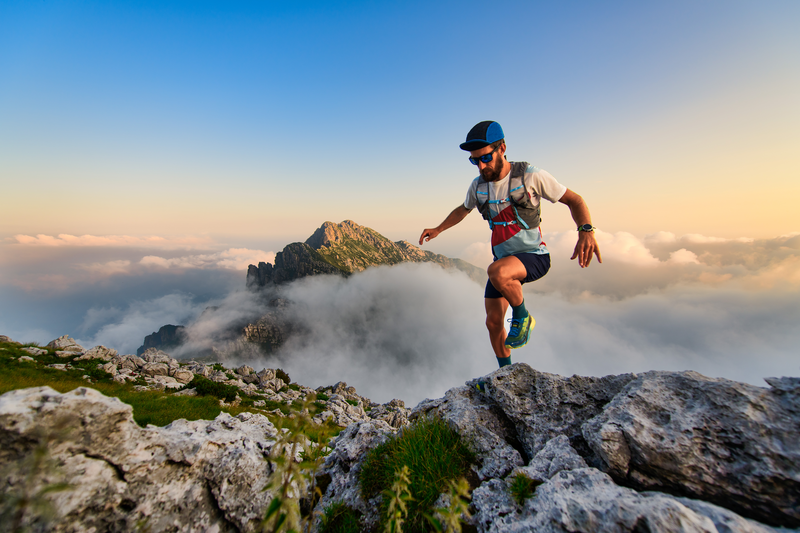
94% of researchers rate our articles as excellent or good
Learn more about the work of our research integrity team to safeguard the quality of each article we publish.
Find out more
MINI REVIEW article
Front. Immunol. , 22 November 2019
Sec. Comparative Immunology
Volume 10 - 2019 | https://doi.org/10.3389/fimmu.2019.02732
This article is part of the Research Topic Immunoparasitology: A Unique Interplay Between Host and Pathogen View all 32 articles
Avian coccidiosis is caused by Eimeria, which is an intracellular apicomplexan parasite that invades through the intestinal tract to cause devastating disease. Upon invasion through the intestinal epithelial cells, a strong inflammatory response is induced that results in complete villous destruction, diarrhea, hemorrhage, and in severe cases, death. Since the life cycle of Eimeria parasites is complex and comprises several intra- and extracellular developmental stages, the host immune responses are diverse and complex. Interferon-γ-mediated T helper (Th)1 response was originally considered to be the predominant immune response in avian coccidiosis. However, recent studies on other avian T cell lineages such as Th17 and T regulatory cells have implicated their significant involvement in maintaining gut homeostasis in normal and disease states including coccidiosis. Therefore, there is a need to understand better their role in coccidiosis. This review focuses on research findings concerning the host immune response induced by avian coccidiosis in the context of T cell immunity, including expression of T-cell-related cytokines and surface molecules that determine the phenotype of T lymphocytes.
Avian coccidiosis is caused by intracellular protozoan parasites that belong to several different species of Eimeria (1, 2). This apicomplexan parasite invades intestinal epithelial tissues and causes severe damage in birds, resulting in enormous economic losses in the poultry industry. The major challenge in coccidiosis control is the diversity among several Eimeria species that target different specific regions of the intestine.
The coccidia exhibit a complex life cycle comprising both intracellular and extracellular stages as well as asexual and sexual reproduction (3, 4). The life cycle mainly consists of an exogenous stage, characterized by excretion of unsporulated oocysts, and endogenous stage of schizogony (asexual reproduction) and gametogony (sexual differentiation) (5, 6). During the exogenous stage, the unsporulated oocysts become sporulated (with four sporocysts, each containing two sporozoites) under the influence of external environmental factors such as moisture, oxygen, and warmth. The endogenous stage occurs inside the host, which involves several stages of asexual reproduction followed by sexual reproduction, fertilization, and shedding of the unsporulated oocysts. In general, two to four generations of asexual reproduction are followed by the sexual phase, in which zygote formation takes place that eventually matures into oocysts that are released in the intestinal mucosa and finally shed into feces (7). The coccidia life cycle is usually short (4–6 days depending on several different species) and production of sporulating oocysts can easily increase the infectivity of the parasites in a large population of chickens. After ingesting the sporulated oocysts, excystation of oocysts occurs in the gizzard and the sporozoites are released, invade the intestinal cells, and cause severe damage as the reproductive cycle of the parasite begins. As a result, symptoms such as bloody diarrhea and reduced body weight and feed intake are observed in the birds.
Upon exposure to developing schizonts, anti-Eimeria immunity develops and is subsequently boosted by multiple re-exposures to oocysts (7). The immunity to avian coccidiosis can be categorized as innate and adaptive (8). As a first line of defense, the innate immune response is activated in response to the conserved antigens. Innate immune responses include recognition of conserved pathogen-associated molecular patterns (PAMPs) by pattern recognition receptors (PRRs) such as Toll-like receptors (TLRs) (5, 9, 10). A major TLR ligand, profilin, is expressed in all the developmental stages of the life cycle of several Eimeria parasites and is conserved (11). Such ligands induce a robust innate response such as immune cell proliferation and cytokine production. The cells involved in innate immune responses to Eimeria parasites at different phases are natural killer (NK) cells, dendritic cells, epithelial cells, heterophils, and macrophages. In particular, macrophage migration inhibitory factor plays a crucial role in mediating innate immunity in coccidiosis (12).
