- 1Department of Oncology, University of Lausanne, Lausanne, Switzerland
- 2Laboratoire d'Immunobiologie, Université Libre de Bruxelles, Brussels, Belgium
Beyond its well-admitted role in development and organogenesis, it is now clear that the transcription factor c-Maf has owned its place in the realm of immune-related transcription factors. Formerly introduced solely as a Th2 transcription factor, the role attributed to c-Maf has gradually broadened over the years and has extended to most, if not all, known immune cell types. The influence of c-Maf is particularly prominent among T cell subsets, where c-Maf regulates the differentiation as well as the function of multiple subsets of CD4 and CD8 T cells, lending it a crucial position in adaptive immunity and anti-tumoral responsiveness. Recent research has also revealed the role of c-Maf in controlling Th17 responses in the intestine, positioning it as an essential factor in intestinal homeostasis. This review aims to present and discuss the recent advances highlighting the particular role played by c-Maf in T lymphocyte differentiation, function, and homeostasis.
Introduction
The Maf (musculoaponeurotic fibrosarcoma) gene encodes the transcription factor c-Maf or MAF. Originally identified in natural musculo-aponeurotic fibrosarcoma of chickens infected with the replication-defective retrovirus AS42, the founding member of the Maf family, named v-Maf, was described as an oncogene (1–3). Using a probe containing the v-Maf sequence, its cellular counterpart, identified as c-Maf, was thereafter cloned from a number of vertebrate genomes (4). In addition to its function as an oncogene, c-Maf was soon found to regulate various cellular differentiation and developmental processes within tissues. In particular, c-Maf expression controls lens fiber cell differentiation, crystalline gene expression, as well as lens development (5–7). In neural tissue, c-Maf controls the expression of mechanoreceptors involved in touch sensation (8, 9). It also regulates the embryonic development of tubular renal cells (10) and the differentiation of chondrocytes during endochondral bone development (11–13). c-Maf plays a predominant role for the erythropoiesis that accompanies erythroblastic islands formation in fetal liver (14). In porcine and human pancreatic islets (15), c-Maf also regulates glucagon hormone production, thereby establishing pancreatic endocrine function (16). In line with the major contributions of c-Maf in developmental and physiological processes, mice lacking c-Maf are embryonically (14) or perinatally (5, 7) lethal depending on the type of C57BL/6 background. Some mice on the BALB/c background live to adulthood (10, 13).
In parallel to the discovery of the many roles of c-Maf within tissue development, c-Maf soon emerged as an immune regulator and was initially identified as a Th2 transcription factor. Similar to its function in tissue development, the role attributed to c-Maf within immune regulation broadened over the years and has extended to most, if not all, known immune cell types. While the role of c-Maf has also been studied within innate immune cell types (17–19) and B lymphocytes (20), we focus on c-Maf within T cell subsets, where c-Maf regulates the differentiation as well as the function of multiple subsets of CD4 T cells, lending it a crucial position in T cell immunity. Recent research has revealed the role of c-Maf in the control of intestinal Th17 responses by regulatory T cells, positioning it as an essential factor in regulatory T cell specification and, more broadly, the maintenance of intestinal homeostasis. This review aims to present and discuss the recent advances highlighting the particular role played by c-Maf in T lymphocyte differentiation, function, and homeostasis.
The c-Maf Transcription Factor
This basic leucine zipper (bZIP) transcription factor belongs to the AP-1 superfamily, which includes Fos, Jun, ATF, and CREB. The Maf transcription factor family is composed of 7 members divided into two subclasses: the large Maf proteins composed of MAFA/L-MAF, MAFB, MAF/c-Maf, and NLR (neural retina leucine zipper), and the small Maf proteins, MAFK, MAFG, and MAFF, which lack the amino-terminal transactivation domain. The Maf family of transcription factors harbors a unique and highly conserved basic region-leucine zipper (bZIP) structure (21). The basic regions of dimeric Maf factors allows them to recognize a palindromic sequence referred to as the Maf Recognition Element (MARE). This sequence is composed of a 7-bp TPA-Responsive Element (TRE) or a 8-bp cyclic AMP-Responsive Element (CRE) core region and a TGC flanking sequence bound by the Extended Homology Region (EHR), exclusively found in Maf proteins (22) (Figure 1). This long recognition sequence thus distinguishes the Maf protein family from other AP-1 family members and contributes to the important functions of the Maf proteins (23).
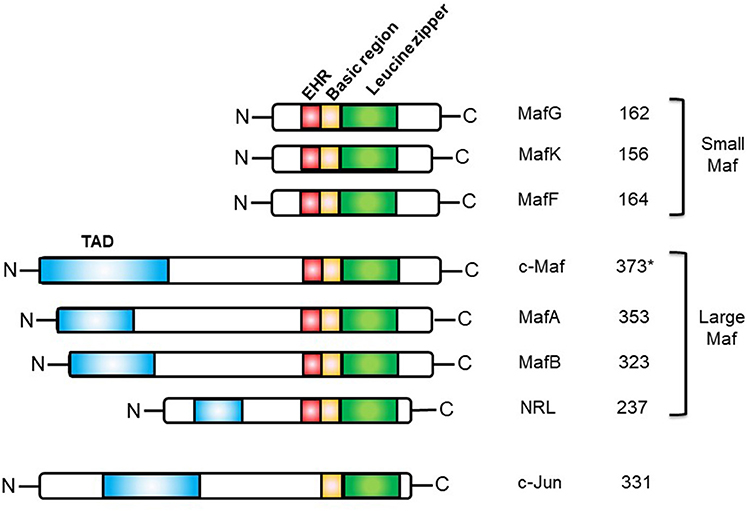
Figure 1. Structures of the human Maf family proteins and the c-Jun bZIP transcription factor. EHR, extended homology region; TAD, transactivation domain. *The short isoform (isoform 1) of human c-Maf is represented.
Thanks to their leucine zipper domain, Maf proteins can form homo- and heterodimers with other compatible bZIP proteins, such as Jun and Fos (24, 25). Maf proteins can also interact with other non-bZIP proteins including specific transcription factors, such as Sox family members (11).
Three isoforms exist for human c-Maf: a short form (373 amino acids), a medium form (383 amino acids), and a long form (30 amino acids more than the short form) of 38.5, 39.6, and 42 kDa respectively. In mice, only two isoforms have been reported: the long form (380 amino acids), called MAF-201, and the short form (370 amino acids). So far, distinct c-Maf products have not shown functional differences, but a potential functional specification cannot be excluded.
c-Maf is located on the chromosome 16q23.2 in humans and on chromosome 8 in mice (26, 27). c-Maf is translocated in 5–10% and/or overexpressed in 50% of multiple myelomas (MM) (28, 29). c-Maf overexpression in MM drives cyclin D2, integrin β7 and ARK5 expression and leads to proliferation, adhesion to bone marrow stroma cells, invasion, and migration of plasma cells (30). c-Maf is also highly expressed in over half of the angioimmunoblastic T-cell lymphomas (AITL) (30, 31). Transgenic overexpression of c-Maf in T cells regulates the same gene expression set as in plasma cells and induces T-cell lymphoma development in mice (30), therefore indicating that c-Maf is a bona fide oncogene contributing to the progression of hematological malignancies. c-Maf is also expressed by other cancers, such as renal or head and neck cancer, yet its expression is not systematically correlated with a bad prognosis (32).
Induction of c-Maf in T Cells
c-Maf expression and activity are regulated at transcriptional, post-transcriptional, as well as post-translational levels. Transcription factors and RNA-mediated silencing control the amount of Maf transcripts, while phosphorylation and SUMOylation modify the activity, sub-cellular localization, and half-life of the protein.
In T cells, antigenic stimuli that modulate the stability of the Maf encoding mRNAs and/or the c-Maf protein may also induce the expression of transcriptional activators, or may cooperate with independent transcriptional stimuli, such as cytokine-driven STAT factors to induce c-Maf transcription. The selective use of those pathways by different stimuli and in distinct cell populations provides the potential for tailoring c-Maf expression to different circumstances.
Transcriptional Regulation
In T cells, TCR stimulation induces the transcription of the Maf gene. However, distinct additional stimuli are required to sustain the expression of Maf, such as co-stimulatory signals (33) or the presence of cytokines, including IL-4 (34), IL-6 (35), TGF-β (36), and IL-27 (37) (Figure 2).
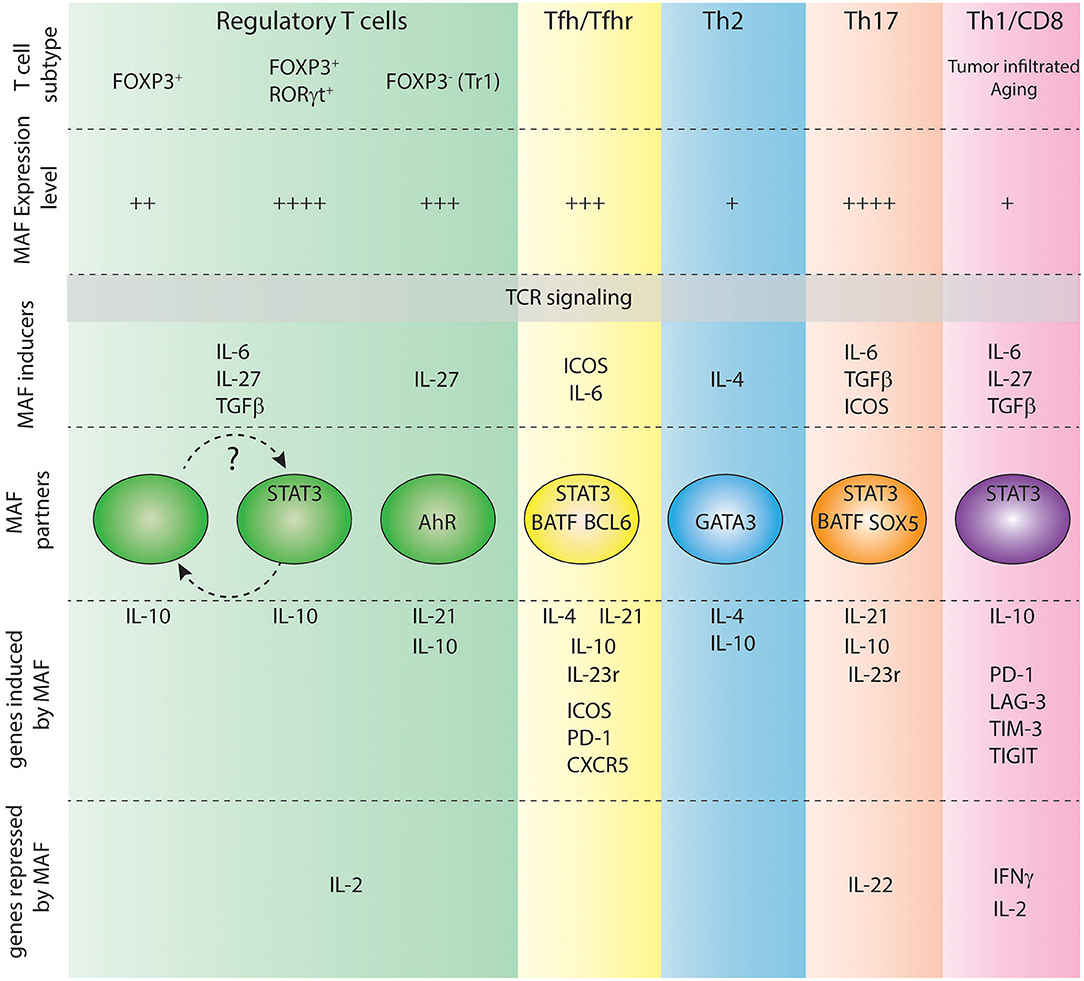
Figure 2. Role of c-Maf in various T cell subtypes. Level of expression of c-Maf, signals regulating its expression, partners of c-Maf and c-Maf target genes in each indicated T cell subtype are shown.
