- 1Department of Pediatric Hematology and Oncology and of Cell and Gene Therapy, Scientific Institute for Research and Healthcare (IRCCS), Bambino Gesù Childrens' Hospital, Rome, Italy
- 2Transfusion Unit, Department of Laboratories, Scientific Institute for Research and Healthcare (IRCCS), Bambino Gesù Childrens' Hospital, Rome, Italy
- 3Department of Radiology and Radiotherapy, Sapienza University, Rome, Italy
- 4Stem Cell Laboratory and Cell Therapy Center, Giannina Gaslini Institute (IRCCS), Genoa, Italy
- 5Sapienza, University of Rome, Rome, Italy
TcRαβ/CD19-cell depleted HLA-haploidentical hematopoietic stem cell transplantation (haplo-HSCT) represents a promising new platform for children affected by acute leukemia in need of an allograft and lacking a matched donor, disease recurrence being the main cause of treatment failure. The use of zoledronic acid to enhance TcRγδ+ lymphocyte function after TcRαβ/CD19-cell depleted haplo-HSCT was tested in an open-label, feasibility, proof-of-principle study. Forty-six children affected by high-risk acute leukemia underwent haplo-HSCT after removal of TcRαβ+ and CD19+ B lymphocytes. No post-transplant pharmacological graft-versus-host disease (GvHD) prophylaxis was given. Zoledronic acid was administered monthly at a dose of 0.05 mg/kg/dose (maximum dose 4 mg), starting from day +20 after transplantation. A total of 139 infusions were administered, with a mean of 3 infusions per patient. No severe adverse event was observed. Common side effects were represented by asymptomatic hypocalcemia and acute phase reactions (including fever, chills, malaise, and/or arthralgia) within 24–48 h from zoledronic acid infusion. The cumulative incidence of acute and chronic GvHD was 17.3% (all grade I-II) and 4.8% (all limited), respectively. Patients given 3 or more infusions of zoledronic acid had a lower incidence of both acute GvHD (8.8 vs. 41.6%, p = 0.015) and chronic GvHD (0 vs. 22.2%, p = 0.006). Transplant-related mortality (TRM) and relapse incidence at 3 years were 4.3 and 30.4%, respectively. Patients receiving repeated infusions of zoledronic acid had a lower TRM as compared to those receiving 1 or 2 administration of the drug (0 vs. 16.7%, p = 0.01). Five-year overall survival (OS) and disease-free survival (DFS) for the whole cohort were 67.2 and 65.2%, respectively, with a trend toward a better OS for patients receiving 3 or more infusions (73.1 vs. 50.0%, p = 0.05). The probability of GvHD/relapse-free survival was significantly worse in patients receiving 1–2 infusions of zoledonic acid than in those given ≥3 infusions (33.3 vs. 70.6%, respectively, p = 0.006). Multivariable analysis showed an independent positive effect on outcome given by repeated infusions of zoledronic acid (HR 0.27, p = 0.03). These data indicate that the use of zoledronic acid after TcRαβ/CD19-cell depleted haploHSCT is safe and may result in a lower incidence of acute GvHD, chronic GvHD, and TRM.
Introduction
Hematopoietic stem cell transplantation (HSCT) is, so far, the best curative option for a number of malignant disorders (1, 2). However, up to 30% of patients lack both a HLA-identical sibling or an alternative donor [i.e., HLA-Matched Unrelated Donor (MUD) or Unrelated Donor Umbilical Cord Blood (UD-UCB)] (3). Thus, HSCT from an HLA-haploidentical relative (haplo-HSCT) represents a very promising option, since it owns particular features, including applicability for virtually all patients, choice of best donor from a panel of potential candidates, immediate accessibility to the transplant procedure, and easy access to donors in case adoptive cell therapies as are required after transplantation (3). However, compared to other types of allograft, haplo-HSCT has been hampered by a delayed immune reconstitution (4, 5), which, in turn, influences the risk of both post-transplant infections and, most importantly, relapse incidence (6, 7). Since it has been demonstrated that specific innate immunity cell subpopulations [e.g., NK (8) and TcRγδ T cells (9)] may influence transplant outcome, we and other groups recently developed a new method of graft manipulation (i.e., TcRαβ/CD19 negative selective depletion) (10–13), which allows to retain large numbers of mature, ready-to-kill effector cells, namely NK and TcRγδ lymphocytes, in the final product. TcRγδ cells are a non-alloreactive, “innate-like,” T lymphocyte subpopulation (normally accounting for 1–10% of circulating T lymphocytes) capable of recognizing targets in an MHC-independent manner (through several activating receptors, like γδ-TcR, NKG2D, and TLRs) and displaying different functions, including anti-infective and anti-tumor activity (14, 15). We have recently reported the largest pediatric cohort receiving this kind of transplant, clearly showing that this approach represents a suitable alternative for children affected by acute leukemia, with outcomes comparable to those of HLA-matched donor HSCT recipients (13).
Preclinical data showed that bisphosphonates, such as pamidronate and zoledronate, commonly used to treat bone diseases and hypercalcemia in multiple myeloma, can mediate the improvement of TcRγδ-mediated tumor cells killing capacity (16, 17). Such an effect is obtained thanks to the activation of a particular subset of TcRγδ cells, through the accumulation of phosphoantigens (18) and, to a lesser extent, the sensitization of tumor target cells (19).
In an attempt to reduce the relapse incidence and the risk of severe infections in high risk-patients given TcRαβ/CD19-cell depleted haploHSCT, we explored the administration of zoledronic acid in the post-transplant period (20). We previously reported detailed cytofluorimetric, functional, and proteomic analysis of TcRγδ cells of patients treated with this strategy, showing that zoledronate administration promotes γδ T-cell differentiation and cytotoxicity (20). Here we report the long-term outcomes of 46 patients treated with zoledronic acid after TcRαβ/CD19-depleted haploHSCT.
Patients and Methods
Patients
Patients aged 0.3 to 21 years, affected by acute leukemia, who were in need of an allograft while lacking an HLA-matched related or unrelated donor between January 1st, 2013 and September 26th, 2016 at IRCCS Ospedale Pediatrico Bambino Gesù in Rome, were considered eligible. All patients or legal guardians provided written informed consent, and the research was conducted under a Hospital Ethical Committee- approved protocol, in accordance with the Declaration of Helsinki.
Transplantation Procedure
All the 46 enrolled patients received a fully myeloablative conditioning regimen, which was based on the use of total body irradiation (TBI) in 37 (80%) children and chemo-based in 9 patients (20%) (details are reported in the Supplementary Material). Pre-transplantation rabbit anti-T lymphocyte globulin (ATLG, Grafalon®, Neovii) was administered, at a dose of 4 mg/kg/day for 3 consecutive days (days−5 to−3), in order to prevent both graft failure and graft-versus-host disease (GvHD), through in vivo T-cell depletion and/or modulation of bidirectional alloreactivity. On day−1, children were also given rituximab 200 mg/m2 for in vivo donor and recipient B-cell depletion to reduce the risk of Epstein-Barr virus (EBV)-related post-transplantation lymphoproliferative disorders (PTLD). No patient was given any post-transplant pharmacologic GvHD prophylaxis.
The donor was chosen according to immune-genetic criteria, giving priority to natural killer (NK) alloreactivity (evaluated according to the killer immunoglobulin-like receptor (KIR)-KIR ligand model), NK cell B haplotype, and higher B content, as previously described (10, 21). The donor was a parent for all patients but one, who was transplanted from her HLA-haploidentical brother. Granulocyte colony-stimulating factor (G-CSF) at a dose of 10–12 μg/kg/day was administered by subcutaneous injection to all donors to mobilize in peripheral blood hematopoietic stem cells from day−5 until leukapheresis (day−1). Ten donors (21.7%) with circulating CD34+ cell count <0.04 x 109/L on day−2 also received a single-dose of plerixafor (240 μg/kg) 6–9 h before cell collection. Graft manipulation was performed using the CliniMACS device as previously described (22).
