- Department of Biomedical Sciences, Center for Immunobiology, Western Michigan University Homer Stryker M.D. School of Medicine, Kalamazoo, MI, United States
Plasma cells (PCs) represent the terminal differentiation step of mature B lymphocytes. These cells are most recognizable for their extended lifespan as well as their ability to secrete large amounts of antibodies (Abs) thus positioning this cell type as a key component of humoral immunity. However, it is now appreciated that PCs can have far reaching effects on pathologic as well as non-pathologic processes independent of Ab secretion. This is highlighted by recent studies showing that PCs function as key regulators of processes such as hematopoiesis as well as neuro-inflammation. In part, PCs accomplish this by integrating extrinsic signals from their environment which dictate their downstream functionality. Here we summarize the current understanding of PC biology focusing on their ever-growing functional repertoire independent of Ab production. Furthermore, we discuss potential applications of PC immunotherapy and its implementation for translational benefit.
Introduction
Trademarks of the adaptive immune system include the ability to respond to diverse sets of antigenic stimuli and the long-term durability of this response. The former is initiated early in B and T lymphocyte development and is driven by V(D)J recombination of antigenic gene loci (1). In the case of B lymphocytes, this antigenic diversity can be further modified via somatic hypermutation (SHM) (2) and class switching (3) during a subsequent immune response. In contrast, the durability of an immune response results from the formation of long-term immunological “memory” which includes cell types such as memory B and T lymphocytes (4) as well as plasma cells (PCs) (5).
A significant goal upon vaccination or infection is the production of protective Abs for a sustained period of time. Given this, it can be rationally perceived that PCs sit at the apex of adaptive immunity in the sense that these cells have the potential to survive indefinitely in both mice (6–9) and humans (10–12) while also continuously secreting Abs (9). This latter phenotype being thanks in part to an expanded Golgi-network that provides PCs with their signature peri-nuclear halo when viewed under a microscope (13). As such, the accumulation of these cells over a lifetime potentially represents a historical record of humoral immune responses. Not surprisingly, numerous studies have focused on the induction of PCs differentiation from the perspective of generating antigen-specific Ab responses (14).
However, the field has recently begun to appreciate the multitude of functions that PCs possess aside from Ab secretion (15–18). This Perspective highlights the evolving functions of PCs and discusses the potential for environmental interactions to program these diverse regulatory behaviors. Furthermore, we consider the long-term effects of these cells on various biological processes including aging and introduce strategies that may become key means of modulating PC functionality for a beneficial outcome.
More than Just Antibody Factories
Regulation of Infectious and Autoimmune Immune Responses
For decades, it has been presumed that upon maturation, PCs migrate to the bone marrow (BM) where they remain quiescent and ONLY secrete copious amounts of Abs. However, this viewpoint has now been challenged (Figure 1). In a landmark study, it was shown that stimulation of mice with lipopolysaccharide (LPS) led to the generation of a population of granulocyte-macrophage colony-stimulating factor (GM-CSF) producing cells referred to as innate response activator (IRA) B cells (19). Phenotyping of IRA B cells demonstrated the expression of the PC marker, CD138. Transcriptional analysis of these cells further supported the idea that IRA B cells may be a bona fide subset of PCs as their gene signature clustered closest to PCs compared to other B cell subsets. Similarly, recent studies in mice examining responses to infectious agents such as Trypanosoma cruzi and Salmonella enterica have demonstrated the propensity of PCs to express cytokines such as interleukin (IL)-17 (20) as well as IL-35 and IL-10 (21), respectively. In this regard, B lymphocyte derived IL-17 was absolutely required for efficient control of T. cruzi infection and dampening of infection-associated inflammation following pathogen clearance (20). In contrast, B lymphocyte-derived IL-35 was detrimental in the context of Salmonella infection as its deletion led to enhanced monocyte and T lymphocyte responses upon infection (21). While these studies focused on B lymphocyte-specific cytokine ablation and not just PC-specific cytokine deletion, they clearly demonstrated that factors produced by PCs as well as other B lymphocyte populations play a critical role in regulating host-pathogen interactions.
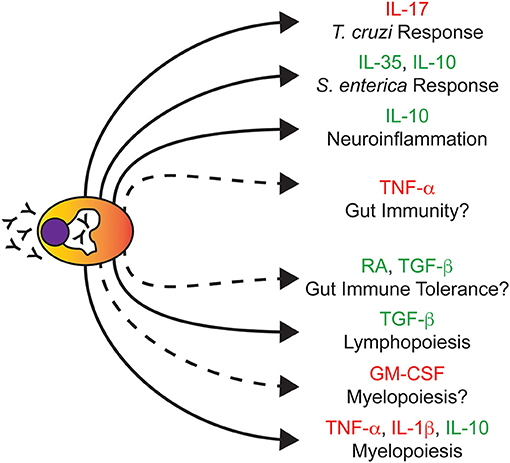
Figure 1. Plasma cells regulate biological processes independent of immunoglobulins. Due to their enhanced endoplasmic reticulum-Golgi structure (white peri-nuclear halo), PCs are best known for their ability to secrete Ig (depicted as Y). Illustrated here are various non-Ig secreted factors that PCs produce and the biological processes that PCs are known to regulate. Solid connections represent studies in which the removal of PCs and/or their secreted factors had a documented biological outcome. Dashed connections represent predicted regulatory nodes based on the cytokines produced which require further experimental validation. Cytokines highlighted in red or green are commonly associated with being pro-inflammatory or anti-inflammatory, respectively. Note that this figure does not summarize studies of Ig-based PC effector function.
