an independent risk factor for both DGF and 1-year graft failure, yet the predictive power was low with a c-statistic of only 0.58 (34). Similar findings are reported by de Vries et al. and Parikh et al., showing only modest correlation with early graft function (35, 36). Likewise, perfusate analyses indicated that biomarkers, such as NAG or H-FABP, are associated with DGF, but again with low predictive value in differentiating functioning versus non-functioning grafts (37). Another group described levels of microRNA-21 (miR-21) to correlate with early graft function, but no data on association with long-term graft function is available (38). Hence, so far, neither dynamic machine perfusion characteristics such as renal resistance nor machine perfusate biomarkers can be used as stand-alone criteria for organ quality assessment with sufficient precision (36, 39).
Yet, novel techniques using normothermic perfusion allow for further assessments of functional parameter in addition to the above described flow/resistance markers. Hosgood et al. (40). described an ex vivo kidney perfusion quality assessment score (EVKP score) based on macroscopic appearance, renal blood flow and urine output after ex vivo normothermic kidney perfusion correlating with DGF but not long-term outcome. The same group correlated urine biomarkers of injury with this score. They measured a significant correlation between levels of urinary endothelin-1 and NGAL and perfusate parameters as well as between the EVKP score and donor creatinine at organ retrieval, while no correlation was found for KIM-1 (41). Similar results, reporting a lack of correlation of KIM-1 with donor AKI, have also been reported by other groups, likely due to the fact that KIM-1, in contrast to NGAL, is a rather late marker of kidney injury. However, the predictive power of these urinary biomarkers, despite being sensitive for structural kidney damage, is still unclear. Of note, a large multicenter deceased donor study of 2,430 kidney transplant recipients from 1298 donors did not find an association of the donor urine injury biomarkers microalbumin, NGAL, KIM-1, IL-18, and L-FABP with graft failure at a median follow-up of 4 years, questioning the predictive utility of urinary biomarker measurements during normothermic ex vivo perfusion (42).
Future evaluations will show whether novel techniques, such as normothermic machine perfusion, may allow better assessment of organ quality and function under near-physiological conditions (43). A key advantage of machine perfusion might well be the additional time gained for organ evaluation and the clinical decision to use or not use the organ.
As outlined above, evaluation of organ quality by clinical scores, histopathology or perfusion characteristics lacks discriminatory power to guide clinicians to accept or discard an organ, in particular in the situation of marginal donors.
Over the recent years molecular analysis of biopsy samples has become a reliable, technically robust, not too expensive methodology including transcriptome, proteome and metabolome technologies. The unbiased, quantitative “omics’ approaches have become standard of care in oncology, classifying tumors and individualizing therapy. Hence, great expectations have been based on molecular diagnostics as they potentially offer an alternative, more objective and quantitative method for organ evaluation. Molecular profiling indeed demonstrated to go beyond histopathologic evaluation being able to detect changes not captured by histopathology. In a previous review we have summarized molecular studies of 0-hour biopsies (both pre-implantation and post-reperfusion) published till 2010 (44). It could be shown that transcriptome profiles provide a quantitative measurement of inflammatory burden, detect coordinated activation of pathways of immune activation, defense response, oxidative stress and a parallel inhibition of metabolism and transport or ion binding. In particular, transcriptome patterns identified changes in kidneys such as susceptibility to DGF, which was not reflected using clinical and histopathological scores (45). However, despite the number of promising findings, no robust set of predictive molecular markers for organ quality measurement had been identified in these early studies.
Since then a number of new studies have been conducted to further assess the potential to evaluate organ quality and transplant outcomes. Table 2 summarizes studies on molecular analyses of peri-transplant biopsies assessing organ quality that have been published since 2011 and are listed in PubMed.
Table 2. Molecular diagnostics of early kidney transplant biopsies [summarizing studies published since 2011, studies published before were previously summarized (44)].
A majority of studies focused on DGF as a surrogate marker for organ quality and early outcome (46–52). They largely confirm the earlier findings that DGF is usually better predicted with molecular changes than histology or clinical scores at time of transplantation (46, 49–52). They identified molecular changes associated with kidney injury (such as NGAL, syn. LCN2, KIM1, syn. HAVCR1, NTN1) (46, 49) and aging (CDKN2A) (50–52) as DGF biomarkers. However, these peri-transplant changes associated with DGF did not allow a robust prediction of medium- to long-term functional outcomes.
