- 1Institut de la Vision, Sorbonne Université, INSERM, CNRS, Paris, France
- 2Université de Tours, Inserm, N2C UMR 1069, Faculté de Médecine, Tours, France
- 3Sorbonne Université, Inserm, CNRS, Centre d'Immunologie et des Maladies Infectieuses, Cimi-Paris, Paris, France
- 4Department of Dentistry, University of Alberta, Edmonton, AB, Canada
- 5Faculty of Pharmacy, Université de Montréal, Montreal, QC, Canada
- 6Departments of Pediatrics, Ophthalmology and Pharmacology, Université de Montréal, Montreal, QC, Canada
Background: CD36, a member of the class B scavenger receptor family, participates in Toll-like receptor signaling on mononuclear phagocytes (MP) and can promote sterile pathogenic inflammation. We here analyzed the effect of CD36 deficiency on retinal inflammation and photoreceptor degeneration, the hallmarks of age-related macular degeneration (AMD), that characterize Cx3cr1−/−mice.
Methods: We analyzed subretinal MP accumulation, and cone- and rod-degeneration in light-challenged and aged, CD36 competent or deficient, hyper-inflammatory Cx3cr1−/− mice, using histology and immune-stained retinal flatmounts. Monocytes (Mo) were subretinally adoptively transferred to evaluate their elimination rate from the subretinal space and Interleukin 6 (IL-6) secretion from cultured Mo-derived cells (MdCs) of the different mouse strains were analyzed.
Results: CD36 deficient Cx3cr1−/− mice were protected against age- and light-induced subretinal inflammation and associated cone and rod degeneration. CD36 deficiency in Cx3cr1−/− MPs inhibited their prolonged survival in the immune-suppressive subretinal space and reduced the exaggerated IL-6 secretion observed in Cx3cr1−/− MPs that we previously showed leads to increased subretinal MP survival.
Conclusion: Cd36 deficiency significantly protected hyperinflammatory Cx3cr1−/− mice against subretinal MP accumulation and associated photoreceptor degeneration. The observed CD36-dependent induction of pro-inflammatory IL-6 might be at least partially responsible for the prolonged MP survival in the immune-suppressive environment and its pathological consequences on photoreceptor homeostasis.
Introduction
Age-related Macular Degeneration (AMD) is a common (1), highly heritable, neuroinflammatory disorder characterized by central, sizeable deposits under the retinal pigment epithelium (drusen) in its early form and choroidal neovascularisation (CNV, wet AMD) or an extending lesion of the outer central retina (geographic atrophy) in its late form (2).
We (3–6) and others (7, 8) showed that both advanced forms [wet AMD (5) and Geographic Atrophy, GA (3, 4, 6)] are associated with non-resolving accumulation of mononuclear phagocytes (MP) in the subretinal space. MPs are a family of cells that include monocyte (Mo), resident macrophages (rMϕ) such as microglial cells (MC), and monocyte-derived inflammatory macrophages/dendritic cells (MdC) that arise during inflammation (9). Physiologically, the subretinal space does not contain blood- and lymphatic-vessels, and is additionally devoid of immune cells, including resident MCs (4, 5, 7, 8, 10). Among others this particularity is due to the potent immunosuppressive, pro-apoptotic factors produced by the RPE that eliminate infiltrating leukocytes (4, 11). While MP accumulation observed around large drusen in early AMD (4, 6) might control debris accumulation, we showed that MdCs invariably participate in the infiltrate in and around GA lesions (6), where they are closely associated with rod and cone degeneration (3). Indeed, MPs, and in particular MdCs, an important source of inflammatory cytokines that play a critical role in animal models of pathological choroidal neovascularisation (CNV) (12, 13) and photoreceptor degeneration (5, 6, 14–18). In particular we showed that the inflammatory cytokines CCL2, IL-6, and IL-1 lead to the excessive MdC accumulation, diminish the subretinal immunosuppressivity and induce photoreceptor degeneration and CNV, respectively (3, 4, 6, 18, 19).
In the eye, CX3CL1 is constitutively expressed as a transmembrane protein in inner retinal neurons (20, 21) and provides a tonic inhibitory signal to CX3CR1 bearing retinal MCs that keeps these cells in a quiescent surveillance mode under physiological conditions (5, 22). Cx3cr1 deficiency in mice leads to a strong increase of subretinal MP accumulation with age, after light-challenge and laser-injury (5, 23, 24), in diabetes (25), and in a paraquat-induced retinopathy model (26).
We showed that Cx3cr1-deficient MPs express high levels of Apolipoprotein E, also observed in humanized transgenic mice expressing the AMD-risk APOE2 isoform (TRE2-mice) and subretinal MPs of AMD patients (4, 6, 27). The excessive expression of APOE in turn can activate the CD14/TLR2/TLR4-dependent innate immunity receptor complex (IIRC) on MPs (4, 27), likely by modifying the cholesterol content of the lipid raft, in which the complex is located (28). Its activation in subretinal MPs induces CCL2 (27), which increases Mo recruitment (6), but also IL-6, which we show reduces RPE FasL expression and MP elimination (4). The age-dependent accumulation of subretinal Cx3cr1-deficient MPs is associated with a significant degeneration of rods and cones (5, 29, 30), but not with RPE atrophy. They therefore quite accurately model the transitional zone of GA and a subtype of GA called incomplete outer retinal atrophy [iORA; (31)].
CD36, a member of the class B scavenger receptor family, is expressed in a variety of cell types and binds a diverse array of ligands (32). It is expressed on Mϕ, MC, RPE cells, and microvascular endothelial cells (32), all cell types potentially involved in AMD. On vascular endothelial cells CD36 has been shown to mediate the antiproliferative effect of the thrombospondins (33, 34). Its expression on RPE cells has been shown to influence the phagocytosis of rod outer segments (ROS) (35, 36), in particular oxydized ROS (37, 38), but also oxydized lipoproteins (39), whose CD36-mediated uptake activates the NLRP3 inflammasome in the RPE (40). Finally, it is expressed on MC and Mϕ where it is a co-receptor of the toll-like receptor 2 (TLR2) and activates a proinflammatory signaling cascade and the release of inflammatory cytokines (41, 42). On the other hand, internalization of apoptotic bodies via CD36 can inhibit proinflammatory pathways (43, 44).
