- 1Department of Biomolecular Innovation, Institute for Biomedical Sciences, Shinshu University, Nagano, Japan
- 2Graduate School of Integrated Sciences for Life, Hiroshima University, Higashi-Hiroshima, Japan
- 3Department of Biomedical Engineering, Graduate School of Science and Technology, Shinshu University, Nagano, Japan
The bacterium Flavonifractor plautii (FP), which is found in human feces, has been reported to participate in catechin metabolism in the gut, but this bacterium's effects on immune function are unclear. We assessed the effect of oral administration of FP on the immune response in ovalbumin (OVA) -sensitized mice. We demonstrated that the FP treatment suppressed interleukin (IL)-4 in splenocytes and OVA-specific IgE production in serum from OVA-sensitized mice. Moreover, oral administration of FP augmented CD4+CD25+ T cells and CD103+CD11c+ DCs. In animals of the FP group, the proportion of FP was increased in the mesenteric lymph nodes (MLNs), as was the proportion of Deferribacteres in the cecum. Oral administration of FP may inhibit the Th2 immune response by incorporation into the MLNs and/or by inducing changes in the gut microbiota. Thus, FP may be useful in alleviating antigen-induced Th2 immune responses.
Introduction
In recent years, the number of individuals suffering from allergic diseases has been rising globally, which has developed into an increasingly serious issue for societies worldwide (1, 2). Allergic diseases are thought to be caused by a combination of genetic, lifestyle-related, and environmental factors. Both the activation of T-helper-2 (Th2) cell immune responses and the immune regulation of regulatory T cells (Tregs) have been implicated in the pathogenesis of allergic reactions (3–5). An allergy is an excessive immune response to a specific environmental antigen that normally is tolerated by the general population; the term also has become synonymous with hypersensitivity (6). Th2 cells are thought to play a key role in the onset of allergic reactions, given that this class of cells produces the pro-inflammatory cytokines interleukin (IL)-4, IL-5, and IL-13, and induces the differentiation of B cells into plasma cells. In response to stimulation by these cytokines, particularly IL-4, B cells undergo a class switch and differentiate into plasma cells, producing high-affinity IgE antibodies (7).
It has been reported that allergic symptoms can be alleviated by the intake of certain foods, components thereof, or probiotics such as tea (extracts of Camellia sinensis L.), catechin, lactic acid bacteria, and bifidobacteria (8, 9). In particularly, tea catechins, a group of polyphenolic compounds like epigallocatechin-3-O-(3-O-methyl) gallate (EGCG3”Me) and epigallocatechin-3-O-(4-O-methyl) gallate (EGCG4”Me), exert strong anti-allergic effects (10–13). Catechins constitute 30–42% of the dry weight of green tea. Major catechins are (+)-epigallocatechin gallate (EGCG), (-)-epicatechin gallate (ECG), and (-)-epicatechin (EC) (14). The majority of tea catechins are biotransformed by gut microbiota and then absorbed into the blood stream or excreted in the feces (15). The reciprocal relationship between polyphenols and gut microbiota contributes to host health benefits. However, it is unclear whether catechin-metabolizing bacteria have anti-allergic effects.
The body surface, intraoral region, gastrointestinal tract, and skin are colonized by huge numbers of different bacteria. The gut microbiota is the most abundant group, constituting more than 90% of commensal bacteria in the whole body (16). Recently, the host immune regulatory function has been shown to be regulated by the gut microbiome (17, 18). Moreover, the gut microbiota has been reported to be involved in systemic disease. For instance, the composition of the gut microbiota has been shown to be altered in infants with an allergic disease compared with that in healthy infants (19). Feehley et al. also reported that fecal transplantation from an infant with a milk allergy into germ-free mice induced anaphylactic reactions to milk in the mice (20). These reports suggest that the gut microbiota can regulate allergic immune responses. The composition of the gut microbiota also is known to be changed by factors such as the environment, lifestyle, exercise, and stress (21).
In the present study, we focused on one constituent of the gut microbiota, Flavonifractor plautii (FP). FP is a gram-positive anaerobic bacterium of the genus Clostridium. FP has been isolated from human feces and shown to metabolize catechins (15, 22). Atarashi et al. also reported that a mix of 17 bacterial species including FP induced the activation of Tregs, resulting in alleviation of pathology in mice with colitis and allergic disease (23). In the present study, we evaluated the anti-Th2 immune response associated with FP.
Materials and Methods
Reagents
Cell culture reagents and supplies were purchased from Thermo Fisher Scientific, Inc. (Waltham, MA, USA, RRID:SCR_008452). All other chemicals were purchased from Nacalai Tesque (Kyoto, Japan, RRID:SCR_013519). Antibody reagents were purchased from BioLegend (San Diego, CA, USA, RRID:SCR_001134) and Sigma-Aldrich (St. Louis, MO, USA, RRID:SCR_008988).
Culture Conditions for FP
FP was obtained from the American Type Culture Collection (Flavonifractor plautii ATCC ®29863™, Rockville, MD, USA, RRID:SCR_001672). The strain was cultured in GAM broth (Nissui Pharmaceutical Co., Ltd., Tokyo, Japan) according to the medium manufacturer's protocol. The bacterial cells were pelleted by centrifugation at 8,000 × g, 4°C, for 5 min and then washed with and re-suspended in sterile water to yield a suspension at a density of 1 × 1011 colony-forming units [cfu] per mL. The resulting suspension was lyophilized and the bacterial cells were stored at −80°C until used for the experiments.
Ovalbumin-Sensitized Mice
Female BALB/c mice (RRID:IMSR_APB:8492) (4 weeks of age) were purchased from Japan SLC (Hamamatsu, Japan), housed under temperature- and light-controlled conditions, and provided with ad libitum access to a standard diet (MF; Oriental Yeast Co. Ltd., Tokyo, Japan) and sterile water for 1 week. At 6 and 7 weeks of age, mice were sensitized by intraperitoneal (i.p.) injection with 50 μg of albumin from chicken egg white (ovalbumin, OVA, grade V; Sigma); aluminum hydroxide gel was used as an adjuvant at an allergen:adjuvant ratio of 1:50. From 8 weeks of age, OVA-sensitized mice were used for cell culture experiment (Figure 1A).