Mas and her group showed that transcript changes associated with early kidney function but not with DGF per se correlate with outcome. Kidneys with DGF and also a low GFR at 1-month post-transplant showed inferior medium- to long-term outcomes. The pre-implantation biopsies of these kidneys showed an increased expression of pathways associated with immune activation and inflammation. Gene transcripts of CCL5, CXCR4, and ITGB2 discriminated best between low vs. high GFR. This difference in kidney function remained throughout the period of observation of 2 years (48, 53). Findings of the Halloran group confirm the lack of predictive power of gene changes associated with DGF (54, 55). They identified gene transcript changes associated with AKI in transplant biopsies. These so-called injury and repair associated transcripts (IRRATs) correlate with degree of injury, repair capacity and functional outcome but not with DGF (54, 55). However, with a sufficient long-term follow up of more than 2 years, peri-transplant molecular phenotypes at time of, or early after transplantation seem not to correlate with medium- to long-term transplant function. Molecular changes in 6-week protocol biopsies correlated with atrophy and scarring at 6 months but not with future functional decline (47), implant biopsies did not predict late function (54, 55). In contrast, long-term function correlated with histopathology changes associated with aging or clinical scores, in particular donor age (53, 55).
Consequently a number of studies focused on molecular markers for biological age as parameters for organ quality (50–52). In particular increased expression of CDKN2A associated with graft function, probably better reflecting the allostatic load of “wear and tear” of an organ and its resilience to cope with the peri- and post-transplant stressors (50–52). However, the clear added value of markers of biological age like CDKN2A or others like telomere length, microRNAs or epigenetic changes to the simple measurement of chronological age is not clear. In addition, the age allocation bias, i.e., old kidneys are predominantly given to old recipients, and hence likely poorer quality organs are transplanted into recipients with more comorbidities and inferior outcomes, makes it difficult to identify and validate robust quality markers in old kidneys (55).
An interesting, recent study analyzed gene expression in cell infiltrates at time of transplantation and 4 months post-transplant (56). This study indicated gene expression of inflammatory and fibrotic markers at 4 months, and differences between 4 months and baseline, correlated negatively with renal function up to 5 years. Another small, exploratory but cutting-edge methodology study by Kaisar et al. (57). suggests that proteomics analyses are able to discriminate different outcomes that were not predicted by common evaluation methods such as clinical (KDPI), histology or AKI scores (57). These promising studies need further validation and larger numbers.
In general none of the molecular analyses outlined here have entered the clinical routine diagnostics and organ quality is still evaluated exclusively by clinical and histopathology-based scores.
The question is why these molecular analyses have not yet identified robust quality markers and hence successfully translated into clinical useful tests? This might be due to intrinsic limitations of molecular studies, selection of insufficient surrogate markers and end points for outcome studies, or the principal unpredictability of long-term outcomes with donor organ characteristics given heterogeneity and multitude of hits during the post-transplant life of the donor kidney.
Molecular analyses of donor kidney biopsies might not depict structural changes or reflect nephron mass. They measure tissue cell mixtures depending on the location of the biopsy site, cannot predict the multitude of additional immune and non-immune hits and recipient factors that occur in the long run. They are drowned by the tidal wave in expression changes due to brain death and the associated SIRS-like syndrome.
The surrogate markers for kidney quality used for the identification of molecular changes is another likely reason for the lack of established kidney quality profiles. Delayed graft function, chronological rather than biological age, incomplete disease phenotyping, weak markers of kidney function (such as creatinine), short follow-up periods, small samples sizes or lack of validation studies all contribute to the still unfulfilled promise of molecular diagnostics for organ quality assessment.
Pre-transplantation assessments of organ quality in non-kidney solid organs primarily rely on clinical scores and markers assessed during ex vivo machine perfusion. Comparable to kidney transplantation there is no established molecular assessment of biopsy samples and few examples are given below. In-depth analysis of organ quality assessment measures for other organs than the kidney is out of the scope of this review.
In liver transplantation, organ quality has been correlated with cumulative bile acid production and coagulation parameters (58). Also metabolomic signatures associated with early graft function comprising key pathways involved in lipid homeostasis and histidine pathway have been described (59). With respect to analysis of molecular markers, investigation of microRNA profiles in graft preservation solutions has been shown to be predictive of ischemic-type biliary lesions after liver transplantation, which are the second most common cause of graft failure after liver transplantation (60). The ratio of hepatocyte to cholangiocyte-derived miRNAs (with special focus of miR 122 and miR 222) was predictive of graft viability (60–62). In pancreas transplantation, assessment of organ quality is performed during machine perfusion measuring insulin secretion, acid-base balance and perfusion characteristics (63). Likewise, in lung transplantation organ quality assessment is reported through ventilation parameters, analysis of arterial blood gases on perfusate samples with recent focus on metabolic components of glucose consumption and lactate production (64). Other groups indicated that levels of inflammatory cytokines (65, 66), endothelin-1 (67), adhesion molecules (68) or neutrophil extracellular traps (69) in lung perfusate are associated with post-transplant primary graft function. Similarly, assessment of donor heart quality prior to transplantation is attempted by analyzing perfusate during machine perfusion (70).
