- Department of Infectious Diseases and Microbiology, University of Lübeck, Lübeck, Germany
Neutrophils act as the first line of defense against invading pathogens. Although traditionally considered in context of their antimicrobial effector functions, the importance of tumor-associated neutrophils (TANs) in the development of cancer has become increasingly clear during the last decade. With regard to their high plasticity, neutrophils were shown to acquire an anti-tumorigenic N1 or a pro-tumorigenic N2 phenotype. Despite the urgent need to get a comprehensive understanding of the interaction of TANs with their tumor microenvironment, most studies still rely on murine tumor models. Here we present for the first time a polarization attempt to generate N1 and N2 neutrophils from primary human neutrophils in vitro. Our results underscore that N1-polarized neutrophils have a pro-inflammatory phenotype characterized among others by a higher level of intercellular adhesion molecule (ICAM)-1 and high secretion of interferon (IFN)γ-induced protein 10 (IP-10)/C-X-C motif chemokine 10 (CXCL10) and tumor necrosis factor (TNF). Further, we demonstrate that neutrophils incubated under a tumor-mimicking in vitro environment show a high cell surface expression of C-X-C motif chemokine receptor 2 (CXCR2) and secrete high levels of interleukin (IL)-8. These findings suggest that it is feasible to polarize blood-derived primary human neutrophils toward N1- and N2-like phenotypes in vitro. Further, we hypothesized that the presence of anti-inflammatory neutrophil phenotype is not a phenomenon limited to cancer but also occurs when neutrophils are infected with intracellular pathogens. Indeed, our findings indicate that N2-polarized neutrophils exert a markedly decreased capacity to kill the protozoan parasite Leishmania donovani and therefore permit parasite persistence.
Introduction
Neutrophils, also known as polymorphonuclear leukocytes (PMN), are the predominant immune cell population in human blood. Neutrophils are produced in large numbers, ∼1011 cells per day, in the bone marrow from hematopoietic stem cells during granulopoiesis (1). They are regarded as the body’s first line of defense against invading pathogens, which they fight by using an array of diverse antimicrobial effector mechanisms ranging from phagocytosis, the release of granules to the formation of NETs. In recent years, however, accumulating evidence has shown that neutrophils possess greater functional diversity than previously appreciated and appear to play an important role under non-infectious conditions as well, most notably in cancer. Apart from cancer cells, solid tumors contain often different types of immune cells, including infiltrating neutrophils (2). The neutrophils found in the tumor are referred to as TANs. The mobilization of neutrophils from the circulation toward the tumor is controlled by the C-X-C motif chemokine receptor 2 (CXCR2) axis (3). Various cell types in the tumor release CXCR2 ligands, such as C-X-C motif chemokine (CXCL)1–3 and CXCL5–8 (4). Neutrophils expressing CXCR2 on their surface sense the chemotactic gradient move toward the higher concentration of CXCR2 ligands and get in direct contact with the tumor. Neutrophils display a high plasticity with the ability to adapt their function to the needs of their surrounding micromilieu. Several factors in the tumor microenvironment influence the polarization of recruited neutrophils toward distinct phenotypes. Based on their polarization status, two populations of TANs were recognized, the anti-tumorigenic N1 and the pro-tumorigenic N2 phenotype. This nomenclature was first proposed by Fridlender et al. in 2009 (5) based on similarity to TAMs and the terms M1 and M2. N1 and N2 populations of neutrophils are primarily defined by their functional phenotype because no specific cell surface markers have been identified so far for N1 and N2 neutrophils. N1 neutrophils exhibit an increased tumor cell cytotoxicity and an immunoactivating ability by the elevated production of TNF, intercellular adhesion molecule (ICAM)-1, ROS, and Fas (CD95), as well as the property to decrease the arginase expression (5, 6). In contrast, N2 neutrophils are characterized by higher expression of pro-tumor factors supporting tumor growth (5, 7). Further, in murine tumors, both populations can be distinguished morphologically as N1 neutrophils have hypersegmented nuclei in contrast to the N2 neutrophils, which are circular (5, 8). From a classical view, neutrophils are fully differentiated cells with a short life span. However, TANs display a remarkable longevity (9), and N1 and N2 TANs can possibly be converted into each other. The exposure of neutrophils to transforming growth factor β (TGF-β) (5, 7, 10) or granulocyte colony-stimulating factor (G-CSF) (11) was shown to induce the polarization toward the N2 phenotype. In contrast, a blockade of the TGF-β signaling or a type I interferon (IFN) treatment (12) results in N1 polarization in vivo.
The occurrence of opposing neutrophil subpopulations driving disease progression is not limited to cancer. Pro- and anti-inflammatory populations of neutrophils were further observed in myocardial infarction (13), SLE, rheumatoid arthritis or rheumatic fever (14), sepsis (15), psoriasis (16), HIV infection (17), asthma (18), antineutrophil cytoplasmic antibody (ANCA)-associated vasculitis (19), malaria (20), and Chagas’ disease (21). The consideration of neutrophil subpopulations in infection and inflammation might explain the exacerbation of certain pathologies if one neutrophil phenotype gets prevalent. An example for the contribution of neutrophils to disease outcome is the infection with the intracellular protozoan parasite Leishmania. Neutrophils possess direct antileishmanial capacities (22). However, as evidenced by parasite persistence (23) and abuse of neutrophils as “Trojan horses” to silently enter macrophages (24, 25), direct parasite killing by neutrophils is inefficient. Several similarities between TANs and the neutrophils from VL patients could be observed. During the acute phase of VL, neutrophils show increased expression of the immunosuppressive molecules interleukin (IL-10) and arginase (26). Further, Leishmania infection was reported to extend the life span of neutrophils up to 2–3 days (27). Considering the likelihood of a polarization of neutrophils toward an immunosuppressive phenotype during L. donovani infection, we hypothesize here that in VL, L. donovani can induce the polarization of neutrophils toward an anti-inflammatory and non-antimicrobial N2 phenotype in order to survive inside neutrophils.
Despite the enormous interest in TANs in the recent years, our current understanding of the role of neutrophils in tumor development is primarily based on murine models of cancer. To our knowledge, this is the first polarization attempt to polarize human N1 and N2 neutrophils in vitro. In this experimental setup, we cultivated primary human neutrophils in the presence of a pro-inflammatory polarization cocktail to generate N1 neutrophils, whereas N2 neutrophils were generated by incubation with a polarization cocktail mimicking hallmarks of the tumor microenvironment. To evaluate whether the neutrophil phenotypes generated in our in vitro study share characteristics with in vivo polarized neutrophils, phenotypic markers and features described for N1 or N2 TANs were assessed. In addition, as a measure of antimicrobial capacity, the anti-leishmanial capacity of the in vitro polarized neutrophils was determined.
Materials and Methods
Ethics Statement
Blood collection was conducted with the agreement and written consent form of each participant and was approved by the Ethical Committee of the University of Lübeck (18–186).
Isolation of Primary Human Neutrophils
Peripheral blood was collected by venipuncture from healthy adult volunteers using lithium–heparin collection tubes (S-Monovette® 9 ml LH, Sarstedt, Nümbrecht, Germany). Blood was layered on a two-layer density gradient consisting of an upper layer of Histopaque® 1077 (Sigma Aldrich, Steinheim, Germany) and a lower layer of Histopaque® 1119 (Sigma Aldrich) and centrifuged for 5 min at 300 × g followed by 25 min at 800 × g. Cells from the upper layer consisting mainly of lymphocytes and monocytes were discarded. The granulocyte-rich lower layer was collected, leaving the erythrocyte pellet at the bottom of the tube. Granulocytes were washed once in 1 × Dulbecco’s phosphate-buffered saline (DPBS) (Thermo Fisher, Germany) for 10 min at 800 × g, resuspended in complete medium [RPMI 1640 Medium (Sigma Aldrich) supplemented with 2 mM L-glutamine (Merck, Darmstadt, Germany), 10 mM 4-(2-hydroxyethyl)-1-piperazineethanesulfonic acid (HEPES; Life Technologies, Darmstadt, Germany), 10% heat-inactivated (FCS; Gibco, Germany), 100 U/ml penicillin, and 100 μg/ml streptomycin (Biochrom, Berlin, Germany)] and further fractionated on a discontinuous Percoll® (GE Healthcare, Braunschweig, Germany) gradient consisting of layers with densities of 1.105 g/ml (85%), 1.100 g/ml (80%), 1.087 g/ml (70%), and 1.081 g/ml (65%). After centrifugation for 25 min at 800 × g, the interface between the 80% and 70% Percoll® layers was collected. The cells were washed once in 1× DPBS for 10 min at 800 × g and resuspended in complete medium to a concentration of 5 × 106 cells/ml. All described procedures were conducted at room temperature and under sterile conditions. Cell counting was conducted with a hemocytometer (Imp. Neubauer, 0.0025 mm2, depth 0.100 mm, VWR, Dresden, Germany) and crystal violet staining. The preparations contained ≥99% granulocytes (Supplementary Figure S1), of which >95% were neutrophils and 1%–4% were eosinophils, as determined by Giemsa staining (Diff Quik Fix, Medion Diagnostics, Berlin, Germany) of cytocentrifuged (Shandon) samples. Contamination with monocytes, T and B lymphocytes was lower than 1% (Supplementary Figure S2).