The highest levels of Maf transcripts can be detected in Th17 and Tfh cells. During Th17 cell polarization, both TGF-β and IL-6 are required for maximum induction of c-Maf, which in turn depends on STAT3 phosphorylation, but not STAT1 or STAT6 activation (35, 36, 38, 39). Moreover, after IL-6 stimulation, STAT3 binds to the promoter region of Maf in CD4 T cells and transactivates Maf in a luciferase reporter gene assay (35), thus positioning STAT3 as an important STAT transcription factor for c-Maf expression in T cells.
c-Maf expression was initially thought to rely on the IL-4/STAT6 signaling pathway during Th2 cell differentiation, as ectopic expression of activated STAT6 in Th1 cells promoted c-Maf expression, along with Th2-specific cytokines and GATA3 expression (34). However, introducing GATA3 into STAT6-deficient T cells restored c-Maf expression, therefore suggesting an indirect role of STAT6 in c-Maf induction during Th2 differentiation (40). Of note, the IL-6/STAT3 signaling pathway is central to c-Maf expression during Th2 cell development (35, 41). In particular, Th2 cells express activated forms of STAT3 downstream of a STAT6-signaling pathway. Ablation of STAT3 in developing Th2 cells does not preclude GATA3 and IL-4 expression but selectively impairs c-Maf expression (41). This STAT6-to-STAT3 signaling pathway thus reconciles previous contradictory results concerning the role of STAT6 in c-Maf induction and further supports an indirect role of STAT6 in c-Maf expression.
The inducible co-stimulator (ICOS), expressed by activated T cells, promotes expression of c-Maf in murine Th2 cells and in both mouse and human Th17 cells, although the molecular mechanisms beyond this induction are still ill-defined (33, 42–44).
IL-27, a member of the IL-12/IL-23 heterodimeric family of cytokines produced by APCs, is also a potent inducer of c-Maf during Tr1 cell differentiation (45). Interestingly, IL-27 signals through STAT1/STAT3 has been shown to up-regulate ICOS expression, thus activating two independent pathways that might up-regulate c-Maf.
Prostaglandin E2 (PGE2), a pro-inflammatory lipid mediator abundant at inflammatory sites, has recently been shown to inhibit c-Maf expression in developing Tr1 cells (46). PGE2 did not affect STAT1/3 activation and its inhibitory effect was mediated through the EP4 prostaglandin receptor and cAMP signaling (46).
The expression of a c-Maf specific intergenic long non-coding RNA, called linc-MAF-4, in Th1 cells inhibits Maf transcription through the recruitment and activation of chromatin-modifying complexes, including the PCR2-associated histone methyltransferase, enhancer of zeste homolog 2 (EZH2), and the lysine-specific histone demethylase 1A (LSD1) (47). Up-regulation of linc-MAF-4 in human CD4 T cells is directly involved in the down-regulation of Maf expression and correlates with encephalitogenic Th cell differentiation and annual relapse rate in patients with multiple sclerosis (48).
Post-transcriptional Regulation
c-Maf expression is tightly regulated by small non-coding microRNAs (miRNAs). In particular, Maf contains phylogenetically conserved miR-155 seed matches in its 3′-UTR. Luciferase reporter experiments confirmed that the c-Maf 3′-UTR is a direct target of miR-155 (49). MiR-155 is strongly expressed in activated T cells and genetic invalidation of miR-155 led to increased levels of c-Maf in T cell lines, thus positioning miR-155 as a major regulator of c-Maf expression in vivo (49). The role of miR-155 in suppressing c-Maf expression has been further extended to microglia cells during the response to CNS ischemia (50). c-Maf is also targeted by miR-143 and miR-365 in macrophages (51), and by miR-1290 in laryngeal carcinomas (52). However, expression of those miRNAs has not yet been reported in T lymphocytes.
Post-translational Control of the Biological Activity of c-Maf
Upon TCR activation, the CARMA1-dependent activation of the IKK complex results in the phosphorylation of the N-terminus part of c-Maf, which is required for nuclear translocation and binding to the promoter of target genes (53). In particular, the T cell-specific deficiency of either CARMA1 or its substrate IKKβ strongly reduced the DNA binding activity of c-Maf without affecting c-Maf abundance. This IKK-mediated activation of c-Maf is independent of NF-kB activation (53). Loss of CARMA1/IKK signaling resulted only in a partial decrease of c-Maf phosphorylation, suggesting that c-Maf might be phosphorylated by multiple kinases. c-Maf is phosphorylated by the Ser/Thr glycogen synthase kinase 3β (GSK3) in human multiple myeloma cell lines and in the lens, leading to protein stabilization (54, 55). However, whether GSK3 exerts a similar role in T cells is difficult to evaluate as GSK3 inhibition increases expression of c-Maf in this context (56).
Tyrosine phosphorylation of c-Maf is also critical for its recruitment to the IL-4 and IL-21 promoters and for optimal cytokine production. Phosphorylation of c-Maf on tyrosine residues has been shown to be positively and negatively regulated by the TEC tyrosine kinase and the PTPN22 tyrosine phosphatase, respectively (57).
SUMOylation of c-Maf at the lysine 33 residue reduces its ability to bind the Il4 promoter and decreases the transactivating activity of c-Maf in a luciferase reporter assay (58, 59). In addition, a recent report indicated that c-Maf SUMOylation is negatively correlated with Il21 expression in CD4 T cells from diabetogenic NOD mice (60). Furthermore, transgenic expression of a SUMO-defective c-Maf selectively inhibited recruitment of Daxx/HDAC2 to the Il21 promoter and enhanced histone acetylation mediated by CREB-binding protein (CBP) and p300. Thus, the SUMOylation status of c-Maf has a stronger regulatory effect on IL-21 than the level of c-Maf expression, through regulation of epigenetic mechanisms (60).
Roles of c-Maf IN T Helper Cells
Regulation of IL-10 Secretion in Multiple T Cell Subsets
Multiple roles have been attributed to c-Maf in distinct T cell subsets (Figure 2), revealing context-specific effects of this transcription factor. However, c-Maf positively regulates Il10 expression in virtually all immune cells, including T cells, B cells, macrophages, and dendritic cells (36, 37, 61–64), suggesting a common regulatory function beyond distinct T cell subset-specific roles.
IL-10 is an essential anti-inflammatory cytokine that plays important roles as a negative regulator of immune responses to foreign or self-antigens and prevents excessive inflammation during the course of infection [reviewed in (65–67)].
Exploring the role of c-Maf in three different disease models, each characterized by the predominant activity of a different T helper cell subset [malaria—Th1 cells; allergy to house dust mite—Th2 cells; experimental autoimmune encephalitis (EAE)—Th17 cells], Gabryšová et al. recently reported that Il10 expression was significantly lower in the absence of c-Maf, in T helper cells across all three diseases (67). The combined evidence of open chromatin (ATAC-seq analysis) coincident with binding of c-Maf to the Il10 locus (ChIP-seq analysis) confirmed c-Maf as a direct positive regulator of Il10 in vivo in distinct Th cell subsets (67).
c-Maf binds to consensus MARE motifs in the Il10 promoter (36, 37). Although c-Maf can transactivate Il10 by itself to some extent, c-Maf alone is not sufficient to induce optimal Il10 expression in T cells (36, 37). Robust IL-10 expression requires interaction with additional transcriptional regulators that vary among T cell subsets. c-Maf cooperates with the aryl hydrocarbon receptor (AhR) to induce IL-10 in regulatory type 1 (Tr1) cells (37). AhR expression is mainly driven by TGF-β (68) and is not expressed in Th1 cells, in which fine-tuning IL-10 expression mostly relies on the interaction of c-Maf with Blimp-1 (64). IL-10 expression in Th2 cells relies on transcription factors STAT6, GATA3, and IRF4 (69, 70) but whether these factors interact directly with c-Maf awaits further investigation.
Thus, the activity of c-Maf on the Il10 enhancer might not only depend on the accessibility of its motif but also on the nature of the other transcription factors that co-bind to that enhancer. In other words, Il10 expression in Th cells relies on several transcriptional programs that, together with c-Maf, are able to integrate various signals from the environment in order to fine-tune this critical immunosuppressive cytokine.
In addition to a direct positive transcriptional regulation of Il10 expression, c-Maf also provides a common mechanism for a negative regulation of IL-2 signaling in vivo in models of Th1, Th2, and Th17 responses (67).
However, although c-Maf uses common mechanisms of gene regulation in distinct cell subsets, the net outcome of c-Maf-deficiency is different in each cell type, thus indicating that c-Maf has context-specific effects on the immune response, over and above its effects on IL-10 and IL-2 signaling.
Context-Specific Effects of c-Maf on Th Cell Subset Function
Th1/Th2 Cells
The cross-regulation between Th1 and Th2 cells is mediated, in part, by the transcription factors that they express. c-Maf was first described as a Th2-specific gene that induces Il4 gene transcription via direct binding to the Il4 but not the Il5 or Il13 locus (71). Transcription factors GATA3, STAT6, and NFAT can synergize with c-Maf to regulate IL-4 expression in Th2 cells (72–74). Moreover, overexpression of c-Maf skews the immune response toward a Th2 response (75).