Zoledronic Acid Administration
Zoledronic acid was administered monthly at a dose of 0.05 mg/kg/dose (maximal single dose 4 mg) over 1 h, starting after: i) achievement of stable donor engraftment, and ii) at least day +20 from transplantation. The dose was based on literature data about zoledronate use in pediatric bone diseases (23). Since this was an open-label, feasibility, proof-of-principle study, the number of scheduled doses was not fixed; patients continued to receive monthly infusions of up to 5 consecutive doses, unless an event (i.e., side effects related to the drug, disease relapse, severe infections, hospitalization for any cause, patient/parents refusal) occurred. We opted to administer multiple infusions of zoledronic acid, based on current literature data indicating that in vivo activation of TcRγδ T-cells in response to the drug is a transient phenomenon (24). Oral calcitriol, together with calcium supplementation, was administered for 7–10 days after zoledronate infusion, in order to prevent/treat hypocalcemia. Zoledronic acid was administered either in the inpatient or in the outpatient unit.
Statistical Analysis
Quantitative variables were reported as median value and range; categorical variables were expressed as absolute value and percentage. Clinical characteristics of patients were compared using the Chi-square test or Fisher's exact test for categorical variables, while the Mann-Whitney rank sum test or the Student's T-test were used for continuous variables as appropriate (25). The time to neutrophil engraftment was defined as time from HSCT to the first of 3 consecutive days with an absolute neutrophil count equal to or greater than 0.5 × 109 per liter, and the time to platelet engraftment as time from HSCT to the first of 7 consecutive days with an unsupported platelet count equal to or greater than 20 × 109 per liter.
Patients surviving for more than 7 and 100 days after transplantation were considered evaluable for acute and chronic GvHD (aGvHD, cGvHD) occurrence, respectively. Severity of aGvHD and cGvHD was assessed according to Glucksberg and Shulman criteria (26, 27).
Overall survival (OS), disease-free survival (DFS), GvHD/relapse-free survival (GRFS), transplant-related mortality (TRM), aGvHD, cGvHD, and relapse incidence (RI) were estimated from the date of transplantation to the date of an event or last follow-up. Probabilities of OS, DFS, and GRFS were calculated according to the Kaplan and Meier method (28). TRM, aGvHD, cGvHD, and RI were calculated as cumulative incidence curves in order to adjust the estimates for the appropriate competing risks (29). All results were expressed as probability or cumulative incidence (%) and 95% confidence interval (95% CI) (30).
The significance of differences between OS, DFS, and GRFS was estimated by the log–rank test (Mantel–Cox), while Gray's test was used to assess, in univariate analyses, differences between cumulative incidences (31). Multivariate analysis was performed using the Cox proportional hazard regression model (30). P-values < 0.05 were considered to be statistically significant.
Statistical analysis was performed using EZR version 1.32 (Saitama Medical Centre, Jichi Medical University), which is a graphical user interface for R (The R Foundation for Statistical Computing, Vienna, Austria) (32).
Results
Patients
Forty-six patients affected by acute leukemia received a TcRαβ/CD19-cell depleted peripheral blood stem cell allograft from an HLA-haploidentical relative in the study period at IRCCS Ospedale Pediatrico Bambino Gesù, Rome. Table 1 reports patient demographic and disease characteristics, and transplant details. Twenty-six patients had B-cell precursor acute lymphoblastic leukemia (BCP-ALL), 7 T-cell lineage ALL (T-ALL), and 11 acute myeloid leukemia (AML), while 2 patients were affected by mixed phenotype acute leukemia (MPAL). Seven patients had previously received a first HSCT. Two patients were not in morphological complete remission at time of transplantation.
Graft Composition and Hematopoietic Recovery
All children received <1 × 105 TcRαβ cells per kg of recipient body weight (median 0.04 × 106/kg; range 0.001–0.099), with a median Log depletion of 3.93 (range 3.42–5.42), while the median number of TcRγδ cells infused was 7.7 × 106 per kg (range 0.8–42.7) (details of graft composition are reported in Table 1). The median number of infused CD34+ cells per kg was 15.4 × 106 (range 6.5–40.6) (median CD34+ stem cell recovery 91.25%; range 71.67–100), while that of B cells was 0.03 × 106/kg (range 0.001–0.18) (median Log depletion of 3.42; range 2.93–4.50).
All patients achieved engraftment; the median time to neutrophil engraftment was 14.5 days (range 8–21), whereas the median time to platelet recovery was 11 days (range 8–27). Monitoring of donor-recipient chimerism performed to hypervariable regions of human DNA confirmed the engraftment of donor hematopoiesis in all patients.
Zoledronic Acid Infusions and Adverse Reactions
The 46 patients received a total of 139 zoledronic acid infusions, with a mean of 3 infusions per patient (range 1–6). Specifically, 4 patients received a single infusion, 8 patients received 2 infusions, 20 were given 3 infusions, 12 received 4 infusions, while one patient each received 5 and 6 infusions (because of physician decision, based on good tolerability and positive effect on bone metabolism parameters). Treatment started at a median of 39 days (range 20–79). Ten patients (21.7%) experienced an acute phase reaction, including fever, chills, malaise, arthralgia, and/or transient skin rash, within 24–48 h after the first administration. Five patients (10.8%) had a transient decrease in the WBC or platelet count. All patients experienced a reduction of calcium serum levels; however, only one episode of symptomatic hypocalcemia (at first administration) occurred and was rapidly corrected with parenteral calcium supplementation. One patient (2%) presented asymptomatic hypokaliemia. No other adverse event was recorded; in particular, we did not observe any case of osteonecrosis.
TcRγδ T-Cells After Zoledronic Acid Infusion
As already reported (13, 16), TcRγδ T-cells represented the main T cell population in the first weeks after TcRαβ/CD19-depleted haploidentical HSCT. This predominance of TcRγδ T-cells was observed also in zoledronate-treated patients. Subsequently, TcRαβ T-lymphocytes progressively increased over time, exceeding the percentage of TcRγδ T-cells before the third month after transplantation (data not shown). Interestingly, as already shown (20), in patients given zoledronic acid a progressive decrease in the percentage and absolute number of TcRγδ T-cells was observed, thus suggesting that repeated infusions of zoledronic acid do not induce persistence of this cell subset (Figure 1A). No significant difference in the number of circulating TcRγδ T-cells between patients receiving ≥3 infusions of zoledronic acid as compared to those given 1 or 2 infusion was found, although there was a trend toward a higher reduction at 3 months after HSCT in the first group (Figure 1B).
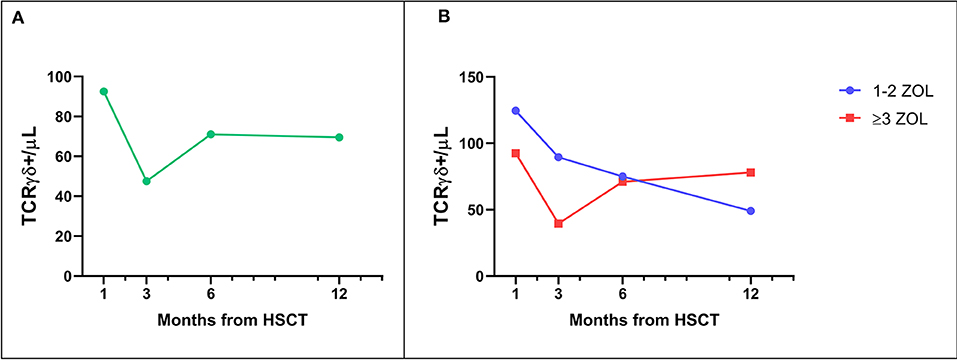
Figure 1. (A) Median number of TcRγδ T-cells in the peripheral blood of patients receiving zoledronic acid after TcRαβ/CD19-depleted haploHSCT. (B) Median number of TcRγδ T-cells in the peripheral blood of patients receiving either 1–2 or 3–6 infusions of zoledronic acid (ZOL).