The role of PCs in the progression of autoimmune disease is well-known with the focus mainly on Ab production as auto-Abs, through their constant regions, can potentially induce a pro-inflammatory cascade (22). Studies in mouse models of lupus (23–26) as well as human systemic lupus erythematosus (SLE) patients (27) have shown that PC depletion reduces the level of autoreactive antibodies as well as disease burden. However, the role of PCs in autoimmunity is not solely limited to SLE (28).
A recent study elegantly demonstrated the role of IL-10 producing PCs in the suppression of neuroinflammation in a mouse model of autoimmune encephalomyelitis (EAE) which recapitulates some features of multiple sclerosis (MS) in humans (18). Using genetic models combined with BM chimeras, the authors demonstrated that in this instance, PC-produced IL-10 was the key molecule in the suppression of EAE-induced pathology. These IL-10-producing PCs were originally derived from the small intestine and migrated to the central nervous system (CNS), an important observation given the association of the microbiota with diseases such as multiple sclerosis (29). Using Mb-1-Cre-mediated deletion of Prdm1 to ablate PC differentiation, a previous report also observed a critical role for PCs in the suppression of EAE in mice (30). In this instance, in vitro co-culture experiments implicated a role for PB-derived IL-10 in the suppression of dendritic cell function and subsequent interferon-gamma (IFN-γ) production by CD4+ T cells. These results point to PCs having a beneficial effect in the context of EAE. However, this is not the case for all autoimmune disorders of the nervous system. For instance, neuromyelitis optica (NMO) targets the optic nerve and spinal cord resulting in their degeneration and this is largely thought to be due to the production of auto-Abs targeting aquaporin 4 (AQP4), which is highly expressed in the central nervous system (CNS) (31, 32). Direct evidence of this was demonstrated in a in a rat model in which AQP4-specific auto-Abs cloned from human patients induced overt NMO pathology highlighted by astrocyte depletion and myelinolysis following their administration (33). Another study demonstrated the reliance of PBs isolated from human NMO patients on the cytokine IL-6 for not only enhanced survival but AQP4 auto-Ab secretion as well (32). Whether or not PBs in NMO can be an autocrine supply of this cytokine becomes an interesting question in light of in vitro experiments that demonstrated the capacity of human PBs to produce IL-6 (34). In the studies of EAE noted above, it is unclear whether or not the responding PCs were clonally derived; that is, antigen specific as is evident in the NMO studies described here as well as in human cases of multiple sclerosis (35).
Regulation of Hematopoiesis by Plasma Cells
In BM, PCs localize in close proximity to stromal cells with ~80% of BM PCs directly contacting stroma (36). While being a key component of the PC survival niche (37), stromal cells are also essential in the proper maintenance of hematopoiesis (38). Therefore, it stands to reason that PCs have the potential to regulate hematopoiesis directly through actions on progenitors or indirectly by regulating the stromal niche. Indeed, this is the case as we identified PCs as key effectors driving enhanced myelopoiesis observed in aging mouse BM (17). Ab-mediated depletion of PCs in aged mice led to the reduction of myelopoiesis to levels observed in young animals in part through alterations in the BM niche highlighted by reduced expression of myeloid promoting factors such as Il1b and Csf1 by BM stromal cells. Co-culture experiments demonstrated that the ability of PCs to drive increased myelopoiesis was age-dependent as young PCs did not promote myelopoiesis in contrast to old PCs. Transcriptional profiling via RNA-sequencing (RNA-seq) demonstrated that old PCs adopted an inflammatory gene signature which could be exacerbated by Toll-like receptor (TLR) stimulation as evidenced by increased Il1b and Tnf gene expression following in vitro lipopolysaccharide (LPS) stimulation. Pharmacological blockade of IL-1 and tumor necrosis factor-alpha (TNF-α) signaling reduced granulopoiesis in aged mice demonstrating the importance of these factors in the age-associated increase in myelopoietic output (17). Notably, a recently published study (16) demonstrated that in vitro derived mouse PCs had the potential to regulate the composition of myeloid cells generated in culture. Here, IL-10 was the critical regulator that skewed the myeloid compartment toward a more macrophage-like cell fate (16).
In regards to lymphopoiesis, both mouse (17) and human (39) PCs have been shown to suppress B lymphopoiesis. In the latter study, the ability to inhibit B lymphopoiesis required interactions between multiple myeloma (MM) cells, a PC-derived neoplasm, and stromal cells which led to increased expression of CCL3 (macrophage inflammatory protein-one alpha, MIP-1α) and CCL4 (macrophage inflammatory protein-one beta, MIP-1β) by stromal cells as well as increased expression of TGFB1 (transforming growth factor-beta one, TGF-β1) by both PCs and stromal cells (39). Addition of either TGF-β1 or MIP-1β to pre-B lymphocyte cultures was sufficient in suppressing B lymphopoiesis in vitro demonstrating the regulatory potential of these factors (39). Collectively, these studies demonstrate the ability of PCs to regulate a fundamental process in hematopoiesis. Furthermore, the data suggest that PCs do so independently of their ability to produce Abs and in at least some instances, a cytokine-dependent manner.