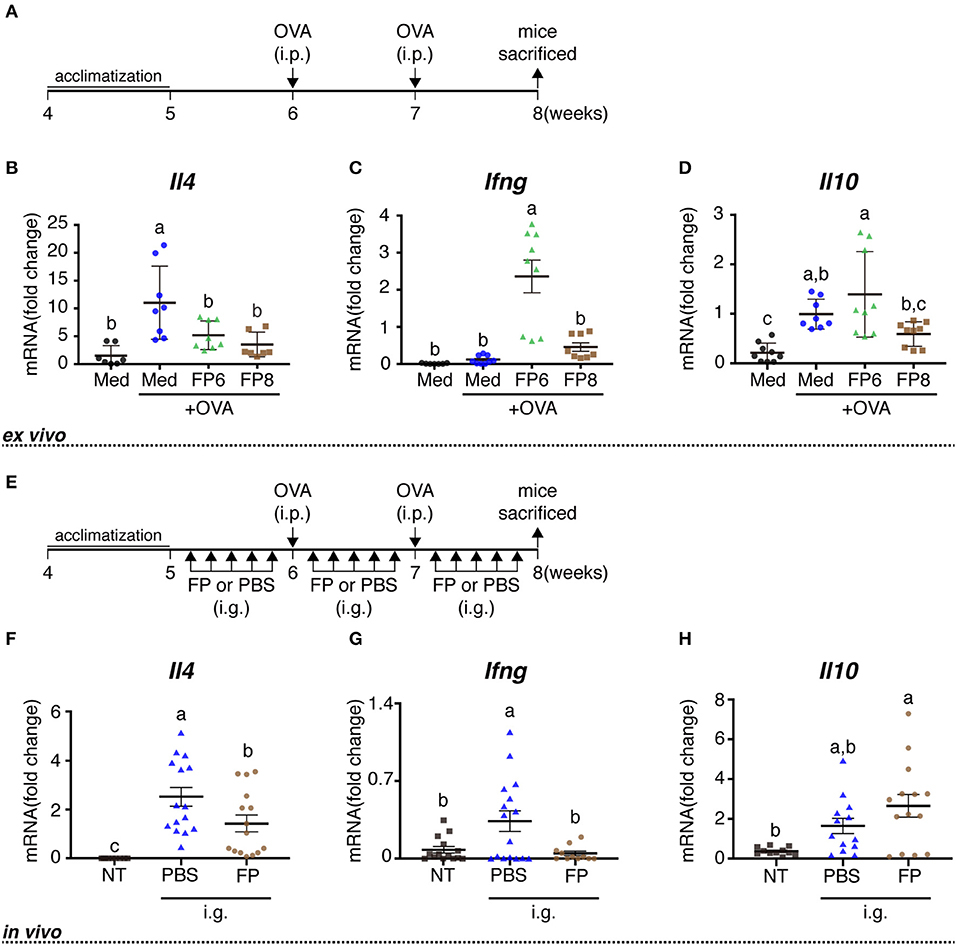
Figure 1. Immune effects of FP treatment or oral administration on OVA-sensitized mice. Schedule for experiments examining the immune activity of FP treatment ex vivo (A) and orally administered FP in vivo (E). Splenocytes from OVA-sensitized mice were treated with Med (Medium), OVA (1 mg/mL ovalbumin), FP6 (1 mg/mL OVA + FP 1x106 cfu/well), or FP8 (1 mg/mL OVA + FP 1 × 108 cfu/well) for 24 h (A,B). The expression of Il4 (B,F), Ifng (C,G), and Il10 (D,H) were measured by qPCR. Data are expressed as mean ± SD and pooled from three or four independent experiments (n = 7–9/group, A-D; n = 8–16/group, E–H); expression was normalized to that of the housekeeping gene encoding β-actin. No detected data were excluded. Different letters (i.e., a, b, c) indicate significant differences among groups (p < 0.05).
Splenocyte Cultures
Splenocytes from OVA-sensitized female BALB/c mice (8 weeks of age) were prepared as described previously (24). Splenocytes were seeded onto 24-well plates (Nalge Nunc International K.K., Tokyo, Japan) at a density of 2 × 106 cells/well in complete Roswell Park Memorial Institute (RPMI) 1640 medium (Sigma-Aldrich) supplemented with 10% fetal bovine serum, 1% non-essential amino acids, and antibiotics (100 U/mL penicillin, 100 mg/mL streptomycin), 25 mM HEPES, 1.0 mM sodium pyruvate, and 0.0035% 2-mercaptoethanol. Splenocytes then were cultured with FP (FP6: 1 × 106 cfu; FP8: 1 × 108 cfu) for 24 h, followed by stimulation with 1 mg/mL OVA at 37°C for 72 h.
Oral Administration of FP into OVA-Sensitized Mice
The OVA-sensitized mice were randomly allocated into three groups: OVA-non-sensitized mice (NT group), OVA-sensitized mice orally administered with phosphate-buffered saline (PBS) (PBS group), and OVA-sensitized mice orally administered with FP (FP group). Mice in the FP group received FP (1 × 106 cfu/mouse) by oral gavage once daily, five times per week, for 3 weeks from 5 to 7 weeks of age (Figure 1E). In contrast, mice in the PBS group received PBS (200 μL/mouse) by oral gavage once daily, five times per week, for 3 weeks (Figure 1E). At 8 weeks of age, mice were euthanized, and then splenocytes, mesenteric lymph nodes (MLNs), and serum from each treated mouse were used for analysis.
Ex vivo Culture of Splenocytes or MLNs
Splenocytes and MLNs from OVA-sensitized female mice (8 weeks of age) were prepared as described previously (24, 25). Cells (2 × 106 cells/well) were suspended in the complete RPMI1640 medium containing 1 mg/mL OVA and incubated at 37°C for 72 h in 24-well plates. Following the incubation, cells and culture supernatants were harvested by centrifugation. Cells were subjected to flow cytometric and gene expression analysis.
T Cell-DC Co-culture Assay
CD11c+ dendritic cells (DCs) were prepared from splenocytes and MLNs using the MACS cell sorting system (Miltenyi Biotec, Auburn, CA, RRID:SCR_008984). DCs (2 × 104~2 × 105 cells/well) were suspended in complete RPMI1640 medium and were incubated at 37°C for 24 h in 96-well plates. The resulting cells were subjected to flow cytometric analysis. Naïve CD4+ T cells and CD11c+ DCs were prepared from MLNs using the MACS cell sorting system. Co-culture analysis was performed as described previously (26). Briefly, T cells and DCs were mixed at a ratio of 6:1 in complete RPMI1640 medium and incubated at 37°C for 96 h in 96-well plates. The resulting cells were subjected to flow cytometric analysis.
Flow Cytometric Analysis
For determination of intracellular levels of IL-4, IL-10, and IFN-γ, cells were incubated at 37°C for 4 h in complete RPMI1640 medium containing 20 ng/mL phorbol-12-myristate-13-acetate, 1 μg/mL ionomycin, and 3 μg/mL brefeldin A. The stimulated cells then were fixed in 4% paraformaldehyde for 15 min at room temperature. Fixed cells were permeabilized in PBS-BSA [PBS containing 1% bovine serum albumin (BSA)] and 0.5% Triton-X100, along with one of the following fluorescently labeled antibodies: phycoerythrin (PE) -conjugated anti-Foxp3 (MF-14, RRID:AB_1089118), PE-conjugated anti-IL-4 (11B11, RRID:AB_315318), or PE-conjugated anti-IFN-γ (XMG1.2, RRID:AB_315401). Labeled isotype control antibodies consisted of PE-conjugated rat IgG1κ (RTK2071, RRID:AB_326514), biotin-conjugated rat IgG2bκ (MRG2b-85, RRID:AB_492999), Alexa Fluor® 488 (AF488) -conjugated Armenian hamster IgG (HTK888, RRID: AB_2814703), and biotin-conjugated rat IgG2aκ (RTK2758, RRID:AB_2783537). After washing with PBS-BSA and centrifugation for 5 min at 300 × g, 4°C. the cells were stained for analysis of surface makers by incubation for 1 h at 4°C in PBS-BSA containing allophycocyanin (APC) -conjugated anti-CD3 (17A2, RRID:AB_312668), AF488-conjugated anti-CD4 (GK1.5, RRID:AB_389302), biotin-conjugated anti-F4/80 (BM8, RRID:AB_893501), biotin-conjugated anti-CD11c (3.9, RRID:AB_313772), and AF488-conjugated anti-CD103 (2E7, RRID:AB_535949). Labeled isotype control antibodies consisted of biotin-conjugated Armenian hamster IgG (HTK888), AF488-conjugated rat IgG2bκ (RTK4530, RRID:AB_389321), AF488-conjugated Armenian hamster IgG (HTK888, RRID:AB_2814703), and biotin-conjugated rat IgG2aκ (RTK2758, RRID:AB_2783537). After washing with PBS-BSA and centrifugation for 5 min at 300 × g, 4°C. APC-, PE-, and PerCP/Cy5.5-conjugated streptavidin were used as linkers for the biotin-conjugated antibodies. Cells were washed and the percentage of Foxp3+ CD4+ CD3+ cells, IL-4+CD4+ cells, IFN-γ+CD4+ cells, and CD103+ CD11c+ DCs were determined using a Sony SH800 Cell Sorter (Sony, Tokyo, Japan) or a FACSCalibur (BD Biosciences, San Jose, CA, USA, RRID:SCR_013311). Each flow cytometer was equipped with both a krypton laser (642 nm) and an argon laser (488 nm). For each of the flow cytometric analyses, 1.0 ×103~1.0 ×104 events were recorded. A compensation setup including a single fluorescent cell with SH800 software was used. The data were analyzed using FlowJo (ver. 10.1r5).