Although these pioneer studies concluded to a pro-Th2 role of c-Maf, normal levels of IL-13 and IgE were observed in c-Maf-deficient mice (72). Contrary to a pro-Th2 effect of c-Maf on the immune response, increased Th2 lung pathology, associated with higher numbers of eosinophils in bronchoalveolar lavage fluids, was observed in the HDM allergy model despite a decreased expression of Il4 in T-cell specific c-Maf deficient mice (67). Of note, cells producing both IL-4 and IL-10, but not IL-4+ IL-10− Th cells, were lost in this allergy model, in keeping with increased pathology. Thus, although c-Maf can activate the Il4 promoter, its net effect over the Th2 inflammatory response is mainly inhibitory.
In contrast with data obtained in naïve Th cells, ectopic expression of c-Maf in mature Th1 cells did not grant them the ability to produce IL-4, but did decrease their production of IFN-γ (75). In a recent study, chronically activated Th1 cells that were cultured with IL-4-producing Th2 cells up-regulate Maf expression (76). These cells were shown to down-regulate Ifng expression and express a dampened Th1 encephalitogenic cell capacity in vivo, despite normal expression of T-bet. Blockade of IL-4R signaling inhibited c-Maf expression in Th1 cells, suggesting that c-Maf may act downstream of IL-4R signaling to inhibit IFN-γ production in chronically activated Th1 cells (76). Thus, IL-4-driven expression of c-Maf in Th1 cells contributes to a transcriptional regulation program dampening their pathogenic immune response through altered cytokine profile.
In the malaria model, c-Maf-depletion led to greater acute-phase pathology, associated with enhanced expression of Tbx21 and production of IFN-γ (67). This suggests a wider role of c-Maf, i.e., dampening expression of the master transcription factor T-bet, in this experimental context. However, no direct binding of c-Maf to the Tbx21 locus was observed in the malaria model, indicating that c-Maf could regulate the expression of Tbx21 through indirect mechanisms. Gabryšová et al. showed that the chromatin landscape of Th1 cells is remodeled by c-Maf. They identified a strong enrichment of the Runx transcription factor-binding site in the remodeled loci and further observed increased Runx expression in c-Maf-deficient Th1 cells. Given the reported effects of Runx factors on IFN-γ production (77), it is tempting to postulate that c-Maf dampens Th1 cell differentiation at least partially via repression of Runx expression. Yet functional validation of this c-Maf/Runx control over Th1 pathology still awaits experimental testing.
Those c-Maf mediated changes in the chromatin landscape were not observed in the context of Th2 or Th17 cell pathologies, again showing that the role of c-Maf varies widely depending on T helper cell type.
Follicular Helper T Cells
Follicular helper T cells (Tfh) are key regulators of T cell-dependent long-term humoral immunity (78). Tfh cells express BCL6, a transcriptional repressor considered as the critical master regulator of Tfh cell development in vivo (79, 80), and also constitute the major source of IL-21, a cytokine necessary for IgG class-switch recombination and antibody affinity maturation (81).
Using retroviral ectopic expression of c-Maf or BCL6 in in vitro-derived human Tfh cells, Kroenke et al. first reported that c-Maf and BCL6 regulate distinct features of Tfh cell functions, with BCL6 required for Tfh cell development and c-Maf for promoting IL-21 secretion (82). However, recent data have shown that c-Maf is expressed early during Tfh cell differentiation and is critical for Tfh cell development in vivo (83). This is in agreement with the finding that Tfh cell differentiation strongly relies on ICOSL/ICOS and IL-6/STAT3 signaling, two pathways known to induce the expression of c-Maf (35, 84–86).
The relative roles of c-Maf, BCL6, and other transcription factors in initiating and maintaining Tfh cell are still poorly defined. As c-Maf-bound genes in Tfh cells hardly correlate with genes bound by BCL6 or Ascl2 (87, 88), it is tempting to speculate that cooperation between c-Maf and BCL6/Ascl2 is required to reach complete Tfh cell fate through orchestration of distinct sets of genes. Although c-Maf does not regulate Bcl6 transcription, a defect in BCL6 expression in CD4 T cells was observed in the absence of c-Maf, thus suggesting that c-Maf could contribute to BCL6 expression in developing Tfh cells (83).
Beside its role in Tfh cell development, c-Maf is also required for adequate IL-4 and IL-21 production through transactivation of the Il4 and Il21 promoters (82, 89, 90). In particular, Sahoo et al. reported that c-Maf promoted IL-4 secretion in Tfh cells through both direct binding to the CNS2 region in the Il4 locus and via induction of IRF4, thus revealing a distinct role of c-Maf in IL-4 secretion between Th2 and Tfh cell subsets (90).
Th17 Cells
c-Maf is highly expressed in Th17 cells and impacts several important aspects of their differentiation and function (33, 36, 39, 61, 64, 67, 91) (Figure 3). It physically associates with the transcription factor Sox5 and, together, they bind and activate the promoter of Rorc in conventional CD4 T cells. The Maf-Sox5 interaction thus controls Th17 development via the induction of RORγt as downstream targets of STAT3 (92). c-Maf also positively regulates certain loci in Th17 cells, including several genes known for controlling inflammation (e.g., Il9, Lif, Il10) (33, 37, 82, 89). c-Maf binds the Il21 promoter, inducing the production of IL-21 which subsequently sustains Th17 expansion (83) and stabilization through IL-23R expression (33). Some evidence also suggests that c-Maf-induced IL-21 secretion could trigger a positive feedback loop by activating STAT3, thus further promoting c-Maf and leading to the development of memory Th17 cells (93). Sato et al. suggested that c-Maf can also directly transactivate the Il23r gene which contains a MARE-like sequence (93). However, the relation between IL-23R and c-Maf is still controversial as it was recently reported that c-Maf downregulates Il23r expression in a subset of memory Th17 cells (91). c-Maf has been shown to act as a repressor of Il2 in CD4 T cells, which indirectly amounts to increased Th17 differentiation in EAE models. Indeed, T cell specific c-Maf deficiency led to an improvement of the disease through increased IL-2 production and decreased Th17 differentiation (67).
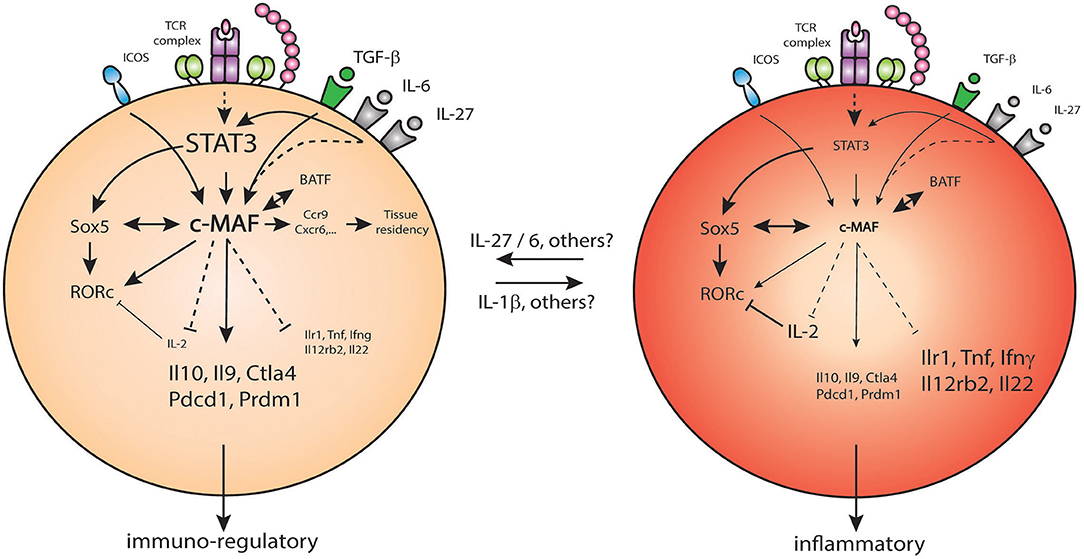
Figure 3. c-Maf regulates pro/anti-inflammatory properties of Th17 cells. Under the control of STAT3, c-Maf level in Th17 cells leads to differential expression of pro-inflammatory genes (Ilr1, Tnfa, Ifng,…) and immune-regulatory genes (Il10, Ctla4, Pdcd1,…) in Th17 cells.
c-Maf also acts as a global negative regulator of genes associated with Th17 function. This was demonstrated by an exhaustive study of the regulatory network for Th17 cell specification, where c-Maf attenuates the expression of pro-inflammatory loci (e.g., Rora, Runx1, Il1r1, Ccr6, Tnf) and repressing genes belonging to pathways regulated by other core transcription factors. c-Maf represses IL-22, but not IL-17A production, in a TGF-β-dependent way. It binds to the Il22 promoter and blocks the positive transcriptional effects of other Th17 transcription factors on the Il22 locus, including RORγt and BATF (39).
Importantly, c-Maf maintains tolerance through the regulation of IL-10, as described above. In Th17 cells, c-Maf regulates the balance between the differentiation toward inflammatory or anti-inflammatory Th17 cells (91). c-Maf controls this balance by binding to enhancers or putative enhancers already available in the general landscape of Th17 cells but not through the direct binding to promoters (91). It promotes an immuno-regulatory program (Il10, Ctla4) while repressing pro-inflammatory associated genes (Ifng, Il22, Il12rb2) (91). Interestingly, c-Maf also promotes the expression of genes associated with memory Th17 cell tissue-residency, such as Ccr9 and Cxcr6 (91).
The environmental cues guiding the transition between inflammatory and anti-inflammatory Th7 cells are still not clearly established. The presence of inflammatory cytokines, such as IL-1β, favors an inflammatory state, as described in Aschenbrenner et al. (91), without directly affecting the level of c-Maf in the cell. The levels of STAT3 activating cytokines, such as IL-6, IL-27, or IL-10, may have the opposite effect and push Th17 cells toward a more anti-inflammatory phenotype through c-Maf induction (36, 91). Altogether, c-Maf plays a critical role in Th17 differentiation and functions by balancing the inflammatory properties of these cells, and by doing so, it plays a critical role in the regulation of tolerance/inflammation.