Gut Homeostasis and Plasma Cells
A majority of PCs reside in the gut as this tissue provides a direct interface between host cells and microbes (40). These cells play critical roles in gut homeostasis through the production of secretory IgA (SIgA) (41) which regulates not only exclusion of IgA-cross reactive bacteria but also the propagation of the immune response (42). However, a recent study in mice (15) has shown that PCs promote the generation of regulatory T (TReg) cells in the gut through the production of TGF-β and retinoic acid (RA) suggesting the importance of PCs in maintaining intestinal immune tolerance. Paradoxically, PCs from the murine lamina propria (43) have also been shown to produce TNF-α as well as inducible nitric oxide synthase (iNOS), a key regulator of IgA class switching (44). It remains to be determined if the same PCs have the potential to make TGF-β, RA, and TNF-α or alternatively, if these represent unique cytokine-producing PC subsets. How the relative balance of anti-inflammatory (TGF-β, RA) and pro-inflammatory (TNF-α) cytokine production in the gut alters its homeostasis is a critical question given the potential roles of these cytokines in pathologies such as intestinal bowel disease (45).
Programming Plasma Cell Function
Plasma Cells Express Functional Membrane-Associated Immunoglobulins
Previous assumption has been that PCs do not express membrane immunoglobulin (mIg). We now know differently as IgM+ and IgA+ PCs in both mouse (17, 46) and human (47) have been shown to express mIg. This is true for IgE+ PCs in mice as well (48). Multiple studies have demonstrated that these surface BCRs are fully functional. In humans, Ig crosslinking of mIgM+ and mIgA+ PCs led to increased amounts of phosphorylated Syk as well as phosphorylated ERK and AKT which represent events proximal and distal to BCR signaling, respectively (47). Given the importance of these kinases in B lymphocyte viability, it is not surprising that low dose mIg crosslinking provided a survival benefit to mIgA+ PCs in vitro (47). In vivo, a recent murine study showed that stimulation of BM mIgM+ PCs through their mIg led to increased surface expression of CD69 as well as changes in gene expression highlighted by increases in the aforementioned Il10 as well as Ccl5 (46), a pro-myelopoietic cytokine (49). Taken together, these data suggest that mIg activation of a previously established pool of PCs may elicit a pro-myelopoietic transcriptional response; however, experiments are needed to validate this hypothesis.
Plasma Cells Are Responsive to Toll-Like Receptor Signals
TLR signaling in B lymphocytes contributes to the formation of the PC compartment (50, 51). Not unlike B lymphocytes, terminally differentiated PCs also express TLRs. In humans, PCs isolated from the peripheral blood as well as the tonsils have been shown to express TLRs 1–10 (52). Additionally, the stimulation of human PCs with TLR ligands such as peptidoglycan, poly(I:C) and flagellin significantly increased Ab secretion in vitro (52). Similarly, TLR expression has been detected in human MM both in cell lines and primary cells (53, 54). In regard to MM, TLR signals have been observed to provide context dependent signals. For example, TLR4 ligation enhanced survival and proliferation (54, 55) whereas TLR1/2 derived signals sensitized MM cells to chemotherapy-induced cell death (53).
However, the classical roles of TLR signaling respective to inflammatory responses remain understudied in both human and mouse PCs. Mentioned previously, PCs isolated from the BM of old mice displayed increased levels of Tlr4 gene expression and were highly responsive to LPS signaling in contrast to their young counterparts (17). Additionally, old murine PCs displayed increased expression of Tlr6, Tlr7, Naip2, Naip6, and Nod2. It will be interesting to determine if these signaling pathways are also functionally responsive in PCs and to what biological outcome.
Selective Elimination of Plasma Cells for Translational Outcomes
It is becoming readily apparent that PCs play significant roles in biology outside of Ab secretion. However, the full spectrum of PC functionality has yet to be defined. In this sense, it will be important to determine the causes, or context dependent signals, that drive plasma cell heterogeneity such as the surrounding cytokine milieu and even the nature of the antigen itself. Indeed, phenotypically long-lived PCs have been identified in various organs in both rodents (56–59) and humans (11, 12, 60) however, it is not fully understood how these differing niches may regulate PC behavior. Furthermore, whether these cues act directly on PCs themselves as has been demonstrated for the model antigen 4-hydroxy-3-nitrophenylacetic-dextran (NP-dextran) (46) or if PC phenotypes are imprinted from the activated B cell that lies upstream will be important to consider. With that being said, our recent study regarding PCs and their effects on age-associated patterns of hematopoiesis in mice (17) provides an example of how PCs can be targeted for a potential therapeutic benefit. Aging is associated with increases in myeloid leukemias which has been correlated with the heightened levels of BM myelopoiesis also observed with age (61, 62). In both humans (63) and mice (64, 65), this is initiated at the most primitive progenitor levels as the hematopoietic stem cell (HSC) compartment becomes more myeloid-biased with age. At least in mice, this is in part due to the preferential expansion of myeloid-biased HSCs (My-HSCs) rather than the loss of HSCs with inherent lymphoid-bias (Ly-HSCs) (66).
Using Abs directed toward CD138, a cell surface determinant common to PCs, we successfully depleted their numbers and reversed the expanded myelopoiesis commonly associated with aging (17). While this strategy accomplished its goal and may potentially be a route toward reducing the risk of age-associated myeloid leukemias, it is not feasible in “real life.” The reason being is that PCs are the major Ab producers in the body and theoretically, provide protective immunity derived from even the earliest of ages. As such, depleting the bulk, if not all, of the PC pool would leave the host severely immunocompromised. This may not be as critical in younger individuals as they generally possess robust immune responses and can be re-vaccinated to restore the PC pool once a particular translational goal is achieved. However, the elderly are more susceptible to infection and possess weakened responses to vaccination (67). In part, this is due to age-associated changes in B lymphocytes which include an altered immune repertoire as well as decreased expression of E2A and activation-induced cytidine deaminase (AID) which would be predicted to compromise PC differentiation (68).