We have previously shown that CD36 expression regulates COX-2 and VEGF expression in RPE cells. Its deficiency leads to a slow, age-related choroidal involution and mild retinal degeneration (45). On the other hand deficiency of CD36 protected against subretinal inflammation and photoreceptor degeneration in an acute model of light toxicity, as it inhibited the release of neurotoxic IL-1beta from subretinal MPs (42).
Here we investigated the influence of CD36 on inflammation and photoreceptor degeneration in Cx3cr1-deficient mice that we previously showed develop pathogenic subretinal MP accumulation with age and after a non-toxic light-challenge.
Results
CD36 Deficiency Prevents Age-Related, Chronic, Pathogenic Subretinal Inflammation in Cx3cr1-Knockout Mice
We first sought to determine whether Cd36 deficiency would alter disease onset and progression in Cx3cr1−/− mice. Quantification of subretinal IBA-1+ MPs on retinal and RPE/choroidal flatmounts of 2–3 month and 12 month old animals showed that the age-related increase in subretinal MPs observed in Cx3cr1−/− mice was significantly inhibited in the absence of CD36 (Figures 1A,B). Next, we evaluated whether Cd36 deficiency influenced the rod and cone degeneration that is associated with MP accumulation in Cx3cr1−/− mice (5, 6, 29). Micrographs revealed that Cx3cr1−/−Cd36−/− mice were protected against the thinning of the outer nuclear layer that hosts the photoreceptor nuclei, which is observed in aged Cx3cr1-deficient mice (6) (Figure 2A). Photoreceptor nuclei row counts (Figure 2B) and calculation of the area under the curve showed CD36 deficiency protected against the photoreceptor cell loss observed in Cx3cr1−/− mice (Figure 2C), while only a slight decrease was observed in CD36−/− compared to control-mice. Similarly, CD36 deficiency completely protected against cone loss observed on peanut agglutinin stained retinal flatmounts of 12 month-old Cx3cr1−/− mice (Figures 2D–F). Thus, we show that CD36 contributes importantly to the chronic, age-related subretinal MP accumulation and associated photoreceptor degeneration observed in Cx3cr1−/− mice.
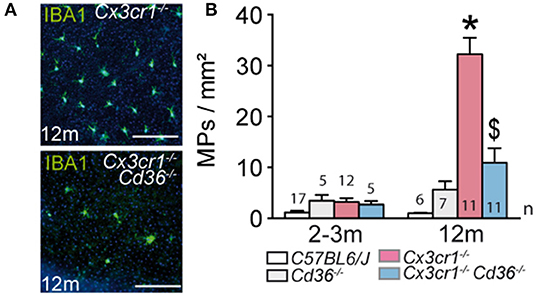
Figure 1. CD36 deficiency prevents age-related chronic subretinal inflammation in Cx3cr1-knockout mice. (A) Representative images of 12 month old IBA-1 (green) stained RPE flatmounts of 12 months old Cx3cr1−/− and Cx3cr1−/−Cd36−/− mice. (B) Quantification of subretinal IBA-1+MPs in 2-3 months (left) and 12 months (right) old mice of the indicated strains (*Mann & Whitney test at 12 months of Cx3cr1−/− vs. 3 months old Cx3cr1−/− mice p = 0.0002; $Mann & Whitney test at 12 months of Cx3cr1−/− vs. 12 months old Cx3cr1−/−Cd36−/− mice p < 0.0001). n = number of replicates indicated in the graphs; replicates represent quantification of eyes from different mice of at least three different cages. Scale bar (A) = 50 m.
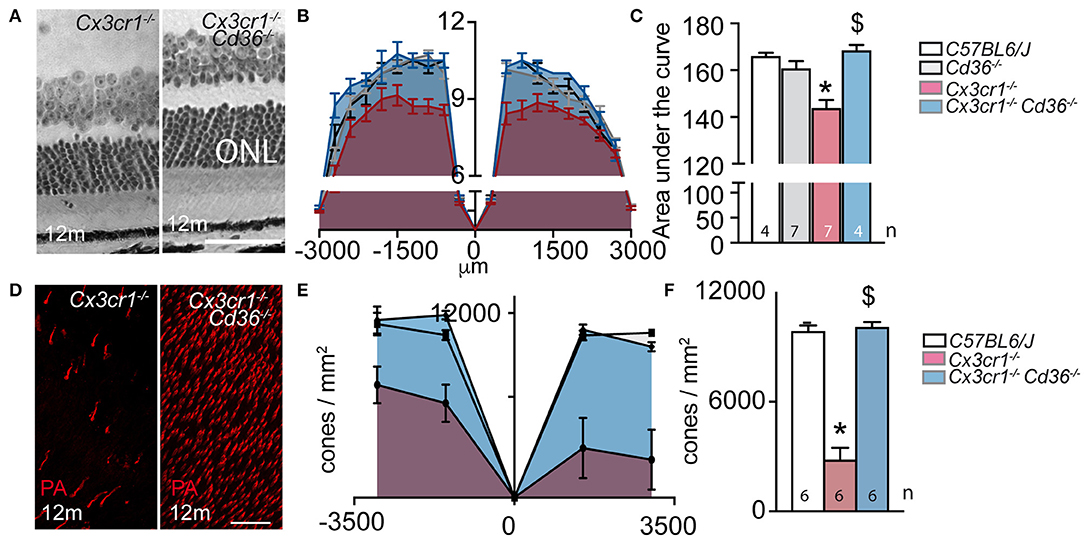
Figure 2. CD36 deficiency prevents age-related rod and cone degeneration in Cx3cr1-knockout mice. (A) Micrographs, taken 1,000 μm from the optic nerve, of 12 month-old Cx3cr1−/− and Cx3cr1−/−Cd36−/− mice. (B) Photoreceptor nuclei rows at increasing distances (−3,000 μm: inferior pole, +3,000 μm: superior pole) from the optic nerve (0 μm) in 12 month-old mice. (C) Quantification of the area under the curve of photoreceptor nuclei row counts of 12 month-old mice of the indicated transgenic mouse strains (Mann Whitney wildtype vs. Cx3cr1−/− mice *p = 0.0024; Mann Whitney Cx3cr1−/− vs. Cx3cr1−/−Cd36−/− mice $p = 0.0024). (D) Micrographs, taken in the superior periphery of peanut agglutinin (staining cone segments, red) stained 12 month-old Cx3cr1−/− and Cx3cr1−/−Cd36−/− mice. (E) Cone density quantification on retinal flatmounts in peripheral and central, inferior and superior retina (−3,000 μm: inferior pole, +3,000 μm: superior pole, optic nerve: 0 μm) and their average (F) in 12 month-old mice of the indicated mouse strains (Mann Whitney wildtype vs. Cx3cr1−/− mice *p = 0.0022; Mann Whitney Cx3cr1−/− vs. Cx3cr1−/−Cd36−/− mice $p = 0.0022). ONL, outer nuclear layer; PNA, peanut agglutinin Scale bar (A,D) = 50 μm. n = number of replicates indicated in the graphs; replicates represent quantification of eyes from different mice of at least three different cages.