In vitro Culture and Polarization of Neutrophils
Polarization of neutrophils toward an N1-like phenotype was conducted in six-well plates (Greiner, Kremsmünster, Austria) with 3 ml/well at a cell concentration of 5 × 106/ml in complete medium supplemented with an N1 polarization cocktail containing 100 ng/ml lipopolysaccharide (LPS; Sigma Aldrich), 50 ng/ml IFNγ (R&D Systems, Wiesbaden, Germany), and 10,000 U/ml IFNβ (R&D Systems) at 37°C in a humidified air atmosphere containing 5% carbon dioxide (CO2). Polarization of neutrophils toward an N2-like phenotype was conducted in six-well plates (Greiner) with 3 ml/well in complete medium supplemented with an N2 polarization cocktail containing 25 mM L-lactate (Sigma Aldrich), 10 μM adenosine (Merck), 20 ng/ml TGF-β (PeproTech, Hamburg, Germany), 10 ng/ml IL-10 (BioLegend, San Diego, CA), 20 ng/ml prostaglandin E2 (PGE2; Tocris, Bristol, United Kingdom), and 100 ng/ml granulocyte colony-stimulating factor (G-CSF; PeproTech). The pH of the medium containing the N2 polarization cocktail was adjusted to 6.7 with hydrochloric acid (HCl; Roth, Carlsruhe, Germany) and sodium hydroxide (NaOH; Merck). Cultivation of N2-like neutrophils was conducted in a humidified hypoxic chamber (Toepffer Lab Systems, Göppingen, Germany) with 2% oxygen (O2) and 5% CO2 at 37°C. Since neutrophils have a short life span and undergo apoptosis within a few hours, the cultivation of neutrophils in the polarization experiments was carried out in the presence of 3 μM pan caspase inhibitor QVD-Oph (R&D Systems). This treatment inhibits spontaneous neutrophil apoptosis and, consequently, enhances its life span. In the polarization experiments, the control cells were cultivated in complete medium containing 3 μM QVD-OPh. These control cells were named N0. All polarization experiments were conducted at 37°C in a humidified air atmosphere containing 5% CO2. Functions and phenotypes of the cells were assessed 24 and 48 h after incubation in the given polarization cocktails (N1 or N2) or without polarizing agents (N0).
Flow Cytometry of Cell Surface Molecules
Polarized neutrophils (500,000 cells/sample) were resuspended in FACS buffer [1 × PBS (Thermo Fisher) supplemented with 1% bovine serum albumin (Albumin fraction V, Roth), 1% human serum, and 0.01% sodium azide (Sigma Aldrich)] in a V-bottom plate. After washing once with FACS buffer, the cells were stained with Pacific BlueTM-conjugated mAb to human CD95 [clone DX2, immunoglobulin G1 (IgG1), Biolegend, San Diego, CA], allophycocyanin (APC)-conjugated mAb to human CD54 (clone HA58, IgG1, Biolegend), APC-conjugated mAb to human CD182 (clone 5E8/CXCr2, IgG1, Biolegend), fluorescein isothiocyanate (FITC)-conjugated mAb to human CD66b (clone G10F5, IgM, BD, Heidelberg, Germany), APC-conjugated mAb to human CD62L (clone DREG-56, IgG1, Biolegend), and phycoerythrin (PE)-conjugated mAb to human CD11b (clone 2LPM19c, IgG1, Dako, Waldbronn, Germany) for 30 min at 4°C protected from light.
After two wash steps with FACS buffer at 4°C, the cells were resuspended in FACS buffer and measured with a BD FACS Canto II (BD) flow cytometer. Data analysis was conducted with FlowJo V10.0.7. Representative FACS plots showing gating strategy (Supplementary Figure S2A), percentage of cells or mean fluorescence intensity (MFI) are shown in Supplementary Figure S3 (CD66b, CD11b), Supplementary Figure S4 (CD62L), and Supplementary Figure S5 (CD95, CD54, CD182).
Determination of Cytokines in Culture Supernatants
Cell-free supernatants of polarized neutrophils were collected and stored at −20°C until cytokine determination. The ELISAs for TNF, IFNγ-induced protein 10 (IP-10), myeloperoxidase (MPO), and IL-8 (all DuoSet® ELISA from R&D Systems) were conducted in accordance to the manufacturer’s instructions.
Detection of Reactive Oxygen Species
The sum of intra- and extracellular MPO-derived ROS (28) was measured by using a luminol-amplified chemiluminescence assay. Polarized neutrophils (400,000 cells/sample) were resuspended in complete medium without FCS and seeded in a flat-bottom white 96-well plate (NuncTM F96 MicroWellTM polystyrol plate, Thermo Fisher). Subsequently, 60 μM luminol (Invitrogen, Germany) was added, and the cells were stimulated by the addition of 20 nM phorbol 12-myristate 13-acetate (PMA; Sigma Aldrich). The chemiluminescence resulting from ROS release was analyzed immediately by an Infinite M200pro-Tecan reader (Tecan, Männedorf, Switzerland) and Tecan i-control 1.7 software. ROS release was monitored every minute for a period of 1 h at 37°C and 5% CO2. Extracellular superoxide was detected by using a lucigenin-amplified chemiluminescence assay (28). This assay was performed the same way as the luminol assay, but with 0.2 mM lucigenin (Alexis, Loerrach, Germany) instead of luminol.
Leishmania donovani Culture
Virulent L. donovani promastigotes (strain MHOM/IN/82/Patna 1) were cultivated in Schneider’s Drosophila Medium with L-glutamine (Genaxxon, Ulm, Germany) supplemented with 10% FCS, 100 U/ml penicillin, 100 μg/ml streptomycin, and 2% sterile filtered human urine at 27°C in a humidified air atmosphere containing 5% CO2. For seeding of cultures, a parasite density of 1 × 106 cells/ml was used. The parasite counting was conducted in a hemocytometer with a chamber depth of 0.02 mm (0.0025 mm2, depth 0.02 mm, VWR). The culture was considered to be in the stationary phase 72 h after seeding. Serial passaging was conducted until passage 10.
In vitro Infection of Neutrophils With Leishmania donovani Promastigotes
Neutrophils and L. donovani promastigotes were resuspended in complete medium and coincubated at a parasite–neutrophil ratio of 10:1 and a neutrophil density of 5 × 106 cells/ml for 3 h at 37°C in a humidified air atmosphere containing 5% CO2. Subsequently, the infection rate was assessed on Giemsa-stained cytocentrifuge samples. The cells were washed six times in 1 × PBS at 400 × g for 10 min to remove extracellular Leishmania. Infected cells were again adjusted to 5 × 106 cells/ml.
Quantification of Leishmania donovani Survival in Polarized Neutrophils
A limiting dilution culture assay was used to detect viable L. donovani parasites in polarized neutrophils as described previously (29). Briefly, serial 1.5-fold dilutions of L. donovani-infected neutrophil suspensions (5 × 106 cells/ml) were plated in four replicates in 96-well flat-bottom microtiter plates containing Schneider’s Drosophila Medium. The plates were incubated at 27°C in humidified air atmosphere containing 5% CO2 for 10–14 days. The growth of L. donovani promastigotes was detected microscopically. The last dilution resulting in a growth of parasites in >50% of the wells is given as a quantitative measure of the parasite load in the neutrophil cell suspension.
Statistical Analysis
If not stated differently, the presented data were generated from a minimum of three independent experiments with neutrophils isolated from different blood donors. Statistical analysis was performed with the GraphPad Prism software 6 using the one-way ANOVA followed by Sidak’s t-test for multiple comparisons or unpaired t-test followed by Welch’s correction. A p-value ≤ 0.05 was considered statistically significant.
Results
In vitro Polarized Neutrophils Express Characteristic Surface Markers of N1 and N2 Neutrophils
The N1/N2 terminology of tumor-infiltrating neutrophils was established in analogy of tumor infiltrating M1 and M2 macrophages (5). M1 macrophages activated by IFNs and bacterial products can elicit tumor-destructive reactions by targeting tumor cells (30). The alternatively activated M2 macrophages, on the other hand, support tumor progression by affecting cancer cell proliferation and survival, angiogenesis, and response to hormones (31). Accordingly, in our in vitro approach, the N1 polarization cocktail was composed of lipopolysaccharide (LPS), IFNγ, and IFNβ (Table 1). IFNγ and the bacterial product LPS are known to activate neutrophil functions (32, 33). IFNβ was shown to be an important factor for the generation of N1 TANs (12).
The term N2-phenotype for tumor-promoting neutrophils is based on the functional similarity to tumor-infiltrating M2 macrophages (5). The alternatively activated M2 macrophages support tumor progression by affecting cancer cell proliferation and survival, angiogenesis, and response to hormones (31). To create the N2 polarization cocktail, we added substances that were reported previously in in vivo studies to be associated with the generation of N2 TANs (Table 1). Accordingly, the N2 polarization cocktail contained TGF-β, IL-10, PGE2, and G-CSF. Since high levels of L-lactate and adenosine are characteristic for a tumor microenvironment, L-lactate and adenosine were added to the N2 polarization cocktail (Table 1). In order to further mimic the tumor environment, polarization toward the N2 phenotype was carried out at a low pH and under low oxygen conditions that are additional characteristic features for the tumor environment. The pH of the medium for the N2 polarization was adjusted to 6.7, and the cells were incubated under hypoxic conditions with 2% oxygen. Primary human neutrophils from healthy donors were incubated for 24 or 48 h in the presence of an N1 or N2 polarization cocktail. As polarization effects were investigated over a longer time period, the cells were treated with the Pan-caspase inhibitor QVD-OPh to inhibit spontaneous apoptosis and, consequently, to prolong their life span (34). Treatment with QVD-OPh efficiently suppressed spontaneous apoptosis (Supplementary Figure S6) and cell death (Supplementary Figures S6, S7) of primary human neutrophils for up to 48 h but did not lead to activation (Supplementary Figure S8) or ROS production (Supplementary Figure S9). Neutrophils treated with QVD-OPh in complete medium served in every experiment as control and were further termed N0.
To test whether the in vitro polarized neutrophils correspond to the N1 and N2 phenotypes observed in vivo, several typical N1 and N2 surface markers (Table 2) were investigated by flow cytometry. These included FasR (CD95) and ICAM-1 (CD54) as typical N1 markers (Table 2). Both markers were significantly increased in neutrophils cultured with the N1 polarization cocktail compared to N0 and N2-like neutrophils (Figures 1A,B and Supplementary Figure S5). As N2 marker, the cell surface expression of CXCR2 (CD182) (Table 2) was assessed. The expression of receptor CXCR2 was significantly increased in N2-like as compared to N1-like neutrophils at least after 24 h (Figure 1C and Supplementary Figure S5).