For PC depletion/modulation to be a successful strategy, the PC pool must be heterogenous in nature and possess cell surface determinants specific for a given PC subpopulation (Figure 2A). To some degree, we already know this to be true. For example, IL-17 producing PCs generated following T. cruzi infection only constitute roughly ~6–8% of the total splenic PC pool at 10 days post-infection (20). Using Il10eGFP reporter mice (69), it was shown that LAG-3 was sufficient to prospectively identify Il10eGFP+ PCs which constituted approximately 20 and 40% of total PCs in BM from young and old mice, respectively. Thus, functional PC subsets exist and, in some instances, can be identified via expression of unique cell surface proteins.
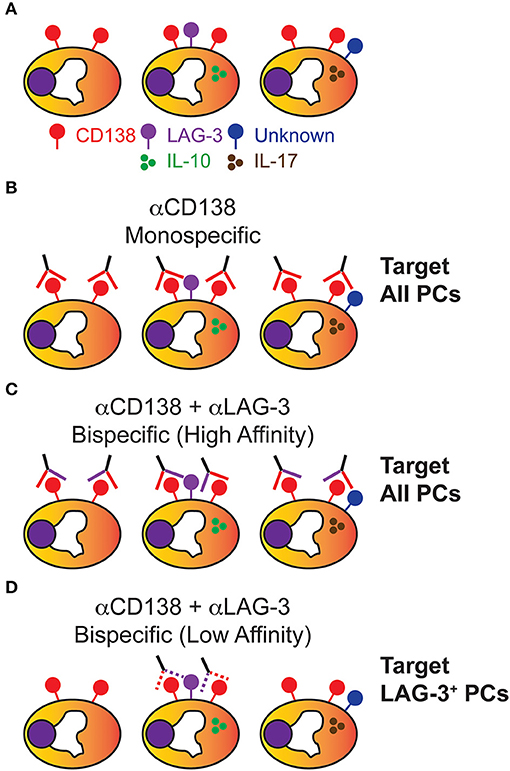
Figure 2. Plasma cells are heterogenous and can be potentially targeted in a subset specific manner. (A) While all PCs express the cell surface determinant CD138, PCs are heterogenous in the cytokines produced and various cell surface markers they express, of which only some are known. (B) Using αCD138 antibodies, PCs can be homogenously targeted for depletion which randomly and significantly depletes the PC-derived Ig pool. (C) Using a high affinity bispecific antibody designed to react against CD138 and LAG-3, all PCs are presumably depleted due to strong CD138 reactivity similar to (B). (D) Using a low affinity bispecific antibody designed to react against CD138 and LAG-3, a therapeutic window has now been theoretically created which allows for the specific depletion of IL-10-producing CD138+ LAG-3+ PCs while still leaving the rest of the PC Ig repertoire intact.
So how do we ablate a particular subset such as LAG-3+ PCs? Cell type-specific targeting would be key as depleting or altering the function of all LAG-3+ cells would potentially lead to the loss of tolerance through adverse effects on the TReg pool (70). Looking toward the field of tumor immunotherapy (71), we find that a potential solution would be the generation of bispecific Abs reactive to both CD138 and LAG-3 which would preferentially target IL-10-producing PCs (Figures 2B–D). Having a high affinity bispecific Ab would be favorable in a tumor setting where elimination of every cell is the desired outcome. However, in terms of targeting a particular subset of PCs, this would still result in targeting all PCs through efficient binding of CD138 (Figure 2C). One would need to develop a bispecific Ab where each epitope is bound sub-optimally and thus only PCs which expressed both CD138 and LAG-3 would be bound with high enough affinity required for depletion (Figure 2D).
Secondarily, the mode of elimination would need to be chosen in an application specific manner. For example, these Abs could be conjugated to a particular toxin such as type I interferon or even bortezomib, a MM chemotherapeutic. However, it is not known if this strategy would cause high levels of local or even systemic inflammation which could have unintended consequences. For example, lymphopoiesis is suppressed by high levels of inflammatory cytokines such as IL-1 while in contrast, inflammation enhances myelopoiesis (72). As such, using an unconjugated Ab may be most prudent. Mechanistically it is not known how unconjugated CD138 antibodies deplete PCs (17). This could be through Ig constant region-mediated interactions such as with complement or even through the deprivation of survival signals as CD138 has been demonstrated to promote PC cell survival through heparan sulfate-mediated interactions with IL-6 and APRIL (73). In this regard, it would be important to assess the depletion efficiency of a CD138 Ab in which the constant region [mouse IgG2a in (17)] has been converted to a different isotype or deleted altogether.
Concluding Remarks
PCs are key contributors to effective humoral immunity through their robust production of antigen specific Abs. However, it is now readily apparent that these cells do more than just secrete Ig and in fact, play critical roles in normal processes such as hematopoiesis as well as diseases such as EAE. But much remains unknown about these cells in regards to the full spectrum of their regulatory potential. In this respect, the present article has focused on PCs in the adult setting. PCs can be found in the blood of humans as early as 1–5 months of age (74) and are important sources of protective IgA in the gut of newborns (75). Whether or not these cells possess critical Ab-independent functions remains to be determined. Fortunately, both genetic and molecular tools are now available to acutely deplete all PCs and observe the resultant effects on a variety of biological systems. While multiple studies have transcriptionally profiled the PC pool as a whole (17, 76, 77), a further understanding is required in regards to the regulation and extent of functional heterogeneity of PCs both in the naïve state as well as following an infectious or autoimmune response before we will have the capacity to target PCs subsets for a translational outcome.