CD36 Deficiency Prevents Pathogenic Subretinal Inflammation in Acute, Light-Induced Cx3cr1-Knockout Mice
Next, we evaluated its impact on acute light-induced stress. The intensity of the light-challenge model used herein was calibrated to induce substantial subretinal MP infiltration in AMD-prone Cx3cr1−/−-mice but not in C57BL6/J controls (6). Quantification of subretinal IBA-1+ MPs on retinal and RPE/choroidal flatmounts of 2–3 month old animals that were exposed to 4500 lux of green light with fully dilated pupils for 96 h, followed by 10 days of housing in the normal animal quarters, revealed an increase in subretinal MPs in Cx3cr1−/− mice, which was significantly inhibited in the absence of CD36 (Figures 3A,B). To quantify photoreceptor apoptosis in light-challenged mice we prepared TUNEL stained retinal flatmounts as previously described (5, 18). Flatmounts from Cx3cr1−/−mice displayed TUNEL positive cells in the outer nuclear layer, where the photoreceptor nuclei are located, to a much greater extent than C57BL6/J controls as previously reported (6). Importantly, Cx3cr1−/−Cd36−/− mice were protected against the photoreceptor apoptosis observed in Cx3cr1−/–mice (Figure 3C).
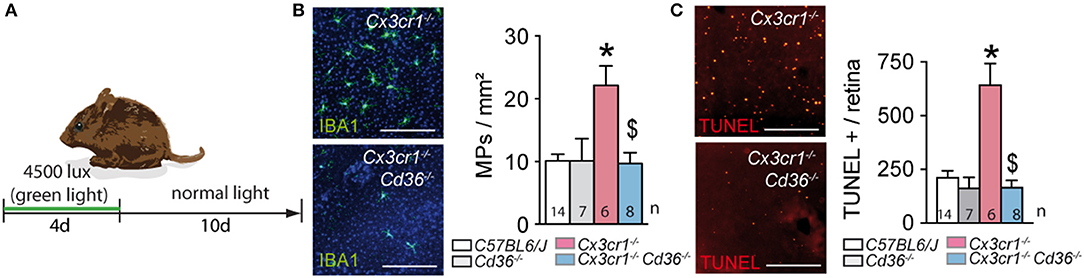
Figure 3. CD36 deficiency prevents pathogenic subretinal inflammation in acute, light-induced Cx3cr1-knockout mice. (A) Schematic representation of the light-challenge model. (B) Representative images and quantification of IBA-1 (green) stained RPE flatmounts of 2 month-old mice of the indicated strains after 10 days after the light-challenge (*Mann & Whitney test C57BL/6J control mice vs. Cx3cr1−/− mice p = 0.0014; $Mann & Whitney test Cx3cr1−/− vs. Cx3cr1−/−Cd36−/− mice p = 0.02). (C) Representative images and quantification of TUNEL+ cells in the outer nuclear layer of TUNEL-stained retinal flatmounts of d14 light-challenged mice of the indicated strains (*Mann & Whitney test C57BL/6J control mice vs. Cx3cr1−/− mice p = 0.0005; $Mann & Whitney test Cx3cr1−/− vs. Cx3cr1−/−Cd36−/− mice p = 0.0012). n, indicated for each bar; Scale bar (A) = 50 μm.
Taken together, CD36-deficiency very significantly inhibited the acute subretinal inflammation and associated photoreceptor apoptosis induced in the light-challenged Cx3cr1−/−mice, similar to our observations in chronic age-related inflammation.
CD36 Deficiency Normalizes the Resistance to Subretinal Elimination of Cx3cr1-Deficient Monocytes and Reduces Their IL-6 Production in vitro
We have previously shown that subretinal MPs in aged- and light-challenges Cx3cr1−/− mice are in part derived from infiltrating Mo (6). We showed that subretinally injected WT Mos, MCs or Mφs quickly undergo apoptosis and are eliminated (4), and that such clearance was significantly delayed when MPs lacked CX3CR1 (4). We demonstrated that this resistance was at least in part due to the fact that Cx3cr1-deficient MPs overexpress interleukin 6 (IL-6), which downregulates FasL expression the RPE that normally induces subretinal MP elimination (4).