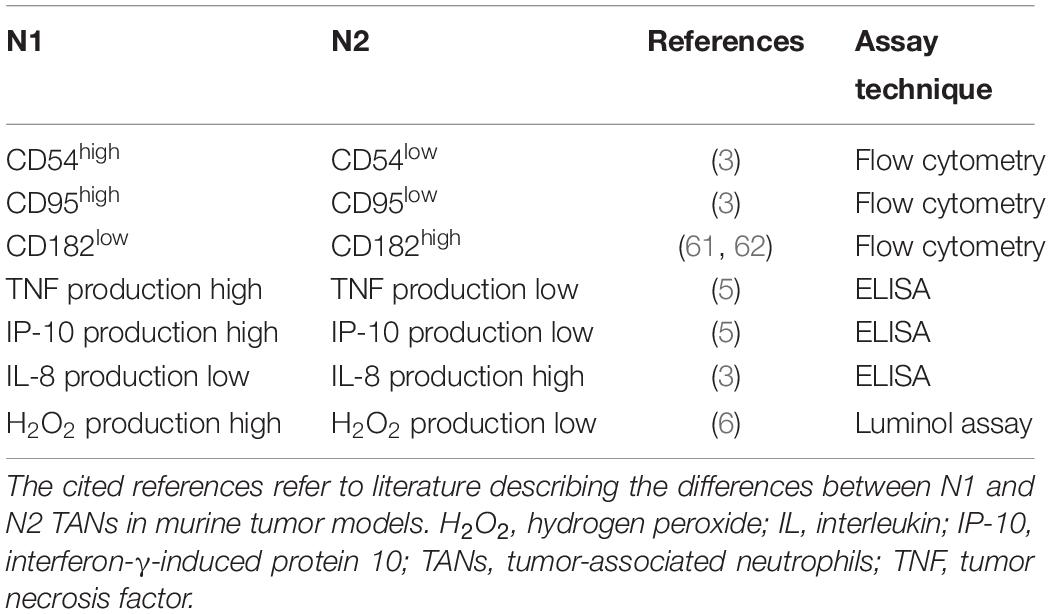
Table 2. Phenotypic features used as readout markers for the characterization of in vitro polarized neutrophils.
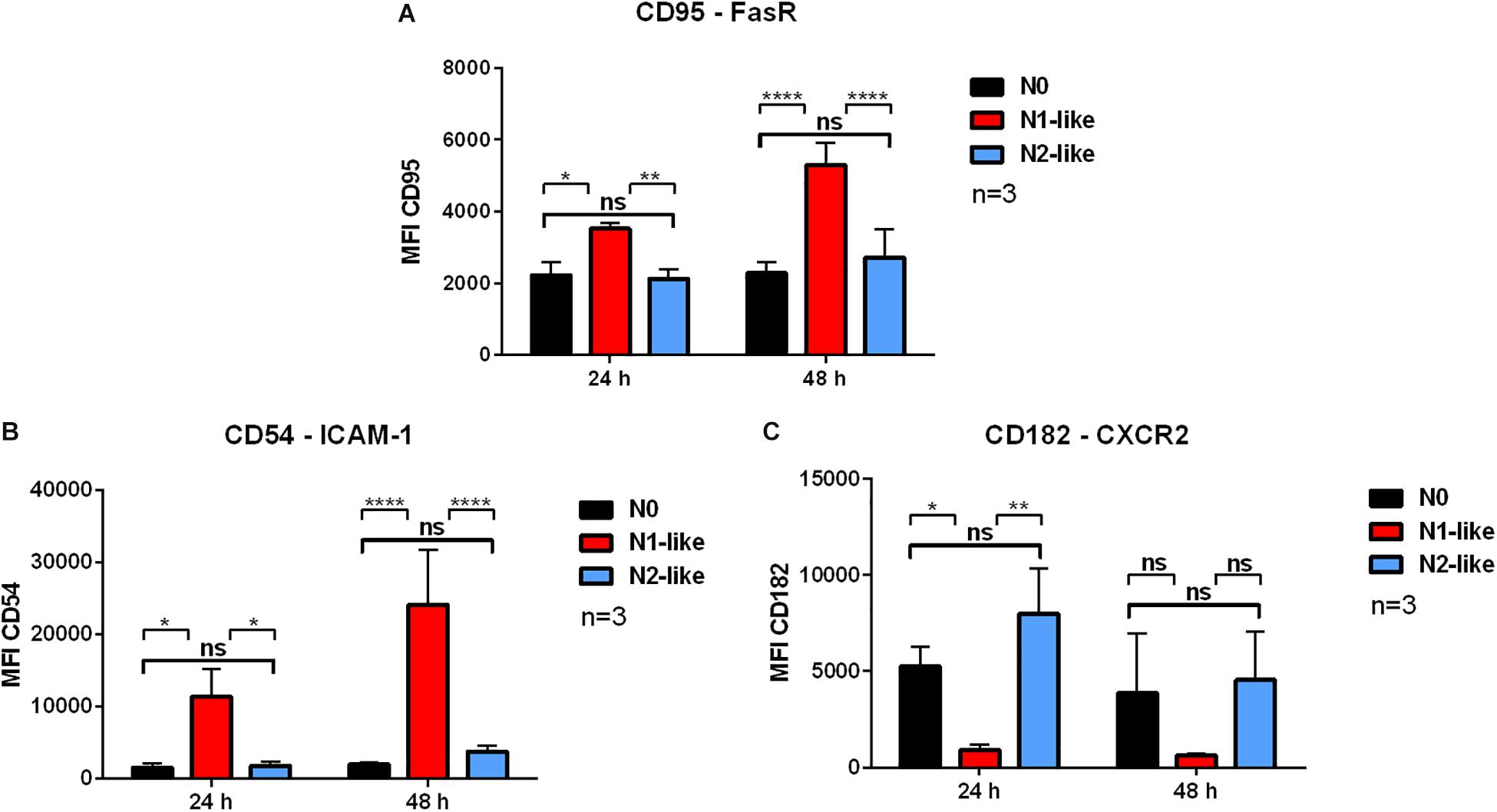
Figure 1. Cell surface expression of typical N1 and N2 markers on in vitro polarized neutrophils. Primary human neutrophils were incubated for 24 or 48 h in the presence of an N1 or N2 polarization cocktail containing the Pan-caspase inhibitor QVD-OPh. Neutrophils which were only treated with QVD-OPh (N0) served as control. The cell surface expression of the typical N1 markers FasR (CD95) (A) and intercellular adhesion molecule (ICAM)-1 (CD54) (B), and the typical N2 marker C-X-C motif chemokine receptor 2 (CXCR2) (CD182) (C) was assessed by using flow cytometry. Bar diagrams show mean fluorescence intensity (MFI) ± SD (n = 3). *p < 0.05, **p < 0.01, ****p < 0.0001, ns = not significant.
In vitro-Generated N1-Like Neutrophils Are Highly Activated
To obtain information about the activation status of the polarized cells, the activation marker L-selectin (CD62L) as well as the degranulation markers CEACAM8 (CD66b) and integrin alpha M (CD11b) were assessed via flow cytometry. Since activation of neutrophils results in the shedding of cell surface L-selectin (35), this cell surface molecule is a widely used marker to distinguish CD62Lneg/low activated cells from CD62Lhigh non-activated neutrophils. Both CD66b and CD11b are present in the membrane of neutrophil granules. Since upon degranulation, the granule membranes fuse with the cell membrane, the enhanced cell surface presence of CD66b and CD11b is a reliable indirect marker of neutrophil degranulation. In addition, degranulation was assessed by measurement of secreted MPO in the supernatants of polarized cells. As compared to N0 and N1-polarized cells, a significantly reduced shedding of CD62L was observed in N2-like polarized neutrophils (Figure 2A and Supplementary Figure S4). The expression of CEACAM8 (CD66b) and integrin alpha M (CD11b) and the secretion of MPO were markedly increased on N1-like polarized cells as compared to N0 and N2-like cells (Figures 2B–D and Supplementary Figure S3).