Gene Expression Analysis
Total RNA from FP- and/or OVA-stimulated splenocytes was extracted using NucleoSpin RNA Kits (TaKaRa Bio Inc., Osaka, Japan) in accordance with the manufacturer's instructions. The purified total RNA was reverse-transcribed using a PrimeScriptTM RT Master Mix (TaKaRa Bio Inc., Osaka, Japan) according to the manufacturer's instructions. Quantitative PCR (qPCR) reactions were performed as described previously (27). Primers for the transcripts encoding β-actin, IL-4, and IFN-γ were purchased from TaKaRa Bio, Inc.
Relative Abundance of Bacterial Phyla by Amount of 16S rDNA
MLNs were prepared as described previously (25). At collection, cecal contents and MLNs were immersed in RNAlater (Qiagen, Valencia, CA, USA, RRID:SCR_008539) at 4°C for 24 h. The RNAlater then was removed from the cecal contents and MLNs by centrifugation at 8,000 × g for 5 min. Thereafter, the cecal contents were stored at −20°C until DNA extraction. At the time of DNA extraction, the cecal contents (5–10 mg) and MLNs were mixed with the Inhibitory Ex buffer (200 μL) of the QIAamp Fast DNA Stool Mini Kit (Qiagen, RRID:SCR_008539) in a Zirco Prep Mini tube (Nippon Genetics Co., Ltd., Tokyo, Japan). The sample was shaken for 5 min using a Bug Crasher μT-12 (Taitec Co., Saitama, Japan). DNA was purified from the mixture using the QIAamp Fast DNA Stool Mini Kit (Qiagen, RRID:SCR_008539). qPCR was performed using the KAPA SYBR FAST qPCR master mix (KAPA Biosystems). The primer sequences are provided in Table 1. The 16S rDNA levels of the gut microbiota in the MLNs and cecal contents were quantified by qPCR with a Thermal Cycler Dice Real Time System II (TaKaRa Bio, Inc.) and the following cycling conditions: 95°C for 10 s, followed by 50 cycles of 95°C for 5 s and 60°C for 30 s. Dissociation was performed by incubation at 95°C for 15 s, 60°C for 30 s, and 95°C for 15 s. Quantification of the target 16S rDNA levels of Firmicutes, Bacteroides/Prevotella, β/γ/δ/ε-Proteobacteria, Actinobacteria, Deferribacteres, Verrucomicrobia, Tenericutes, and FP in cecal contents was performed using the ΔΔCt method with normalization to the total 16S rDNA content (31).
ELISA
Commercially available ELISA kits were used to quantify serum levels of OVA-specific IgE (eBioscience Inc., San Diego, CA, USA, RRID:SCR_003660) and lipopolysaccharide binding protein (LBP) (Biometec, Greifswald, Germany). The concentrations of IL-10 in culture supernatants was measured using a Mouse IL-10 IQELISA™ Kit (RayBiotech, Norcross, GA, USA). These ELISAs were performed according to the respective manufacturer's instructions.
Western Blotting
The flash-frozen MLN samples were thawed and suspended in CelLytic M (Sigma). The resulting lysates then were diluted in sample buffer (FUJIFILM Wako Pure Chemical Co.) and incubated at 70°C for 30 min. Samples were resolved by 15% (v/v) SDS-PAGE. Proteins then were transferred from the gel onto a Hybond-P PVDF membrane (GE Healthcare Japan, Tokyo, Japan). After blocking for 1 h, the membrane was incubated overnight with anti-mouse Foxp3 (SAB2700362), anti-mouse GATA3 (SAB1405059), and anti-mouse β-actin (clone AC-15) antibodies at 4°C, followed by incubation with a secondary antibody. The immunoblots were visualized using the ECL Prime Western Blotting Detection Reagent (GE Healthcare Japan). The protein bands were detected using an ImageQuant LAS 4000mini chemiluminescence analyzer (GE Healthcare Japan) and analyzed using ImageJ software (version 1.51; National Institutes of Health, Bethesda, MD, USA, RRID:SCR_003070). Quantitation of the proteins was performed by densitometry; values were normalized to the expression level of the housekeeping protein β-actin.
Statistical Analysis
Statistical analyses were performed using Prism 7 (GraphPad Software, Inc., San Diego, CA, USA, RRID:SCR_002798). The significance of differences among 3 or more groups were assessed by two-tailed one-way analysis of variance (ANOVA) followed by the Tukey–Kramer test. Differences were considered significant at p < 0.05.
Results
FP Ameliorates Th2 Immune Responses
In an initial set of experiments, we examined the effects of OVA and FP (at 1 ×106 or 1 ×108 cfu/well) on transcription, as assessed in vitro using splenocytes derived from OVA-sensitized mice. Exposure of cells to OVA alone yielded significant increases in the levels of Il4 and Il10, but not in that of Ifng, compared to the effect of medium lacking antigen (Figures 1B–D). Exposure of cells to OVA and FP (at 106 or 108 cfu/well) yielded significant attenuation of the OVA-induced accumulation of Il4 but not that of Il10. Indeed, OVA plus the lower dose of FP potentiated the level of Ifng seen with OVA alone, an effect not seen with the higher dose of FP (Figure 1C). In a second set of experiments, we extended this analysis to the in vivo response of OVA-sensitized mice. Notably, animals administered PBS demonstrated significant accumulation of Il4 and Ifng transcripts (but not of Il10) (Figures 1F–H) and significant decreases of anti-OVA IgE in serum (Figure 2A). Moreover, we found that FP decreased the numbers of IL-4+CD4+, IFN-γ+CD4+ cells (Figures 2B,C) and increased CD4+CD25+ T cells, CD103+CD11c+ DCs in spleen (Figures 2D,E). CD103+CD11c+ DCs in the MLNs of the FP group did not differ significantly from that in the PBS group (Figure 2F). However, the expression of GATA3 in the MLNs of the FP group was significantly decreased compared with the PBS group (Figure 2G).