To evaluate whether CD36 expression on MPs influences their susceptibility to be eliminated by the RPE, we adoptively transferred CFSE-labeled Mos from the different mouse strains into the subretinal space of wildtype mice. The surviving CFSE+ Mos were then enumerated 24 h after injection (Figure 4A). As previously shown, Cx3cr1-deficient Mo were significantly more numerous than subretinally injected wildtype Mos. Importantly, the deletion of Cd36 in Cx3cr1-deficient Mo completely reversed this effect (Figure 4B).
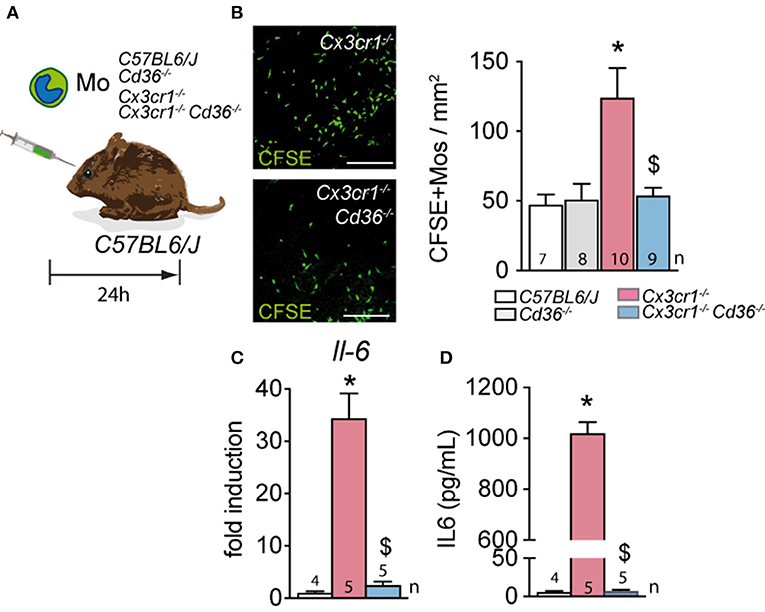
Figure 4. CD36 deficiency normalizes the resistance to subretinal elimination of Cx3cr1-deficient monocytes and reduces their IL-6 production in vitro. (A) Schematic representation of the subretinal adoptive transfer experiments. (B) Representative images and quantification of CSFE+ Mo of the indicated strains on RPE flatmounts 24 h after subretinal transfer to the subretinal space of wildtype mice (*Mann & Whitney test C57BL/6J Mo vs. Cx3cr1−/− Mo p = 0.0019; $Mann & Whitney test Cx3cr1−/− vs. Cx3cr1−/−Cd36−/− Mos p = 0.0079). (C) Quantitative RT–PCR of IL-6 mRNA normalized with S26 mRNA of C57BL/6J, Cx3cr1−/−, and Cx3cr1−/−Cd36−/− monocytes cultured for 24 h (*Mann & Whitney test C57BL/6J Mo vs. Cx3cr1−/− Mo p = 0.0159; $Mann & Whitney test Cx3cr1−/− vs. Cx3cr1−/−Cd36−/− Mos p = 0.0159). (D) IL-6 ELISA of the supernatants of C57BL/6J, Cx3cr1−/−-, and Cx3cr1−/−Cd36−/− monocytes cultured for 24 h (*Mann & Whitney test C57BL/6J Mo vs. Cx3cr1−/− Mo p = 0.0159; $Mann & Whitney test Cx3cr1−/− vs. Cx3cr1−/−Cd36−/− Mos p = 0.0079). n, indicated for each bar; Scale bar (A) = 50 μm.
Cx3cr1-deficient MPs express increased levels of APOE, which we showed activates the TLR2-CD14-dependent innate immunity receptor cluster and induces IL-6 (4). CD36 is a co-receptor of the toll-like receptor 2 (TLR2) and involved in the proinflammatory signaling cascade and the release of inflammatory cytokines (41, 42). Indeed, IL-6 transcription measured by RT-PCR (Figure 4C) and IL-6 secretion evaluated by ELISA (Figure 4D) was significantly increased in bone marrow derived Mo from Cx3cr1-deficient mice compared to control, but the deletion of Cd36 in Cx3cr1-deficient Mo prevented this increase of expression.
Taken together this data demonstrates that Cd36 deletion in Cx3cr1-deficient Mos inhibits the over-expression of IL-6 in Cx3cr1-deficient Mos and reverses their ability to withstand the immunosuppressive environment of the subretinal space.
Discussion
We previously showed that the deletion of Cx3cr1, a gene that is exclusively expressed on MPs in the adult eye, is sufficient to trigger an age-related or light-induced pathogenic non-resolving subretinal inflammation. We here demonstrate that the deletion of CD36 in Cx3cr1-deficient mice prevented the age- and light-challenge-dependent accumulation of subretinal MPs and protected against the associated photoreceptor cell death. Our data demonstrates that CD36 expression on Mo is necessary for the over-expression of IL-6 that we observe in Cx3cr1-deficient MdCs. We previously showed that IL-6 expression in Cx3cr1-deficient MPs is necessary for the accumulation of subretinal MPs in Cx3cr1-deficient mice, as it induces the downregulation of FasL expression the RPE that induces subretinal MP elimination in wildtype animals (4). Indeed, when we subretinally adoptively transferred Cx3cr1−/−- and Cd36−/−Cx3cr1−/−-Mo to wildtype recipients, the deletion of Cd36 on Cx3cr1−/−-monocytes restored the susceptible to subretinal elimination to the level observed in wildtype monocytes. Taken together our data demonstrates that Cd36 deficiency inhibits retinal inflammation and retinal degeneration in Cx3cr1 knockout mice.