Regulatory T Cells (Treg) and Gut Tolerance
Regulatory type 1 (Tr1) cells have emerged as an important subset of T cells with strong immunosuppressive properties but which does not express master transcription factor Forkhead box 3 (Foxp3), contrary to regulatory T cells (Tregs). The protective role of Tr1 cells has been shown in numerous contexts, such as autoimmunity, colitis, graft-versus-host disease, and tissue inflammation (94). The anti-inflammatory effects of Tr1 cells mainly rely on their ability to produce high amounts of IL-10. The cytokine IL-27 is known to promote the expansion and the differentiation of Tr1 cells through the induction of transcription factor c-Maf and co-stimulatory receptor ICOS. In these cells, c-Maf physically interacts with AhR to transactivate the Il10 and Il21 promoters (37, 45, 95). Of note, the expression of IL-21 further sustains c-Maf expression in Tr1 cells, as a feed-forward loop, thus highlighting the major role of c-Maf, both in the induction and the stabilization of the Tr1 cell fate (37, 95).
In Foxp3+ Tregs, c-Maf is expressed at various levels. The fraction of c-Maf+ cells found in Tregs depends on the organ studied. In the thymus, spleen, mesenteric lymph nodes or lungs, 5–40% of Foxp3+ Tregs express c-Maf. Strikingly, a large proportion, ranging from 60 to 80%, of Foxp3+ Tregs express c-Maf in the gut (38, 96–99). In lymphoid organs, c-Maf expression is restricted to effector Tregs (eTreg), which express high levels of CD44 and low levels of CD62L (96, 98, 99). c-Maf expression is also induced when naïve Tregs are stimulated in vitro with anti-CD3 and anti-CD28 antibodies (96).
Distinct Treg populations adopt specialized phenotypes by co-expressing Foxp3 and lineage-defining transcription factors in response to tissue- or inflammatory-driven signals (100). In particular, RORγt+ Tregs represent a subset of Tregs that develops in the intestinal tissue of naïve mice in response to signals arising from a complex microbiota. This subset of peripherally induced Tregs has been shown to efficiently protect from intestinal immunopathology in different colitis models (101, 102) and to mediate immunological tolerance to the gut pathobiont Helicobacter hepaticus (38). RORγt+ Tregs express high levels of c-Maf and genetic ablation of c-Maf leads to a severe defect in the development of this subset (38, 96–99).
Numerous studies published in the last couple of years highlighted the critical role of c-Maf in the regulation of gut homeostasis. As mentioned above, c-Maf promotes IL-10 production in distinct T cell subsets. Using IL-10 reporter mice, Neumann et al. recently showed that IL-10 production by Tregs was strictly associated with c-Maf expressing Tregs (96). IL-10 is critically involved in regulating intestinal homeostasis as its total invalidation (103) or T cell specific inactivation (104–107) led to the development of a strong colitis in mice. IL-10 regulation therefore underlies a pivotal aspect of c-Maf control over gut homeostasis. Of interest, c-Maf regulates the development of RORγt+ Tregs, which are the major source of IL-10 in this organ (38, 97). Thus, c-Maf might control IL-10 production in the intestine both directly, through regulation of Il10 gene transcription, and indirectly, via the differentiation of a high IL-10-producing Treg subset.
Genetic ablation of c-Maf also impairs the differentiation of follicular regulatory T cells (Tfr) in Peyer's patches (98). Tfr cells co-express both Tfh (CXCR5, PD-1, Bcl6) and Treg (Foxp3, CTLA4) markers and represent a regulatory T cell subset that controls the activity of Tfh cells. In the intestine, Tfr cells foster microbiota diversity via the regulation of symbiotic bacteria-specific IgA affinity (108). However, the functional consequences of Tfr cell deficiency in the gut homeostasis of mice harboring c-Maf-deficient Tregs requires further investigation.
Although the control exerted by c-Maf in gut homeostasis was clearly established by different groups, the extent of the c-Maf deficiency-driven inflammation varied drastically in distinct studies. Xu et al. reported that c-Maf inactivation in Tregs (MafΔTreg) induced a colitis with the apparition of a rectal prolapse in a third of the mice (38). This phenotype was observed after infection of the animals with the pathobiont Helicobacter hepaticus, but was much more reduced in the absence of infection. The same mouse model did not develop spontaneous colitis, and only showed mild signs of immune cell infiltration and colon tissue destruction in other studies that were not using the pathobiont (96, 97). Surprisingly, MafΔTreg mice were even protected from acute DSS induced colitis by an increased production of IL-17 and IL-22 (96). Lastly, c-Maf inactivation in all T cell compartments (MafΔT) resulted in a spontaneous strong onset of colitis (97).
This gradation in colitis development might be related to subtle differences in the microbiota composition of mice bred in distinct animal facilities and to a gradient of inflammation induced by the presence or not of certain pathobionts. But it might also reflect the role of c-Maf in multiple T cell subtypes in the gut (Figure 4).
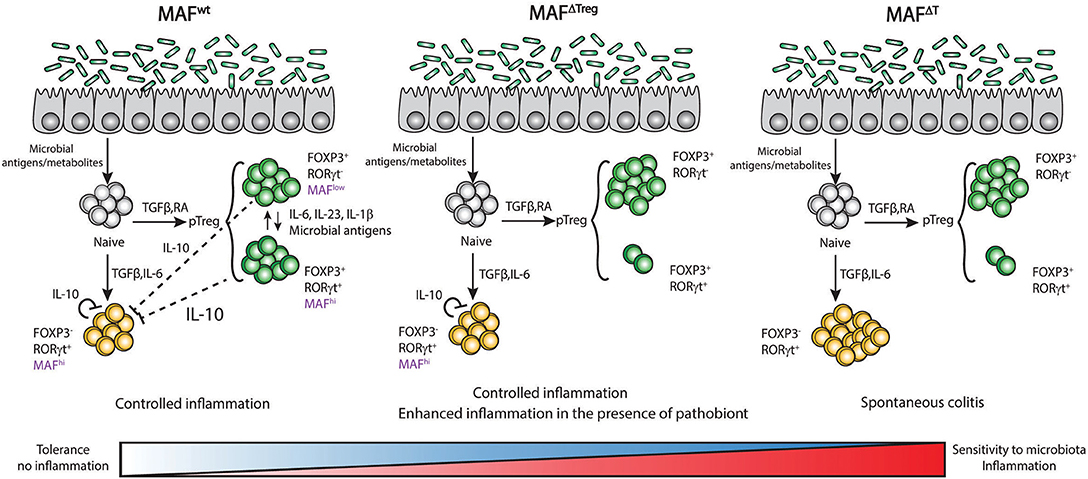
Figure 4. Expression of c-Maf in T cells regulates tolerance/sensitivity to microbiota in the gut. Expression of c-Maf in regulatory T cells (Foxp3+ RORγt− or Foxp3+ RORγt+) as well as in Th17 cells (RORγt+ Foxp3−) regulates the expression level of IL-10 in the colon and is central in the regulation of tolerance to gut microbiota.
The absence of RORγt+ Tregs in the gut of MafΔTreg mice strongly decreases the threshold for the development of colitis. However, unlike MafΔTreg mice, RORγtΔTreg mice do not spontaneously develop colitis, suggesting that c-Maf has a more substantial role than RORγt in the function of intestinal Tregs. Il10 transcripts are still detectable in the colon of MafΔTreg mice (97) and Imbratta et al. recently reported that Th17 cells from the colon express high levels of c-Maf and can produce IL-10. In MafΔT mice, this additional source of IL-10 is lost and the threshold for the development of colitis decreases further. This phenotype is lost when the animals are treated with antibiotics, further confirming that the microbiota plays a critical role in the development of colitis, probably through the induction of c-Maf and the development of RORγt+ Tregs in the colon (97). We can thus hypothesize that the presence of the microbiota induces a level of inflammation that triggers c-Maf expression in both intestinal Tregs and Th17 cells. c-Maf counterbalances this inflammation and promotes resistance to pathobionts through the production of IL-10 and/or the development of RORγt+ Tregs (Figure 4).
c-Maf inactivation strongly impaired IL-10 production in both Helios positive and negative Tregs in distinct organs, showing that c-Maf also regulates IL-10 production in Tregs of thymic and peripheral origin (96). However, ablation of c-Maf in the Treg or other T cell compartments did not result in systemic autoimmune disease, nor did it disturb conventional and regulatory T cell homeostasis in lymphoid organs, and c-Maf-deficient Tregs retained their in vitro suppressive capacity (38, 96, 98). Thus, although the precise role of c-Maf in the suppressive capacity of Tregs in distinct pathophysiological settings awaits further investigation, it seems that this transcription factor is a major player in colon homeostasis and that it drives immunological tolerance to gut pathobionts, thereby restraining inflammatory bowel disease.
Role of c-Maf in Tumor Infiltrating CD8 T Cells
c-Maf is not expressed in CD8 T cells at steady state. The expression of c-Maf in CD8 T cells was first described in tumor infiltrating lymphocytes (TILs) obtained from a mouse melanoma model and from melanoma patient (109). Overexpression of c-Maf in CD8 T cells leads to a strong repression of IFN-γ and IL-2 production, and to an increased expression of genes associated with T cell exhaustion, i.e., a dysfunctional state of T cells observed during chronic infections or in TILs (110, 111). These genes include Bcl6, Pdcd1 (PD-1), Stat3, and Il10, among other genes that are also described to be regulated by c-Maf in other T cell subtypes. When c-Maf was knocked-out in tumor specific CD8 T cells, those cells had a much higher capacity to restrain tumor growth through increased IFN-γ production and increased survival (109). Another study using B16 melanoma in mice confirmed that c-Maf was a major inducer of exhaustion associated genes, in cooperation with Prdm1 (Blimp-1). These two transcription factors had a compensative effect for the regulation of the transcription of many inhibitory receptors, such as PD-1, TIGIT, TIM-3, or LAG-3 (112). The double knockouts for c-Maf and Blimp-1 led to a better control of B16 growth, compared to single knockouts or WT mice (112).
Tc17 cells are a rather rare subtype of CD8 T cells found in gastrointestinal cancers as well as other diseases ranging from fungal infection to bacterial colonization of the skin and response to influenza (113). A study on mouse and human Tc17 cells showed that c-Maf and RORγt were both essential for the development of these cells (113). Similar to what is observed in Th cells, c-Maf expression in CD8 T cells is associated with increased tolerogenic/non-inflammatory functions, a mechanism, which is hijacked by the tumor microenvironment to favor immune escape and tumor development.