Graft-versus-Host Disease
Skin-only aGvHD was observed in 8 children after zoledronic acid infusion, with 3 patients experiencing overall grade I and 5 patient developing grade II aGvHD, the cumulative incidence of aGvHD being 17.3% (95% confidence interval (CI) 5.6–27.6) (Figure 2A). Grade I aGvHD was treated with sole topical steroid application, while patients with grade II aGvHD received systemic corticosteroids with or without extracorporeal photopheresis (ECP), with complete resolution in all cases. Two out of the 42 evaluable patients developed limited cGvHD, with a cumulative incidence of 4.8% (95% CI 0–11.2) (Figure 3A).
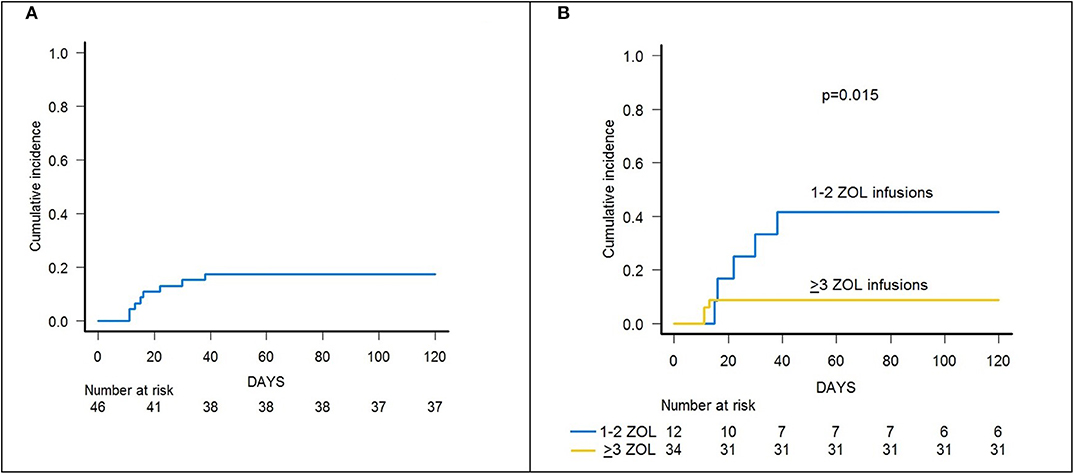
Figure 2. (A) Cumulative incidence of acute GvHD (all grades) of the whole cohort of 46 patients. (B) Cumulative incidence of acute GvHD (all grades) in patients receiving either 1–2 or 3–6 infusions of zoledronic acid (ZOL).
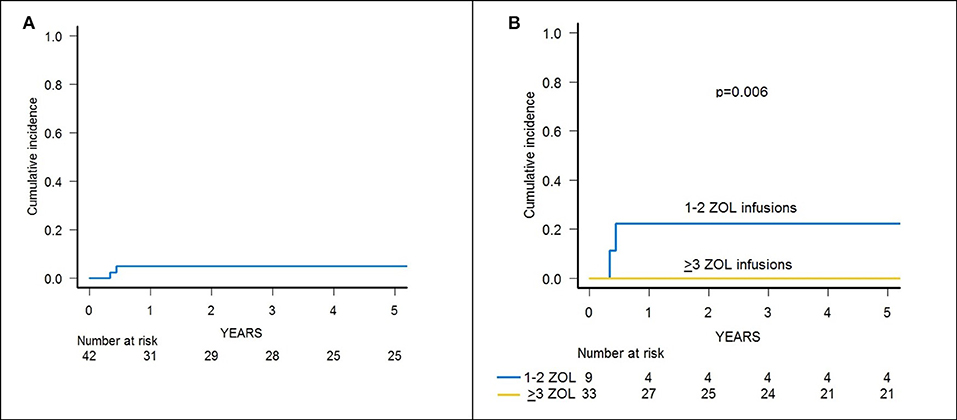
Figure 3. (A) Cumulative incidence of chronic GvHD of the whole cohort of 46 patients. (B) Cumulative incidence of chronic GvHD in patients receiving either 1–2 or 3–6 infusions of zoledronic acid (ZOL).
Notably, patients given 3 or more infusions of zoledronate had a lower incidence of both acute and chronic GvHD. In detail, patients receiving either ≥3 doses or 1–2 doses of zoledronic acid had a cumulative incidence of aGvHD of 8.8% (95% CI 0–17.9) and 41.6% (95% CI 5.9–63.8), respectively (p = 0.015) (Figure 2B).
Since the 2 patients experiencing cGvHD received <3 infusions of zoledronic acid, the cumulative incidence of cGvHD in patients receiving 3 or more infusions of zoledronate compared to those who received 1 or 2 infusions was 0 and 22.2% (95% CI 0–45.1), respectively (p = 0.006) (Figure 3B).
Infections
In terms of early virus reactivation, cytomegalovirus (CMV) DNAemia was recorded in 15 patients, with a cumulative incidence of 30.4% (95% CI 15.7–42.5). However, none progressed to CMV disease thanks to the administration of pre-emptive treatment with ganciclovir. Regarding EBV, no EBV-associated PTLD occurred in our cohort. Nine patients experienced Adenovirus infection (cumulative incidence 19.5%; 95% CI 7.2–30.2); in one case the infection was not controlled by pharmacological therapy, leading to a disseminated infection that ultimately resulted in the patient's death. Three patients developed bacterial infection (2 Gram-positive and 1 Gram-negative sepsis) (cumulative incidence 6.5%; 95% CI 0–13.4), controlled by large-spectrum antibiotic therapy. Finally, one patient suffered from a fatal Aspergillus flavus infection. The number of zoledronic acid infusion did not affect the incidence of viral, bacterial, or fungal infection. However, there was a trend toward a lower incidence of CMV infection in patients given 3–6 infusions (26.4 vs. 41.6% for patient receiving less administrations), but the difference was not statistically significant.
Relapse and Transplant-Related Mortality
Fourteen patients relapsed at a median time of 194 days after HSCT (range 81–1,081), the cumulative incidence of relapse being 30.4% (95% CI 17.8–44.1). It did not differ between patients receiving 1–2 infusions of zoledronic acid and patients receiving 3–6 administrations (33.3 vs. 29.4%, p = n.s.), although there was a trend toward reduction of relapse for patients receiving 4–6 zoledronate doses as compared to those receiving 1–3 infusions (37.5 vs. 14.3%, p = 0.11) (Supplementary Figure 2). Time to first zoledronic acid infusion was comparable between relapsed and disease-free patients (median 39 vs. 42.5 days, p = n.s.). Moreover, time to relapse was comparable between relapsed patients receiving 1–2 zoledronic acid infusions and those receiving 3–6 administrations. Finally, the proportion of children receiving 3–6 infusions was comparable between the subgroup of patients in first complete remission (CR) and those in second CR/other disease status (p = n.s.). As already reported (13), the use of TBI in the conditioning regimen was associated with a reduced incidence of relapse (21.6 vs. 66.7%, p = 0.01).
Since two patients died due to infections, the 3-year cumulative incidence of TRM was 4.3% (95% CI 0.8–13.2) (Supplementary Figure 1). A significantly lower TRM was observed for patients who received 3–6 infusions of zoledronic acid in comparison with those given 2 or less administrations (0 vs. 16.7%, p = 0.01) (Figure 4).
Survival Outcomes
With a median follow-up of 70.4 months (range 39.8–84.0), 31 patients are alive, and the 5-year probability of OS was 67.2% (95% CI 51.5–78.7) (Figure 5A). Since 14 patients relapsed, the 5-year probability of DFS was 65.2 (95% CI 49.6–77.0) (Supplementary Figure 3A). Five-year probability of GRFS was 60.9 (95% CI 45.3–73.3) (Figure 6A). As previously demonstrated in this setting (13), the use of a TBI-based conditioning resulted in an improved outcome. Indeed, DFS was 73.0% (95% CI 55.6–84.4) and 33.3% (95% CI 7.8–62.3) in patients who either did or did not receive TBI, respectively (p = 0.02; Figure 7); OS was 75.7% (95% CI 58.5–86.5) and 44.0% (95% CI 13.6–71.9) for these 2 groups (p = 0.10).