The majority of published studies on molecular assessment of organ quality used DGF, i.e., transient renal failure immediately post-transplantation, as surrogate marker for graft quality and outcome. This is based on the association of reduced graft survival of DGF kidneys in standard brain death donors (DBD) shown in some, but not in all studies. The limitations of DGF as a surrogate outcome marker for poorer organ quality is highlighted by the excellent quality and long-term outcomes of DCD organs. Despite the high percentage of DGF cases these positively selected cases with usually young age and lack of comorbidities show good long-term outcomes. Similar lack of correlation with longer-term outcomes and DGF is seen analyzing mate kidneys. Donor characteristics rather than ischemia times or DGF rates determine the long-term performance (71–74).
Moreover, definition of DGF is not uniform (71). More than 10 different definitions are used and most importantly none of them was associated with poorer graft survival in DCD kidneys (71). The limitations of DGF as a quality marker is further underlined as so far no treatment of DGF translated into significant improvement in long-term outcome (75).
Patho-physiologically the higher risk of DGF in DCD donors compared to DBD donors can be explained by the unavoidable extended warm ischemia time and associated increased ischemia-reperfusion injury. However, full recovery and excellent long-term graft outcome underline repair capacity and nephron mass as organ quality determinants.
Hence, not DGF per se but rather ability to recover from DGF as indicated, e.g., by GFR at 1 month might be a more reliable marker of long-term graft outcome and quality, as recently reported by Lee et al. (76). Donor age, donor final creatinine and cold ischemia time were significantly associated with DGF recovery status (76). DGF is a syndrome and duration of DGF and degree of acute kidney injury is associated with renal outcome, in transplant and non-transplant settings (77, 78). Extent of recovery presumably reflects the intrinsic repair capacity of the donor organ. Age strongly defines repair capacity and this might explain donor age as the most widely used criterion in all clinical scores assessing organ quality pre-transplantation (73, 79).
In summary, the post-transplant course is determined by donor factors, acute peri-transplantation injury as well as recipient factors. DGF per se is a poor, but most frequently used, surrogate marker for organ quality (see Table 2). Hence, the focus on identifying DGF-associated molecular patterns might be one reason that so far molecular diagnostics of organ quality has not translated into clinical decision making. In addition, molecular assessment of repair capacity and biological tissue aging is still ill defined. Ongoing work on robust molecular markers of biological age is promising (see Table 2) but again has not yet translated into clinical utility.
Successful organ transplantation is largely defined by a good and long-term functioning kidney graft. This requires a sufficient nephron mass to meet the increased, long-term metabolic demand and stresses of a single kidney in a transplant recipient. In the unstable setting of brain death and organ donation donor serum creatinine or estimated GFR are unreliable markers of nephron mass or reserve capacity. The same applies to histopathology and clinical scores. The identification of molecular markers for nephron mass in addition to repair capacity would be most valuable but yet, has not been achieved. This might be due in part to the lack of long-term studies. As shown in Table 2 most studies focus on short-term function. The identification of molecular changes in peri-transplant biopsies that correlate with long-term function is needed.
• Comparison of molecular profiles at implantation biopsy between kidney pairs from the same donor both with high eGFR at 1 year post transplantation (i.e., eGFR > 60 ml/min/1.73 m2) and kidney pairs from the same donor with both low eGFR at 1 year post transplantation (i.e., eGFR < 30 ml/min/1.73 m2). This should primarily reflect intrinsic donor factors rather than post-transplant hits and recipient factors. Note: Ratio for taking follow up of 1 year only: if taking too long follow up (longer than 1 year) recipient factors might become additionally relevant.
• Comparison of molecular profiles at implantation biopsy between kidney with low delta of expected and observed creatinine at 1 year post transplantation (e.g., delta 25%; i.e., kidney with good regeneration capacity) and kidneys with high delta of expected and observed creatinine at 1 year (delta > 25%; i.e., kidney with impaired regeneration capacity). Note: make sure taking only kidney with good match of recipient/donor weight (i.e., R/D weigh ratio of 0.8–1.2 allowed) (80).
• Once molecular profiles of kidney with low regeneration capacity is characterized: comparison of delta expected-observed creatinine at 1 year in high risk kidneys preserved with pumping as compared to delta expected-observed creatinine in high risk kidneys preserved with cold storage.
SM and TM designed the first draft of the manuscript. EA and VM revised the manuscript. All authors approved the final version of the manuscript.
VM is partially funded by grants from National Health Institute (NIH), National Institute of Diabetes and Digestive and Kidney Diseases (NIDDK) (RO1DK109581 and RO1DK22682).