Role of c-Maf in Innate Lymphoid Cells and γδ T Cells
Innate lymphoid cells (ILCs) are tissue-resident cells which lack antigen specificity and are preprogrammed for effector function, poising them for rapid cytokine production and for tissular immune priming. Similar to T lymphocytes, ILCs can be classified into three specialized functional subclasses, characterized by the expression of lineage-defining transcription factors and effector cytokines (114). ILC1s are defined by T-bet (Tbx21) and IFN-γ production. ILC2s are characterized by their high GATA3 expression and IL-5 and IL-13 secretion. ILC3s are mainly located in the intestinal lamina propria and are defined by RORγt expression. They play a crucial role in maintaining epithelial barrier integrity by expressing large amounts of IL-22 and IL-17A (115). While c-Maf has been shown to be expressed early in ILC specification (116), it is preferentially expressed in intestinal ILC3s, particularly in CCR6− NKp46+ cells (17, 117).
ILCs show functional and phenotypic plasticity in response to the environment (118). This is exemplified by the ability of ILC3s to upregulate T-bet, lose RORγt expression, and convert to IFN-γ-producing ILC1s, also called “ex-ILC3s,” which are involved in intestinal pathology (119–121). Recent analysis of ILC transcriptional regulatory networks showed that c-Maf regulates the ILC3/ILC1 balance in the intestine (18). c-Maf directly promotes ILC3 identity by upregulating canonical type 3 c-Maf signature genes, common to NKp46+ ILC3s, CCR6+ ILC3s, IL-17-producing γδ T cells, and RORγt+ Tregs (17). Moreover, c-Maf indirectly sustains ILC3 identity by inducing CD127 expression, which strengthens the IL-7-dependent promotion of RORγt expression in ILCs (17). In turn, c-Maf directly represses ILC1 conversion by binding to the Tbx21 locus and inhibiting T-bet expression, and by restraining the type 1 chromatin accessibility landscape (17). Interestingly, this direct binding of c-Maf at the Tbx21 locus was not observed in Th1 cells and seems to be ILC-specific (67).
IL-17-producing RORγt+ γδ T (Tγδ17) cells are innate-like γδ T cells, functionally preprogrammed to produce IL-17, positioning them as the main IL-17-producer in the steady state intestine and in various inflammatory diseases (122, 123). A recent study showed that the collaboration between c-Maf and RORγt is required for Tγδ17 induction and maintenance (19). In a similar dynamic to that observed in ILC3s, c-Maf sustains Tγδ17 differentiation both by supporting activating locus modifications and by antagonizing TCF1 accessibility, a negative regulator of Tγδ17 differentiation.
c-Maf therefore emerges as a global type 3 innate cell insulator, which sustains the expression type 3 genes, while constraining the acquisition of a type 1 phenotype by directly repressing type 1 genes, such as Tbx21 and Tcf7, and by restraining chromatin accessibility. This feature distinguishes type 3 innate-like lymphoid cells from the adaptative Th17 cell subset, in which lineage-defining transcription factor expression and chromatin remodeling are regulated indirectly by c-Maf through negative regulation of the IL-2 signaling (67). c-Maf thus seems to occupy a higher position in the regulatory network controlling type 3 innate cells compared to that of Th17 cells.
Perspectives: Manipulating c-Maf Expression to Treat Patients?
In all T cell subtypes, c-Maf plays an important role in regulating tolerance and homeostasis. Targeting c-Maf expression or function could be a good opportunity to enhance or repress a given immune response. As c-Maf is overexpressed in 50% of multiple myeloma, several laboratories developed strategies to find some potent inhibitors, which could be used to enhance the immune response, in an anti-cancer setting for instance. USP5 is important to regulate the degradation of c-Maf (124). Molecules targeting this pathway, such as Mebendazole, showed some potent anti-myeloma activity by inhibiting the USP5/c-Maf axis (125). Another possible way to inhibit c-Maf activity is by preventing its dimerization. This could be achieved by the design of specific peptide able to destabilize homodimers of c-Maf (126). An older study described that glucocorticoids are potential inhibitors of c-Maf in multiple myeloma (127). However, using these compounds to manipulate the immune system is rather inappropriate, as glucocorticoids strongly dampen it. Lowering c-Maf expression at the cellular level before T cell transfer is also one possibility, with the use of miRNA or similar technologies.
Increasing c-Maf expression in Tregs would be of crucial interest to treat autoimmune diseases, especially in the gut where c-Maf is important in several T cell subtypes. The c-Maf expressing RORγt+ Foxp3+ Treg population is also present in human colon but do not show any variation in proportion of total CD4 T cells when comparing colon from patients with Crohn's disease and healthy tissues (128). How to manipulate and increase c-Maf expression and RORγt+ Tregs remains a challenge that requires further studies. The microbiota appears to play a central role in the regulation of this regulatory population (97, 101, 128, 129) and manipulating c-Maf expression might have dramatic impact on microbiota equilibrium. Thus, a better understanding of the c-Maf-driven Treg/Tfr/microbiota interplay would be required prior to envisage c-Maf-targeted immunotherapy.
Author Contributions
CI, HH, FA, and GV wrote the text and designed the figures.
Funding
This work was supported by the University of Lausanne, grants from the Swiss Cancer League (GV: 3679-08-2015), the Max Cloëtta Foundation (GV), the Swiss National Foundation (GV 310030_182680), the European Regional Development Fund (ERDF), the Walloon Region (Wallonia-Biomed portfolio, 411132-957270), and the Fonds Jean Brachet, and a research credit from the National Fund for Scientific Research, FNRS, Belgium. FA is a Research Associate at the FNRS, HH was supported by a Belgian FRIA fellowship.
Conflict of Interest
The authors declare that the research was conducted in the absence of any commercial or financial relationships that could be construed as a potential conflict of interest.
Acknowledgments
We thank all the members of our labs for their great support.
References
1. Enzinger FM, Shiraki, M. Musculo-aponeurotic fibromatosis of the shoulder girdle (extra-abdominal desmoid). Analysis of thirty cases followed up for ten or more years. Cancer. (1967) 20:1131–40.
2. Nishizawa M, Kataoka K, Goto N, Fujiwara KT, Kawai S. v-maf, a viral oncogene that encodes a “Leucine Zipper” motif. Proc Natl Acad Sci USA. (1989) 86:7711–5. doi: 10.1073/pnas.86.20.7711
3. Kawai S, Goto N, Kataoka K, Saegusa T, Shinno-Kohno H, Nishizawa M. Isolation of the avian transforming retrovirus, AS42, carrying the v-maf oncogene and initial characterization of its gene product. Virology. (1992) 188:778–84. doi: 10.1016/0042-6822(92)90532-T
4. Moussa WA, Tadros MD, Mekhael KG, Darwish AE, Shakir AH, El-Rehim EA. Some simple methods of home processing and their implication with weaning foods. Nahrung. (1992) 36:26–33. doi: 10.1002/food.19920360106
5. Kawauchi S, Takahashi S, Nakajima O, Ogino H, Morita M, Nishizawa M, et al. Regulation of lens fiber cell differentiation by transcription factor c-Maf. J Biol Chem. (1999) 274:19254–60. doi: 10.1074/jbc.274.27.19254
6. Ring BZ, Cordes SP, Overbeek PA, Barsh GS. Regulation of mouse lens fiber cell development and differentiation by the Maf gene. Development. (2000) 127:307–17.
7. Kim JI, Li T, Ho IC, Grusby MJ, Glimcher LH. Requirement for the c-Maf transcription factor in crystallin gene regulation and lens development. Proc Natl Acad Sci USA. (1999) 96:3781–5. doi: 10.1073/pnas.96.7.3781
8. Wende H, Lechner SG, Cheret C, Bourane S, Kolanczyk ME, Pattyn A, et al. The transcription factor c-Maf controls touch receptor development and function. Science. (2012) 335:1373–6. doi: 10.1126/science.1214314
9. Wende H, Lechner SG, Birchmeier C. The transcription factor c-Maf in sensory neuron development. Transcription. (2012) 3:285–9. doi: 10.4161/trns.21809
10. Imaki J, Tsuchiya K, Mishima T, Onodera H, Kim JI, Yoshida K, et al. Developmental contribution of c-maf in the kidney: distribution and developmental study of c-maf mRNA in normal mice kidney and histological study of c-maf knockout mice kidney and liver. Biochem Biophys Res Commun. (2004) 320:1323–7. doi: 10.1016/j.bbrc.2004.05.222
11. Huang W, Lu N, Eberspaecher H, De Crombrugghe B. A new long form of c-Maf cooperates with Sox9 to activate the type II collagen gene. J Biol Chem. (2002) 277:50668–75. doi: 10.1074/jbc.M206544200
12. Hong E, di Cesare PE, Haudenschild DR. Role of c-maf in chondrocyte differentiation: a review. Cartilage. (2011) 2:27–35. doi: 10.1177/1947603510377464
13. MacLean HE, Kim JI, Glimcher MJ, Wang J, Kronenberg HM, Glimcher LH. Absence of transcription factor c-maf causes abnormal terminal differentiation of hypertrophic chondrocytes during endochondral bone development. Dev Biol. (2003) 262:51–63. doi: 10.1016/S0012-1606(03)00324-5
14. Kusakabe M, Hasegawa K, Hamada M, Nakamura M, Ohsumi T, Suzuki H, et al. c-Maf plays a crucial role for the definitive erythropoiesis that accompanies erythroblastic island formation in the fetal liver. Blood. (2011) 118:1374–85. doi: 10.1182/blood-2010-08-300400
15. Tsuchiya M, Taniguchi S, Yasuda K, Nitta K, Maeda A, Shigemoto M, et al. Potential roles of large mafs in cell lineages and developing pancreas. Pancreas. (2006) 32:408–16. doi: 10.1097/01.mpa.0000220867.64787.99
16. Kataoka K, Shioda S, Ando K, Sakagami K, Handa H, Yasuda K. Differentially expressed Maf family transcription factors, c-Maf and MafA, activate glucagon and insulin gene expression in pancreatic islet alpha- and beta-cells. J Mol Endocrinol. (2004) 32:9–20. doi: 10.1677/jme.0.0320009
17. Parker ME, Barrera A, Wheaton JD, Zuberbuehler MK, Allan DSJ, Carlyle JR, et al. c-Maf regulates the plasticity of group 3 innate lymphoid cells by restraining the type 1 program. J Exp Med. (2020) 217:e20191030. doi: 10.1084/jem.20191030
18. Pokrovskii M, Hall JA, Ochayon DE, Yi R, Chaimowitz NS, Seelamneni H, et al. Characterization of transcriptional regulatory networks that promote and restrict identities and functions of intestinal innate lymphoid cells. Immunity. (2019) 51:185–97.e186. doi: 10.1016/j.immuni.2019.06.001
19. Zuberbuehler MK, Parker ME, Wheaton JD, Espinosa JR, Salzler HR, Park E, et al. The transcription factor c-Maf is essential for the commitment of IL-17-producing gammadelta T cells. Nat Immunol. (2019) 20:73–85. doi: 10.1038/s41590-018-0274-0
20. Liu M, Zhao X, Ma Y, Zhou Y, Deng M, Ma Y. Transcription factor c-Maf is essential for IL-10 gene expression in B cells. Scand J Immunol. (2018) 88:e12701. doi: 10.1111/sji.12701
21. Vinson C, Acharya A, Taparowsky EJ. Deciphering B-ZIP transcription factor interactions in vitro and in vivo. Biochim Biophys Acta. (2006) 1759:4–12. doi: 10.1016/j.bbaexp.2005.12.005
22. Kataoka K. Multiple mechanisms and functions of Maf transcription factors in the regulation of tissue-specific genes. J Biochem. (2007) 141:775–81. doi: 10.1093/jb/mvm105
23. Kerppola TK, Curran T. A conserved region adjacent to the basic domain is required for recognition of an extended DNA binding site by Maf/Nrl family proteins. Oncogene. (1994) 9:3149–58.