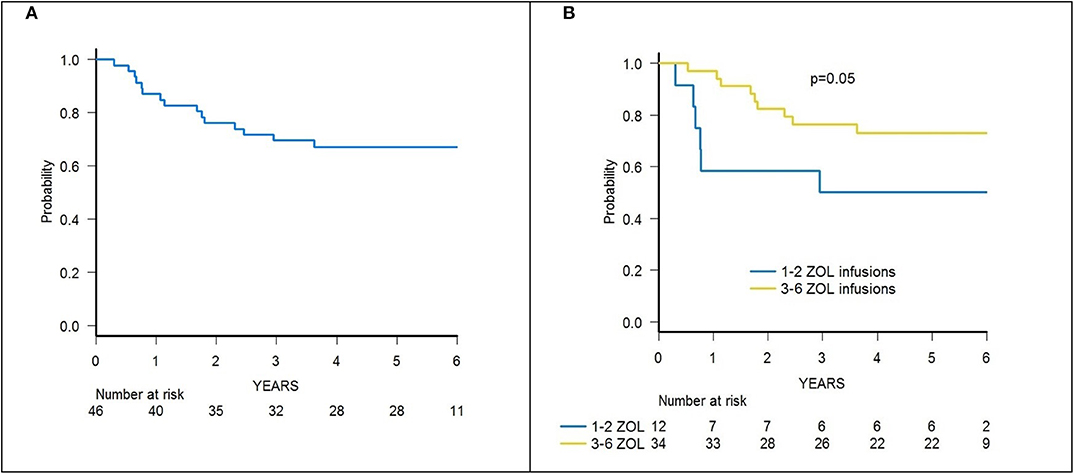
Figure 5. (A) OS of the whole cohort of 46 patients. (B) OS of patients receiving either 1–2 or 3–6 infusions of zoledronic acid (ZOL).
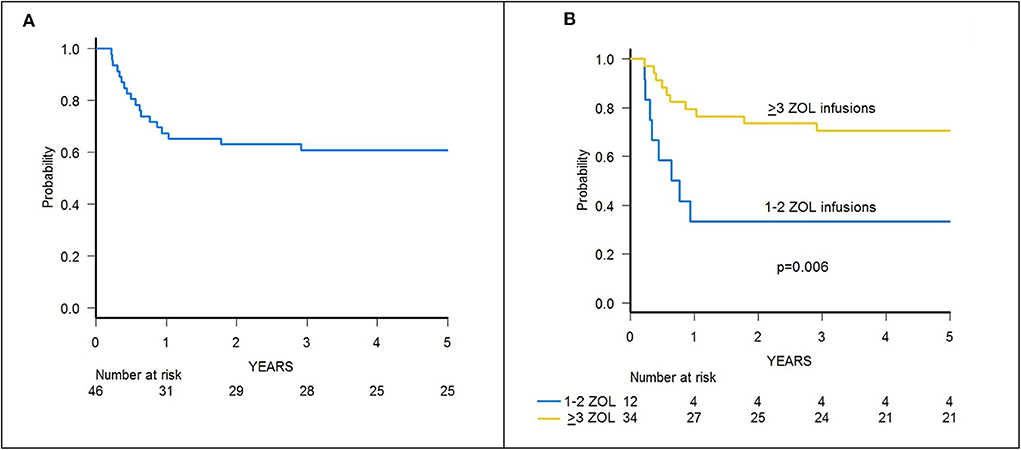
Figure 6. (A) GRFS of the whole cohort of 46 patients. (B) GRFS of patients receiving either 1–2 or ≥3 infusions of zoledronic acid (ZOL).
A trend toward higher survival estimates was observed in patients given 3 or more infusions as compared to those receiving 1–2 infusions, suggesting a possible influence of repeated administrations of zoledronic acid on outcome, although the difference was not statistically significant. In detail, OS was 73.1% (95% CI 54.7–85.1) for patients who received more than 2 infusions vs. 50% (95% CI 20.8–73.6) for those who received 1–2 infusions (p = 0.05) (Figure 5B), while DFS was 70.6% (95% CI 55.2–83.0) vs. 50.0% (95% CI 20.8–73.6) for patients given 3 or more infusions and those receiving 2 or less infusions, respectively (p = n.s.) (Supplementary Figure 3B). Notably, when stratified according to the conditioning regimen employed (TBI-based vs. chemo-based), the effect of repeated infusions of zoledronic acid was more evident, with the best OS obtained by patients who received both TBI and more than 2 zoledronic acid infusions (80.8%, 95% CI 59.8–91.5) (Figure 8). Moreover, in multivariable analysis model including these 2 variables, together with age at transplant and type of disease (ALL vs. AML), the repeated infusions of zoledronic acid showed an independent positive effect on OS (Table 2). GRFS differed significantly between patients receiving 1–2 infusion of zoledonic acid (33.3%, 95% CI 10.3–58.8) or ≥3 infusions (70.6%, 95% CI 52.2–83.0) (p = 0.006) (Figure 6B).
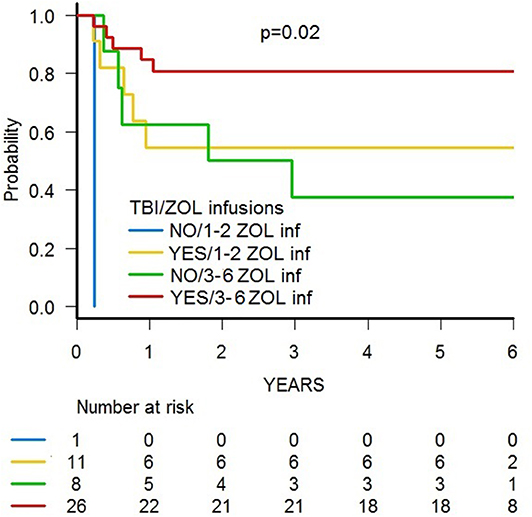
Figure 8. OS of patients stratified according to (i) the conditioning regimen used and (ii) the number of zoledronic acid (ZOL) infusion received after HSCT.
Outcome was not influenced by the number of TcRγδ cells infused with the graft (data not shown), nor by other factors, including age at transplant, type of leukemia (myeloid, T or B lineage, or MPAL), disease status at HSCT, NK alloreactivity, B-content score, or graft composition (data not shown).
Discussion
Despite major improvements in T-cell depleted haploHSCT, disease relapse remains the main cause of treatment failure in acute leukemias (13); thus, strategies aimed at reducing leukemia recurrence are needed. TcRγδ T-lymphocytes are a subpopulation of T cells with features between innate and adaptive immunity endowed with notable properties (lysis of infected or stressed cells and cytokine and chemokine production among the others) through which they give unique contributions to immune reactions (33). Several studies have demonstrated the cytotoxic activity of TcRγδ T-cells against hematologic malignancies both in vitro (34) and in vivo (35), through direct recognition (i.e., in a MHC-independent way) of targets present on leukemia cells (36). Thus, not surprisingly, a faster immune reconstitution of this subset has been associated with an improved DFS after allogeneic HSCT in patients with either ALL or AML (9, 37, 38). In particular, seminal works by Lamb and colleagues in T-cell depleted HSCT from partially mismatched related donors demonstrated that accelerated TcRγδ T-cell recovery was associated with improved DFS (9, 37), mainly due to a decreased relapse incidence (39).