Using single cell sequencing approaches, it will now be possible to fully visualize the PC landscape and better understand the molecular underpinnings of various subsets of PCs. Analyses of these data may provide important answers to remaining questions such as: What types of B lymphocytes give rise to a particular PC subset? What extracellular signals drive the derivation of a specific type of PC? What core transcriptional elements regulate various PC fractions? Ultimately, this has the potential to lead to the development of PC-targeted immunotherapies for the purposes of modulating specific biological outcomes.
Author Contributions
PP conceptualized and wrote the manuscript.
Funding
PP was currently supported by startup funds from the Western Michigan University Homer Stryker M.D. School of Medicine.
Conflict of Interest
The author declares that the research was conducted in the absence of any commercial or financial relationships that could be construed as a potential conflict of interest.
Acknowledgments
PP thanks KimAnh Pioli for careful review of the manuscript and insightful commentary.
References
1. Thomas LR, Cobb RM, Oltz EM. Dynamic regulation of antigen receptor gene assembly. Adv Exp Med Biol. (2009) 650:103–15. doi: 10.1007/978-1-4419-0296-2_9
2. Zuo T, Gautam A, Wesemann DR. Affinity war: forging immunoglobulin repertoires. Curr Opin Immunol. (2019) 57:32–9. doi: 10.1016/j.coi.2018.12.002
3. Tudor D, Yu H, Maupetit J, Drillet AS, Bouceba T, Schwartz-Cornil I, et al. Isotype modulates epitope specificity, affinity, and antiviral activities of anti-HIV-1 human broadly neutralizing 2F5 antibody. Proc Natl Acad Sci USA. (2012) 109:12680–5. doi: 10.1073/pnas.1200024109
5. Khodadadi L, Cheng Q, Radbruch A, Hiepe F. The maintenance of memory plasma cells. Front Immunol. (2019) 10:721. doi: 10.3389/fimmu.2019.00721
6. Chernova I, Jones DD, Wilmore JR, Bortnick A, Yucel M, Hershberg U, et al. Lasting antibody responses are mediated by a combination of newly formed and established bone marrow plasma cells drawn from clonally distinct precursors. J Immunol. (2014) 193:4971–9. doi: 10.4049/jimmunol.1401264
7. Manz RA, Lohning M, Cassese G, Thiel A, Radbruch A. Survival of long-lived plasma cells is independent of antigen. Int Immunol. (1998) 10:1703–11. doi: 10.1093/intimm/10.11.1703
8. Manz RA, Thiel A, Radbruch A. Lifetime of plasma cells in the bone marrow. Nature. (1997) 388:133–4. doi: 10.1038/40540
9. Slifka MK, Antia R, Whitmire JK, Ahmed R. Humoral immunity due to long-lived plasma cells. Immunity. (1998) 8:363–72. doi: 10.1016/S1074-7613(00)80541-5
10. Amanna IJ, Carlson NE, Slifka MK. Duration of humoral immunity to common viral and vaccine antigens. N Engl J Med. (2007) 357:1903–15. doi: 10.1056/NEJMoa066092
11. Halliley JL, Tipton CM, Liesveld J, Rosenberg AF, Darce J, Gregoretti IV, et al. Long-lived plasma cells are contained within the CD19(-)CD38(hi)CD138(+) subset in human bone marrow. Immunity. (2015) 43:132–45. doi: 10.1016/j.immuni.2015.06.016
12. Landsverk OJ, Snir O, Casado RB, Richter L, Mold JE, Reu P, et al. Antibody-secreting plasma cells persist for decades in human intestine. J Exp Med. (2017) 214:309–17. doi: 10.1084/jem.20161590
13. Ribatti D. The discovery of plasma cells: an historical note. Immunol Lett. (2017) 188:64–7. doi: 10.1016/j.imlet.2017.06.006
14. Higgins BW, McHeyzer-Williams LJ, McHeyzer-Williams MG. Programming isotype-specific plasma cell function. Trends Immunol. (2019) 40:345–57. doi: 10.1016/j.it.2019.01.012
15. Kim MS, Kim TS. IgA+ plasma cells in murine intestinal lamina propria as a positive regulator of Treg differentiation. J Leukoc Biol. (2014) 95:461–9. doi: 10.1189/jlb.0613310
16. Meng L, Almeida LN, Clauder AK, Lindemann T, Luther J, Link C, et al. Bone marrow plasma cells modulate local myeloid-lineage differentiation via IL-10. Front Immunol. (2019) 10:1183. doi: 10.3389/fimmu.2019.01183
17. Pioli PD, Casero D, Montecino-Rodriguez E, Morrison SL, Dorshkind K. Plasma cells are obligate effectors of enhanced myelopoiesis in aging bone marrow. Immunity. (2019) 51:351–66.e6. doi: 10.1016/j.immuni.2019.06.006
18. Rojas OL, Probstel AK, Porfilio EA, Wang AA, Charabati M, Sun T, et al. Recirculating intestinal IgA-producing cells regulate neuroinflammation via IL-10. Cell. (2019) 177:492–3. doi: 10.1016/j.cell.2019.03.037
19. Rauch PJ, Chudnovskiy A, Robbins CS, Weber GF, Etzrodt M, Hilgendorf I, et al. Innate response activator B cells protect against microbial sepsis. Science. (2012) 335:597–601. doi: 10.1126/science.1215173