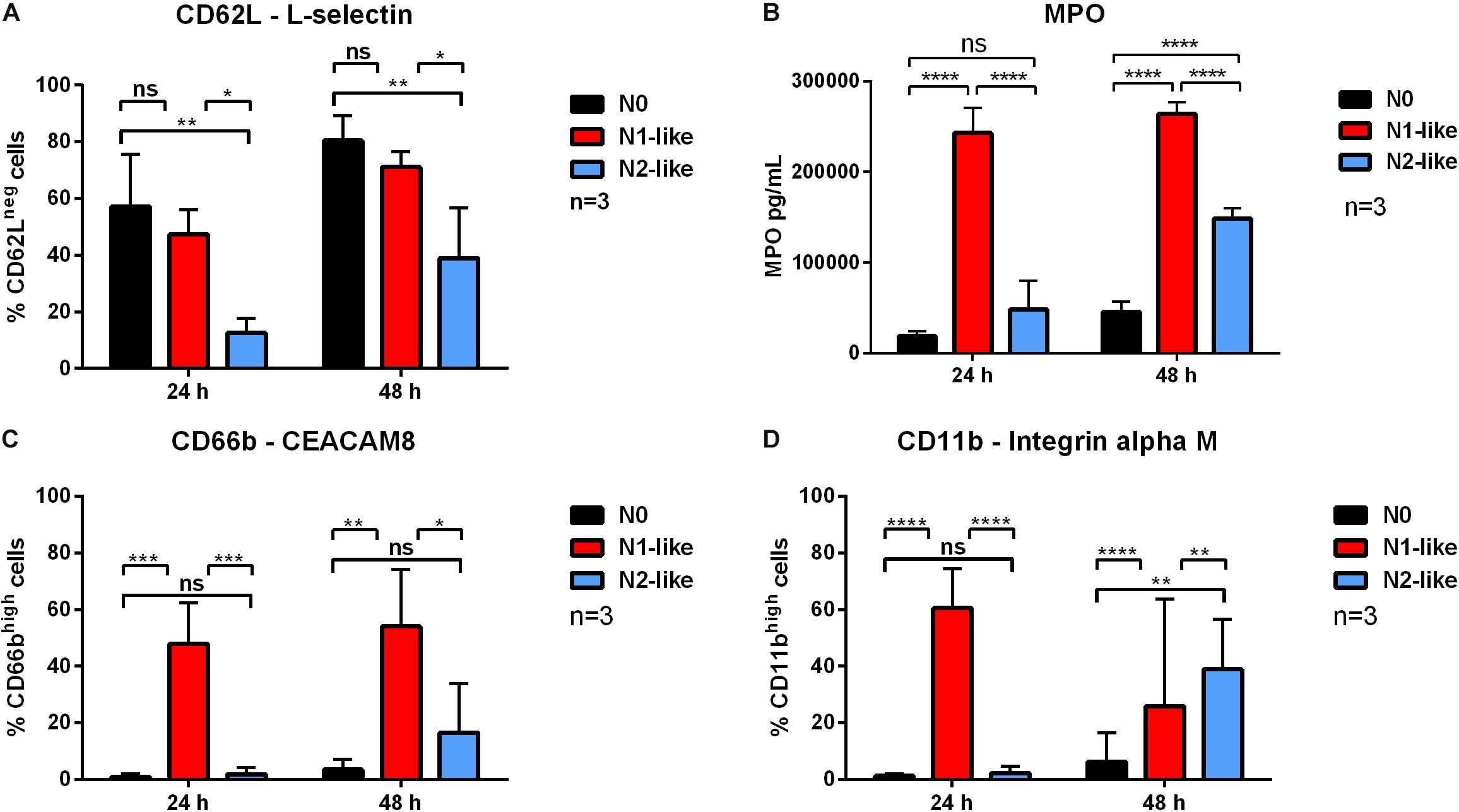
Figure 2. Cell surface expression of the activation marker CD62L and of the degranulation markers CEACAM8 (CD66b), integrin alpha M (CD11b), as well as secretion of myeloperoxidase (MPO) by in vitro polarized neutrophils. Primary human neutrophils were incubated for 24 and 48 h in the presence of an N1 or N2 polarization cocktail including the Pan-caspase inhibitor QVD-OPh. The cell surface expression of CD62L (A), CD66b (C), and CD11b (D) was assessed by using flow cytometry. For the detection of MPO (B), cell-free supernatants were collected after 24 and 48 h. The concentration of MPO was measured by ELISA. Bar diagrams show mean fluorescence intensity (MFI) ± SD (n = 3). *p < 0.05, **p < 0.01, ***p < 0.001, ****p < 0.0001, ns = not significant.
These data indicate an activated state of N1-polarized neutrophils as compared to N2-like neutrophils.
In vitro Polarized Neutrophils Show Typical N1 or N2 Cytokine/Chemokine Profiles
Next, we assessed the secretion of soluble mediators by in vitro polarized neutrophils. Available data from in vivo studies indicated an increased release of the pro-inflammatory cytokines TNF and IP-10 (5) by N1 neutrophils. On the contrary, it was shown that N2 neutrophils secrete high amounts of IL-8 (3), which is likely involved in the recruiting of additional neutrophils into the tumor. Cell-free culture supernatants of in vitro polarized neutrophils were investigated after 24 and 48 h using ELISA. TNF and IP-10 were only released by N1-like neutrophils; N0 and N2-like polarized neutrophils did not release these pro-inflammatory cytokines (Figures 3A,B). Neutrophils which were cultured with the N2 polarization cocktail for 24 h produced significantly more IL-8 than N1-polarized cells (Figure 3C).
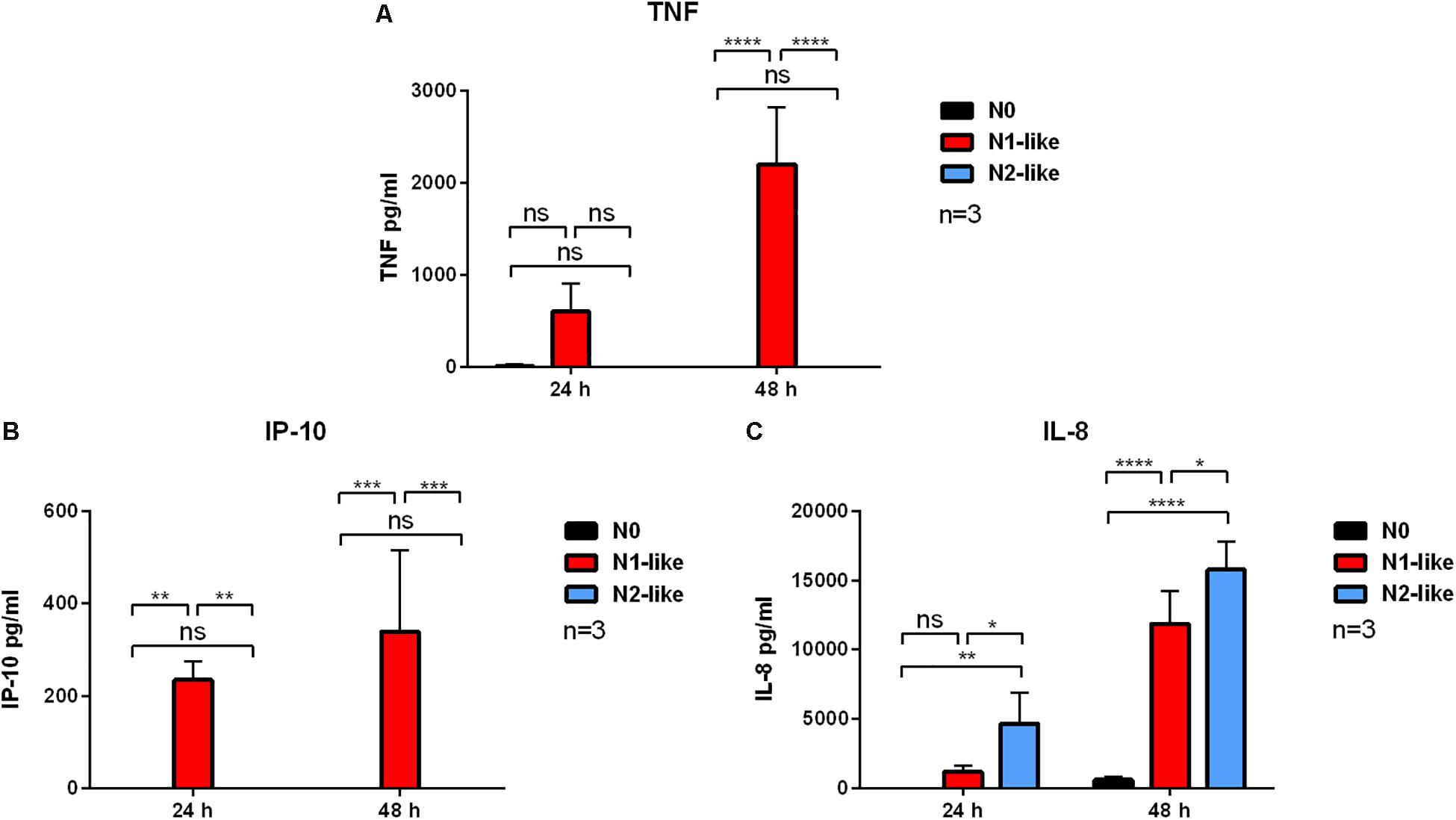
Figure 3. Secretion of tumor necrosis factor (TNF), interferon (IFN)γ-induced protein 10 (IP-10), and interleukin (IL)-8 by N1- and N2-polarized neutrophils. Primary human neutrophils were incubated in the presence of an N1 or N2 polarization cocktail including the Pan-caspase inhibitor QVD-OPh. Neutrophils which were only treated with QVD-OPh (N0) served as control. Cell-free culture supernatants were collected after 24 and 48 h. The concentrations of TNF (A), IP-10 (B), and IL-8 (C) were measured by ELISA. Bar diagrams show mean ± SD (n = 3). *p < 0.05, **p < 0.01, ***p < 0.001, ****p < 0.0001; ns = not significant.
In vitro Polarized N1-Like Neutrophils Show a Tendency to Release More Myeloperoxidase-Derived Reactive Oxygen Species but Less Superoxide Than N2-Like Neutrophils
The role of ROS in the context of TANs is under debate. Although a higher ROS production was reported as a feature of the pro-inflammatory N1 phenotype (6), ROS can have a negative effect on the antitumor defense as well. We assessed the unstimulated and PMA-induced MPO-derived ROS production and extracellular superoxide of in vitro polarized neutrophils by the luminol-based and lucigenin-based chemiluminescence assay, respectively. For both time points, 24 and 48 h, N1-polarized neutrophils showed a tendency of higher MPO-derived ROS production compared to N0 and N2-like neutrophils as depicted by the time kinetics of the assay (Figures 4A–D). However, the differences were not statistically significant (Figures 4E,F). Superoxide formation (Figure 5) was significantly increased in N1-polarized neutrophils after 24 h (Figures 5A,E). After 48 h and in the presence of PMA, N2-polarized neutrophils showed a significantly increased superoxide production compared to N0 and N1-polarized cells (Figures 5D,F).