Kunzmann and colleagues first reported the activation and expansion of TcRγδ T-cells following the administration of aminobisphosphonates (17). The authors provided in vitro evidence that the proliferative response of TcRγδ T-cells to bisphosphonates was IL-2 dependent, whereas their activation occurred in the absence of cytokines (40). It has been shown that nitrogen-containing bisphosphonates, such as zoledronic acid, cause the inhibition of farnesyl diphosphate synthase in tumor cell lines, which leads to the accumulation of isopentenyl pyrophosphate (IPP), a type of non-peptidic-phosphorylated metabolite (nPAgs), which indirectly activates γδ T cell (41, 42). What still remains unclear is the precise mechanism by which TcRγδ T-cells are activated by nPAgs, although the γδ-TcR appears to undergo a conformational change or clustering of the butyrophilin family member 3A1 (BTN3A1) molecule (18). Although zoledronic acid increases the cytotoxic capacity of both principal subsets of TcRγδ T-cells (i.e., Vδ1 and Vδ2 (33)), Vδ2 T cells are more prone to this effect (20).
As detailed in recently published studies (12, 22), TcRαβ/CD19-depletion of G-CSF mobilized PBSC of haploidentical donors is an effective manipulation strategy, able to efficiently remove TcRαβ lymphocytes while retaining in the graft high numbers of effector cells, namely mature NK and TcRγδ T-cells. We characterized, by means of immunophenotypic and functional assays, the immune reconstitution of TcRγδ T-lymphocyte after this type of T-cell depleted haploHSCT (16), demonstrating that: i) TcRγδ T-cells are the predominant T-cell population in the early post-transplantation period, mainly deriving from cells infused with the graft; ii) these cells expand in vivo after transplantation; iii) they display a cytotoxic phenotype and degranulate when challenged with primary acute myeloid and lymphoid leukemia blasts; and iv) Vδ2 cells more efficiently lyse primary lymphoid and myeloid blasts in vitro after their exposure to zoledronic acid. Thus, this transplant platform constitutes the ideal setting to test the immune-modulatory properties of in vivo administration of aminobisphosphonates. Indeed, zoledronic acid has been previously demonstrated to be safe in pediatric age in studies on osteopenia (43) and, more importantly, at escalating doses (up to 4 mg/m2), in patients affected by recurrent/refractory neuroblastoma (44). Moreover, the use of bisphosphonates in HSCT recipients ameliorates osteoporosis, a well-known complication of transplantation (45). Indeed, the Australasian Leukemia and Lymphoma Group showed that post-transplant administration of zoledronate, based on a risk-adapted algorithm, minimizes bone loss (46).
We previously reported biological characterization, as well as short-term clinical data [median follow-up of surviving patients was 7.5 months (range 2.5–15) as compared to 70.4 months in the present study], of 43 patients receiving zoledronic acid after TcRαβ/CD19-depleted haploHSCT (20).
Our findings confirm that multiple infusions of zoledronic acid (up to 6 administrations) in children after HSCT, starting even a few weeks after the infusion of HSC, are well-tolerated and safe. In particular, no serious adverse event was recorded (specifically no cases of osteonecrosis of the jaw), as previously reported by Dieli in adults affected by prostate cancer (24) and by the aforementioned study of Russell in neuroblastoma patients (44). Moreover, the drug was safely administered in an outpatient setting, even in children aged 1 year or less.
As already reported (13), despite the lack of any pharmacological post-transplant prophylaxis, in our study the incidence of both acute and chronic GvHD was limited (with no patient experiencing grade III-IV acute or extensive chronic GvHD), much lower than that of other similar case series (47) or other type of graft manipulation, such as CD3/CD19-depletion (48). Of note, none of the patients experienced de novo onset or worsening of previously developed aGvHD, supporting the hypothesis that γδ T-lymphocytes do not cause GvHD (34). Moreover, our findings showing that patients receiving multiple infusions of zoledronic acid have a reduced incidence of both acute and chronic GvHD are of particular interest. Indeed, this is the first experience (20) of such an induced effect in vivo and deserves further investigation. Immunoregulatory properties of TcRγδ T-cells were first described by Patel and colleagues (49) and are now beginning to be investigated. Notably, Drobyski and colleagues noted that, in mice, activated TcRγδ T-cells are capable of modulating the ability of MHC-incompatible TcRαβ T-cells to cause GvHD after HSCT (50). Both Vδ1 and Vδ2 subsets may display regulatory properties, depending on different settings. Moreover, stimulation with pyrophosphates can induce regulatory capacities on Vδ2 T cells a few days after initial stimulation (51). In particular, IPP-stimulated Vδ2 T lymphocytes can inhibit the proliferation of CD4+ and CD8+ αβ T cells in response to strong recall antigens (52). Interestingly, strong co-stimulatory antigen-processing cell (APC) signals (i.e., acute GvHD initiating condition) seem to play an important role in the induction of these suppressive properties. The mechanisms of suppression by TcRγδ T-cells are still not clear; while some authors identified TGFβ, IL-10, and other suppressive cytokines as mediators of these effects (53), other data suggested that cell-to-cell interactions, via CD80, CD86, and PDL-1 expressed on Vδ2 T lymphocytes, are necessary to achieve suppression of other cell populations (54). Another unresolved issue is the lack of defined regulatory γδ T-cell phenotype (51), although CD39 (55) and latency-associated peptide (a membrane-bound TGF-β1) (56) have been identified as putative markers; thus, further studies are needed to address this question.
The cumulative incidence of TRM in our study cohort was remarkably low (4.3%), with 2 patients dying due to infection. Repeated infusions of zoledronic acid seems to also have a role in reducing TRM, since we recorded no infectious deaths in children who received more than 2 administrations. The well-known anti-infectious role of TcRγδ T-cells has been very recently demonstrated by Perko and colleagues in children after HSCT (38). Indeed, using a logistic regression model, they showed that the higher the number of TcRγδ T-cells after HSCT, the lower the risk of infection, which results in an improved EFS. However, because of the limited number of patients per cohort, subanalysis on different types of donors (siblings, MUDs, haploidentical relatives, and umbilical cord blood) failed to demonstrate a similar effect in any single subgroup. In our study, repeated infusions of zoledronic acid did not affect the incidence of infections of either viral, bacterial, or fungal origin. However, patients receiving multiple infusions had a reduced, although not statistically significant, cumulative incidence of CMV infection. Given the low number of patients enrolled in this study, it is possible that the number of events was not sufficient to highlight such an effect.
The OS estimate observed in our study (without any difference between ALL, either of T or B lineage, AML patients), in line with our recently published cohort (13), is higher than that reported in other pediatric trials of CD34 selected (57, 58) or CD3/CD19-depleted haploidentical HSCT (48), suggesting a favorable impact of TcRγδ T-cells on outcome. Outcome data were also similar to those reported by Maschan et al., who used the same type of graft manipulation, although with different study population and donors (47), making comparison difficult to interpret. Smetak and colleagues studied a different method of T-cell depletion, namely based on CD4+ and CD8+ depletion, able to leave in the graft innate lymphocytes such as TcRγδ T cells and NK cells (59). Despite similar depletion capacity, other authors noted that the B-cell content of the graft was high, raising concerns about the risk of EBV-associated PTLD (60).
We previously demonstrated that the use of TBI in the conditioning regimen positively influenced the outcome in this setting, probably due to a potent antileukemia effect, compensating for the lack of TcRαβ cell–mediated GvL effect (13). Here, we show that patients receiving more than 2 infusions of zoledronic acid seem to have an improved OS, although the difference did not reach the statistical significance level. However, when stratified according to the type of conditioning regimen employed, the independent beneficial effect of zoledronic acid on OS became evident, as also highlighted by multivariable analysis. As zoledronic acid improves cytotoxicity of TcRγδ T-cells, the initial hypothesis was that multiple infusions could reduce RI. However, due to the limited number of patients enrolled and events observed, it was not possible to draw any firm conclusion; in particular, we were not able to highlight a significant reduction of RI. Since time to relapse and disease status were comparable between patients receiving 1–2 or 3–6 infusions of zoledronic acid, the trend toward a better outcome of patients receiving multiple infusions does not seem to be due to the fact that those patients had longer DFS allowing for multiple infusions nor because they had a less aggressive disease.