20. Bermejo DA, Jackson SW, Gorosito-Serran M, Acosta-Rodriguez EV, Amezcua-Vesely MC, Sather BD, et al. Trypanosoma cruzi trans-sialidase initiates a program independent of the transcription factors RORgammat and Ahr that leads to IL-17 production by activated B cells. Nat Immunol. (2013) 14:514–22. doi: 10.1038/ni.2569
21. Shen P, Roch T, Lampropoulou V, O'Connor RA, Stervbo U, Hilgenberg E, et al. IL-35-producing B cells are critical regulators of immunity during autoimmune and infectious diseases. Nature. (2014) 507:366–70. doi: 10.1038/nature12979
22. Huang H, Benoist C, Mathis D. Rituximab specifically depletes short-lived autoreactive plasma cells in a mouse model of inflammatory arthritis. Proc Natl Acad Sci USA. (2010) 107:4658–63. doi: 10.1073/pnas.1001074107
23. Khodadadi L, Cheng Q, Alexander T, Sercan-Alp O, Klotsche J, Radbruch A, et al. Bortezomib plus continuous B cell depletion results in sustained plasma cell depletion and amelioration of lupus nephritis in NZB/W F1 mice. PLoS ONE. (2015) 10:e0135081. doi: 10.1371/journal.pone.0135081
24. Lu LD, Stump KL, Wallace NH, Dobrzanski P, Serdikoff C, Gingrich DE, et al. Depletion of autoreactive plasma cells and treatment of lupus nephritis in mice using CEP-33779, a novel, orally active, selective inhibitor of JAK2. J Immunol. (2011) 187:3840–53. doi: 10.4049/jimmunol.1101228
25. Neubert K, Meister S, Moser K, Weisel F, Maseda D, Amann K, et al. The proteasome inhibitor bortezomib depletes plasma cells and protects mice with lupus-like disease from nephritis. Nat Med. (2008) 14:748–55. doi: 10.1038/nm1763
26. Taddeo A, Khodadadi L, Voigt C, Mumtaz IM, Cheng Q, Moser K, et al. Long-lived plasma cells are early and constantly generated in New Zealand Black/New Zealand White F1 mice and their therapeutic depletion requires a combined targeting of autoreactive plasma cells and their precursors. Arthritis Res Ther. (2015) 17:39. doi: 10.1186/s13075-015-0551-3
27. Alexander T, Sarfert R, Klotsche J, Kuhl AA, Rubbert-Roth A, Lorenz HM, et al. The proteasome inhibitior bortezomib depletes plasma cells and ameliorates clinical manifestations of refractory systemic lupus erythematosus. Ann Rheum Dis. (2015) 74:1474–8. doi: 10.1136/annrheumdis-2014-206016
28. Hofmann K, Clauder AK, Manz RA. Targeting B cells and plasma cells in autoimmune diseases. Front Immunol. (2018) 9:835. doi: 10.3389/fimmu.2018.00835
29. Antonini M, Lo Conte M, Sorini C, Falcone M. How the interplay between the commensal microbiota, gut barrier integrity, and mucosal immunity regulates brain autoimmunity. Front Immunol. (2019) 10:1937. doi: 10.3389/fimmu.2019.01937
30. Matsumoto M, Baba A, Yokota T, Nishikawa H, Ohkawa Y, Kayama H, et al. Interleukin-10-producing plasmablasts exert regulatory function in autoimmune inflammation. Immunity. (2014) 41:1040–51. doi: 10.1016/j.immuni.2014.10.016
31. Chihara N, Aranami T, Oki S, Matsuoka T, Nakamura M, Kishida H, et al. Plasmablasts as migratory IgG-producing cells in the pathogenesis of neuromyelitis optica. PLoS ONE. (2013) 8:e83036. doi: 10.1371/journal.pone.0083036
32. Chihara N, Aranami T, Sato W, Miyazaki Y, Miyake S, Okamoto T, et al. Interleukin 6 signaling promotes anti-aquaporin 4 autoantibody production from plasmablasts in neuromyelitis optica. Proc Natl Acad Sci USA. (2011) 108:3701–6. doi: 10.1073/pnas.1017385108
33. Bennett JL, Lam C, Kalluri SR, Saikali P, Bautista K, Dupree C, et al. Intrathecal pathogenic anti-aquaporin-4 antibodies in early neuromyelitis optica. Ann Neurol. (2009) 66:617–29. doi: 10.1002/ana.21802
34. Chavele KM, Merry E, Ehrenstein MR. Cutting edge: circulating plasmablasts induce the differentiation of human T follicular helper cells via IL-6 production. J Immunol. (2015) 194:2482–5. doi: 10.4049/jimmunol.1401190
35. Eggers EL, Michel BA, Wu H, Wang SZ, Bevan CJ, Abounasr A, et al. Clonal relationships of CSF B cells in treatment-naive multiple sclerosis patients. JCI Insight. (2017) 2:92724. doi: 10.1172/jci.insight.92724
36. Zehentmeier S, Roth K, Cseresnyes Z, Sercan O, Horn K, Niesner RA, et al. Static and dynamic components synergize to form a stable survival niche for bone marrow plasma cells. Eur J Immunol. (2014) 44:2306–17. doi: 10.1002/eji.201344313
37. Minges Wols HA, Underhill GH, Kansas GS, Witte PL. The role of bone marrow-derived stromal cells in the maintenance of plasma cell longevity. J Immunol. (2002) 169:4213–21. doi: 10.4049/jimmunol.169.8.4213
38. Crane GM, Jeffery E, Morrison SJ. Adult haematopoietic stem cell niches. Nat Rev Immunol. (2017) 17:573–90. doi: 10.1038/nri.2017.53