24. Kerppola TK, Curran T. Maf and Nrl can bind to AP-1 sites and form heterodimers with Fos and Jun. Oncogene. (1994) 9:675–84.
25. Kataoka K, Fujiwara KT, Noda M, Nishizawa M. MafB, a new Maf family transcription activator that can associate with Maf and Fos but not with Jun. Mol Cell Biol. (1994) 14:7581–91. doi: 10.1128/MCB.14.11.7581
26. Yoshida MC, Nishizawa M, Kataoka K, Goto N, Fujiwara KT, Kawai S. Localization of the human MAF protooncogene on chromosome 16 to bands q22-q23. (Abstract). Cytogenet Cell Genet. (1991) 58:2003.
27. Jamieson RV. Domain disruption and mutation of the bZIP transcription factor, MAF, associated with cataract, ocular anterior segment dysgenesis and coloboma. Hum Mol Genet. (2002) 11:33–42. doi: 10.1093/hmg/11.1.33
28. Chesi M, Bergsagel PL, Shonukan OO, Martelli ML, Brents LA, Chen T, et al. Frequent dysregulation of the c-maf proto-oncogene at 16q23 by translocation to an Ig locus in multiple myeloma. Blood. (1998) 91:4457–63. doi: 10.1182/blood.V91.12.4457.412k48_4457_4463
29. Hurt EM, Wiestner A, Rosenwald A, Shaffer AL, Campo E, Grogan T, et al. Overexpression of c-maf is a frequent oncogenic event in multiple myeloma that promotes proliferation and pathological interactions with bone marrow stroma. Cancer Cell. (2004) 5:191–9. doi: 10.1016/S1535-6108(04)00019-4
30. Morito N, Yoh K, Fujioka Y, Nakano T, Shimohata H, Hashimoto Y, et al. Overexpression of c-Maf contributes to T-cell lymphoma in both mice and human. Cancer Res. (2006) 66:812–9. doi: 10.1158/0008-5472.CAN-05-2154
31. Murakami YI, Yatabe Y, Sakaguchi T, Sasaki E, Yamashita Y, Morito N, et al. C-Maf expression in angioimmunoblastic T-cell lymphoma. Am J Surg Pathol. (2007) 31:1695–702. doi: 10.1097/PAS.0b013e318054dbcf
32. Uhlen M, Zhang C, Lee S, Sjöstedt E, Fagerberg L, Bidkhori G, et al. A pathology atlas of the human cancer transcriptome. Science. (2017) 357:eaan2507. doi: 10.1126/science.aan2507
33. Bauquet AT, Jin H, Paterson AM, Mitsdoerffer M, Ho I-C, Sharpe AH, et al. The costimulatory molecule ICOS regulates the expression of c-Maf and IL-21 in the development of follicular T helper cells and TH-17 cells. Nat Immunol. (2009) 10:167–175. doi: 10.1038/ni.1690
34. Kurata H, Lee HJ, O'Garra A, Arai N. Ectopic expression of activated Stat6 induces the expression of Th2-specific cytokines and transcription factors in developing Th1 cells. Immunity. (1999) 11:677–88. doi: 10.1016/S1074-7613(00)80142-9
35. Yang Y, Ochando J, Yopp A, Bromberg JS, Ding Y. IL-6 plays a unique role in initiating c-Maf expression during early stage of CD4 T cell activation. J Immunol. (2005) 174:2720–9. doi: 10.4049/jimmunol.174.5.2720
36. Xu J, Yang Y, Qiu G, Lal G, Wu Z, Levy DE, et al. c-Maf regulates IL-10 expression during Th17 polarization. J Immunol. (2009) 182:6226–36. doi: 10.4049/jimmunol.0900123
37. Apetoh L, Quintana FJ, Pot C, Joller N, Xiao S, Kumar D, et al. The aryl hydrocarbon receptor interacts with c-Maf to promote the differentiation of type 1 regulatory T cells induced by IL-27. Nat Immunol. (2010) 11:854–61. doi: 10.1038/ni.1912
38. Xu M, Pokrovskii M, Ding Y, Yi R, Au C, Harrison OJ, et al. c-MAF-dependent regulatory T cells mediate immunological tolerance to a gut pathobiont. Nature. (2018) 554:373–7. doi: 10.1038/nature25500
39. Rutz S, Noubade R, Eidenschenk C, Ota N, Zeng W, Zheng Y, et al. Transcription factor c-Maf mediates the TGF-beta-dependent suppression of IL-22 production in T(H)17 cells. Nat Immunol. (2011) 12:1238–45. doi: 10.1038/ni.2134
40. Ouyang W, Löhning M, Gao Z, Assenmacher M, Ranganath S, Radbruch A, et al. Stat6-independent GATA-3 autoactivation directs IL-4-independent Th2 development and commitment. Immunity. (2000) 12:27–37. doi: 10.1016/S1074-7613(00)80156-9
41. Mari N, Hercor M, Denanglaire S, Leo O, Andris F. The capacity of Th2 lymphocytes to deliver B-cell help requires expression of the transcription factor STAT3. Eur J Immunol. (2013) 43:1489–98. doi: 10.1002/eji.201242938
42. Coyle AJ, Lehar S, Lloyd C, Tian J, Delaney T, Manning S, et al. The CD28-related molecule ICOS is required for effective T cell-dependent immune responses. Immunity. (2000) 13:95–105. doi: 10.1016/S1074-7613(00)00011-X
43. Nurieva RI, Duong J, Kishikawa H, Dianzani U, Rojo JM, Ho IC, et al. Transcriptional regulation of Th2 differentiation by inducible costimulator. Immunity. (2003) 18:801–11. doi: 10.1016/S1074-7613(03)00144-4
44. Paulos CM, Paulos CM, Carpenito C, Plesa G, Suhoski MM, Varela-rohena A, et al. The inducible costimulator (ICOS) is critical for the development of human T H 17 cells. (2010) 2:55ra78. doi: 10.1126/scitranslmed.3000448
45. Pot C, Jin H, Awasthi A, Liu SM, Lai C-Y, Madan R, et al. Cutting edge: IL-27 induces the transcription factor c-Maf, cytokine IL-21, and the costimulatory receptor ICOS that coordinately act together to promote differentiation of IL-10-producing Tr1 cells. J Immunol. (2009) 183:797–801. doi: 10.4049/jimmunol.0901233
46. Hooper KM, Kong W, Ganea D. Prostaglandin E2 inhibits Tr1 cell differentiation through suppression of c-Maf. PLoS ONE. (2017) 12:e0179184. doi: 10.1371/journal.pone.0179184
47. Ranzani V, Rossetti G, Panzeri I, Arrigoni A, Bonnal RJ, Curti S, et al. The long intergenic noncoding RNA landscape of human lymphocytes highlights the regulation of T cell differentiation by linc-MAF-4. Nat Immunol. (2015) 16:318–25. doi: 10.1038/ni.3093
48. Zhang F, Liu G, Wei C, Gao C, Hao J. Linc-MAF-4 regulates Th1/Th2 differentiation and is associated with the pathogenesis of multiple sclerosis by targeting MAF. FASEB J. (2017) 31:519–25. doi: 10.1096/fj.201600838R
49. Rodriguez A, Vigorito E, Clare S, Warren MV, Couttet P, Soond DR, et al. Requirement of bic/microRNA-155 for normal immune function. Science. (2007) 316:608–11. doi: 10.1126/science.1139253
50. Su W, Hopkins S, Nesser NK, Sopher B, Silvestroni A, Ammanuel S, et al. The p53 transcription factor modulates microglia behavior through microRNA-dependent regulation of c-Maf. J Immunol. (2014) 192:358–66. doi: 10.4049/jimmunol.1301397
51. Tamgue O, Gcanga L, Ozturk M, Whitehead L, Pillay S, Jacobs R, et al. Differential targeting of c-Maf, Bach-1, and Elmo-1 by microRNA-143 and microRNA-365 promotes the intracellular growth of Mycobacterium tuberculosis in alternatively IL-4/IL-13 activated macrophages. Front Immunol. (2019) 10:421. doi: 10.3389/fimmu.2019.00421
52. Janiszewska J, Szaumkessel M, Kostrzewska-Poczekaj M, Bednarek K, Paczkowska J, Jackowska J, et al. Global miRNA expression profiling identifies miR-1290 as novel potential oncomiR in laryngeal carcinoma. PLoS ONE. (2015) 10:e0144924. doi: 10.1371/journal.pone.0144924
53. Blonska M, Joo D, Nurieva RI, Zhao X, Chiao P, Sun SC, et al. Activation of the transcription factor c-Maf in T cells is dependent on the CARMA1-IKKbeta signaling cascade. Sci Signal. (2013) 6:ra110. doi: 10.1126/scisignal.2004273
54. Qiang YW, Ye S, Chen Y, Buros AF, Edmonson R, van Rhee F, et al. MAF protein mediates innate resistance to proteasome inhibition therapy in multiple myeloma. Blood. (2016) 128:2919–30. doi: 10.1182/blood-2016-03-706077
55. Niceta M, Stellacci E, Gripp KW, Zampino G, Kousi M, Anselmi M, et al. Mutations impairing GSK3-mediated MAF phosphorylation cause cataract, deafness, intellectual disability, seizures, and a down syndrome-like facies. Am J Hum Genet. (2015) 96:816–25. doi: 10.1016/j.ajhg.2015.03.001
56. Hill EV, Ng TH, Burton BR, Oakley CM, Malik K, Wraith DC. Glycogen synthase kinase-3 controls IL-10 expression in CD4(+) effector T-cell subsets through epigenetic modification of the IL-10 promoter. Eur J Immunol. (2015) 45:1103–15. doi: 10.1002/eji.201444661
57. Liu CC, Lai CY, Yen WF, Lin YH, Chang HH, Tai TS, et al. Reciprocal regulation of C-Maf tyrosine phosphorylation by Tec and Ptpn22. PLoS ONE. (2015) 10:e0127617. doi: 10.1371/journal.pone.0127617
58. Leavenworth JW, Ma X, Mo YY, Pauza ME. SUMO conjugation contributes to immune deviation in nonobese diabetic mice by suppressing c-Maf transactivation of IL-4. J Immunol. (2009) 183:1110–9. doi: 10.4049/jimmunol.0803671