SM declares a travel grant from the Astellas outside from the submitted work.
The remaining authors declare that the research was conducted in the absence of any commercial or financial relationships that could be construed as a potential conflict of interest.
The reviewer MN declared a past co-authorship with several of the authors to the handling Editor.
AKI, acute kidney injury; CIT, cold ischemia time; D, donor; DBD, donation after brain death; DCD, donation after cardiac death; DD, deceased donor; DGF, delayed graft function; ECD, extended criteria donor; EVKP, score ex vivo kidney perfusion score; GFR, glomerular filtration rate; HCV, hepatitis C; I/RI, ischemia reperfusion injury; IRRATs, injury and repair response associated transcripts; KDPI, kidney donor percentile index; KDRI, kidney donor risk index; LD, living donor; MAPI, Maryland aggregate pathology index; PBTs, pathogenesis based transcript sets; PRA, panel reactive antigen; R, recipient; SCD, standard criteria donor; TPL, transplantation.
1. Stewart DE, Garcia VC, Rosendale JD, Klassen DK, Carrico BJ. Diagnosing the decades-long rise in the deceased donor kidney discard rate in the United States. Transplantation. (2017) 101:575–87. doi: 10.1097/TP.0000000000001539
2. Mohan S, Chiles MC, Patzer RE, Pastan SO, Husain SA, Carpenter DJ, et al. Factors leading to the discard of deceased donor kidneys in the United States. Kidney Int. (2018) 94:187–98. doi: 10.1016/j.kint.2018.02.016
3. Lentine KL, Naik AS, Schnitzler MA, Randall H, Wellen JR, Kasiske BL, et al. Variation in use of procurement biopsies and its implications for discard of deceased donor kidneys recovered for transplantation. Am J Transplant. (2019) 19:2241–51. doi: 10.1111/ajt.15325
4. Aubert O, Reese PP, Audry B, Bouatou Y, Raynaud M, Viglietti D, et al. Disparities in acceptance of deceased donor kidneys between the United States and france and estimated effects of increased us acceptance. JAMA Intern Med. (2019) 179:1365–74. doi: 10.1001/jamainternmed.2019.2322
5. Cecka JM, Cohen B, Rosendale J, Smith M. Could more effective use of kidneys recovered from older deceased donors result in more kidney transplants for older patients? Transplantation. (2006) 81:966–70. doi: 10.1097/01.tp.0000216284.81604.d4
6. Gaber LW, Moore LW, Alloway RR, Amiri MH, Vera SR, Gaber AO. Glomerulosclerosis as a determinant of posttransplant function of older donor renal allografts. Transplantation. (1995) 60:334–9. doi: 10.1097/00007890-199508270-00006
7. Wang CJ, Wetmore JB, Crary GS, Kasiske BL. The donor kidney biopsy and its implications in predicting graft outcomes: a systematic review. Am J Transplant. (2015) 15:1903–14. doi: 10.1111/ajt.13213
8. Kasiske BL, Stewart DE, Bista BR, Salkowski N, Snyder JJ, Israni AK, et al. The role of procurement biopsies in acceptance decisions for kidneys retrieved for transplant. Clin J Am Soc Nephrol. (2014) 9:562–71. doi: 10.2215/CJN.07610713
9. Naesens, M. Zero-time renal transplant biopsies: a comprehensive review. Transplantation. (2016) 100:1425–39. doi: 10.1097/TP.0000000000001018
10. Carpenter D, Husain SA, Brennan C, Batal I, Hall IE, Santoriello D, et al. Procurement biopsies in the evaluation of deceased donor kidneys. Clin J Am Soc Nephrol. (2018) 13:1876–85. doi: 10.2215/CJN.04150418
11. Azancot MA, Moreso F, Salcedo M, Cantarell C, Perello M, Torres IB, et al. The reproducibility and predictive value on outcome of renal biopsies from expanded criteria donors. Kidney Int. (2014) 85:1161–8. doi: 10.1038/ki.2013.461
12. Liapis H, Gaut JP, Klein C, Bagnasco S, Kraus E, Farris AB.IIIet al. Banff histopathological consensus criteria for preimplantation kidney biopsies. Am J Transplant. (2017) 17:140–50. doi: 10.1111/ajt.13929
13. Lee AP, Abramowicz D. Is the Kidney Donor Risk Index a step forward in the assessment of deceased donor kidney quality? Nephrol Dial Transplant. (2015) 30:1285–90. doi: 10.1093/ndt/gfu304
14. Chertow GM, Milford EL, Mackenzie HS, Brenner BM. Antigen-independent determinants of cadaveric kidney transplant failure. JAMA. (1996) 276:1732–6.