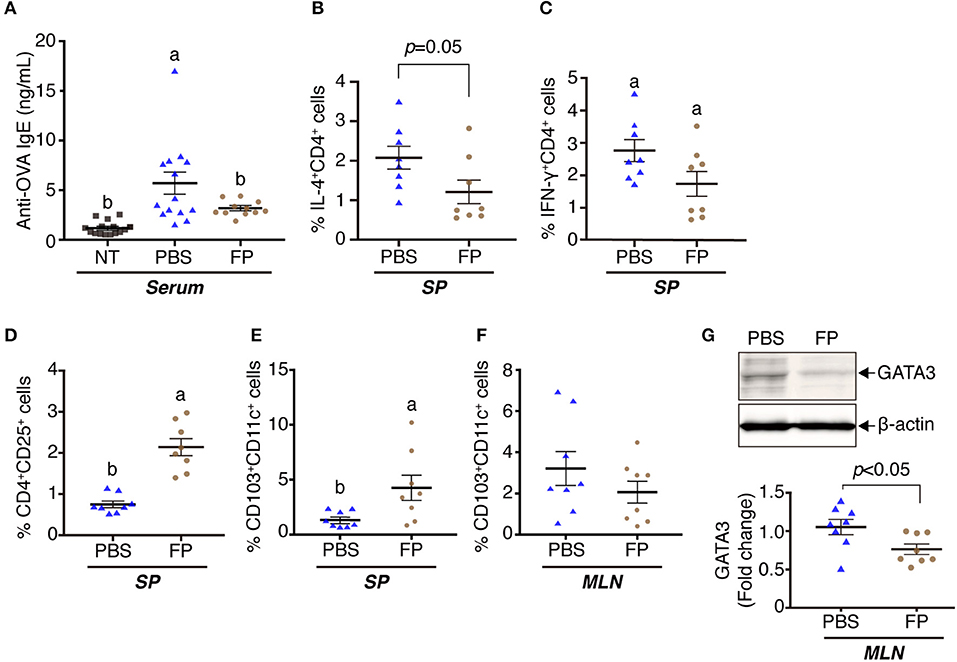
Figure 2. Oral administration of FP augmented immune regulatory effects on OVA-sensitized mice. The levels of anti-OVA-specific IgE were measured in serum by ELISA (A). IL-4+CD4+ (B), IFN-γ+CD4+ (C), CD4+CD25+ (D), and CD103+CD11c+ (E,F) cells among the splenocytes (B–E) or MLNs (F) were analyzed by flow cytometry. GATA3 were determined by western blotting (G). Data are expressed as mean ± SE and pooled from four independent experiments (n = 8–16/group). Different letters (i.e., a, b, c) indicate significant differences among groups (p < 0.05).
FP Reaches MLNs Following Oral Administration
We next examined the destination of FP following oral administration, and effects of the bacteria on the gut microbiota. The proportion of FP in the MLNs of the FP group was increased significantly compared with that in the PBS group (Figure 3A). In contrast, the proportion of FP in the cecal contents did not differ significantly between the FP and PBS groups (Figure 3B). It is formally possible that FP gained access to the MLNs in these animals by means of damage to the intestinal barrier. The expression of Il4 in spleen showed a significant negative correlation with the relative abundance of FP in the MLNs when comparing between the FP and PBS groups (ρ = −0.73, p = 0.004; Figure 3C). To address this issue, we assessed the state of the intestinal barrier in these mice by measuring the serum level of lipopolysaccharide-binding protein (LBP). Secreted LBP would affect intestinal barrier against bacteria. Notably, we detected no significant difference in the serum LBP levels between the FP and PBS groups (Figure 3D). The proportion of Deferribacteres in the cecal contents of the FP group was increased significantly compared with that in the NT group (Figure 4). We found no significant differences for any of the other comparisons. These results suggested that FP did not affect the intestinal barrier function, indicating that FP (or at least some FP genomic DNA) may reach the MLNs.
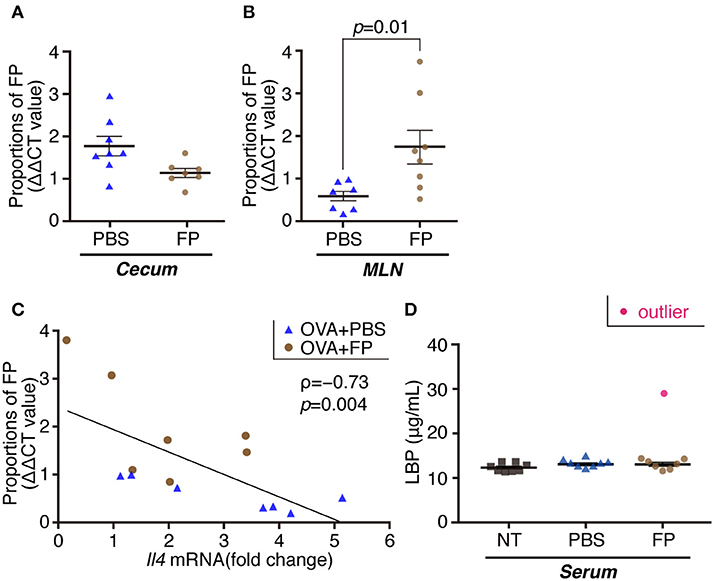
Figure 3. Proportions of FP composition of cecal contents/MLNs and the levels of serum LBP. Proportions of FP were determined by qPCR in cecal contents (A) and MLNs (B). Correlation between Il4 expression in splenocytes and FP composition of MLNs (C). ρ indicates Spearman's correlation coefficient; and p indicates p value (n = 7) (C). The levels of serum LBP were determined by ELISA (D). Data are expressed as mean ± SE and pooled from two independent experiments (n = 7–8/group). There were significant differences between PBS and FP groups (p = 0.01).
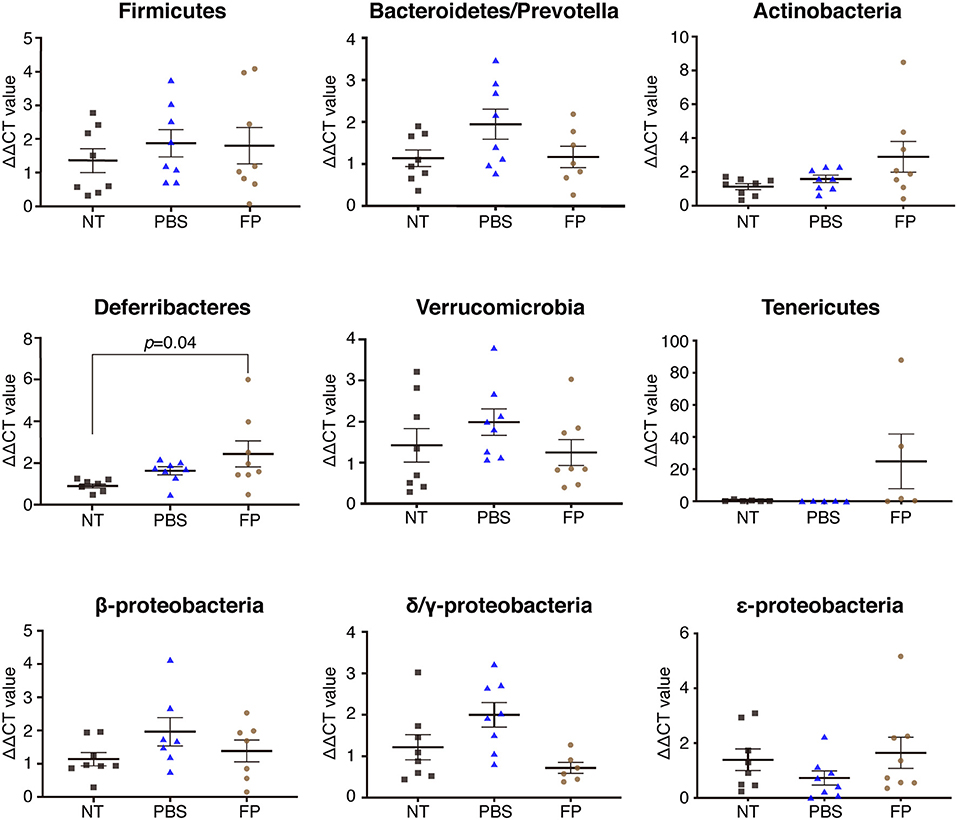
Figure 4. Effect of FP on the cecal microbiota. Proportions of Firmicutes, Bacteroides/Prevotella, Actinobacteria, Deferribacteres, Verrucomicrobia, Tenericutes, β-Proteobacteria, δ/γ-Proteobacteria, and ε-Proteobacteria were assessed in cecal contents by qPCR. Data are expressed as mean ± SE and pooled from two independent experiments (n = 5–8/group).