We were not able to demonstrate an association between an increased number of TcRγδ T-cells after HSCT and an improved outcome (data not shown), as demonstrated by Lamb et al. (9, 37). A possible explanation for these apparently discordant findings can be given by the observation that, as shown by our data, the percentage and absolute number of TcRγδ T-lymphocytes, and especially of the Vδ2 subset, decrease over time after prolonged exposure to zoledronic acid (20). This phenomenon, in contrast with the first in vitro observations by Kunzmann (40), was previously proven by Dieli and coauthors in patients treated with zoledronic acid for hormone-refractory prostate cancer (24) and by Kalyan and colleagues in post-menopausal women treated with bisphosphonates for osteoporosis (61). These authors demonstrated an inhibitory effect on TcRγδ T-cells produced by neutrophils upon bisphosphonate uptake through the production of reactive oxygen species (62). Although the use of IL-2 could improve proliferation of TcRγδ T-lymphocytes (24), we decided not to administer this cytokine because of a possible increase in the risk of GvHD (63).
The main limitations of this study include: i) the lack of a control arm and ii) a variability of the administration schedule, this limiting the possibility to draw firm conclusions on the number and timing of zoledronic acid administration. Finally, we did not systematically collect data on bone metabolism pre and post zoledronic acid infusions. Since it is well-known that patients undergoing HSCT suffer from osteopenia (64), data on bone metabolism after zoledronic acid administration could help guide post-transplant supportive therapies.
In conclusion, our data indicate that the infusion of zoledronic acid after TcRαβ/CD19-depleted haploidentical HSCT is safe. Three or more infusions of zoledronic acid result in a lower incidence of both acute and chronic GvHD and lower TRM. Moreover, they had an independent effect in ameliorating the outcome. Further investigation (a non-randomized prospective trial is currently ongoing at Wisconsin University, ClinicalTrials.gov Identifier: NCT02508038), namely a randomized-controlled trial, is needed to confirm these data.
Data Availability Statement
The datasets generated for this study are available on request to the corresponding author.
Ethics Statement
The study involving human participants was reviewed and approved by the Ethical Committee of Bambino Gesù Children's Hospital. Written informed consent to participate in this study was provided by the participants' legal guardian/next of kin.
Author Contributions
PM, MA, FG, GM, GP, LS, DP, and FL designed the study, analyzed data, and wrote the paper. VB, SB, EG, MS, and IA performed graft manipulation, characterized the graft, and edited the paper. PM, MA, FG, GM, MB, GL, EB, LG, SG, DP, and FL treated patients, collected data, analyzed data, and edited the paper. All authors approved the final version of the paper.
Funding
This work was partly supported by grants from: AIRC (Associazione Italiana Ricerca sul Cancro, Investigator Grant-ID 21724), Ministero della Salute (Ricerca Finalizzata to FL), and Ministero dell'Istruzione, Università e Ricerca (MIUR, Project PRIN 2017-ID 2017WC8499-to FL).
Conflict of Interest
The authors declare that the research was conducted in the absence of any commercial or financial relationships that could be construed as a potential conflict of interest.
Supplementary Material
The Supplementary Material for this article can be found online at: https://www.frontiersin.org/articles/10.3389/fimmu.2020.00699/full#supplementary-material
Supplementary Figure 1. Cumulative incidence of relapse and TRM of the whole cohort of 46 patients.
Supplementary Figure 2. Cumulative incidence of relapse of patients receiving either 1–3 or 4–6 infusions of zoledronic acid (ZOL).
Supplementary Figure 3. DFS of the whole cohort of 46 patients. B. DFS of patients receiving either 1-2 or 3-6 infusions of zoledronic acid (ZOL).
References
1. Copelan EA. Hematopoietic stem-cell transplantation. N Engl J Med. (2006) 354:1813–26. doi: 10.1056/NEJMra052638
2. Locatelli F, Giorgiani G, Di-Cesare-Merlone A, Merli P, Sparta V, Moretta F. The changing role of stem cell transplantation in childhood. Bone Marrow Transplant. (2008) 41(Suppl. 2):S3–7. doi: 10.1038/bmt.2008.45
3. Reisner Y, Hagin D, Martelli MF. Haploidentical hematopoietic transplantation: current status and future perspectives. Blood. (2011) 118:6006–17. doi: 10.1182/blood-2011–07-338822
4. Oevermann L, Lang P, Feuchtinger T, Schumm M, Teltschik HM, Schlegel P, et al. Immune reconstitution and strategies for rebuilding the immune system after haploidentical stem cell transplantation. Ann N Y Acad Sci. (2012) 1266:161–70. doi: 10.1111/j.1749–6632.2012.06606.x
5. de Koning C, Plantinga M, Besseling P, Boelens JJ, Nierkens S. Immune reconstitution after allogeneic hematopoietic cell transplantation in children. Biol Blood Marrow Transplant. (2016) 22:195–206. doi: 10.1016/j.bbmt.2015.08.028
6. Bayraktar UD, Milton DR, Guindani M, Rondon G, Chen J, Al-Atrash G, et al. Optimal threshold and time of absolute lymphocyte count assessment for outcome prediction after bone marrow transplantation. Biol Blood Marrow Transplant. (2015) 22:505–13. doi: 10.1016/j.bbmt.2015.10.020
7. Platzbecker U, Ehninger G, Bornhauser M. Allogeneic transplantation of CD34+ selected hematopoietic cells–clinical problems and current challenges. Leuk Lymphoma. (2004) 45:447–53 doi: 10.1080/10428190310001615684
8. Ruggeri L, Capanni M, Urbani E, Perruccio K, Shlomchik WD, Tosti A, et al. Effectiveness of donor natural killer cell alloreactivity in mismatched hematopoietic transplants. Science. (2002) 295:2097–100. doi: 10.1126/science.1068440
9. Godder KT, Henslee-Downey PJ, Mehta J, Park BS, Chiang KY, Abhyankar S, et al. Long term disease-free survival in acute leukemia patients recovering with increased gammadelta T cells after partially mismatched related donor bone marrow transplantation. Bone Marrow Transplant. (2007) 39:751–7. doi: 10.1038/sj.bmt.1705650
10. Bertaina A, Merli P, Rutella S, Pagliara D, Bernardo ME, Masetti R, et al. HLA-haploidentical stem cell transplantation after removal of αβ+ T and B-cells in children with non-malignant disorders. Blood. (2014) 124:822–6. doi: 10.1182/blood-2014–03-563817
11. Chaleff S, Otto M, Barfield RC, Leimig T, Iyengar R, Martin J, et al. A large-scale method for the selective depletion of alphabeta T lymphocytes from PBSC for allogeneic transplantation. Cytotherapy. (2007) 9:746–54. doi: 10.1080/14653240701644000
12. Schumm M, Lang P, Bethge W, Faul C, Feuchtinger T, Pfeiffer M, et al. Depletion of T-cell receptor alpha/beta and CD19 positive cells from apheresis products with the CliniMACS device. Cytotherapy. (2013) 15:1253–8. doi: 10.1016/j.jcyt.2013.05.014
13. Locatelli F, Merli P, Pagliara D, Li Pira G, Falco M, Pende D, et al. Outcome of children with acute leukemia given HLA-haploidentical HSCT after alphabeta T-cell and B-cell depletion. Blood. (2017) 130:677–85. doi: 10.1182/blood-2017–04-779769
14. Bonneville M, O'Brien RL, Born WK. Gammadelta T cell effector functions: a blend of innate programming and acquired plasticity. Nat Rev Immunol. (2010) 10:467–78. doi: 10.1038/nri2781
15. Locatelli F, Merli P, Rutella S. At the Bedside: innate immunity as an immunotherapy tool for hematological malignancies. J Leukoc Biol. (2013) 94:1141–57. doi: 10.1189/jlb.0613343
16. Airoldi I, Bertaina A, Prigione I, Zorzoli A, Pagliara D, Cocco C, et al. gammadelta T-cell reconstitution after HLA-haploidentical hematopoietic transplantation depleted of TCR-alphabeta+/CD19+ lymphocytes. Blood. (2015) 125:2349–58. doi: 10.1182/blood-2014–09-599423