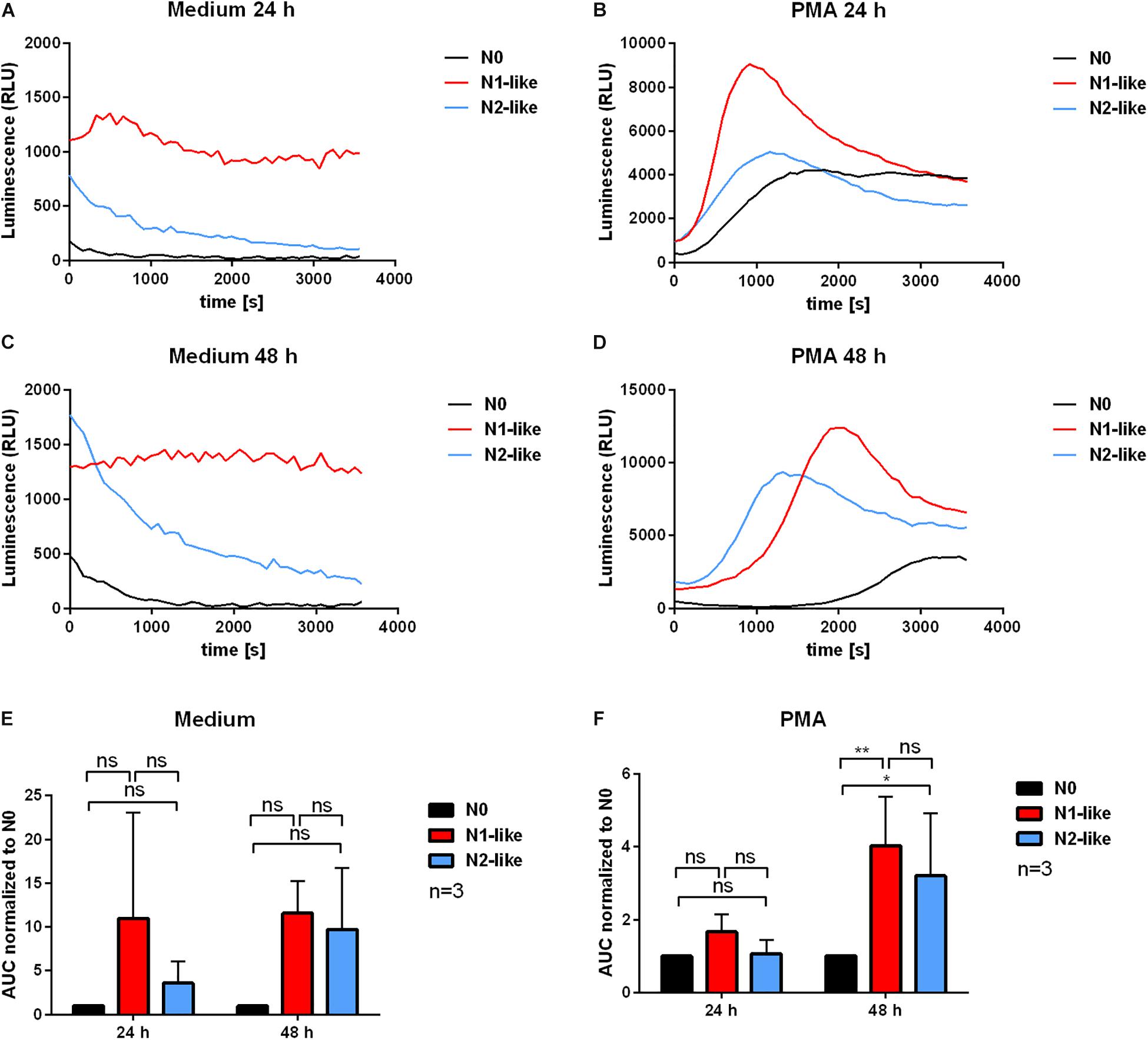
Figure 4. Myeloperoxidase (MPO)-derived reactive oxygen species (ROS) release by in vitro polarized neutrophils. Primary human neutrophils were incubated in the presence of an N1 or N2 polarization cocktail including the Pan-caspase inhibitor QVD-OPh. Neutrophils which were only treated with QVD-OPh (N0) served as control. After 24 and 48 h, the MPO-derived ROS release was measured for 1 h at 37°C in medium alone or in the presence or absence of 20 nM phorbol 12-myristate 13-acetate (PMA) by using the luminol-based chemiluminescence assay. Representative curves of luminol chemiluminescence are shown in panels (A–D). Bar diagrams of the area under the curve (AUC) values normalized to N0 (mean ± SD; n = 3) are shown in panels (E) and (F). *p < 0.05, **p < 0.01, ns = not significant.
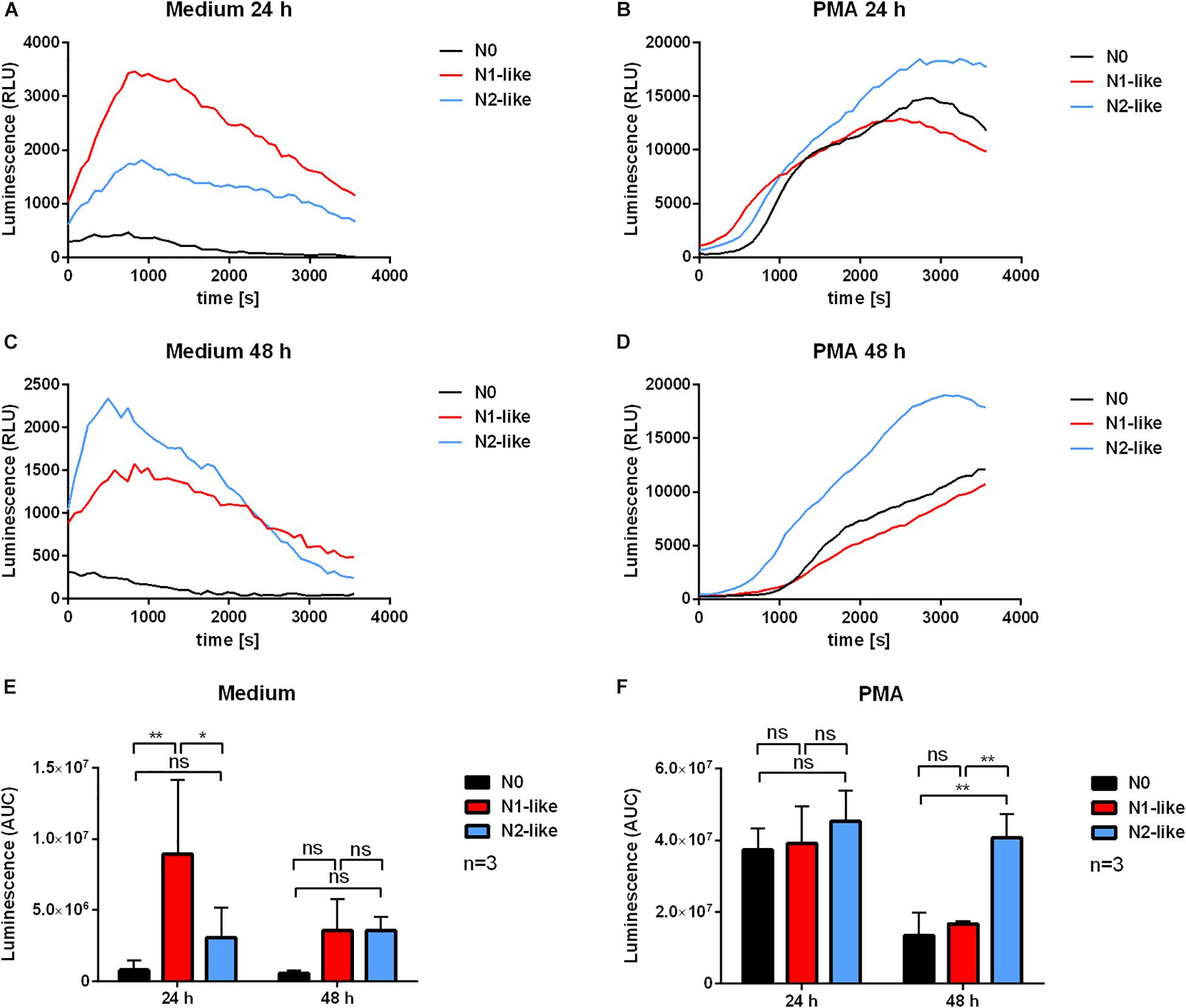
Figure 5. Superoxide release by in vitro polarized neutrophils. Primary human neutrophils were incubated in the presence of an N1 or N2 polarization cocktail including the Pan-caspase inhibitor QVD-OPh. Neutrophils which were only treated with QVD-OPh (N0) served as control. After 24 and 48 h, the reactive oxygen species (ROS) release was measured for 1 h at 37°C in medium alone or in the presence of 20 nM phorbol 12-myristate 13-acetate (PMA) by using the lucigenin-based chemiluminescence assay. Representative curves of the time kinetics of lucigenin chemiluminescence are shown in panels (A–D). Bar diagrams of the area under the curve (AUC) values normalized to N0 (mean ± SD; n = 3) are shown in panels (E) and (F). *p < 0.05, **p < 0.01, ns = not significant.
N2-Polarized Neutrophils Exert Decreased Capacity to Kill Leishmania donovani Promastigotes
To address our hypothesis that N2-polarized neutrophils are permissive for L. donovani, we assessed the anti-leishmanial capacity of in vitro polarized neutrophils by a limiting dilution assay. Primary human neutrophils were infected with L. donovani promastigotes. The infection rate was nearly 100%, with all neutrophils harboring more than one ingested parasites (Figure 6A). The infected cells were incubated in the presence of an N1 or N2 polarization cocktail for 24 and 48 h, and the parasite load was determined by using a limiting dilution (LD) culture assay. As shown in Figure 6B, N2-like neutrophils harbored significantly more viable parasites as compared to N1-like neutrophils, indicating a decreased killing ability of L. donovani promastigotes by N2-like neutrophils.