15. Port FK, Bragg-Gresham JL, Metzger RA, Dykstra DM, Gillespie BW, Young EW, et al. Donor characteristics associated with reduced graft survival: an approach to expanding the pool of kidney donors. Transplantation. (2002) 74:1281–6.
16. Gourishankar S, Grebe SO, Mueller TF. Prediction of kidney graft failure using clinical scoring tools. Clin Transplant. (2013) 27:517–22. doi: 10.1111/ctr.12135
17. Rao PS, Schaubel DE, Guidinger MK, Andreoni KA, Wolfe RA, Merion RM, et al. A comprehensive risk quantification score for deceased donor kidneys: the kidney donor risk index. Transplantation. (2009) 88:231–6. doi: 10.1097/TP.0b013e3181ac620b
18. Zens TJ, Danobeitia JS, Leverson G, Chlebeck PJ, Zitur LJ, Redfield RR, et al. The impact of kidney donor profile index on delayed graft function and transplant outcomes: a single-center analysis. Clin Transplant. (2018) 32:e13190. doi: 10.1111/ctr.13190
19. Dare AJ, Pettigrew GJ, Saeb-Parsy, K. Preoperative assessment of the deceased-donor kidney: from macroscopic appearance to molecular biomarkers. Transplantation. (2014) 97:797–807. doi: 10.1097/01.TP.0000441361.34103.53
20. Bae S, Massie AB, Luo X, Anjum S, Desai NM, Segev DL. Changes in Discard Rate After the Introduction of the Kidney Donor Profile Index (KDPI). Am J Transplant. (2016) 16:2202–7. doi: 10.1111/ajt.13769
21. Lehner LJ, Kleinsteuber A, Halleck F, Khadzhynov D, Schrezenmeier E, Duerr M, et al. Assessment of the Kidney Donor Profile Index in a European cohort. Nephrol Dial Transplant. (2018) 33:1465–72. doi: 10.1093/ndt/gfy030
22. Sexton DJ, O’Kelly P, Kennedy C, Denton M, de Freitas DG, Magee C, et al. Assessing the discrimination of the kidney donor risk index/kidney donor profile index scores for allograft failure and estimated glomerular filtration rate in ireland’s national kidney transplant programme. Clin Kidney J. (2019) 12:569–73. doi: 10.1093/ckj/sfy130
23. Maenosono R, Tullius SG. Saving lives by saving kidneys for transplant. JAMA Intern Med. (2019) 179:1374–5. doi: 10.1001/jamainternmed.2019.2609
24. Massie AB, Luo X, Chow EK, Alejo JL, Desai NM, Segev DL. Survival benefit of primary deceased donor transplantation with high-KDPI kidneys. Am J Transplant. (2014) 14:2310–6. doi: 10.1111/ajt.12830
25. Ekser B, Powelson JA, Fridell JA, Goggins WC, Taber TE. Is the kidney donor profile index (KDPI) universal or UNOS-specific? Am J Transplant. (2018) 18:1031–2. doi: 10.1111/ajt.14652
26. Graham JA, Ajaimy M, Carrero J, Jones T, Torabi J, Alani O, et al. Hepatitis C virus positivity should not be included in the kidney donor profile index calculation when transplanting HCV-positive kidneys into noninfected recipients in the era of direct-acting antiviral agents. Prog Transplant. (2019) 29:371–2. doi: 10.1177/1526924819874373
27. Hall IE, Akalin E, Bromberg JS, Doshi MD, Greene T, Harhay MN, et al. Deceased-donor acute kidney injury is not associated with kidney allograft failure. Kidney Int. (2019) 95:199–209. doi: 10.1016/j.kint.2018.08.047
29. Moers C, Pirenne J, Paul A, Ploeg RJ, Machine Preservation, G. Trial Study, Machine perfusion or cold storage in deceased-donor kidney transplantation. N Engl J Med. (2012) 366:770–1.
30. Lo Faro ML, Akhtar MZ, Boffa C, Ploeg R. Should pulsatile preservation be the gold standard in kidney transplantation? Curr Trans Rep. (2015) 2:105–12.
31. De Deken J, Kocabayoglu P, Moers C. Hypothermic machine perfusion in kidney transplantation. Curr Opin Organ Transplant. (2016) 21:294–300.
32. Tingle SJ, Figueiredo RS, Moir JA, Goodfellow M, Talbot D, Wilson CH. Machine perfusion preservation versus static cold storage for deceased donor kidney transplantation. Cochrane Database Syst Rev. (2019) 3:CD011671. doi: 10.1002/14651858.CD011671.pub2
33. Kataria A, Magoon S, Makkar B, Gundroo A. Machine perfusion in kidney transplantation. Curr Opin Organ Transplant. (2019) 24:378–84.