17. Kunzmann V, Bauer E, Wilhelm M. Gamma/delta T-cell stimulation by pamidronate. N Engl J Med. (1999) 340:737–8. doi: 10.1056/NEJM199903043400914
18. Gu S, Nawrocka W, Adams EJ. Sensing of pyrophosphate metabolites by Vgamma9Vdelta2 T cells. Front Immunol. (2014) 5:688. doi: 10.3389/fimmu.2014.00688
19. Nishio N, Fujita M, Tanaka Y, Maki H, Zhang R, Hirosawa T, et al. Zoledronate sensitizes neuroblastoma-derived tumor-initiating cells to cytolysis mediated by human gammadelta T cells. J Immunother. (2012) 35:598–606. doi: 10.1097/CJI.0b013e31826a745a
20. Bertaina A, Zorzoli A, Petretto A, Barbarito G, Inglese E, Merli P, et al. Zoledronic acid boosts γδ T-cell activity in children receiving αβ+ T and CD19+ cell depleted grafts from an HLA-haploidentical donor. Oncoimmunology. (2016) 27:e1216291. doi: 10.1080/2162402X.2016.1216291
21. Moretta L, Locatelli F, Pende D, Marcenaro E, Mingari MC, Moretta A. Killer Ig-like receptor-mediated control of natural killer cell alloreactivity in haploidentical hematopoietic stem cell transplantation. Blood. (2011) 117:764–71. doi: 10.1182/blood-2010–08-264085
22. Li Pira G, Malaspina D, Girolami E, Biagini S, Cicchetti E, Conflitti G, et al. Selective depletion of alphabeta T cells and B cells for human leukocyte antigen-haploidentical hematopoietic stem cell transplantation. A three-year flow-up of procedure efficiency. Biol Blood Marrow Transplant. (2016) 22:2056–64. doi: 10.1016/j.bbmt.2016.08.006
23. Ooi HL, Briody J, Biggin A, Cowell CT, Munns CF. Intravenous zoledronic Acid given every 6 months in childhood osteoporosis. Horm Res Paediatr. (2013) 80:179–84. doi: 10.1159/000354303
24. Dieli F, Vermijlen D, Fulfaro F, Caccamo N, Meraviglia S, Cicero G, et al. Targeting human {gamma}delta} T cells with zoledronate and interleukin-2 for immunotherapy of hormone-refractory prostate cancer. Cancer Res. (2007) 67:7450–7. doi: 10.1158/0008–5472.CAN-07–0199
25. Byar DP. Identification of prognostic factorsm, in Cancer clinical trials, eds Buyse ME, Staquet MJ, Sylvester RJ (New York, NY: Oxford University Press) (1988) 423–43.
26. Glucksberg H, Storb R, Fefer A, Buckner CD, Neiman PE, Clift RA, et al. Clinical manifestations of graft-versus-host disease in human recipients of marrow from HL-A-matched sibling donors. Transplantation. (1974) 18:295–304. doi: 10.1097/00007890–197410000-00001
27. Shulman HM, Sullivan KM, Weiden PL, McDonald GB, Striker GE, Sale GE, et al. Chronic graft-versus-host syndrome in man. A long-term clinicopathologic study of 20 Seattle patients. Am J Med. (1980) 69:204–17. doi: 10.1016/0002–9343(80)90380–0
28. Kaplan E, Meier P. Non parametric estimation from incomplete observations. J Am Stat Assoc. (1958) 53:457–81. doi: 10.1080/01621459.1958.10501452
29. Gooley TA, Leisenring W, Crowley J, Storer BE. Estimation of failure probabilities in the presence of competing risks: new representations of old estimators. Stat Med. (1999) 18:695–706.
30. lein JP, Rizzo JD, Zhang MJ, Keiding N. Statistical methods for the analysis and presentation of the results of bone marrow transplants. part I: unadjusted analysis. Bone Marrow Transplant. (2001) 28:909–15. doi: 10.1038/sj.bmt.1703260
31. Gray R. A class of K-sample tests for comparing the cumulative incidence of a competing risk. Ann Stat. (1988) 16:1141–54. doi: 10.1214/aos/1176350951
32. Kanda Y. Investigation of the freely available easy-to-use software 'EZR' for medical statistics. Bone Marrow Transplant. (2013) 48:452–8. doi: 10.1038/bmt.2012.244
33. Vantourout P, Hayday A. Six-of-the-best: unique contributions of γδ T cells to immunology. Nat Rev Immunol. (2013) 13:88–100. doi: 10.1038/nri3384
34. Lamb LS Jr, Musk P, Ye Z, van Rhee F, Geier SS, Tong JJ, et al. Human gammadelta(+) T lymphocytes have in vitro graft vs leukemia activity in the absence of an allogeneic response. Bone Marrow Transplant. (2001) 27:601–6. doi: 10.1038/sj.bmt.1702830
35. Wilhelm M, Kunzmann V, Eckstein S, Reimer P, Weissinger F, Ruediger T, et al. Gammadelta T cells for immune therapy of patients with lymphoid malignancies. Blood. (2003) 102:200–6. doi: 10.1182/blood-2002–12-3665
36. Born WK, Kemal Aydintug M, O'Brien RL. Diversity of γδ T-cell antigens. Cell Mol Immunol. (2013) 10:13–20. doi: 10.1038/cmi.2012.45
37. Lamb LS Jr, Henslee-Downey PJ, Parrish RS, Godder K, Thompson J, Lee C, et al. Increased frequency of TCR gamma delta + T cells in disease-free survivors following T cell-depleted, partially mismatched, related donor bone marrow transplantation for leukemia. J Hematother. (1996) 5:503–9. doi: 10.1089/scd.1.1996.5.503
38. Perko R, Kang G, Sunkara A, Leung W, Thomas PG, Dallas MH. Gamma delta T cell reconstitution is associated with fewer infections and improved event-free survival after hematopoietic stem cell transplantation for pediatric leukemia. Biol Blood Marrow Transplant. (2015) 21:130–6. doi: 10.1016/j.bbmt.2014.09.027
39. Lamb LS Jr, Gee AP, Hazlett LJ, Musk P, Parrish RS, O'Hanlon TP, et al. Influence of T cell depletion method on circulating gammadelta T cell reconstitution and potential role in the graft-versus-leukemia effect. Cytotherapy. (1999) 1:7–19 doi: 10.1080/0032472031000141295
40. Kunzmann V, Bauer E, Feurle J, Weissinger F, Tony HP, Wilhelm M. Stimulation of gammadelta T cells by aminobisphosphonates and induction of antiplasma cell activity in multiple myeloma. Blood. (2000) 96:384–92. doi: 10.1182/blood.V96.2.384
41. Gober HJ, Kistowska M, Angman L, Jeno P, Mori L, de Libero G. Human T cell receptor gammadelta cells recognize endogenous mevalonate metabolites in tumor cells. J Exp Med. (2003) 197:163–8. doi: 10.1084/jem.20021500
42. Idrees AS, Sugie T, Inoue C, Murata-Hirai K, Okamura H, Morita CT, et al. Comparison of gammadelta T cell responses and farnesyl diphosphate synthase inhibition in tumor cells pretreated with zoledronic acid. Cancer Sci. (2013) 104:536–42. doi: 10.1111/cas.12124
43. Simm PJ, Johannesen J, Briody J, McQuade M, Hsu B, Bridge C, et al. Zoledronic acid improves bone mineral density, reduces bone turnover and improves skeletal architecture over 2 years of treatment in children with secondary osteoporosis. Bone. (2011) 49:939–43. doi: 10.1016/j.bone.2011.07.031
44. Russell HV, Groshen SG, Ara T, DeClerck YA, Hawkins R, Jackson HA, et al. A phase I study of zoledronic acid and low-dose cyclophosphamide in recurrent/refractory neuroblastoma: a new approaches to neuroblastoma therapy (NANT) study. Pediatr Blood Cancer. (2011) 57:275–82. doi: 10.1002/pbc.22821
45. McClune BL, Polgreen LE, Burmeister LA, Blaes AH, Mulrooney DA, Burns LJ, et al. Screening, prevention and management of osteoporosis and bone loss in adult and pediatric hematopoietic cell transplant recipients. Bone Marrow Transplant. (2011) 46:1–9. doi: 10.1038/bmt.2010.198
46. Grigg A, Butcher B, Khodr B, Bajel A, Hertzberg M, Patil S, et al. An individualised risk-adapted protocol of pre- and post transplant zoledronic acid reduces bone loss after allogeneic stem cell transplantation: results of a phase II prospective trial. Bone Marrow Transplant. (2017) 52:1288–93. doi: 10.1038/bmt.2017.108
47. Maschan M, Shelikhova L, Ilushina M, Kurnikova E, Boyakova E, Balashov D, et al. TCR-alpha/beta and CD19 depletion and treosulfan-based conditioning regimen in unrelated and haploidentical transplantation in children with acute myeloid leukemia. Bone Marrow Transplant. (2016) 51:668–74. doi: 10.1038/bmt.2015.343
48. Lang P, Teltschik HM, Feuchtinger T, Muller I, Pfeiffer M, Schumm M, et al. Transplantation of CD3/CD19 depleted allografts from haploidentical family donors in paediatric leukaemia. Br J Haematol. (2014) 165:688–98. doi: 10.1111/bjh.12810
49. Patel SS, Wacholtz MC, Duby AD, Thiele DL, Lipsky PE. Analysis of the functional capabilities of CD3+CD4-CD8- and CD3+CD4+CD8+ human T cell clones. J Immunol. (1989) 143:1108–17.