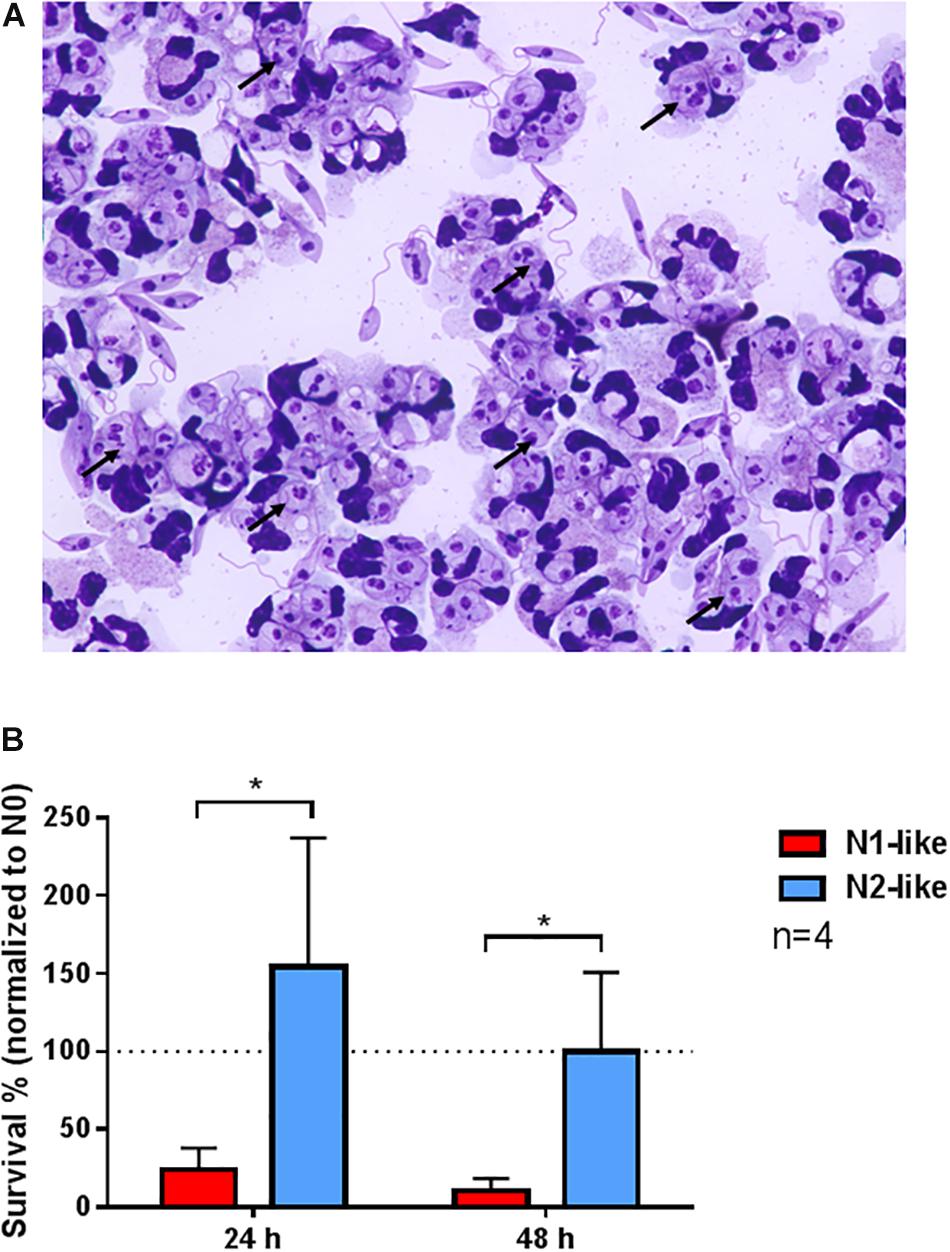
Figure 6. Enhanced survival of Leishmania donovani promastigotes in N2-polarized neutrophils in vitro. Primary human neutrophils were infected with L. donovani promastigotes (ratio 1:10) for 3 h at 37°C, 5% carbon dioxide (CO2). Successful infection was determined by Giemsa staining of cytocentrifuged samples, representative microscopy shown in (A). Arrows show representative ingested (intracellular) Leishmania. After removing the free, non-ingested parasites, the infected cells were incubated in the presence of an N1 or N2 polarization cocktail at 37°C, 5% CO2. Survival of parasites was assessed after 24 and 48 h by the limiting dilution assay (B). Bar diagrams show mean ± SD (n = 4). *p < 0.05.
Discussion
Equipped with great plasticity, neutrophils can present different phenotypes not only with regard to their stage of differentiation and age but, most importantly, also with regard to their functionality in response to their surrounding microenvironment. The important role of N1 and N2 TANs in the growth and progression of cancer has become clearly evident during the last decade. So far, our current understanding of the N1 and N2 phenotype as well as neutrophil heterogeneity is limited to animal models. Here, we present for the first time a polarization approach to generate N1 and N2 neutrophils in vitro. To study long-term polarization effects, primary human neutrophils from healthy donors were incubated up to 48 h in the presence of defined N1 and N2 polarization cocktails containing the caspase inhibitor QVD-OPh to prolong the neutrophil life span. As specific markers for N1 and N2 cells are still missing, we characterized our polarized neutrophils with regard to surface markers, cytokine/chemokine profiles, and effector functions that, based on in vivo studies, have been proposed to be characteristic for N1 and N2 neutrophils (Table 2). To our expectation, neutrophils cultured in the presence of N1 polarization cocktail were highly activated, showed a high expression of ICAM-1 and FasR on their surface, and secreted high amounts of IP-10 and TNF. IP-10 (CXCL10) is regarded as a “key driver chemokine” attracting CD8+ and CD4+ T cells to tumor sites and potentiating their function (36). In addition, IP-10 is a major chemokine recruiting natural killer (NK) cells and exerts antitumor effects also by inhibiting angiogenesis (37), making IP-10 a potent antitumor factor (38). Tumor antigen presentation by dendritic cells (DCs) to T cells in lymphoid organs is crucial for induction of antitumor immune responses. Tumor necrosis factor transgene-expressing DCs undergo augmented cellular maturation and induce more robust T-cell activation and antitumor immunity than DCs generated in recombinant TNF (39). Importantly, IP-10 and TNF were shown to act in synergy to induce antitumor effects (40).
Neutrophils treated with the N2 polarization cocktail were less activated than N1-like neutrophils and showed lower L-selectin shedding. Further, they expressed the typical N2 receptor CXCR2 to a high extent on their surface and secreted elevated amounts of IL-8. The IL-8/IL-8R axis is a signaling pathway used by tumor cells to recruit neutrophils initially to the tumor site. As N2 neutrophils are a potent source of IL-8, the entrapping influx of neutrophils into the tumor is established as a positive feedback loop (41).
As an important effector function of neutrophils, we measured ROS release of in vitro polarized cells. Our findings show a tendency of N1-like neutrophils for a higher MPO-derived ROS release than N2-like neutrophils. However, these differences were statistically not significant. Also the assessment of superoxide production produced inconsistent results being higher in N1-like cells after 24 h but being higher in PMA-treated N2-like cells after 48 h of culture. These findings may reflect the two-faced role of ROS in tumor biology. On one hand, ROS can induce oxidative damage on tumor cells (42). On the other hand, neutrophil-derived ROS may be mutagenic and contribute to genomic instability. In addition, both ROS-dependent and -independent processes for neutrophil antibody-dependent cell cytotoxicity have been suggested (43). Although neutrophil-derived ROS might damage and destroy tumor cells, they can also cause genotoxicity and act in an immunosuppressive manner by reducing the expression of the T cell receptor CD3 ζ chain (44).
In previous studies, we demonstrated that Leishmania uses neutrophils as a “Trojan horse” to enter their definite host cells, macrophages (45). Despite numerous effector mechanisms, it seems that the anti-leishmanial capacities of neutrophils are not always sufficient to eliminate the parasite. Here, we hypothesized that neutrophils are polarized during L. donovani infection toward an N2-like phenotype which is permissive for the parasite and favors the parasite’s survival. Our results showed that in vitro polarized N2-like neutrophils exert a decreased killing ability for L. donovani promastigotes compared to N1-like neutrophils which eliminated the majority of parasites. As neutrophils are the first innate immune cells at the site of infection, it would be of advantage for the parasite to polarize the neutrophils toward a favorable phenotype. Although our results suggest that the presence of pro- and anti-inflammatory neutrophil phenotypes can modulate the outcome of VL, they must be interpreted with caution as our polarization cocktail is based on conditions present in the tumor microenvironment. However, several factors that are characteristic for the tumor microenvironment such as hypoxia (46, 47) or the cytokines IL-10 (48, 49), TGF-β (50, 51), and the lipid mediator PGE2 (48, 49) are also found in tissues infected with Leishmania.
Our study revealed that it is feasible to polarize mature human neutrophils in vitro to distinct phenotypes. We could show functional and phenotypical differences between neutrophils cultured in the presence of N1- or N2-polarizing cocktails. Our findings indicate that even fully differentiated mature neutrophils maintain their plasticity and can acquire typical phenotypic characteristics observed so far only in the tumor microenvironment. Our novel in vitro model for the polarization of N1- and N2-like neutrophils represents a first step, allowing the study of pro- and anti-inflammatory neutrophils. Investigation of in vitro polarized pro- and anti-inflammatory neutrophil populations could be a major advantage for the identification of biomarkers for tumor-promoting neutrophils and could contribute to the development of therapeutic strategies to target disease-promoting neutrophils.
Data Availability Statement
The raw data supporting the conclusion of this article will be made available by the authors, without undue reservation, to any qualified researcher.
Ethics Statement
The studies involving human participants were reviewed and approved by the Ethical Committee of the University of Lübeck. The patients/participants provided their written informed consent to participate in this study.
Author Contributions
MO designed the study, carried out the experiments, and wrote the manuscript. SM carried out the experiments. TL designed the study and wrote the manuscript.
Funding
This work was carried out in frame of the Infect-ERA “INLEISH” project and was financially funded by the Bundesministerium für Bildung und Forschung (BMBF).
Conflict of Interest
The authors declare that the research was conducted in the absence of any commercial or financial relationships that could be construed as a potential conflict of interest.