34. Jochmans I, Moers C, Smits JM, Leuvenink HG, Treckmann J, Paul A, et al. The prognostic value of renal resistance during hypothermic machine perfusion of deceased donor kidneys. Am J Transplant. (2011) 11:2214–20. doi: 10.1016/j.juro.2012.01.053
35. de Vries EE, Hoogland ER Winkens.B, Snoeijs MG, van Heurn, LW. Renovascular resistance of machine-perfused DCD kidneys is associated with primary nonfunction. Am J Transplant. (2011) 11:2685–91. doi: 10.1111/j.1600-6143.2011.03755.x
36. Parikh CR, Hall IE, Bhangoo RS, Ficek J, Abt PL, Thiessen-Philbrook H, et al. Associations of perfusate biomarkers and pump parameters with delayed graft function and deceased donor kidney allograft function. Am J Transplant. (2016) 16:1526–39. doi: 10.1111/ajt.13655
37. Moers C, Varnav OC, van Heurn E, Jochmans I, Kirste GR, Rahmel A, et al. The value of machine perfusion perfusate biomarkers for predicting kidney transplant outcome. Transplantation. (2010) 90:966–73. doi: 10.1097/TP.0b013e3181f5c40c
38. Khalid U, Ablorsu E, Szabo L, Jenkins RH, Bowen T, Chavez R, et al. MicroRNA-21 (miR-21) expression in hypothermic machine perfusate may be predictive of early outcomes in kidney transplantation. Clin Transplant. (2016) 30:99–104. doi: 10.1111/ctr.12679
39. Jochmans I, Pirenne J. Graft quality assessment in kidney transplantation: not an exact science yet! Curr Opin Organ Transplant. (2011) 16:174–9. doi: 10.1097/MOT.0b013e3283446b31
40. Hosgood SA, Barlow AD, Hunter JP, Nicholson ML. Ex vivo normothermic perfusion for quality assessment of marginal donor kidney transplants. Br J Surg. (2015) 102:1433–40. doi: 10.1002/bjs.9894
41. Hosgood SA, Nicholson ML. An Assessment of Urinary Biomarkers in a Series of Declined Human Kidneys Measured During Ex Vivo Normothermic Kidney Perfusion. Transplantation. (2017) 101:2120–5. doi: 10.1097/TP.0000000000001504
42. Koyawala N, Reese PP, Hall IE, Jia Y, Thiessen-Philbrook HR, Mansour SG, et al. Urine injury biomarkers are not associated with kidney transplant failure. Transplantation. (2019). [Epub ahead of print]
43. Weissenbacher A, Vrakas G, Nasralla D, Ceresa CDL. The future of organ perfusion and re-conditioning. Transpl Int. (2019) 32:586–97.
44. Mueller TF, Solez K, Mas V. Assessment of kidney organ quality and prediction of outcome at time of transplantation. Semin Immunopathol. (2011) 33:185–99. doi: 10.1007/s00281-011-0248-x
45. Mueller TF, Reeve J, Jhangri GS, Mengel M, Jacaj Z, Cairo L, et al. The transcriptome of the implant biopsy identifies donor kidneys at increased risk of delayed graft function. Am J Transplant. (2008) 8:78–85. doi: 10.1111/j.1600-6143.2007.02032.x
46. Korbely R, Wilflingseder J, Perco P, Kainz A, Langer RM, Mayer B, et al. Molecular biomarker candidates of acute kidney injury in zero-hour renal transplant needle biopsies. Transpl Int. (2011) 24:143–9. doi: 10.1111/j.1432-2277.2010.01162.x
47. Mengel M, Chang J, Kayser D, Gwinner W, Schwarz A, Einecke G, et al. The molecular phenotype of 6-week protocol biopsies from human renal allografts: reflections of prior injury but not future course. Am J Transplant. (2011) 11:708–18. doi: 10.1111/j.1600-6143.2010.03339.x
48. Mas VR, Scian MJ, Archer KJ, Suh JL, David KG, Ren Q, et al. Pretransplant transcriptome profiles identify among kidneys with delayed graft function those with poorer quality and outcome. Mol Med. (2011) 17:1311–22. doi: 10.2119/molmed.2011.00159
49. Wohlfahrtova M, Brabcova I, Zelezny F, Balaz P, Janousek L, Honsova E, et al. Tubular atrophy and low netrin-1 gene expression are associated with delayed kidney allograft function. Transplantation. (2014) 97:176–83. doi: 10.1097/TP.0b013e3182a95d04
50. Gingell-Littlejohn M, McGuinness D, McGlynn LM, Kingsmore D, Stevenson KS, Koppelstaetter C, et al. Pre-transplant CDKN2A expression in kidney biopsies predicts renal function and is a future component of donor scoring criteria. PLoS One. (2013) 8:e68133. doi: 10.1371/journal.pone.0068133