59. Lin BS, Tsai PY, Hsieh WY, Tsao HW, Liu MW, Grenningloh R, et al. SUMOylation attenuates c-Maf-dependent IL-4 expression. Eur J Immunol. (2010) 40:1174–84. doi: 10.1002/eji.200939788
60. Hsu CY, Yeh LT, Fu SH, Chien MW, Liu YW, Miaw SC, et al. SUMO-defective c-Maf preferentially transactivates Il21 to exacerbate autoimmune diabetes. J Clin Invest. (2018) 128:3779–93. doi: 10.1172/JCI98786
61. Ciofani M, Madar A, Galan C, Sellars M, Mace K, Pauli F, et al. A validated regulatory network for Th17 cell specification. Cell. (2012) 151:289–303. doi: 10.1016/j.cell.2012.09.016
62. Cao S, Liu J, Song L, Ma X. The Protooncogene c-Maf is an essential transcription factor for IL-10 gene expression in macrophages. J Immunol. (2005) 174:3484–92. doi: 10.4049/jimmunol.174.6.3484
63. Saraiva M, Christensen JR, Veldhoen M, Murphy TL, Murphy KM, O'Garra A. Interleukin-10 production by Th1 cells requires interleukin-12-induced STAT4 transcription factor and ERK MAP kinase activation by high antigen dose. Immunity. (2009) 31:209–19. doi: 10.1016/j.immuni.2009.05.012
64. Neumann C, Heinrich F, Neumann K, Junghans V, Mashreghi M-F, Ahlers J, et al. Role of Blimp-1 in programing Th effector cells into IL-10 producers. J Exp Med. (2014) 211:1807–19. doi: 10.1084/jem.20131548
65. Ouyang W, Rutz S, Crellin NK, Valdez PA, Hymowitz SG. Regulation and functions of the IL-10 family of cytokines in inflammation and disease. Annu Rev Immunol. (2011) 29:71–109. doi: 10.1146/annurev-immunol-031210-101312
66. Rojas JM, Avia M, Martin V, Sevilla N. IL-10: a multifunctional cytokine in viral infections. J Immunol Res. (2017) 2017:6104054. doi: 10.1155/2017/6104054
67. Gabryšová L, Alvarez-Martinez M, Luisier R, Cox LS, Sodenkamp J, Hosking C, et al. C-Maf controls immune responses by regulating disease-specific gene networks and repressing IL-2 in CD4+T cells article. Nat Immunol. (2018) 19:497–507. doi: 10.1038/s41590-018-0083-5
68. Veldhoen M, Hirota K, Westendorf AM, Buer J, Dumoutier L, Renauld JC, et al. The aryl hydrocarbon receptor links TH17-cell-mediated autoimmunity to environmental toxins. Nature. (2008) 453:106–9. doi: 10.1038/nature06881
69. Chang HD, Helbig C, Tykocinski L, Kreher S, Koeck J, Niesner U, et al. Expression of IL-10 in Th memory lymphocytes is conditional on IL-12 or IL-4, unless the IL-10 gene is imprinted by GATA-3. Eur J Immunol. (2007) 37:807–17. doi: 10.1002/eji.200636385
70. Ahyi AN, Chang HC, Dent AL, Nutt SL, Kaplan MH. IFN regulatory factor 4 regulates the expression of a subset of Th2 cytokines. J Immunol. (2009) 183:1598–606. doi: 10.4049/jimmunol.0803302
71. Ho IC, Hodge MR, Rooney JW, Glimcher LH. The proto-oncogene c-maf is responsible for tissue-specific expression of interleukin-4. Cell. (1996) 85:973–83. doi: 10.1016/S0092-8674(00)81299-4
72. Kim JI, Ho IC, Grusby MJ, Glimcher LH. The transcription factor c-Maf controls the production of interleukin-4 but not other Th2 cytokines. Immunity. (1999) 10:745–51. doi: 10.1016/S1074-7613(00)80073-4
73. Hodge MR, Chun HJ, Rengarajan J, Alt A, Lieberson R, Glimcher LH. NF-AT-driven interleukin-4 transcription potentiated by NIP45. Science. (1996) 274:1903–5. doi: 10.1126/science.274.5294.1903
74. Li B, Tournier C, Davis RJ, Flavell RA. Regulation of IL-4 expression by the transcription factor JunB during T helper cell differentiation. Eur Mol Biol Org J. (1999) 18:420–32. doi: 10.1093/emboj/18.2.420
75. Ho IC, Lo D, Glimcher LH. c-maf promotes T helper cell type 2 (Th2) and attenuates Th1 differentiation by both interleukin 4-dependent and -independent mechanisms. J Exp Med. (1998) 188:1859–66. doi: 10.1084/jem.188.10.1859
76. Mitchell RE, Hassan M, Burton BR, Britton G, Hill EV, Verhagen J, et al. IL-4 enhances IL-10 production in Th1 cells: implications for Th1 and Th2 regulation. Sci Rep. (2017) 7:11315. doi: 10.1038/s41598-017-11803-y
77. Djuretic IM, Levanon D, Negreanu V, Groner Y, Rao A, Ansel KM. Transcription factors T-bet and Runx3 cooperate to activate Ifng and silence Il4 in T helper type 1 cells. Nat Immunol. (2007) 8:145–53. doi: 10.1038/ni1424
78. Vinuesa CG, Linterman MA, Yu D, MacLennan IC. Follicular helper T cells. Annu Rev Immunol. (2016) 34:335–68. doi: 10.1146/annurev-immunol-041015-055605
79. Johnston RJ, Poholek AC, DiToro D, Yusuf I, Eto D, Barnett B, et al. Bcl6 and Blimp-1 are reciprocal and antagonistic regulators of T follicular helper cell differentiation. Science. (2009) 325:1006–10. doi: 10.1126/science.1175870
80. Nurieva RI, Chung Y, Martinez GJ, Yang XO, Tanaka S, Matskevitch TD, et al. Bcl6 mediates the development of T follicular helper cells. Science. (2009) 325:1001–5. doi: 10.1126/science.1176676
81. Leopold MF, Begeman L, van Bleijswijk JD, LL IJ, Witte HJ, Grone A. Exposing the grey seal as a major predator of harbour porpoises. Proc Biol Sci. (2015) 282:20142429. doi: 10.1098/rspb.2014.2429
82. Kroenke MA, Eto D, Locci M, Cho M, Davidson T, Haddad EK, et al. Bcl6 and Maf cooperate to instruct human follicular helper CD4 T cell differentiation. J Immunol. (2012) 188:3734–44. doi: 10.4049/jimmunol.1103246
83. Andris F, Denanglaire S, Anciaux M, Hercor M, Hussein H, Leo O. The transcription factor c-Maf promotes the differentiation of follicular helper T cells. Front Immunol. (2017) 8:480. doi: 10.3389/fimmu.2017.00480
84. Eddahri F, Denanglaire S, Bureau F, Spolski R, Leonard WJ, Leo O, et al. Interleukin-6/STAT3 signaling regulates the ability of naive T cells to acquire B-cell help capacities. Blood. (2009) 113:2426–33. doi: 10.1182/blood-2008-04-154682
85. Nurieva RI, Mai XM, Forbush K, Bevan MJ, Dong C. B7h is required for T cell activation, differentiation, and effector function. Proc Natl Acad Sci USA. (2003) 100:14163–8. doi: 10.1073/pnas.2335041100
86. Mak TW, Shahinian A, Yoshinaga SK, Wakeham A, Boucher LM, Pintilie M, et al. Costimulation through the inducible costimulator ligand is essential for both T helper and B cell functions in T cell-dependent B cell responses. Nat Immunol. (2003) 4:765–72. doi: 10.1038/ni947
87. Liu X, Chen X, Zhong B, Wang A, Wang X, Chu F, et al. Transcription factor achaete-scute homologue 2 initiates follicular T-helper-cell development. Nature. (2014) 507:513–8. doi: 10.1038/nature12910
88. Liu X, Lu H, Chen T, Nallaparaju KC, Yan X, Tanaka S, et al. Genome-wide analysis identifies Bcl6-controlled regulatory networks during T follicular helper cell differentiation. Cell Rep. (2016) 14:1735–47. doi: 10.1016/j.celrep.2016.01.038
89. Hiramatsu Y, Suto A, Kashiwakuma D, Kanari H, Kagami S-i, Ikeda K, et al. c-Maf activates the promoter and enhancer of the IL-21 gene, and TGF- inhibits c-Maf-induced IL-21 production in CD4+ T cells. J Leukoc Biol. (2010) 87:703–12. doi: 10.1189/jlb.0909639
90. Sahoo A, Alekseev A, Tanaka K, Obertas L, Lerman B, Haymaker C, et al. Batf is important for IL-4 expression in T follicular helper cells. Nat Commun. (2015) 6:7997. doi: 10.1038/ncomms8997
91. Aschenbrenner D, Foglierini M, Jarrossay D, Hu D, Weiner HL, Kuchroo VK, et al. An immunoregulatory and tissue-residency program modulated by c-MAF in human TH17 cells. Nat Immunol. (2018) 19:1126–36. doi: 10.1038/s41590-018-0200-5
92. Tanaka S, Suto A, Iwamoto T, Kashiwakuma D, Kagami S-i, Suzuki K, et al. Sox5 and c-Maf cooperatively induce Th17 cell differentiation via RORγt induction as downstream targets of Stat3. J Exp Med. (2014) 211:1857–74. doi: 10.1084/jem.20130791
93. Sato K, Miyoshi F, Yokota K, Araki Y, Asanuma Y, Akiyama Y, et al. Marked induction of c-Maf protein during Th17 cell differentiation and its implication in memory Th cell development. J Biol Chem. (2011) 286:14963–71. doi: 10.1074/jbc.M111.218867
94. Roncarolo MG, Gregori S, Battaglia M, Bacchetta R, Fleischhauer K, Levings MK. Interleukin-10-secreting type 1 regulatory T cells in rodents and humans. Immunol Rev. (2006) 212:28–50. doi: 10.1111/j.0105-2896.2006.00420.x
95. Pot C, Apetoh L, Kuchroo VK. Type 1 regulatory T cells (Tr1) in autoimmunity. Semin Immunol. (2011) 23:202–8. doi: 10.1016/j.smim.2011.07.005