39. Tsujimoto T, Lisukov IA, Huang N, Mahmoud MS, Kawano MM. Plasma cells induce apoptosis of pre-B cells by interacting with bone marrow stromal cells. Blood. (1996) 87:3375–83. doi: 10.1182/blood.V87.8.3375.bloodjournal8783375
40. Jahnsen FL, Baekkevold ES, Hov JR, Landsverk OJ. Do long-lived plasma cells maintain a healthy microbiota in the gut? Trends Immunol. (2018) 39:196–208. doi: 10.1016/j.it.2017.10.006
41. Pabst O, Cerovic V, Hornef M. Secretory IgA in the coordination of establishment and maintenance of the microbiota. Trends Immunol. (2016) 37:287–96. doi: 10.1016/j.it.2016.03.002
42. Kunisawa J, Gohda M, Hashimoto E, Ishikawa I, Higuchi M, Suzuki Y, et al. Microbe-dependent CD11b+ IgA+ plasma cells mediate robust early-phase intestinal IgA responses in mice. Nat Commun. (2013) 4:1772. doi: 10.1038/ncomms2718
43. Fritz JH, Rojas OL, Simard N, McCarthy DD, Hapfelmeier S, Rubino S, et al. Acquisition of a multifunctional IgA+ plasma cell phenotype in the gut. Nature. (2011) 481:199–203. doi: 10.1038/nature10698
44. Lee MR, Seo GY, Kim YM, Kim PH. iNOS potentiates mouse Ig isotype switching through AID expression. Biochem Biophys Res Commun. (2011) 410:602–7. doi: 10.1016/j.bbrc.2011.06.035
45. Rubin DC, Shaker A, Levin MS. Chronic intestinal inflammation: inflammatory bowel disease and colitis-associated colon cancer. Front Immunol. (2012) 3:107. doi: 10.3389/fimmu.2012.00107
46. Blanc P, Moro-Sibilot L, Barthly L, Jagot F, This S, de Bernard S, et al. Mature IgM-expressing plasma cells sense antigen and develop competence for cytokine production upon antigenic challenge. Nat Commun. (2016) 7:13600. doi: 10.1038/ncomms13600
47. Pinto D, Montani E, Bolli M, Garavaglia G, Sallusto F, Lanzavecchia A, et al. A functional BCR in human IgA and IgM plasma cells. Blood. (2013) 121:4110–4. doi: 10.1182/blood-2012-09-459289
48. Achatz-Straussberger G, Zaborsky N, Konigsberger S, Luger EO, Lamers M, Crameri R, et al. Migration of antibody secreting cells towards CXCL12 depends on the isotype that forms the BCR. Eur J Immunol. (2008) 38:3167–77. doi: 10.1002/eji.200838456
49. Ergen AV, Boles NC, Goodell MA. Rantes/Ccl5 influences hematopoietic stem cell subtypes and causes myeloid skewing. Blood. (2012) 119:2500–9. doi: 10.1182/blood-2011-11-391730
50. Kallies A, Nutt SL. Terminal differentiation of lymphocytes depends on Blimp-1. Curr Opin Immunol. (2007) 19:156–62. doi: 10.1016/j.coi.2007.01.003
51. Minnich M, Tagoh H, Bonelt P, Axelsson E, Fischer M, Cebolla B, et al. Multifunctional role of the transcription factor Blimp-1 in coordinating plasma cell differentiation. Nat Immunol. (2016) 17:331–43. doi: 10.1038/ni.3349
52. Dorner M, Brandt S, Tinguely M, Zucol F, Bourquin JP, Zauner L, et al. Plasma cell toll-like receptor (TLR) expression differs from that of B cells, and plasma cell TLR triggering enhances immunoglobulin production. Immunology. (2009) 128:573–9. doi: 10.1111/j.1365-2567.2009.03143.x
53. Abdi J, Mutis T, Garssen J, Redegeld F. Characterization of the Toll-like receptor expression profile in human multiple myeloma cells. PLoS ONE. (2013) 8:e60671. doi: 10.1371/journal.pone.0060671
54. Bohnhorst J, Rasmussen T, Moen SH, Flottum M, Knudsen L, Borset M, et al. Toll-like receptors mediate proliferation and survival of multiple myeloma cells. Leukemia. (2006) 20:1138–44. doi: 10.1038/sj.leu.2404225
55. Bagratuni T, Sklirou AD, Kastritis E, Liacos CI, Spilioti C, Eleutherakis-Papaiakovou E, et al. Toll-Like receptor 4 activation promotes multiple myeloma cell growth and survival via suppression of the endoplasmic reticulum stress factor chop. Sci Rep. (2019) 9:3245. doi: 10.1038/s41598-019-39672-7
56. Bohannon C, Powers R, Satyabhama L, Cui A, Tipton C, Michaeli M, et al. Long-lived antigen-induced IgM plasma cells demonstrate somatic mutations and contribute to long-term protection. Nat Commun. (2016) 7:11826. doi: 10.1038/ncomms11826
57. Cassese G, Lindenau S, de Boer B, Arce S, Hauser A, Riemekasten G, et al. Inflamed kidneys of NZB / W mice are a major site for the homeostasis of plasma cells. Eur J Immunol. (2001) 31:2726–32. doi: 10.1002/1521-4141(200109)31:9<2726::aid-immu2726>3.0.co;2-h
58. Lemke A, Kraft M, Roth K, Riedel R, Lammerding D, Hauser AE. Long-lived plasma cells are generated in mucosal immune responses and contribute to the bone marrow plasma cell pool in mice. Mucosal Immunol. (2016) 9:83–97. doi: 10.1038/mi.2015.38
59. Slifka MK, Matloubian M, Ahmed R. Bone marrow is a major site of long-term antibody production after acute viral infection. J Virol. (1995) 69:1895–902.