We previously showed that Cx3cr1-deficient MPs are characterized by an over-expression of APOE that triggers NFκB activation and cytokine secretion. ApoE-deletion in Cx3cr1-deficient mice significantly prevents the accumulation of pathogenic subretinal MPs (4, 6, 27), similar to Cd36 deficiency. Mechanistically, we showed that the excess of APOE activates the CD14/TLR2-dependent innate immunity receptor complex (IIRC) on MPs (4, 27). This is likely due to APOE-induced cholesterol extraction from the lipid rafts of MPs, which lifts the physiological separation of CD14 (located in the lipid raft) from TLR2 (located in non-lipid raft membrane) and triggers NFκB activation and cytokine secretion in the absence of TLR ligands, as previously demonstrated for APOA-I (28, 46).
CD36 is also part of the innate immunity receptor complex (47, 48) and an obligate co-receptor of the toll-like receptor 2 (TLR2). Its inhibition blocks the TLR2-dependent NFκB activation and pro-inflammatory signaling cascade and the release of inflammatory cytokines such as IL-6 (41, 42). In Cx3cr1-deficient mice the deletion of Cd36 therefore likely counters the APOE-induced activation of the IIRC, activation of NFκB and IL-6 secretion, which we show reduces RPE FasL expression and MP elimination (4). As a result, the CD36-deficient MPs are quickly eliminated from the subretinal space, preventing pathogenic subretinal inflammation. Interestingly, it has recently been demonstrated that Cd36 deletion and pharmacological inhibition of CD36 also inhibits pathogenic inflammation in an acute model of light-induced degeneration in Cx3cr1-competent mice (42). The authors demonstrated that the protective effect is likely due to the inhibition of IL-1 that we showed also plays an important role in photoreceptor degeneration in Cx3cr1-deficient mice (3, 18).
In summary, the inhibition of CD36 on MPs in diseases that are characterized by pathogenic subretinal inflammation such as AMD, holds the promise to reduce MP accumulation and their production of pathogenic cytokines. On the other hand CD36 expressed by RPE cells has an important role in the maintenance of the choriocapillaries and the elimination of lipids from Bruchs membrane (39, 45). Ideally, future therapies for AMD might therefore want to specifically target CD36 on MPs.
Materials and Methods
Animals
Cx3cr1−/−CD36−/−mouse strains on C57BL/6 background were generated from Cx3cr1−/−mice and CD36−/−mouse strains, which were generated as previously described (49, 50). All mice were negative for the Crb1rd8, Pde6brd1, and Gnat2cpfl3 mutations. Mice were housed in the animal facility under specific pathogen-free condition, in a 12/12 h light/dark (100–500 lux) cycle with water and normal diet food available ad libitum. All experimental protocols and procedures were approved by the local animal care ethics committee “Comité d'Éthique en Expérimentation Animale Charles Darwin” (N° p3/2008/54).
Light-Challenge Model
Two- to four-month-old mice were adapted to darkness for 6 h and pupils were fully dilated with 1% Atropine (Novartis). Animals were then exposed to green LED light (4500 Lux, JP Vezon équipements) for 4 days and subsequently kept in cyclic 12/12 h normal animal facility conditions. MP accumulation and retinal degeneration were assessed at 10 days after light exposure (6).
Immunohistochemistry and TUNEL Staining of Retinal Flatmounts
Eyes were enucleated, fixed in 4% paraformaldehyde for 20 min at room temperature and sectioned at the limbus; the cornea and lens were discarded. The retinas were peeled from the RPE/choroid/sclera and incubated overnight at 4°C in PBS-1% triton with the following primary antibodies: peanut agglutinin Alexa fluor® 594 (Thermo Fisher Scientific; 1/100), and goat polyclonal anti-IBA1 (1/100, Wako). After few washes, the retinas were incubated for 2 h at room temperature with appropriate Alexa Fluor® conjugated secondary antibodies (Thermo Fisher Scientific; 1:500) in PBS-1% triton and nuclei were counterstained with Hoechst (1:1,000, Sigma Aldrich). The retinas were flatmounted and viewed with a fluorescence microscope (DM5500, Leica). Images centered on the area with the lowest number of PNA+ cone arrestin+ cells were captured with a confocal laser-scanning microscope (FV1000, Olympus) using a 40X lens. Each cell population was manually counted in a masked fashion. IBA-1+ cells were quantified on flatmounts on the outer segment side of the detached retina while PNA+ cone arrestin+ cells were counted on confocal microscopy Z-stacks using ImageJ software.
For histology, eyes were fixed in 0.5% glutaraldehyde, 4% PFA for 2 h, dehydrated and mounted in Historesin (Leica). Five millimeters of oriented sections crossing inferior pole, optic nerve and superior pole were cut and stained with toluidin blue. Rows of nuclei in the ONL were counted at different distances from the optic nerve (6).
For Terminal deoxynucleotidyl transferase dUTP nick end labeling (TUNEL), 4% PFA fixed retinal flatmounts were pre-treated with frozen methanol for 30 min and then frozen methanol/acetic acid (2:1) for another 30 min. After washing with PBS, flatmounts were incubated overnight at 4°C with the reaction mixture as described by manufacturer's protocol (In Situ Cell Death Detection Kit, Roche Diagnostics) and then for 90 min at 37°C. After reaction was stopped by washing with PBS at RT, nuclei were counterstained with Hoechst (Sigma–Aldrich).
Cell Preparations and Cell Culture
Bone marrow-derived monocytes (in serum-free X-Vivo 15 medium) were performed as previously described (6). RT-PCRs using Sybr Green (Life Technologies) and ELISAs using mouse IL-6 DuoSet (R&D Systems) were performed as previously described (6).