50. Drobyski WR, Vodanovic-Jankovic S, Klein J. Adoptively transferred gamma delta T cells indirectly regulate murine graft-versus-host reactivity following donor leukocyte infusion therapy in mice. J Immunol. (2000) 165:1634–40. doi: 10.4049/jimmunol.165.3.1634
51. Wesch D, Peters C, Siegers GM. Human gamma delta T regulatory cells in cancer: fact or fiction? Front Immunol. (2014) 5:598. doi: 10.3389/fimmu.2014.00598
52. Traxlmayr MW, Wesch D, Dohnal AM, Funovics P, Fischer MB, Kabelitz D, et al. Immune suppression by gammadelta T-cells as a potential regulatory mechanism after cancer vaccination with IL-12 secreting dendritic cells. J Immunother. (2010) 33:40–52. doi: 10.1097/CJI.0b013e3181b51447
53. Kuhl AA, Pawlowski NN, Grollich K, Blessenohl M, Westermann J, Zeitz M, et al. Human peripheral gammadelta T cells possess regulatory potential. Immunology. (2009) 128:580–8. doi: 10.1111/j.1365–2567.2009.03162.x
54. Peters C, Oberg HH, Kabelitz D, Wesch D. Phenotype and regulation of immunosuppressive Vδ2-expressing γδ T cells. Cell Mol Life Sci. (2014) 71:1943–60. doi: 10.1007/s00018–013-1467–1
55. Otsuka A, Hanakawa S, Miyachi Y, Kabashima K. CD39: a new surface marker of mouse regulatory γδ T cells. J Allergy Clin Immunol. (2013) 132:1448–51. doi: 10.1016/j.jaci.2013.05.037
56. Rezende RM, da Cunha AP, Kuhn C, Rubino S, M'Hamdi H, Gabriely G, et al. Identification and characterization of latency-associated peptide-expressing gammadelta T cells. Nat Commun. (2015) 6:8726. doi: 10.1038/ncomms9726
57. Klingebiel T, Cornish J, Labopin M, Locatelli F, Darbyshire P, Handgretinger R, et al. Results and factors influencing outcome after fully haploidentical hematopoietic stem cell transplantation in children with very high-risk acute lymphoblastic leukemia: impact of center size: an analysis on behalf of the acute leukemia and pediatric disease working parties of the European blood and marrow transplant group. Blood. (2010) 115:3437–46. doi: 10.1182/blood-2009–03-207001
58. Marks DI, Khattry N, Cummins M, Goulden N, Green A, Harvey J, et al. Haploidentical stem cell transplantation for children with acute leukaemia. Br J Haematol. (2006) 134:196–201. doi: 10.1111/j.1365–2141.2006.06140.x
59. Smetak M, Kimmel B, Birkmann J, Schaefer-Eckart K, Einsele H, Wilhelm M, et al. Clinical-scale single-step CD4(+) and CD8(+) cell depletion for donor innate lymphocyte infusion (DILI). Bone Marrow Transplant. (2008) 41:643–50. doi: 10.1038/sj.bmt.1705942
60. Lamb LS Jr, Sande J. B-cell enrichment and infusion in CD4+/CD8+ T-cell depleted products for donor innate immune lymphocyte infusion: is risk of EBV-associated lymphocyte post transplantation lymphoproliferative disease a concern? Bone Marrow Transplant. (2008) 41:995–6. doi: 10.1038/bmt.2008.17
61. Kalyan S, Quabius ES, Wiltfang J, Monig H, Kabelitz D. Can peripheral blood gammadelta T cells predict osteonecrosis of the jaw? An immunological perspective on the adverse drug effects of aminobisphosphonate therapy. J Bone Miner Res. (2013) 28:728–35. doi: 10.1002/jbmr.1769
62. Kalyan S, Chandrasekaran V, Quabius ES, Lindhorst TK, Kabelitz D. Neutrophil uptake of nitrogen-bisphosphonates leads to the suppression of human peripheral blood gammadelta T cells. Cell Mol Life Sci. (2014) 71:2335–46. doi: 10.1007/s00018–013-1495-x
63. Lucarelli B, Merli P, Bertaina V, Locatelli F. Strategies to accelerate immune recovery after allogeneic hematopoietic stem cell transplantation. Expert Rev Clin Immunol. (2016) 12:343–58. doi: 10.1586/1744666X.2016.1123091
Keywords: TcRγδ+ lymphocytes, zoledronic acid, TcRαβ/CD19 cell depleted haploidentical stem cell transplantation, acute leukemia, children
Citation: Merli P, Algeri M, Galaverna F, Milano GM, Bertaina V, Biagini S, Girolami E, Palumbo G, Sinibaldi M, Becilli M, Leone G, Boccieri E, Grapulin L, Gaspari S, Airoldi I, Strocchio L, Pagliara D and Locatelli F (2020) Immune Modulation Properties of Zoledronic Acid on TcRγδ T-Lymphocytes After TcRαβ/CD19-Depleted Haploidentical Stem Cell Transplantation: An analysis on 46 Pediatric Patients Affected by Acute Leukemia. Front. Immunol. 11:699. doi: 10.3389/fimmu.2020.00699
Received: 19 February 2020; Accepted: 27 March 2020;
Published: 12 May 2020.
Edited by:
Bruno Silva-Santos, Universidade de Lisboa, PortugalReviewed by:
Matthias Eberl, Cardiff University, United KingdomEmmanuel Scotet, Institut National de la Santé et de la Recherche Médicale (INSERM), France
Copyright © 2020 Merli, Algeri, Galaverna, Milano, Bertaina, Biagini, Girolami, Palumbo, Sinibaldi, Becilli, Leone, Boccieri, Grapulin, Gaspari, Airoldi, Strocchio, Pagliara and Locatelli. This is an open-access article distributed under the terms of the Creative Commons Attribution License (CC BY). The use, distribution or reproduction in other forums is permitted, provided the original author(s) and the copyright owner(s) are credited and that the original publication in this journal is cited, in accordance with accepted academic practice. No use, distribution or reproduction is permitted which does not comply with these terms.
*Correspondence: Pietro Merli, pietro.merli@opbg.net