60. Ellyard JI, Avery DT, Phan TG, Hare NJ, Hodgkin PD, Tangye SG. Antigen-selected, immunoglobulin-secreting cells persist in human spleen and bone marrow. Blood. (2004) 103:3805–12. doi: 10.1182/blood-2003-09-3109
61. Rossi DJ, Bryder D, Weissman IL. Hematopoietic stem cell aging: mechanism and consequence. Exp Gerontol. (2007) 42:385–90. doi: 10.1016/j.exger.2006.11.019
62. Signer RA, Montecino-Rodriguez E, Witte ON, McLaughlin J, Dorshkind K. Age-related defects in B lymphopoiesis underlie the myeloid dominance of adult leukemia. Blood. (2007) 110:1831–9. doi: 10.1182/blood-2007-01-069401
63. Pang WW, Price EA, Sahoo D, Beerman I, Maloney WJ, Rossi DJ, et al. Human bone marrow hematopoietic stem cells are increased in frequency and myeloid-biased with age. Proc Natl Acad Sci USA. (2011) 108:20012–7. doi: 10.1073/pnas.1116110108
64. Beerman I, Bhattacharya D, Zandi S, Sigvardsson M, Weissman IL, Bryder D, et al. Functionally distinct hematopoietic stem cells modulate hematopoietic lineage potential during aging by a mechanism of clonal expansion. Proc Natl Acad Sci USA. (2010) 107:5465–70. doi: 10.1073/pnas.1000834107
65. Benz C, Copley MR, Kent DG, Wohrer S, Cortes A, Aghaeepour N, et al. Hematopoietic stem cell subtypes expand differentially during development and display distinct lymphopoietic programs. Cell Stem Cell. (2012) 10:273–83. doi: 10.1016/j.stem.2012.02.007
66. Montecino-Rodriguez E, Kong Y, Casero D, Rouault A, Dorshkind K, Pioli PD. Lymphoid-biased hematopoietic stem cells are maintained with age and efficiently generate lymphoid progeny. Stem Cell Rep. (2019) 12:584–96. doi: 10.1016/j.stemcr.2019.01.016
67. McElhaney JE. The unmet need in the elderly: designing new influenza vaccines for older adults. Vaccine. (2005) 23(Suppl 1):S10–25. doi: 10.1016/j.vaccine.2005.04.019
68. Cancro MP, Hao Y, Scholz JL, Riley RL, Frasca D, Dunn-Walters DK, et al. B cells and aging: molecules and mechanisms. Trends Immunol. (2009) 30:313–8. doi: 10.1016/j.it.2009.04.005
69. Lino AC, Dang VD, Lampropoulou V, Welle A, Joedicke J, Pohar J, et al. LAG-3 inhibitory receptor expression identifies immunosuppressive natural regulatory plasma cells. Immunity. (2018) 49:120–33 e129. doi: 10.1016/j.immuni.2018.06.007
70. Huang CT, Workman CJ, Flies D, Pan X, Marson AL, Zhou G, et al. Role of LAG-3 in regulatory T cells. Immunity. (2004) 21:503–13. doi: 10.1016/j.immuni.2004.08.010
71. Runcie K, Budman DR, John V, Seetharamu N. Bi-specific and tri-specific antibodies- the next big thing in solid tumor therapeutics. Mol Med. (2018) 24:50. doi: 10.1186/s10020-018-0051-4
72. Pietras EM. Inflammation: a key regulator of hematopoietic stem cell fate in health and disease. Blood. (2017) 130:1693–8. doi: 10.1182/blood-2017-06-780882
73. McCarron MJ, Park PW, Fooksman DR. CD138 mediates selection of mature plasma cells by regulating their survival. Blood. (2017) 129:2749–59. doi: 10.1182/blood-2017-01-761643
74. Blanco E, Perez-Andres M, Arriba-Mendez S, Contreras-Sanfeliciano T, Criado I, Pelak O, et al. Age-associated distribution of normal B-cell and plasma cell subsets in peripheral blood. J Allergy Clin Immunol. (2018) 141:2208–19 e2216. doi: 10.1016/j.jaci.2018.02.017
75. Gustafson CE, Higbee D, Yeckes AR, Wilson CC, De Zoeten EF, Jedlicka P, et al. Limited expression of APRIL and its receptors prior to intestinal IgA plasma cell development during human infancy. Mucosal Immunol. (2014) 7:467–77. doi: 10.1038/mi.2013.64
76. Lam WY, Jash A, Yao CH, D'Souza L, Wong R, Nunley RM, et al. Metabolic and transcriptional modules independently diversify plasma cell lifespan and function. Cell Rep. (2018) 24:2479–92 e2476. doi: 10.1016/j.celrep.2018.07.084
Keywords: plasmablasts, plasma cells, inflammation, hematopoiesis, Toll-like receptor, immunoglobulin
Citation: Pioli PD (2019) Plasma Cells, the Next Generation: Beyond Antibody Secretion. Front. Immunol. 10:2768. doi: 10.3389/fimmu.2019.02768
Received: 10 September 2019; Accepted: 12 November 2019;
Published: 22 November 2019.
Edited by:
Deborah K. Dunn-Walters, University of Surrey, United KingdomReviewed by:
Michael Zemlin, Saarland University Hospital, GermanyAnja Erika Hauser, Charité Medical University of Berlin, Germany
Copyright © 2019 Pioli. This is an open-access article distributed under the terms of the Creative Commons Attribution License (CC BY). The use, distribution or reproduction in other forums is permitted, provided the original author(s) and the copyright owner(s) are credited and that the original publication in this journal is cited, in accordance with accepted academic practice. No use, distribution or reproduction is permitted which does not comply with these terms.
*Correspondence: Peter D. Pioli, peter.pioli@med.wmich.edu