Subretinal Mononuclear Phagocyte Cell Clearance
Bone marrow-derived monocytes (~95% pure) were labeled in 10 μM CFSE (Life technologies). Cells were washed and resuspended in PBS. Twelve thousand cells (4 μl) were injected in the subretinal space of anesthetized 2 month-old mice using a microinjector and glass microcapillaries (Eppendorf). A hole was pierced with the glass capillary prior to the subretinal injection to avoid intra-ocular pressure increase and to allow retinal detachment with 4 μl of solution. The subretinal injection was verified by fundoscopy. Eyes were enucleated after 24 h, fixed in 4% PFA, and flatmounted. CFSE+transplanted cells were counted on the subretinal aspect of the retinal flatmount and the RPE/choroid flatmount of each eye. Eyes with subretinal hemorrhages were discarded.
Statistical Analysis
Sample sizes for our experiments were determined according to our previous studies Graph Pad Prism 6 (GraphPad Software) was used for data analysis and graphic representation. All values are reported as mean ± SEM. Statistical analysis was performed by Mann–Whitney U-test (2-group comparisons). The n and p-values are indicated in the figure legends.
Data Availability Statement
All datasets generated for this study are included in the article.
Ethics Statement
The animal study was reviewed and approved by Comité d'Ethique en Expérimentation Animale Charles Darwin.
Author Contributions
SL, J-BC, ST, CR, MH, SA, and WR conducted the experiments. CC, MF, HO, SC, J-AS, CD, XG, and FS designed and analyzed the experiments.
Funding
This work was supported by grants from INSERM, ANR MACLEAR (ANR-15-CE14-0015-01), UNADEV- Aviesan 2018–2019 Les maladies de la vision: origines et traitements, LABEX LIFESENSES (ANR-10-LABX-65) supported by the ANR [Programme d'Investissements d'Avenir (ANR-11-IDEX-0004-02)], Carnot, and a generous donation by Doris and Michael Bunte.
Conflict of Interest
The authors declare that the research was conducted in the absence of any commercial or financial relationships that could be construed as a potential conflict of interest.
References
1. Wong WL, Su X, Li X, Cheung CM, Klein R, Cheng CY, et al. Global prevalence of age-related macular degeneration and disease burden projection for 2020 and 2040: a systematic review and meta-analysis. Lancet Glob Health. (2014) 2:e106–16. doi: 10.1016/S2214-109X(13)70145-1
2. Sarks SH. Ageing and degeneration in the macular region: a clinico-pathological study. Br J Ophthalmol. (1976) 60:324–41. doi: 10.1136/bjo.60.5.324
3. Eandi CM, Charles Messance H, Augustin S, Dominguez E, Lavalette S, Forster V, et al. Subretinal mononuclear phagocytes induce cone segment loss via IL-1beta. eLife. (2016). 5:9. doi: 10.7554/eLife.16490.009
4. Levy O, Calippe B, Lavalette S, Hu SJ, Raoul W, Dominguez E, et al. Apolipoprotein E promotes subretinal mononuclear phagocyte survival and chronic inflammation in age-related macular degeneration. EMBO Mol Med. (2015) 7:211–26. doi: 10.15252/emmm.201404524
5. Combadiere C, Feumi C, Raoul W, Keller N, Rodero M, Pezard A, et al. CX3CR1-dependent subretinal microglia cell accumulation is associated with cardinal features of age-related macular degeneration. J Clin Invest. (2007) 117:2920–8. doi: 10.1172/JCI31692
6. Sennlaub F, Auvynet C, Calippe B, Lavalette S, Poupel L, Hu SJ, et al. CCR2(+) monocytes infiltrate atrophic lesions in age-related macular disease and mediate photoreceptor degeneration in experimental subretinal inflammation in Cx3cr1 deficient mice. EMBO Mol Med. (2013) 5:1775–93. doi: 10.1002/emmm.201302692
7. Lad EM, Cousins SW, Van Arnam JS, Proia AD. Abundance of infiltrating CD163+ cells in the retina of postmortem eyes with dry and neovascular age-related macular degeneration. Graefes Arch Clin Exp Ophthalmol. (2015) 253:1941–5. doi: 10.1007/s00417-015-3094-z
8. Gupta N, Brown KE, Milam AH. Activated microglia in human retinitis pigmentosa, late-onset retinal degeneration, and age-related macular degeneration. Exp Eye Res. (2003) 76:463–71. doi: 10.1016/S0014-4835(02)00332-9
9. Nathan C, Ding A. Nonresolving inflammation. Cell. (2010) 140:871–82. doi: 10.1016/j.cell.2010.02.029
10. Penfold PL, Madigan MC, Gillies MC, Provis JM. Immunological and aetiological aspects of macular degeneration. Prog Retin Eye Res. (2001) 20:385–414. doi: 10.1016/S1350-9462(00)00025-2
11. Griffith TS, Brunner T, Fletcher SM, Green DR, Ferguson TA. Fas ligand-induced apoptosis as a mechanism of immune privilege. Science. (1995) 270:1189–92. doi: 10.1126/science.270.5239.1189
12. Tsutsumi C, Sonoda KH, Egashira K, Qiao H, Hisatomi T, Nakao S, et al. The critical role of ocular-infiltrating macrophages in the development of choroidal neovascularization. J Leukoc Biol. (2003) 74:25–32. doi: 10.1189/jlb.0902436
13. Sakurai E, Anand A, Ambati BK, van Rooijen N, Ambati J. Macrophage depletion inhibits experimental choroidal neovascularization. Invest Ophthalmol Vis Sci. (2003) 44:3578–85. doi: 10.1167/iovs.03-0097
14. Rutar M, Natoli R, Provis JM. Small interfering RNA-mediated suppression of Ccl2 in Muller cells attenuates microglial recruitment and photoreceptor death following retinal degeneration. J Neuroinflammation. (2012) 9:221. doi: 10.1186/1742-2094-9-221