FP Induces CD103+ DC and Foxp3+ T cell Differentiation
We examined the differentiation of CD103+ DCs by FP. Significantly increased fold-changes in the numbers of CD103+ DCs among the splenocyte DCs and MLN DCs were observed in the FP group compared with those in the Med group (Figures 5A,B). Additionally, we examined the differentiation of Foxp3+ T cells by FP. Again, significantly increased fold-changes in the numbers of Foxp3+ T cells among the splenocyte T cells and MLNs T cells were observed in the FP group (Figures 5C,D).
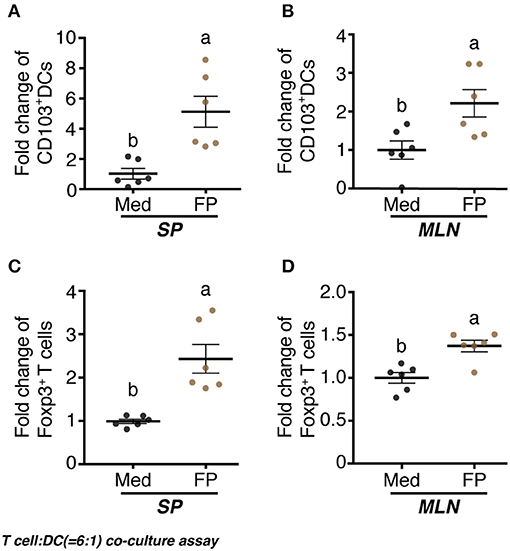
Figure 5. Effect of FP on T cell-DC (6:1) co-culture assay. naïve CD4+ T cells and CD11c+ DCs were prepared from splenocytes and MLNs using the MACS cell sorting system. DCs were treated with Med or FP (FP 1 ×108 cfu/well) at 37°C for 24 h in 96-well plates. T cells and DCs were mixed at a ratio of 6:1 and treated with Med or FP at 37°C for 96 h in 96-well plates. CD103+ DCs (A,B) and Foxp3+ T (C,D) cells in splenocytes (A,C), or MLNs (B,D) were analyzed by flow cytometry. Data are expressed as mean ± SD and pooled from two independent experiments (n = 6/group). Different letters (i.e., a, b) indicate significant differences among groups (p < 0.05).
Discussion
The FP species has been classified into Clostridia cluster IV in the Ruminococcaceae family. Several reports have shown that the gut microbiota of Clostridia cluster IV is capable of inhibiting inflammation. For instance, Li et al. reported that Clostridium leptum induces proliferation of Treg cells (32); Rossi et al. reported that Faecalibacterium prausnitzii (a member of the Ruminococcaceae family) strongly induces IL-10 production (33); and Wu et al. reported that decreases in the proportion of the Ruminococcaceae family induce IgE-associated eczema in infants (34). The results of the present study showed that Th2 immune responses induced by the addition of OVA were attenuated significantly by the ingestion of FP in OVA-sensitized mice. Thus, our work suggested that FP influences the balance of the host immune system.
In vitro, the expression of mRNAs encoding IFN-γ (a Th1 cytokine) and IL-10 (a regulatory cytokine) was increased by FP, effects that were not seen for expression of the gene encoding IL-4 (a Th2 cytokine). These results suggested that FP inhibits Th2 responses by activating Treg and Th1 cells. Indeed, it has been reported that Tregs are induced and Th2 responses are suppressed under conditions that lead to increases in the number of CD103+ DCs that are tolerant to the gram-positive bacteria Bifidobacterium and Lactobacillus (L.) rhamnosus GG as well as to the FP strain (35, 36). The present study revealed that FP induces CD103+ DC differentiation in vitro. However, mice administered FP did not exhibit an increase in CD103+ MLN DCs. Activated-DCs move to the MLN via Peyer's patches and induce T cell differentiation. The differentiated T cells are subsequently involved in the systemic immune response (37). In this study, the MLN was collected from mice 48 h after FP administration. The in vivo experiment does not simulate the in vitro experiment with the direct stimulation by FP. In other words, the CD103+ DC differentiation phenomenon with FP administration will need to be tested using more detailed time monitoring. In vivo, Il10 expression and the population of CD4+CD25+ cells in splenocytes was increased in the FP group. Foxp3, Treg transcription factor, has been reported to be specifically expressed on CD4+CD25+ T cells and is used as a cell surface marker for Tregs (38, 39). CD4+CD25+ T cells have been reported to contribute to the alleviation and prevention of allergic diseases (40, 41). Orally administered FP strains may reach the intestinal tract and stimulate intestinal cells. A number of Treg cells are present in the intestinal tract and contribute to the induction of tolerance (42). FP has been reported to increase the number of Treg cells in colonic tissues and to exert anti-allergic effects (23). Indeed, we found that FP induces Foxp3+CD4+ T cell differentiation in vitro. We also showed that FP decreases the expression of GATA3, a transcription factor for Th2 cells, in the MLNs of OVA-sensitized mice. It will be important to investigate how the oral administration of FP downregulates the Th2 immune response through DC and/or Treg responses in the MLN. Some reports have established that immune cell transplantation plays a fundamental role in animal physiology (43–45). Further studies will be necessary to confirm whether FP-treated DCs/Tregs downregulate the Th2 immune response by inhibiting the differentiation of Th2 cells. We infer that oral administration of FP and induction of Tregs dominates the intestinal immune response, and is followed by migration of FP through the bloodstream and lymphatic system, where the bacteria contribute to the splenic tissue immune response. However, in the present study, in vivo Ifng levels differed from those in the in vitro study. In addition, immune responses appeared to be decreased in the in vivo experiments compared to those in the in vitro experiments. Similar results were obtained in the report of Tobita et al. using L. crispatus (46). This phenomenon may reflect differences in the environments in which the bacteria act. The spleen cells used in the in vitro study were already OVA-sensitized, meaning that the Th2 cells already had been activated. Therefore, Th1 and Treg presumably were activated by the immunosuppressive effects of the FP strain, restoring the T cell balance to normal. On the other hand, since the FP was administered prior to OVA sensitization in the in vivo test, the immunosuppressive effect of FP on Tregs would predominate in that experiment, preventing Th2 activation without Th1 activation.