Acknowledgments
The authors thank Ms. Heidi Tackenberg and Mr. Daniel Dömer, and the members of the INLEISH consortium (Dres. Ricardo Silvestre, Braga, Portugal; Bhaskar Saha, Pune, India; Arup Sarkar, Bhubaneswar, India) for constructive discussions.
Supplementary Material
The Supplementary Material for this article can be found online at: https://www.frontiersin.org/articles/10.3389/fimmu.2020.00532/full#supplementary-material
Abbreviations
ANCA, anti-neutrophil cytoplasmic antibodies; ANOVA, analysis of variance; APC, allophycocyanin; AUC, area under the curve; ELISA, enzyme-linked immunosorbent assay; FACS, fluorescence-activated cell sorting; FCS, fetal calf serum; FITC, fluorescein isothiocyanate; G-CSF, granulocyte-colony stimulating factor; HIV, human immunodeficiency virus; ICAM-1, intercellular adhesion molecule 1; IFN, interferon; IgG, immunglobulin G; IgM, immunglobulin M; IL, interleukin; LPS, lipopolysaccharide; mAb, monoclonal antibody; MMP, matrix metalloproteinase; NETs, neutrophil extracellular traps; PE, phycoerythrin; PMA, phorbol-12-myristate-13-acetate; PMN, polymorphonuclear leukocytes; ROS, reactive oxygen species; SLE, systemic lupus erythematosus; TAMs, tumor-associated macrophages; TANs, tumor-associated neutrophils; TGF- β, transforming growth factor beta; TNF, tumor necrosis factor; VEGF, vascular endothelial growth factor; VL, visceral leishmaniasis.
References
1. Rosales C. Neutrophil: a cell with many roles in inflammation or several cell types? Front Physiol. (2018) 9:113. doi: 10.3389/fphys.2018.00113
2. Treffers LW, Hiemstra IH, Kuijpers TW, van den Berg TK, Matlung HL. Neutrophils in cancer. Immunol Rev. (2016) 273:312–28. doi: 10.1111/imr.12444
3. Fridlender ZG, Albelda SM. Tumor-associated neutrophils: friend or foe? Carcinogenesis. (2012) 33:949–55. doi: 10.1093/carcin/bgs123
4. Sharma B, Nawandar DM, Nannuru KC, Varney ML, Singh RK. Targeting CXCR2 enhances chemotherapeutic response, inhibits mammary tumor growth, angiogenesis, and lung metastasis. Mol Cancer Ther. (2013) 12:799–808. doi: 10.1158/1535-7163.MCT-12-0529
5. Fridlender ZG, Sun J, Kim S, Kapoor V, Cheng G, Ling L, et al. Polarization of tumor-associated neutrophil phenotype by TGF-beta: “N1” versus “N2”, TAN. Cancer Cell. (2009) 16:183–94. doi: 10.1016/j.ccr.2009.06.017
6. Mizuno R, Kawada K, Itatani Y, Ogawa R, Kiyasu Y, Sakai Y. The role of tumor-associated neutrophils in colorectal cancer. IJMS. (2019) 20:529. doi: 10.3390/ijms20030529
7. Coffelt SB, Wellenstein MD, de Visser KE. Neutrophils in cancer: neutral no more. Nat Rev Cancer. (2016) 16:431–46. doi: 10.1038/nrc.2016.52
8. Grecian R, Whyte MKB, Walmsley SR. The role of neutrophils in cancer. Br Med Bull. (2018) 128:5–14. doi: 10.1093/bmb/ldy029
9. Andzinski L, Wu C-F, Lienenklaus S, Kröger A, Weiss S, Jablonska J. Delayed apoptosis of tumor associated neutrophils in the absence of endogenous IFN-β. Int J Cancer. (2015) 136:572–83. doi: 10.1002/ijc.28957
10. Shaul ME, Levy L, Sun J, Mishalian I, Singhal S, Kapoor V, et al. Tumor-associated neutrophils display a distinct N1 profile following TGFβ modulation: a transcriptomics analysis of pro- vs. antitumor TANs. Oncoimmunology. (2016) 5:e1232221. doi: 10.1080/2162402X.2016.1232221
11. Casbon A-J, Reynaud D, Park C, Khuc E, Gan DD, Schepers K, et al. Invasive breast cancer reprograms early myeloid differentiation in the bone marrow to generate immunosuppressive neutrophils. Proc Natl Acad Sci USA. (2015) 112:E566–75. doi: 10.1073/pnas.1424927112
12. Andzinski L, Kasnitz N, Stahnke S, Wu C-F, Gereke M, von Köckritz-Blickwede M, et al. Type IIFNs induce anti-tumor polarization of tumor associated neutrophils in mice and human: TANs in tumorigenesis. Int J Cancer. (2016) 138:1982–93. doi: 10.1002/ijc.29945
13. Ma Y, Yabluchanskiy A, Iyer RP, Cannon PL, Flynn ER, Jung M, et al. Temporal neutrophil polarization following myocardial infarction. Cardiovasc Res. (2016) 110:51–61. doi: 10.1093/cvr/cvw024
14. Hacbarth E, Kajdacsy-Balla A. Low density neutrophils in patients with systemic lupus erythematosus, rheumatoid arthritis, and acute rheumatic fever. Arthritis Rheum. (1986) 29:1334–42. doi: 10.1002/art.1780291105
15. Morisaki T, Goya T, Ishimitsu T, Torisu M. The increase of low density subpopulations and CD10 (CALLA) negative neutrophils in severely infected patients. Surg Today. (1992) 22:322–7. doi: 10.1007/BF00308740
16. Lin AM, Rubin CJ, Khandpur R, Wang JY, Riblett M, Yalavarthi S, et al. Mast cells and neutrophils release IL-17 through extracellular trap formation in psoriasis. J Immunol. (2011) 187:490–500. doi: 10.4049/jimmunol.1100123
17. Cloke T, Munder M, Taylor G, Müller I, Kropf P. Characterization of a novel population of low-density granulocytes associated with disease severity in HIV-1 infection. PLoS One. (2012) 7:e48939. doi: 10.1371/journal.pone.0048939
18. Fu J, Tobin MC, Thomas LL. Neutrophil-like low-density granulocytes are elevated in patients with moderate to severe persistent asthma. Ann Allergy Asthma Immunol. (2014) 113:635–40.e2. doi: 10.1016/j.anai.2014.08.024
19. Grayson PC, Carmona-Rivera C, Xu L, Lim N, Gao Z, Asare AL, et al. Neutrophil-related gene expression and low-density granulocytes associated with disease activity and response to treatment in antineutrophil cytoplasmic antibody-associated vasculitis. Arthritis Rheumatol. (2015) 67:1922–32. doi: 10.1002/art.39153
20. Rocha BC, Marques PE, Leoratti FMS, Junqueira C, Pereira DB, Antonelli LRDV, et al. Type I interferon transcriptional signature in neutrophils and low-density granulocytes are associated with tissue damage in malaria. Cell Rep. (2015) 13:2829–41. doi: 10.1016/j.celrep.2015.11.055
21. Tosello Boari J, Amezcua Vesely MC, Bermejo DA, Ramello MC, Montes CL, Cejas H, et al. IL-17RA signaling reduces inflammation and mortality during trypanosoma cruzi infection by recruiting suppressive IL-10-producing neutrophils. PLoS Pathog. (2012) 8:e1002658. doi: 10.1371/journal.ppat.1002658
22. Guimarães-Costa AB, Nascimento MTC, Froment GS, Soares RPP, Morgado FN, Conceição-Silva F, et al. Leishmania amazonensis promastigotes induce and are killed by neutrophil extracellular traps. Proc Natl Acad Sci USA. (2009) 106:6748–53. doi: 10.1073/pnas.0900226106
23. Carlsen ED, Hay C, Henard CA, Popov V, Garg NJ, Soong L. Leishmania amazonensis amastigotes trigger neutrophil activation but resist neutrophil microbicidal mechanisms. Infect Immun. (2013) 81:3966–74. doi: 10.1128/IAI.00770-13
24. Ritter U, Frischknecht F, van Zandbergen G. Are neutrophils important host cells for Leishmania parasites? Trends Parasitol. (2009) 25:505–10. doi: 10.1016/j.pt.2009.08.003
25. van Zandbergen G, Klinger M, Mueller A, Dannenberg S, Gebert A, Solbach W, et al. Cutting edge: neutrophil granulocyte serves as a vector for Leishmania entry into macrophages. J Immunol. (2004) 173:6521–5. doi: 10.4049/jimmunol.173.11.6521
26. Singh SS, Sharma S, Sundar S, Nylén S, Davis RE, Kumar R, et al. The phenotype of circulating neutrophils during visceral leishmaniasis. Am J Trop Med Hyg. (2017) 97:767–70. doi: 10.4269/ajtmh.16-0722
27. Aga E, Katschinski DM, van Zandbergen G, Laufs H, Hansen B, Müller K, et al. Inhibition of the spontaneous apoptosis of neutrophil granulocytes by the intracellular parasite Leishmania major. J Immunol. (2002) 169:898–905. doi: 10.4049/jimmunol.169.2.898
28. Stevens P, Hong D. The role of myeloperoxidase and superoxide anion in the luminol- and lucigenin-dependent chemiluminescence of human neutrophils. Microchem J. (1984) 30:135–46.
29. Laskay T, Wittmann I, Diefenbach A, Röllinghoff M, Solbach W. Control of Leishmania major infection in BALB/c mice by inhibition of early lymphocyte entry into peripheral lymph nodes. J Immunol. (1997) 158:1246–53.