15. Suzuki M, Tsujikawa M, Itabe H, Du ZJ, Xie P, Matsumura N, et al. Chronic photo-oxidative stress and subsequent MCP-1 activation as causative factors for age-related macular degeneration. J Cell Sci. (2012) 125:2407–15. doi: 10.1242/jcs.097683
16. Kohno H, Chen Y, Kevany BM, Pearlman E, Miyagi M, Maeda T, et al. Photoreceptor proteins initiate microglial activation via toll-like receptor 4 in retinal degeneration mediated by all-trans-retinal. J Biol Chem. (2013) 288:15326–41. doi: 10.1074/jbc.M112.448712
17. Cruz-Guilloty F, Saeed AM, Echegaray JJ, Duffort S, Ballmick A, Tan Y, et al. Infiltration of proinflammatory m1 macrophages into the outer retina precedes damage in a mouse model of age-related macular degeneration. Int J Inflam. (2013) 2013:503725. doi: 10.1155/2013/503725
18. Hu SJ, Calippe B, Lavalette S, Roubeix C, Montassar F, Housset M, et al. Upregulation of P2RX7 in Cx3cr1-deficient mononuclear phagocytes leads to increased interleukin-1β secretion and photoreceptor neurodegeneration. J Neurosci. (2015) 35:6987–96. doi: 10.1523/JNEUROSCI.3955-14.2015
19. Lavalette S, Raoul W, Houssier M, Camelo S, Levy O, Calippe B, et al. Interleukin-1beta inhibition prevents choroidal neovascularization and does not exacerbate photoreceptor degeneration. Am J Pathol. (2011) 178:2416–23. doi: 10.1016/j.ajpath.2011.01.013
20. Zieger M, Ahnelt PK, Uhrin P. CX3CL1 (Fractalkine) protein expression in normal and degenerating mouse retina: in vivo studies. PLoS ONE. (2014) 9:e106562. doi: 10.1371/journal.pone.0106562
21. Silverman MD, Zamora DO, Pan Y, Texeira PV, Baek SH, Planck SR, et al. Constitutive and inflammatory mediator-regulated fractalkine expression in human ocular tissues and cultured cells. Invest Ophthalmol Vis Sci. (2003) 44:1608–15. doi: 10.1167/iovs.02-0233
22. Ransohoff RM. Chemokines and chemokine receptors: standing at the crossroads of immunobiology and neurobiology. Immunity. (2009) 31:711–21. doi: 10.1016/j.immuni.2009.09.010
23. Raoul W, Keller N, Rodero M, Behar-Cohen F, Sennlaub F, Combadiere C. Role of the chemokine receptor CX3CR1 in the mobilization of phagocytic retinal microglial cells. J Neuroimmunol. (2008) 198:56–61. doi: 10.1016/j.jneuroim.2008.04.014
24. Ma W, Zhao L, Fontainhas AM, Fariss RN, Wong WT. Microglia in the mouse retina alter the structure and function of retinal pigmented epithelial cells: a potential cellular interaction relevant to AMD. PLoS ONE. (2009) 4:e7945. doi: 10.1371/journal.pone.0007945
25. Kezic JM, Chen X, Rakoczy EP, McMenamin PG. The effects of age and Cx3cr1 deficiency on retinal microglia in the Ins2Akita diabetic mouse. Invest Ophthalmol Vis Sci. (2013) 54:854–63. doi: 10.1167/iovs.12-10876
26. Chen M, Luo C, Penalva R, Xu H. Paraquat-induced retinal degeneration is exaggerated in CX3CR1-deficient mice and is associated with increased retinal inflammation. Invest Ophthalmol Vis Sci. (2013) 54:682–90. doi: 10.1167/iovs.12-10888
27. Levy O, Lavalette S, Hu SJ, Housset M, Raoul W, Eandi C, et al. APOE-isoforms control pathogenic subretinal inflammation in age related macular degeneration. J Neurosci. (2015) 35:13568–76. doi: 10.1523/JNEUROSCI.2468-15.2015
28. Guillonneau X, Eandi CM, Paques M, Sahel JA, Sapieha P, Sennlaub F. On phagocytes and macular degeneration. Prog Retin Eye Res. (2017) 61:98–128. doi: 10.1016/j.preteyeres.2017.06.002
29. Calippe B, Augustin S, Beguier F, Charles-Messance H, Poupel L, Conart JB, et al. Complement factor H inhibits CD47-mediated resolution of inflammation. Immunity. (2017) 46:261–72. doi: 10.1016/j.immuni.2017.01.006
30. Jobling AI, Waugh M, Vessey KA, Phipps JA, Trogrlic L, Greferath U, et al. The role of the microglial Cx3cr1 pathway in the postnatal maturation of retinal photoreceptors. J Neurosci. (2018) 38:4708–23. doi: 10.1523/JNEUROSCI.2368-17.2018
31. Sadda SR, Guymer R, Holz FG, Schmitz-Valckenberg S, Curcio CA, Bird AC, et al. Consensus definition for atrophy associated with age-related macular degeneration on OCT: classification of atrophy report 3. Ophthalmology. (2018) 125:537–48. doi: 10.1016/j.ophtha.2017.09.028
32. Febbraio M, Hajjar DP, Silverstein RL. CD36: a class B scavenger receptor involved in angiogenesis, atherosclerosis, inflammation, and lipid metabolism. J Clin Invest. (2001) 108:785–91. doi: 10.1172/JCI14006
33. Jimenez B, Volpert OV, Crawford SE, Febbraio M, Silverstein RL, Bouck N. Signals leading to apoptosis-dependent inhibition of neovascularization by thrombospondin-1. Nat Med. (2000) 6:41–8. doi: 10.1038/71517
34. Dawson DW, Pearce SF, Zhong R, Silverstein RL, Frazier WA, Bouck NP. CD36 mediates the in vitro inhibitory effects of thrombospondin-1 on endothelial cells. J Cell Biol. (1997) 138:707–17. doi: 10.1083/jcb.138.3.707
35. Finnemann SC, Silverstein RL. Differential roles of CD36 and alphavbeta5 integrin in photoreceptor phagocytosis by the retinal pigment epithelium. J Exp Med. (2001) 194:1289–98. doi: 10.1084/jem.194.9.1289
36. Ryeom SW, Sparrow JR, Silverstein RL. CD36 participates in the phagocytosis of rod outer segments by retinal pigment epithelium. J Cell Sci. (1996) 109:387–95.