51. McGuinness D, Leierer J, Shapter O, Mohammed S, Gingell-Littlejohn M, Kingsmore DB, et al. Identification of molecular markers of delayed graft function based on the regulation of biological ageing. PLoS One. (2016) 11:e0146378. doi: 10.1371/journal.pone.0151506
52. McGuinness D, Mohammed S, Monaghan L, Wilson PA, Kingsmore DB, Shapter O, et al. A molecular signature for delayed graft function. Aging Cell. (2018) 17:e12825. doi: 10.1111/acel.12825
53. Scian MJ, Maluf DG, Archer KJ, Turner SD, Suh JL, David KG, et al. Identification of biomarkers to assess organ quality and predict posttransplantation outcomes. Transplantation. (2012) 94:851–8. doi: 10.1097/TP.0b013e318263702b
54. Famulski KS, de Freitas DG, Kreepala C, Chang J, Sellares J, Sis B, et al. Molecular phenotypes of acute kidney injury in kidney transplants. J Am Soc Nephrol. (2012) 23:948–58. doi: 10.1681/ASN.2011090887
55. Kreepala C, Famulski KS, Chang J, Halloran PF. Comparing molecular assessment of implantation biopsies with histologic and demographic risk assessment. Am J Transplant. (2013) 13:415–26. doi: 10.1111/ajt.12043
56. Guillen-Gomez E, Dasilva I, Silva I, Arce Y, Facundo C, Ars E, et al. Early macrophage infiltration and sustained inflammation in kidneys from deceased donors are associated with long-term renal function. Am J Transplant. (2017) 17:733–43. doi: 10.1111/ajt.13998
57. Kaisar M, van Dullemen L, Charles P, Akhtar ZM, Thezenas ML, Huang H, et al. Subclinical changes in deceased donor kidney proteomes are associated with 12-month allograft function posttransplantation-a preliminary study. Transplantation. (2019) 103:323–8. doi: 10.1097/TP.0000000000002358
58. Kollmann D, Selzner M. Recent advances in the field of warm ex-vivo liver perfusion. Curr Opin Organ Transplant. (2017) 22:555–62. doi: 10.1097/MOT.0000000000000471
59. Cortes M, Pareja E, Garcia-Canaveras JC, Donato MT, Montero S, Mir J, et al. Metabolomics discloses donor liver biomarkers associated with early allograft dysfunction. J Hepatol. (2014) 61:564–74. doi: 10.1016/j.jhep.2014.04.023
60. Verhoeven CJ, Farid WR, de Ruiter PE, Hansen BE, Roest HP, de Jonge J, et al. MicroRNA profiles in graft preservation solution are predictive of ischemic-type biliary lesions after liver transplantation. J Hepatol. (2013) 59:1231–8. doi: 10.1016/j.jhep.2017.09.001
61. Matton APM, Selten JW, Roest HP, de Jonge J, Jnm IJ, de Meijer VE, et al. Cell-free microRNAs as early predictors of graft viability during ex vivo normothermic machine perfusion of human donor livers. Clin Transplant. (2020) 34:e13790. doi: 10.1111/ctr.13790
62. Selten JW, Verhoeven CJ, Heedfeld V, Roest HP, de Jonge J, Pirenne J, et al. The release of microRNA-122 during liver preservation is associated with early allograft dysfunction and graft survival after transplantation. Liver Transpl. (2017) 23:946–56. doi: 10.1002/lt.24766
63. Hamaoui K, Papalois V. Machine perfusion and the pancreas: will it increase the donor pool? Curr Diab Rep. (2019) 19:56. doi: 10.1007/s11892-019-1165-y
64. Ali A, Cypel M. Ex-vivo lung perfusion and ventilation: where to from here? Curr Opin Organ Transplant. (2019) 24:297–304. doi: 10.1097/MOT.0000000000000647
65. Andreasson ASI, Borthwick LA, Gillespie C, Jiwa K, Scott J, Henderson P, et al. The role of interleukin-1beta as a predictive biomarker and potential therapeutic target during clinical ex vivo lung perfusion. J Heart Lung Transplant. (2017) 36:985–95. doi: 10.1016/j.healun.2017.05.012
66. Machuca TN, Cypel M, Yeung JC, Bonato R, Zamel R, Chen M, et al. Protein expression profiling predicts graft performance in clinical ex vivo lung perfusion. Ann Surg. (2015) 261:591–7. doi: 10.1097/SLA.0000000000000974
67. Machuca TN, Cypel M, Zhao Y, Grasemann H, Tavasoli F, Yeung JC, et al. The role of the endothelin-1 pathway as a biomarker for donor lung assessment in clinical ex vivo lung perfusion. J Heart Lung Transplant. (2015) 34:849–57. doi: 10.1016/j.healun.2015.01.003
68. Hashimoto K, Cypel M, Kim H, Machuca TN, Nakajima D, Chen M, et al. Soluble adhesion molecules during ex vivo lung perfusion are associated with posttransplant primary graft dysfunction. Am J Transplant. (2017) 17:1396–404. doi: 10.1111/ajt.14160