30. Mantovani A. The yin-yang of tumor-associated neutrophils. Cancer Cell. (2009) 16:173–4. doi: 10.1016/j.ccr.2009.08.014
31. Mantovani A, Allavena P, Sica A, Balkwill F. Cancer-related inflammation. Nature. (2008) 454:436–44. doi: 10.1038/nature07205
32. Gomes NE, Brunialti MKC, Mendes ME, Freudenberg M, Galanos C, Salomão R. Lipopolysaccharide-induced expression of cell surface receptors and cell activation of neutrophils and monocytes in whole human blood. Braz J Med Biol Res. (2010) 43:853–8. doi: 10.1590/S0100-879X2010007500078
33. Ellis TN, Beaman BL. Interferon-gamma activation of polymorphonuclear neutrophil function. Immunology. (2004) 112:2–12. doi: 10.1111/j.1365-2567.2004.01849.x
34. Caserta TM, Smith AN, Gultice AD, Reedy MA, Brown TL. Q-VD- OPh, a broad spectrum caspase inhibitor with potent antiapoptotic properties. Apoptosis. (2003) 8:345–52.
35. Ley K, Gaehtgens P, Fennie C, Singer MS, Lasky LA, Rosen SD. Lectin-like cell adhesion molecule 1 mediates leukocyte rolling in mesenteric venules in vivo. Blood. (1991) 77:2553–5.
36. Karin N, Razon H. Chemokines beyond chemo-attraction: CXCL10 and its significant role in cancer and autoimmunity. Cytokine. (2018) 109:24–8. doi: 10.1016/j.cyto.2018.02.012
37. Strieter RM, Kunkel SL, Arenberg DA, Burdick MD, Polverini PJ. Interferon gamma-inducible protein 10 (IP-10), a member of the C-X-C chemokine family, is an inhibitor of angiogenesis. Biochem Biophys Res Commun. (1995) 210:51–7. doi: 10.1006/bbrc.1995.1626
38. Giese NA, Raykov Z, DeMartino L, Vecchi A, Sozzani S, Dinsart C, et al. Suppression of metastatic hemangiosarcoma by a parvovirus MVMp vector transducing the IP-10 chemokine into immunocompetent mice. Cancer Gene Ther. (2002) 9:432–42. doi: 10.1038/sj.cgt.7700457
39. Zhang W, Chen Z, Li F, Kamencic H, Juurlink B, Gordon JR, et al. Tumour necrosis factor-alpha (TNF-alpha) transgene-expressing dendritic cells (DCs) undergo augmented cellular maturation and induce more robust T-cell activation and anti-tumour immunity than DCs generated in recombinant TNF-alpha. Immunology. (2003) 108:177–88.
40. Enderlin M, Kleinmann EV, Struyf S, Buracchi C, Vecchi A, Kinscherf R, et al. TNF-alpha and the IFN-gamma-inducible protein 10 (IP- 10/CXCL-10) delivered by parvoviral vectors act in synergy to induce antitumor effects in mouse glioblastoma. Cancer Gene Ther. (2009) 16:149–60. doi: 10.1038/cgt.2008.62
41. David JM, Dominguez C, Hamilton DH, Palena C. The IL-8/IL-8R axis: a double agent in tumor immune resistance. Vaccines (Basel). (2016) 4:22. doi: 10.3390/vaccines4030022
42. Zivkovic M, Poljak-Blazi M, Zarkovic K, Mihaljevic D, Schaur RJ, Zarkovic N. Oxidative burst of neutrophils against melanoma B16-F10. Cancer Lett. (2007) 246:100–8. doi: 10.1016/j.canlet.2006.02.002
43. Dallegri F, Patrone F, Frumento G, Sacchetti C. Antibody-dependent killing of tumor cells by polymorphonuclear leukocytes. involvement of oxidative and nonoxidative mechanisms23. J Natl Cancer Inst. (1984) 73:331–9. doi: 10.1093/jnci/73.2.331
44. Schmielau J, Finn OJ. Activated granulocytes and granulocyte-derived hydrogen peroxide are the underlying mechanism of suppression of t-cell function in advanced cancer patients. Cancer Res. (2001) 61:4756–60.
45. Laskay T, van Zandbergen G, Solbach W. Neutrophil granulocytes – Trojan horses for Leishmania major and other intracellular microbes? Trends Microbiol. (2003) 11:210–4. doi: 10.1016/S0966-842X(03)00075-1
46. Mahnke A, Meier RJ, Schatz V, Hofmann J, Castiglione K, Schleicher U, et al. Hypoxia in Leishmania major skin lesions impairs the NO-dependent leishmanicidal activity of macrophages. J. Invest. Dermatol. (2014) 134:2339–46. doi: 10.1038/jid.2014.121
47. Singh AK, Mukhopadhyay C, Biswas S, Singh VK, Mukhopadhyay CK. Intracellular pathogen Leishmania donovani activates hypoxia inducible factor-1 by dual mechanism for survival advantage within macrophage. PLoS One. (2012) 7:e38489. doi: 10.1371/journal.pone.0038489
48. Kane MM, Mosser DM. The role of IL-10 in promoting disease progression in leishmaniasis. J Immunol. (2001) 166:1141–7. doi: 10.4049/jimmunol.166.2.1141
49. Noben-Trauth N, Lira R, Nagase H, Paul WE, Sacks DL. The relative contribution of IL-4 receptor signaling and IL-10 to susceptibility to Leishmania major. J Immunol. (2003) 170:5152–8. doi: 10.4049/jimmunol.170.10.5152
50. Anderson CF, Lira R, Kamhawi S, Belkaid Y, Wynn TA, Sacks D. IL-10 and TGF-β control the establishment of persistent and transmissible infections produced by Leishmania tropica in C57BL/6 Mice. J Immunol. (2008) 180:4090–7. doi: 10.4049/jimmunol.180.6.4090
51. Wilson ME, Young BM, Davidson BL, Mente KA, McGowan SE. The importance of TGF-beta in murine visceral leishmaniasis. J Immunol. (1998) 161:6148–55.
52. Gerlini G, Tun-Kyi A, Dudli C, Burg G, Pimpinelli N, Nestle FO. Metastatic melanoma secreted IL-10 down-regulates CD1 molecules on dendritic cells in metastatic tumor lesions. Am J Pathol. (2004) 165:1853–63.
53. Gholamin M, Moaven O, Memar B, Farshchian M, Naseh H, Malekzadeh R, et al. Overexpression and interactions of interleukin-10, transforming growth factor β, and vascular endothelial growth factor in esophageal squamous cell carcinoma. World J Surg. (2009) 33:1439–45. doi: 10.1007/s00268-009-0070-y
54. Brown JR, DuBois RN. COX-2: a molecular target for colorectal cancer prevention. JCO. (2005) 23:2840–55. doi: 10.1200/JCO.2005.09.051
55. Greenhough A, Smartt HJM, Moore AE, Roberts HR, Williams AC, Paraskeva C, et al. The COX-2/PGE2 pathway: key roles in the hallmarks of cancer and adaptation to the tumour microenvironment. Carcinogenesis. (2009) 30:377–86. doi: 10.1093/carcin/bgp014
56. Chakraborty A, Guha S. Granulocyte colony-stimulating factor/granulocyte colony-stimulating factor receptor biological axis promotes survival and growth of bladder cancer cells. Urology. (2007) 69:1210–5. doi: 10.1016/j.urology.2007.02.035
57. Joshita S, Nakazawa K, Sugiyama Y, Kamijo A, Matsubayashi K, Miyabayashi H, et al. Granulocyte-colony stimulating factor-producing pancreatic adenosquamous carcinoma showing aggressive clinical course. Intern Med. (2009) 48:687–91. doi: 10.2169/internalmedicine.48.1900
58. Kyo S, Kanaya T, Takakura M, Inoue MA. Case of cervical cancer with aggressive tumor growth: possible autocrine growth stimulation by G-CSF and Il-6. Gynecol Oncol. (2000) 78:383–7. doi: 10.1006/gyno.2000.5904
59. Romero-Garcia S, Moreno-Altamirano MMB, Prado-Garcia H, Sánchez-García FJ. Lactate contribution to the tumor microenvironment: mechanisms, effects on immune cells and therapeutic relevance. Front Immunol. (2016) 7:52. doi: 10.3389/fimmu.2016.00052
60. Leone RD, Emens LA. Targeting adenosine for cancer immunotherapy. J Immunother Cancer. (2018) 6:57. doi: 10.1186/s40425-018-0360-8
61. Chao T, Furth EE, Vonderheide RH. CXCR2-dependent accumulation of tumor-associated neutrophils regulates T-cell immunity in pancreatic ductal adenocarcinoma. Cancer Immunol Res. (2016) 4:968–82. doi: 10.1158/2326-6066.CIR-16-0188
62. Nywening TM, Belt BA, Cullinan DR, Panni RZ, Han BJ, Sanford DE, et al. Targeting both tumour-associated CXCR2 + neutrophils and CCR2 + macrophages disrupts myeloid recruitment and improves chemotherapeutic responses in pancreatic ductal adenocarcinoma. Gut. (2018) 67:1112–23. doi: 10.1136/gutjnl-2017-313738
Keywords: neutrophils, tumor-associated neutrophils, N1, N2, polarization, neutrophil heterogeneity, Leishmania donovani, visceral leishmaniasis
Citation: Ohms M, Möller S and Laskay T (2020) An Attempt to Polarize Human Neutrophils Toward N1 and N2 Phenotypes in vitro. Front. Immunol. 11:532. doi: 10.3389/fimmu.2020.00532
Received: 06 December 2019; Accepted: 09 March 2020;
Published: 28 April 2020.
Edited by:
Martin Herrmann, University Hospital Erlangen, GermanyReviewed by:
Payel Sil, National Institute of Environmental Health Sciences (NIEHS), United StatesMihaela Gadjeva, Harvard Medical School, United States
Copyright © 2020 Ohms, Möller and Laskay. This is an open-access article distributed under the terms of the Creative Commons Attribution License (CC BY). The use, distribution or reproduction in other forums is permitted, provided the original author(s) and the copyright owner(s) are credited and that the original publication in this journal is cited, in accordance with accepted academic practice. No use, distribution or reproduction is permitted which does not comply with these terms.
*Correspondence: Tamás Laskay, dGFtYXMubGFza2F5QHVrc2guZGU=