Oral administration of L. reuterii, a gram-positive bacterium similar to FP, in a mouse allergy model has been reported to decrease Il4 and Ifng, and increase Il10 mRNA expression in the spleen (47). Moreover, FP may be responsible for suppressing allergic responses by improving intestinal barrier function. Based on the above results, we infer that FP contributes to control of the allergic response by activating Tregs and suppressing the expression of Il4, a Th2-related gene. In the future, to confirm these mechanistic differences, it will be necessary to test the immune status of mice treated with the FP strain prior to OVA sensitization. Kwon et al. reported that oral administration of L. sakei WIKIM30 induces tolerogenic DCs and Treg cells, thereby improving allergic symptoms in mouse models of allergic dermatitis (48). It also has been reported that oral administration of Bifidobacterium increases Treg numbers and improves allergic symptoms in OVA-sensitized allergy model mice (49, 50).
This study showed that the serum titer of OVA-specific IgE was decreased significantly by the intake of FP. In contrast, there was no significant difference (between the FP and PBS groups) in the expression of genes encoding proteins (IL-7 and IL-21) related to class switching (51, 52) (Figure S1). Moreover, no significant differences in the levels of SCFAs were detected between the FP and PBS groups, in either the cecal contents or sera of these mice (Figure S2). Thus, oral administration of FP did not yield a significant change in SCFA production. These results suggested that the SCFAs do not mediate Th2 suppression by FP. Thus, FP may not be involved in the IgE class-switch recombination. Accordingly, FP may be involved in the inhibition of OVA-specific IgE by regulating the Th2 immune response. The expression of Il4 in splenocytes showed a significant negative correlation with the relative abundance of FP in the MLNs when comparing between the FP and PBS groups. Accordingly, the accumulation of FP in the MLNs may regulate the Th2 immune response. The proportion of Deferribacteres in the cecal contents of the FP group was increased significantly compared to that in the NT group. This observation may relate to a report by Ma et al. indicating that the proportion of Deferribacteres was increased following oral administration of probiotics in an OVA-induced mouse model of food allergy (53). Deferribacteres bacteria are able to obtain energy through obligate or facultative anaerobic metabolism. The iron metabolism of Deferribacteres in intestinal flora has been shown to be related to iron balance in the gastrointestinal tract (54). By analogy, we infer that FP also may be related to iron metabolism.
In conclusion, the collection of FP in MLNs may be involved in inhibition of the Th2 immune response. Our results demonstrated that FP mediates the inhibition of OVA-specific IgE production in a Th2-dominant environment. Given that allergy is closely associated with the production of antigen-specific IgE, FP may be useful in alleviating antigen-specific Th2 immune responses in a Th2-dominant environment. The results described here suggest that FP might be employed as a probiotic in the treatment of allergy. However, other work has suggested this organism as a possible causative agent of acute cholecystitis in a patient with a bloodstream infection (55). Clearly, further studies addressing the safety of FP will be needed before this bacterium can be employed as an anti-allergy probiotic.
Data Availability Statement
All datasets generated for this study are included in the article/Supplementary Material.
Ethics Statement
All experimental procedures were carried out in accordance with the Regulations for Animal Experimentation of Shinshu University. All experimental procedures were reviewed by the Committee for Animal Experiments of Shinshu University and found to be compliant with national regulations and guidelines, as specified by Law No. 105 and Notification No. 6. Therefore, the animal protocol was approved by the Committee for Animal Experiments of Shinshu University as Approval No. 290074.
Author Contributions
TO, YY, SS, TSa, and TSh conceived and designed the experiments. TO, YY, and AM conducted the experiments and performed mathematical analyses. TO, YY, and TSh wrote the paper. TSh designed and supervised the work. All authors reviewed and approved the manuscript.
Conflict of Interest
The authors declare that the research was conducted in the absence of any commercial or financial relationships that could be construed as a potential conflict of interest.
Acknowledgments
We thank Ms. Chika Fukasawa (Faculty of Agriculture, Shinshu University) for excellent animal care. We thank Dr. Ayumi Oda and Dr. Hiroaki Hara of Division of Instrumental Research, Research Center for Supports to Advanced Science, Shinshu University for technical support. This study was supported by a Grant-in-Aid for Young Scientists (B) (No. 17K15268) to TO.
Supplementary Material
The Supplementary Material for this article can be found online at: https://www.frontiersin.org/articles/10.3389/fimmu.2020.00379/full#supplementary-material
Figure S1. Il7 and Il21 response to FP administration. Expression of mRNAs encoding IL-7 (A) and IL-21 (B) were assessed in splenocytes isolated from OVA-sensitized mice. Data are expressed as mean ± SE of two independent experiments (n = 12–16).
Figure S2. Short-chain fatty acid levels in cecum and serum. Short-chain fatty acid (SCFA) analysis was performed using a high-performance liquid chromatography (HPLC) system according to the method. SCFAs were separated using a Shimadzu HPLC system. An Aminex HPX-87H column (Bio-Rad Laboratories, Hercules, CA, USA, RRID:SCR_008426) was used at 35°C. Absorbance at 210 nm was monitored to detect SCFAs using a Shimazu SPD-10A UV–Vis detector. Levels of acetate (A), butyrate (B), isovalerate (C), propionate (D,H), isobutyrate (E,I), lactate (F,J), and succinate (G,K) were measured in cecum contents (A–G) and serum (H–K) by HPLC. Data are expressed as mean ± SE of two independent experiments (n = 5–8). Outliers and no detected data were excluded.