37. Ryeom SW, Silverstein RL, Scotto A, Sparrow JR. Binding of anionic phospholipids to retinal pigment epithelium may be mediated by the scavenger receptor CD36. J Biol Chem. (1996) 271:20536–9. doi: 10.1074/jbc.271.34.20536
38. Sun M, Finnemann SC, Febbraio M, Shan L, Annangudi SP, Podrez EA, et al. Light-induced oxidation of photoreceptor outer segment phospholipids generates ligands for CD36-mediated phagocytosis by retinal pigment epithelium: a potential mechanism for modulating outer segment phagocytosis under oxidant stress conditions. J Biol Chem. (2006) 281:4222–30. doi: 10.1074/jbc.M509769200
39. Picard E, Houssier M, Bujold K, Sapieha P, Lubell W, Dorfman A, et al. CD36 plays an important role in the clearance of oxLDL and associated age-dependent sub-retinal deposits. Aging. (2010) 2:981–9. doi: 10.18632/aging.100218
40. Gnanaguru G, Choi AR, Amarnani D, D'Amore PA. Oxidized lipoprotein uptake through the CD36 receptor activates the NLRP3 inflammasome in human retinal pigment epithelial cells. Invest Ophthalmol Vis Sci. (2016) 57:4704–12. doi: 10.1167/iovs.15-18663
41. Hoebe K, Georgel P, Rutschmann S, Du X, Mudd S, Crozat K, et al. CD36 is a sensor of diacylglycerides. Nature. (2005) 433:523–7. doi: 10.1038/nature03253
42. Mellal K, Omri S, Mulumba M, Tahiri H, Fortin C, Dorion MF, et al. Immunometabolic modulation of retinal inflammation by CD36 ligand. Sci. Rep. (2019) 9:12903. doi: 10.1038/s41598-019-49472-8
43. Fadok VA, Bratton DL, Konowal A, Freed PW, Westcott JY, Henson PM. Macrophages that have ingested apoptotic cells in vitro inhibit proinflammatory cytokine production through autocrine/paracrine mechanisms involving TGF-beta, PGE2, and PAF. J Clin Invest. (1998) 101:890–8. doi: 10.1172/JCI1112
44. Voll RE, Herrmann M, Roth EA, Stach C, Kalden JR, Girkontaite I. Immunosuppressive effects of apoptotic cells. Nature. (1997) 390:350–1. doi: 10.1038/37022
45. Houssier M, Raoul W, Lavalette S, Keller N, Guillonneau X, Baragatti B, et al. CD36 deficiency leads to choroidal involution via COX2 down-regulation in rodents. PLoS Med. (2008) 5:e39. doi: 10.1371/journal.pmed.0050039
46. Smoak KA, Aloor JJ, Madenspacher J, Merrick BA, Collins JB, Zhu X, et al. Myeloid differentiation primary response protein 88 couples reverse cholesterol transport to inflammation. Cell Metab. (2010) 11:493–502. doi: 10.1016/j.cmet.2010.04.006
47. Pfeiffer A, Bottcher A, Orso E, Kapinsky M, Nagy P, Bodnar A, et al. Lipopolysaccharide and ceramide docking to CD14 provokes ligand-specific receptor clustering in rafts. Eur J Immunol. (2001) 31:3153–64. doi: 10.1002/1521-4141(200111)31:11<3153::aid-immu3153>3.0.co;2-0
48. Schmitz G, Orso E. CD14 signalling in lipid rafts: new ligands and co-receptors. Curr Opin Lipidol. (2002) 13:513–21. doi: 10.1097/00041433-200210000-00007
49. Combadiere C, Potteaux S, Gao JL, Esposito B, Casanova S, Lee EJ, et al. Decreased atherosclerotic lesion formation in CX3CR1/apolipoprotein E double knockout mice. Circulation. (2003) 107:1009–16. doi: 10.1161/01.CIR.0000057548.68243.42
Keywords: age related macular degeneration, mononuclear phagocyte, CX3CR1, CD36, IL-6, photoreceptor
Citation: Lavalette S, Conart J-B, Touhami S, Roubeix C, Houssier M, Augustin S, Raoul W, Combadière C, Febbraio M, Ong H, Chemtob S, Sahel J-A, Delarasse C, Guillonneau X and Sennlaub F (2020) CD36 Deficiency Inhibits Retinal Inflammation and Retinal Degeneration in Cx3cr1 Knockout Mice. Front. Immunol. 10:3032. doi: 10.3389/fimmu.2019.03032
Received: 24 October 2019; Accepted: 10 December 2019;
Published: 08 January 2020.
Edited by:
Heping Xu, Queen's University Belfast, United KingdomReviewed by:
Erica Lucy Fletcher, The University of Melbourne, AustraliaBernahrd Ryffel, Centre National de la Recherche Scientifique (CNRS), France
Copyright © 2020 Lavalette, Conart, Touhami, Roubeix, Houssier, Augustin, Raoul, Combadière, Febbraio, Ong, Chemtob, Sahel, Delarasse, Guillonneau and Sennlaub. This is an open-access article distributed under the terms of the Creative Commons Attribution License (CC BY). The use, distribution or reproduction in other forums is permitted, provided the original author(s) and the copyright owner(s) are credited and that the original publication in this journal is cited, in accordance with accepted academic practice. No use, distribution or reproduction is permitted which does not comply with these terms.
*Correspondence: Xavier Guillonneau, eGF2aWVyLmd1aWxsb25uZWF1QGluc2VybS5mcg==; Florian Sennlaub, Zmxvcmlhbi5zZW5ubGF1YkBpbnNlcm0uZnI=