96. Neumann C, Blume J, Roy U, Teh PP, Vasanthakumar A, Beller A, et al. c-Maf-dependent Treg cell control of intestinal TH17 cells and IgA establishes host-microbiota homeostasis. Nat Immunol. (2019) 20:471–81. doi: 10.1038/s41590-019-0316-2
97. Imbratta C, Leblond MM, Bouzourene H, Speiser DE, Velin D, Verdeil G. Maf deficiency in T cells dysregulates Treg - TH17 balance leading to spontaneous colitis. Sci Rep. (2019) 9:6135. doi: 10.1038/s41598-019-42486-2
98. Wheaton JD, Yeh CH, Ciofani M. Cutting edge: c-Maf is required for regulatory T cells to adopt RORgammat(+) and follicular phenotypes. J Immunol. (2017) 199:3931–6. doi: 10.4049/jimmunol.1701134
99. Hussein HDS, Van Gool F, Azouz A, Ajouaou Y, El-Khatib H, Oldenhove G, et al. Multiple environmental signaling pathways control the differentiation of RORγt-expressing regulatory T cells. Front Immunol. (2019) 10:3007. doi: 10.3389/fimmu.2019.03007
100. Panduro M, Benoist C, Mathis D. Tissue tregs. Annu Rev Immunol. (2016) 34:609–33. doi: 10.1146/annurev-immunol-032712-095948
101. Ohnmacht C, Park JH, Cording S, Wing JB, Atarashi K, Obata Y, et al. Mucosal immunology. The microbiota regulates type 2 immunity through RORgammat(+) T cells. Science. (2015) 349:989–93. doi: 10.1126/science.aac4263
102. Lochner M, Ohnmacht C, Presley L, Bruhns P, Si-Tahar M, Sawa S, et al. Microbiota-induced tertiary lymphoid tissues aggravate inflammatory disease in the absence of RORgamma t and LTi cells. J Exp Med. (2011) 208:125–34. doi: 10.1084/jem.20100052
103. Kühn R, Löhler J, Rennick D, Rajewsky K, Müller W. Interleukin-10-deficient mice develop chronic enterocolitis. Cell. (1993) 75:263–74. doi: 10.1016/0092-8674(93)80068-P
104. Roers A, Siewe L, Strittmatter E, Deckert M, Schlüter D, Stenzel W, et al. T cell–specific inactivation of the interleukin 10 gene in mice results in enhanced T cell responses but normal innate responses to lipopolysaccharide or skin irritation. J Exp Med. (2004) 200:1289–97. doi: 10.1084/jem.20041789
105. Huber S, Gagliani N, Esplugues E, O'Connor W Jr, Huber FJ, Chaudhry A, et al. Th17 cells express interleukin-10 receptor and are controlled by Foxp3(-) and Foxp3+ regulatory CD4+ T cells in an interleukin-10-dependent manner. Immunity. (2011) 34:554–65. doi: 10.1016/j.immuni.2011.01.020
106. Rubtsov YP, Rasmussen JP, Chi EY, Fontenot J, Castelli L, Ye X, et al. Regulatory T cell-derived interleukin-10 limits inflammation at environmental interfaces. Immunity. (2008) 28:546–58. doi: 10.1016/j.immuni.2008.02.017
107. Chaudhry A, Samstein RM, Treuting P, Liang Y, Pils MC, Heinrich J-m, et al. Interleukin-10 signaling in regulatory T cells is required for suppression of Th17 cell-mediated inflammation. Immunity. (2011) 34:566–78. doi: 10.1016/j.immuni.2011.03.018
108. Rescigno M. Tfr cells and IgA join forces to diversify the microbiota. Immunity. (2014) 41:9–11. doi: 10.1016/j.immuni.2014.06.012
109. Giordano M, Henin C, Maurizio J, Imbratta C, Bourdely P, Buferne M, et al. Molecular profiling of CD8 T cells in autochthonous melanoma identifies Maf as driver of exhaustion. EMBO J. (2015) 34:2042–58. doi: 10.15252/embj.201490786
110. Speiser DE, Ho PC, Verdeil G. Regulatory circuits of T cell function in cancer. Nat Rev Immunol. (2016) 16:599–611. doi: 10.1038/nri.2016.80
112. Chihara N, Madi A, Kondo T, Zhang H, Acharya N, Singer M, et al. Induction and transcriptional regulation of the co-inhibitory gene module in T cells. Nature. (2018) 558:454–9. doi: 10.1038/s41586-018-0206-z
113. Mielke LA, Liao Y, Clemens EB, Firth MA, Duckworth B, Huang Q, et al. TCF-1 limits the formation of Tc17 cells via repression of the MAF-RORgammat axis. J Exp Med. (2019) 216:1682–99. doi: 10.1084/jem.20181778
114. Vivier E, Artis D, Colonna M, Diefenbach A, Di Santo JP, Eberl G, et al. Innate lymphoid cells: 10 years on. Cell. (2018) 174:1054–66. doi: 10.1016/j.cell.2018.07.017
115. Sawa S, Lochner M, Satoh-Takayama N, Dulauroy S, Berard M, Kleinschek M, et al. RORgammat+ innate lymphoid cells regulate intestinal homeostasis by integrating negative signals from the symbiotic microbiota. Nat Immunol. (2011) 12:320–6. doi: 10.1038/ni.2002
116. Harly C, Cam M, Kaye J, Bhandoola A. Development and differentiation of early innate lymphoid progenitors. J Exp Med. (2018) 215:249–62. doi: 10.1084/jem.20170832
117. Gury-BenAri M, Thaiss CA, Serafini N, Winter DR, Giladi A, Lara-Astiaso D, et al. The spectrum and regulatory landscape of intestinal innate lymphoid cells are shaped by the microbiome. Cell. (2016) 166:1231–46.e1213. doi: 10.1016/j.cell.2016.07.043
118. Colonna M. Innate lymphoid cells: diversity, plasticity, and unique functions in immunity. Immunity. (2018) 48:1104–17. doi: 10.1016/j.immuni.2018.05.013
119. Song C, Lee JS, Gilfillan S, Robinette ML, Newberry RD, Stappenbeck TS, et al. Unique and redundant functions of NKp46+ ILC3s in models of intestinal inflammation. J Exp Med. (2015) 212:1869–82. doi: 10.1084/jem.20151403
120. Buonocore S, Ahern PP, Uhlig HH, Ivanov II, Littman DR, Maloy KJ, et al. Innate lymphoid cells drive interleukin-23-dependent innate intestinal pathology. Nature. (2010) 464:1371–5. doi: 10.1038/nature08949
121. Klose CS, Kiss EA, Schwierzeck V, Ebert K, Hoyler T, d'Hargues Y, et al. A T-bet gradient controls the fate and function of CCR6-RORgammat+ innate lymphoid cells. Nature. (2013) 494:261–5. doi: 10.1038/nature11813
122. Papotto PH, Ribot JC, Silva-Santos B. IL-17(+) gammadelta T cells as kick-starters of inflammation. Nat Immunol. (2017) 18:604–11. doi: 10.1038/ni.3726
123. Cua DJ, Tato CM. Innate IL-17-producing cells: the sentinels of the immune system. Nat Rev Immunol. (2010) 10:479–89. doi: 10.1038/nri2800
124. Wang S, Juan J, Zhang Z, Du Y, Xu Y, Tong J, et al. Inhibition of the deubiquitinase USP5 leads to c-Maf protein degradation and myeloma cell apoptosis. Cell Death Dis. (2017) 8:e3058. doi: 10.1038/cddis.2017.450
125. Chen XH, Xu YJ, Wang XG, Lin P, Cao BY, Zeng YY, et al. Mebendazole elicits potent antimyeloma activity by inhibiting the USP5/c-Maf axis. Acta Pharmacol Sin. (2019) 40:1568–77. doi: 10.1038/s41401-019-0249-1
126. Pellegrino S, Ronda L, Annoni C, Contini A, Erba E, Gelmi ML, et al. Molecular insights into dimerization inhibition of c-Maf transcription factor. Biochim Biophys Acta. (2014) 1844:2108–15. doi: 10.1016/j.bbapap.2014.09.003
127. Mao X, Stewart AK, Hurren R, Datti A, Zhu X, Zhu Y, et al. A chemical biology screen identifies glucocorticoids that regulate c-maf expression by increasing its proteasomal degradation through up-regulation of ubiquitin. Blood. (2007) 110:4047–54. doi: 10.1182/blood-2007-05-088666
128. Sefik E, Geva-Zatorsky N, Oh S, Konnikova L, Zemmour D, McGuire AM, et al. Mucosal immunology. Individual intestinal symbionts induce a distinct population of RORgamma(+) regulatory T cells. Science. (2015) 349:993–7. doi: 10.1126/science.aaa9420
Keywords: T cells, tolerance, c-Maf, interleukin-10, gut
Citation: Imbratta C, Hussein H, Andris F and Verdeil G (2020) c-MAF, a Swiss Army Knife for Tolerance in Lymphocytes. Front. Immunol. 11:206. doi: 10.3389/fimmu.2020.00206
Received: 16 December 2019; Accepted: 27 January 2020;
Published: 14 February 2020.
Edited by:
Remy Bosselut, National Cancer Institute (NCI), United StatesReviewed by:
Michele Kay Anderson, University of Toronto, CanadaBooki Min, Case Western Reserve University, United States
Copyright © 2020 Imbratta, Hussein, Andris and Verdeil. This is an open-access article distributed under the terms of the Creative Commons Attribution License (CC BY). The use, distribution or reproduction in other forums is permitted, provided the original author(s) and the copyright owner(s) are credited and that the original publication in this journal is cited, in accordance with accepted academic practice. No use, distribution or reproduction is permitted which does not comply with these terms.
*Correspondence: Fabienne Andris, fabienne.andris@ulb.be; Grégory Verdeil, gregory.verdeil@unil.ch
†These authors share last authorship