69. Caldarone L, Mariscal A, Sage A, Khan M, Juvet S, Martinu T, et al. Neutrophil extracellular traps in ex vivo lung perfusion perfusate predict the clinical outcome of lung transplant recipients. Eur Respir J. (2019) 53:1801736. doi: 10.1183/13993003.01736-2018
70. Sunjaya AF, Sunjaya AP. Combating donor organ shortage: organ care system prolonging organ storage time and improving the outcome of heart transplantations. Cardiovasc Ther. (2019) 2019:9482797. doi: 10.1155/2019/9482797
71. Mallon DH, Summers DM, Bradley JA, Pettigrew GJ. Defining delayed graft function after renal transplantation: simplest is best. Transplantation. (2013) 96:885–9. doi: 10.1097/TP.0b013e3182a19348
72. Kayler LK, Srinivas TR, Schold JD. Influence of CIT-induced DGF on kidney transplant outcomes. Am J Transplant. (2011) 11:2657–64. doi: 10.1016/j.juro.2012.02.2513
73. Summers DM, Johnson RJ, Allen J, Fuggle SV, Collett D, Watson CJ, et al. Analysis of factors that affect outcome after transplantation of kidneys donated after cardiac death in the UK: a cohort study. Lancet. (2010) 376:1303–11. doi: 10.1016/S0140-6736(10)60827-6
74. Weber M, Dindo D, Demartines N, Ambuhl PM, Clavien PA. Kidney transplantation from donors without a heartbeat. N Engl J Med. (2002) 347:248–55.
75. Lim MA, Bloom RD. Medical therapies to reduce delayed graft function and improve long-term graft survival: are we making progress? Clin J Am Soc Nephrol. (2020) 15:13–5. doi: 10.2215/CJN.13961119
76. Lee J, Song SH, Lee JY, Kim DG, Lee JG, Kim BS, et al. The recovery status from delayed graft function can predict long-term outcome after deceased donor kidney transplantation. Sci Rep. (2017) 7:13725. doi: 10.1038/s41598-017-14154-w
77. Lim WH, Johnson DW, Teixeira-Pinto A, Wong G. Association between duration of delayed graft function, acute rejection, allograft outcome after deceased donor kidney transplantation. Transplantation. (2019) 103:412–9. doi: 10.1097/TP.0000000000002275
78. Chawla LS, Bellomo R, Bihorac A, Goldstein SL, Siew ED, Bagshaw SM, et al. Quality initiative, acute kidney disease and renal recovery: consensus report of the acute disease quality initiative (ADQI) 16 workgroup. Nat Rev Nephrol. (2017) 13:241–57. doi: 10.1038/nrneph.2017.2
79. Terasaki PI, Gjertson DW, Cecka JM, Takemoto S, Cho YW. Significance of the donor age effect on kidney transplants. Clin Transplant. (1997) 11:366–72.
Keywords: marginal organs, molecular diagnostics, implant biopsies, organ quality, surrogate marker
Citation: von Moos S, Akalin E, Mas V and Mueller TF (2020) Assessment of Organ Quality in Kidney Transplantation by Molecular Analysis and Why It May Not Have Been Achieved, Yet. Front. Immunol. 11:833. doi: 10.3389/fimmu.2020.00833
Received: 17 January 2020; Accepted: 14 April 2020;
Published: 12 May 2020.
Edited by:
Oriol Bestard, Hospital Universitario de Bellvitge, SpainReviewed by:
Maarten Naesens, KU Leuven, BelgiumCopyright © 2020 von Moos Akalin, Mas and Mueller. This is an open-access article distributed under the terms of the Creative Commons Attribution License (CC BY). The use, distribution or reproduction in other forums is permitted, provided the original author(s) and the copyright owner(s) are credited and that the original publication in this journal is cited, in accordance with accepted academic practice. No use, distribution or reproduction is permitted which does not comply with these terms.
*Correspondence: Thomas F. Mueller, dGhvbWFzLm11ZWxsZXJAdXN6LmNo
Disclaimer: All claims expressed in this article are solely those of the authors and do not necessarily represent those of their affiliated organizations, or those of the publisher, the editors and the reviewers. Any product that may be evaluated in this article or claim that may be made by its manufacturer is not guaranteed or endorsed by the publisher.
Research integrity at Frontiers
Learn more about the work of our research integrity team to safeguard the quality of each article we publish.