References
1. Yu W, Freeland DMH, Nadeau KC. Food allergy: immune mechanisms, diagnosis and immunotherapy. Nat Rev Immunol. (2016) 16:751–65. doi: 10.1038/nri.2016.111
2. Tang ML, Mullins RJ. Food allergy: is prevalence increasing? Intern Med J. (2017) 47:256–61. doi: 10.1111/imj.13362
3. Strachan DP. Hay fever, hygiene, and household size. BMJ. (1989) 299:1259–60. doi: 10.1136/bmj.299.6710.1259
4. Julia V, Macia L, Dombrowicz D. The impact of diet on asthma and allergic diseases. Nat Rev Immunol. (2015) 15:308–22. doi: 10.1038/nri3830
5. Jain N, Walker WA. Diet and host-microbial crosstalk in postnatal intestinal immune homeostasis. Nat Rev Gastroenterol Hepatol. (2015) 12:14–25. doi: 10.1038/nrgastro.2014.153
6. Yamamoto Y, Shigemori S, Nigar S, Oshiro K, Wang Y, Sato T, et al. Development of a simple IgE-independent anaphylactic model using buckwheat antigen and B-type CpG oligodeoxynucleotide from Streptococcus thermophilus. Anim Sci J. (2016) 87:710–7. doi: 10.1111/asj.12479
7. Chu DK, Mohammed-Ali Z, Jimenez-Saiz R, Walker TD, Goncharova S, Llop-Guevara A, et al. T helper cell IL-4 drives intestinal Th2 priming to oral peanut antigen, under the control of OX40L and independent of innate-like lymphocytes. Mucosal Immunol. (2014) 7:1395–404. doi: 10.1038/mi.2014.29
8. Maeda-Yamamoto M, Ema K, Shibuichi I. In vitro and in vivo anti-allergic effects of 'benifuuki' green tea containing O-methylated catechin and ginger extract enhancement. Cytotechnology. (2007) 55:135–42. doi: 10.1007/s10616-007-9112-1
9. Kim WG, Kang GD, Kim HI, Han MJ, Kim DH. Bifidobacterium longum IM55 and Lactobacillus plantarum IM76 alleviate allergic rhinitis in mice by restoring Th2/Treg imbalance and gut microbiota disturbance. Benef Microbes. (2019) 10:55–67. doi: 10.3920/BM2017.0146
10. Sano M, Suzuki M, Miyase T, Yoshino K, Maeda-Yamamoto M. Novel antiallergic catechin derivatives isolated from oolong tea. J Agric Food Chem. (1999) 47:1906–10. doi: 10.1021/jf981114l
11. Suzuki M, Yoshino K, Maeda-Yamamoto M, Miyase T, Sano M. Inhibitory effects of tea catechins and O-methylated derivatives of (-)-epigallocatechin-3-O-gallate on mouse type IV allergy. J Agric Food Chem. (2000) 48:5649–53. doi: 10.1021/jf000313d
12. Fujimura Y, Tachibana H, Maeda-Yamamoto M, Miyase T, Sano M, Yamada K. Antiallergic tea catechin, (-)-epigallocatechin-3-O-(3-O-methyl)-gallate, suppresses FcepsilonRI expression in human basophilic KU812 cells. J Agric Food Chem. (2002) 50:5729–34. doi: 10.1021/jf025680z
13. Maeda-Yamamoto M, Inagaki N, Kitaura J, Chikumoto T, Kawahara H, Kawakami Y, et al. O-methylated catechins from tea leaves inhibit multiple protein kinases in mast cells. J Immunol. (2004) 172:4486–92. doi: 10.4049/jimmunol.172.7.4486
14. Sang S, Lambert JD, Ho CT, Yang CS. The chemistry and biotransformation of tea constituents. Pharmacol Res. (2011) 64:87–99. doi: 10.1016/j.phrs.2011.02.007
15. Ozdal T, Sela DA, Xiao J, Boyacioglu D, Chen F, Capanoglu E. The reciprocal interactions between polyphenols and gut microbiota and effects on bioaccessibility. Nutrients. (2016) 8:78. doi: 10.3390/nu8020078
16. Dethlefsen L, Eckburg PB, Bik EM, Relman DA. Assembly of the human intestinal microbiota. Trends Ecol Evol. (2006) 21:517–23. doi: 10.1016/j.tree.2006.06.013
17. Rooks MG, Garrett WS. Gut microbiota, metabolites and host immunity. Nat Rev Immunol. (2016) 16:341–52. doi: 10.1038/nri.2016.42
18. Pamer EG. Microbial tuning of the mammalian immune system. Trends Mol Med. (2017) 23:379–80. doi: 10.1016/j.molmed.2017.03.006
19. Wopereis H, Sim K, Shaw A, Warner JO, Knol J, Kroll JS. Intestinal microbiota in infants at high risk for allergy: effects of prebiotics and role in eczema development. J Allergy Clin Immunol. (2018) 141:1334–42e5. doi: 10.1016/j.jaci.2017.05.054
20. Feehley T, Plunkett CH, Bao R, Choi Hong SM, Culleen E, Belda-Ferre P, et al. Healthy infants harbor intestinal bacteria that protect against food allergy. Nat Med. (2019) 25:448–53. doi: 10.1038/s41591-018-0324-z
21. Flandroy L, Poutahidis T, Berg G, Clarke G, Dao MC, Decaestecker E, et al. The impact of human activities and lifestyles on the interlinked microbiota and health of humans and of ecosystems. Sci Total Environ. (2018) 627:1018–38. doi: 10.1016/j.scitotenv.2018.01.288
22. Carlier JP, Bedora-Faure M, K'Ouas G, Alauzet C, Mory F. Proposal to unify Clostridium orbiscindens Winter et al. 1991 and Eubacterium plautii (Seguin 1928) Hofstad and Aasjord (1982). with description of Flavonifractor plautii gen. nov., comb. nov., and reassignment of Bacteroides capillosus to Pseudoflavonifractor capillosus gen. nov., comb. nov. Int J Syst Evol Microbiol. (2010) 60 (Pt 3):585-90. doi: 10.1099/ijs.0.016725-0
23. Atarashi K, Tanoue T, Oshima K, Suda W, Nagano Y, Nishikawa H, et al. Treg induction by a rationally selected mixture of Clostridia strains from the human microbiota. Nature. (2013) 500:232–6. doi: 10.1038/nature12331
24. Ogita T, Nakashima M, Morita H, Saito Y, Suzuki T, Tanabe S. Streptococcus thermophilus ST28 ameliorates colitis in mice partially by suppression of inflammatory Th17 cells. J Biomed Biotechnol. (2011) 2011:378417. doi: 10.1155/2011/378417
25. Ogita T, Bergamo P, Maurano F, D'Arienzo R, Mazzarella G, Bozzella G, et al. Modulatory activity of Lactobacillus rhamnosus OLL2838 in a mouse model of intestinal immunopathology. Immunobiology. (2015) 220:701–10. doi: 10.1016/j.imbio.2015.01.004
26. Denning TL, Wang YC, Patel SR, Williams IR, Pulendran B. Lamina propria macrophages and dendritic cells differentially induce regulatory and interleukin 17-producing T cell responses. Nat Immunol. (2007) 8:1086–94. doi: 10.1038/ni1511
27. Yamamoto Y, Sugimura R, Watanabe T, Shigemori S, Okajima T, Nigar S, et al. Class A CpG Oligonucleotide priming rescues mice from septic shock via activation of platelet-activating factor acetylhydrolase. Front Immunol. (2017) 8:1049. doi: 10.3389/fimmu.2017.01049
28. Oswari H, Prayitno L, Dwipoerwantoro PG, Firmansyah A, Makrides M, Lawley B, et al. Comparison of stool microbiota compositions, stool alpha1-antitrypsin and calprotectin concentrations, and diarrhoeal morbidity of Indonesian infants fed breast milk or probiotic/prebiotic-supplemented formula. J Paediatr Child Health. (2013) 49:1032–9. doi: 10.1111/jpc.12307
29. Thomas P. Intense association of non-culturable endophytic bacteria with antibiotic-cleansed In vitro watermelon and their activation in degenerating cultures. Plant Cell Rep. (2011) 30:2313–25. doi: 10.1007/s00299-011-1158-z
30. Yang YW, Chen MK, Yang BY, Huang XJ, Zhang XR, He LQ, et al. Use of 16S rRNA gene-targeted group-specific primers for real-time PCR analysis of predominant bacteria in mouse feces. Appl Environ Microbiol. (2015) 81:6749–56. doi: 10.1128/AEM.01906-15
31. Tuomisto S, Karhunen PJ, Pessi T. Time-dependent post mortem changes in the composition of intestinal bacteria using real-time quantitative PCR. Gut Pathogens. (2013) 5:35 doi: 10.1186/1757-4749-5-35
32. Li YN, Huang F, Cheng HJ, Li SY, Liu L, Wang LY. Intestine-derived Clostridium leptum induces murine tolerogenic dendritic cells and regulatory T cells in vitro. Hum Immunol. (2014) 75:1232–8. doi: 10.1016/j.humimm.2014.09.017
33. Rossi O, van Berkel LA, Chain F, Tanweer Khan M, Taverne N, Sokol H, et al. Faecalibacterium prausnitzii A2-165 has a high capacity to induce IL-10 in human and murine dendritic cells and modulates T cell responses. Sci Rep. (2016) 6:18507. doi: 10.1038/srep18507
34. West CE, Ryden P, Lundin D, Engstrand L, Tulic MK, Prescott SL. Gut microbiome and innate immune response patterns in IgE-associated eczema. Clin Exp Allergy. (2015) 45:1419–29. doi: 10.1111/cea.12566
35. Fu L, Song J, Wang C, Fu S, Wang Y. Bifidobacterium infantis potentially alleviates shrimp tropomyosin-induced allergy by tolerogenic dendritic cell-dependent induction of regulatory T Cells and alterations in gut microbiota. Front Immunol. (2017) 8:1536. doi: 10.3389/fimmu.2017.01536
36. Zhang J, Ma JY, Li QH, Su H, Sun X. Lactobacillus rhamnosus GG induced protective effect on allergic airway inflammation is associated with gut microbiota. Cell Immunol. (2018) 332:77–84. doi: 10.1016/j.cellimm.2018.08.002
37. Islam SA, Luster AD. T cell homing to epithelial barriers in allergic disease. Nat Med. (2012) 18:705–15. doi: 10.1038/nm.2760
38. Yagi H, Nomura T, Nakamura K, Yamazaki S, Kitawaki T, Hori S, et al. Crucial role of FOXP3 in the development and function of human CD25+CD4+ regulatory T cells. Int Immunol. (2004) 16:1643–56. doi: 10.1093/intimm/dxh165
39. Sakaguchi S, Yamaguchi T, Nomura T, Ono M. Regulatory T cells and immune tolerance. Cell. (2008) 133:775–87. doi: 10.1016/j.cell.2008.05.009
40. Ling EM, Smith T, Nguyen XD, Pridgeon C, Dallman M, Arbery J, et al. Relation of CD4+CD25+ regulatory T-cell suppression of allergen-driven T-cell activation to atopic status and expression of allergic disease. Lancet. (2004) 363:608–15. doi: 10.1016/S0140-6736(04)15592-X
41. Li Q, Shen HH. Neonatal bacillus Calmette-Guerin vaccination inhibits de novo allergic inflammatory response in mice via alteration of CD4+CD25+ T-regulatory cells. Acta Pharmacol Sin. (2009) 30:125–33. doi: 10.1038/aps.2008.3
42. Omenetti S, Pizarro TT. The Treg/Th17 axis: a dynamic balance regulated by the gut microbiome. Front Immunol. (2015) 6(Article 639). doi: 10.3389/fimmu.2015.00639
43. Fujita S, Seino K, Sato K, Sato Y, Eizumi K, Yamashita N, et al. Regulatory dendritic cells act as regulators of acute lethal systemic inflammatory response. Blood. (2006) 107:3656–64. doi: 10.1182/blood-2005-10-4190
44. Nikolic A, Simovic Markovic B, Gazdic M, Randall Harrell C, Fellabaum C, Jovicic N, et al. Intraperitoneal administration of mesenchymal stem cells ameliorates acute dextran sulfate sodium-induced colitis by suppressing dendritic cells. Biomed Pharmacother. (2018) 100:426–32. doi: 10.1016/j.biopha.2018.02.060
45. Zhang X, Izikson L, Liu L, Weiner HL. Activation of CD25(+)CD4(+) regulatory T cells by oral antigen administration. J Immunol. (2001) 167:4245–53. doi: 10.4049/jimmunol.167.8.4245
46. Tobita K, Yanaka H, Otani H. Heat-treated Lactobacillus crispatus KT strains reduce allergic symptoms in mice. J Agric Food Chem. (2009) 57:5586–90. doi: 10.1021/jf900703q
47. Huang C-H, Lin Y-C, Jan T-R. Lactobacillus reuteri induces intestinal immune tolerance against food allergy in mice. J Funct Foods. (2017) 31:44–51. doi: 10.1016/j.jff.2017.01.034
48. Kwon MS, Lim SK, Jang JY, Lee J, Park HK, Kim N, Yun M, et al. Lactobacillus sakei WIKIM30 ameliorates atopic dermatitis-like skin lesions by inducing regulatory T cells and altering gut microbiota structure in mice. Front Immunol. (2018) 9:1905. doi: 10.3389/fimmu.2018.01905
49. Ren J, Zhao Y, Huang S, Lv D, Yang F, Lou L, et al. Immunomodulatory effect of Bifidobacterium breve on experimental allergic rhinitis in BALB/c mice. Exp Ther Med. (2018) 16:3996–4004. doi: 10.3892/etm.2018.6704
50. Kozakova H, Schwarzer M, Tuckova L, Srutkova D, Czarnowska E, Rosiak I, et al. Colonization of germ-free mice with a mixture of three lactobacillus strains enhances the integrity of gut mucosa and ameliorates allergic sensitization. Cell Mol Immunol. (2016) 13:251–62. doi: 10.1038/cmi.2015.09
51. Suto A, Nakajima H, Hirose K, Suzuki K, Kagami S, Seto Y, et al. Interleukin 21 prevents antigen-induced IgE production by inhibiting germ line C(epsilon) transcription of IL-4-stimulated B cells. Blood. (2002) 100:4565–73. doi: 10.1182/blood-2002-04-1115
52. Jeannin P, Delneste Y, Lecoanet-Henchoz S, Gretener D, Bonnefoy JY. Interleukin-7 (IL-7) enhances class switching to IgE and IgG4 in the presence of T cells via IL-9 and sCD23. Blood. (1998) 91:1355–61. doi: 10.1182/blood.V91.4.1355
53. Ma J, Zhang J, Li Q, Shi Z, Wu H, Zhang H, et al. Oral administration of a mixture of probiotics protects against food allergy via induction of CD103+ dendritic cells and modulates the intestinal microbiota. J Funct Foods. (2019) 55:65–75. doi: 10.1016/j.jff.2019.02.010
54. Li Y, Luan Y, Yue X, Xiang F, Mao D, Cao Y, et al. Effects of Codonopis bulleynana forest ex diels on Deferribacteres in constipation predominant intestine tumor: differential analysis. Saudi J Biol Sci. (2019) 26:395–401. doi: 10.1016/j.sjbs.2018.11.008
Keywords: Flavonifractor plautii, Th2, MLN, Deferribacteres, microbiota
Citation: Ogita T, Yamamoto Y, Mikami A, Shigemori S, Sato T and Shimosato T (2020) Oral Administration of Flavonifractor plautii Strongly Suppresses Th2 Immune Responses in Mice. Front. Immunol. 11:379. doi: 10.3389/fimmu.2020.00379
Received: 17 August 2019; Accepted: 17 February 2020;
Published: 28 February 2020.
Edited by:
Juarez Antonio Simões Quaresma, Instituto Evandro Chagas, BrazilReviewed by:
YunJae Jung, Gachon University, South KoreaSatoshi Hachimura, The University of Tokyo, Japan
Tong-Rong Jan, National Taiwan University, Taiwan
Andrey Santos, Campinas State University, Brazil
Copyright © 2020 Ogita, Yamamoto, Mikami, Shigemori, Sato and Shimosato. This is an open-access article distributed under the terms of the Creative Commons Attribution License (CC BY). The use, distribution or reproduction in other forums is permitted, provided the original author(s) and the copyright owner(s) are credited and that the original publication in this journal is cited, in accordance with accepted academic practice. No use, distribution or reproduction is permitted which does not comply with these terms.
*Correspondence: Takeshi Shimosato, shimot@shinshu-u.ac.jp