On the other hand, adaptive immunity is specific and regulates the antigen-specific immune responses to prevent colonization and growth of the pathogen inside the host. Like mammals, two major lymphocyte types, B cells (producing surface immunoglobulins) and T cells (T cell receptors), are the major components of adaptive immune responses in birds (13). Anticoccidial antibodies in serum and mucosal secretions have been reported in avian coccidiosis (13). Although B cell depletion studies (14) have revealed that antibodies do not play a specific role in anticoccidial protective immunity, other studies have emphasized the importance of passively transferred humoral immunity in Eimeria infection in chickens (15–18). Cell-mediated immunity in avian coccidiosis is characterized by antigen-specific or non-specific activation of several immune cells such as T cells, NK cells, and macrophages. The CD4+ T helper (Th) cells and CD8+ cytotoxic T lymphocytes (CTLs) are the two major T-cell subsets that are involved in anticoccidial immunity (19–22). Although the role of several T-cell subpopulations in avian coccidiosis remains to be elucidated, T cells are the most important for protection against Eimeria infections in birds.
In this article, we reviewed the historical progress of immunological studies on the host immune response to avian coccidiosis, with an emphasis on recent findings in the understanding of the complexity of T-cell immune responses in avian coccidiosis, especially those mediated by Th17 and T regulatory (Treg) cells.
Since the first report of chicken coccidiosis in the cecum in the late eighteenth century (1), immunity to several Eimeria parasites has been investigated thoroughly. An important contribution from Rose and colleagues (23) defined the basic principles of avian immunity to coccidial parasites in terms of specificity, wherein one species of Eimeria offers little protection against heterologous challenge with other species. Over the past few decades, studies focusing on investigating the role of avian immunity in response to various coccidial parasites has shown promising developments toward better understanding of avian immunity to coccidiosis (20, 24, 25). From all these studies, it was apparent that out of the two types of immunity, cellular immunity was more important than humoral immunity in coccidiosis, as the later offered little protection against the infection. Early investigations in both mammalian and avian species have revealed that the cellular immune responses through T cells and their associated cytokines play an important role in anticoccidial immunity (2, 26, 27). Acquired immunity to murine coccidiosis is attributed more to T cells than B cells (26). Several immune cell types including NK cells, dendritic cells, and macrophages are involved in innate immune responses to avian coccidiosis (8, 27). B-cell-deficient chickens have shown increased oocyst production after primary infection with Eimeria species. However, secondary infection does not yield clinical coccidiosis in the bursectomized chickens due to the protective immunity acquired by the primary infection (8). This indicates that the anticoccidial immunity acquired after primary infection is B cell independent. It is also apparent that chicken coccidiosis can be prevented by adaptive transfer of peripheral blood lymphocytes and splenocytes from Eimeria-infected chickens in the syngeneic recipients (28). Subsequently, the T-cell immunosuppressant cyclosporin A abolished the protective immunity offered by Eimeria re-infection, thus further emphasizing the integral role of cellular immune mechanisms in chicken coccidiosis (14).
The early findings indicated that T cells serve as a key factor to mediate anticoccidial immunity in chickens (8, 14). Greater numbers of CTLs expressing CD8 cell surface antigen were predominantly observed in chickens after primary infection (29–31). Furthermore, the differential role of CD4+ and CD8+ T lymphocytes in offering resistance to primary and secondary coccidial infection was also reported (32, 33). Increased populations of T cells are linked to elevated production of proinflammatory cytokine interferon (IFN)-γ, which has an immunoregulatory effect (34), as well as inhibiting intracellular development of the parasite (35, 36). The role of T cells in mediating host immunity to coccidiosis became more evident when flow cytometric analysis of intestinal epithelial lymphocytes (IELs), using lymphocyte-specific immune reagents, revealed their significance in innate immunity in naïve chickens and adaptive immunity in previously infected chickens (19, 37, 38). More studies showed that different IEL subtypes are involved in anti-Eimeria defense in the gut (39, 40). Research over the past several years has shown that, as a part of protective immunity against avian coccidiosis, T cells produce numerous secretions besides IFN-γ, such as cytokines interleukin (IL)-1, IL-2, IL-4–6, IL-8, IL-10, IL-12, IL-13, and IL-15–18, tumor necrosis factor (TNF)-α, lipopolysaccharide-induced TNF-α factor (LITAF), TNF-α superfamily 15 (TNFSF15), transforming growth factor (TGF)-β1–4, and granulocyte–macrophage colony-stimulatory factor (GM-CSF). All these findings are based on research oriented toward investigating the immunoregulatory responses of these molecules after primary and/or secondary coccidiosis (40–60). More recent work has indicated the involvement of TLR4 and TLR15 as a part of the innate immune response to Eimeria infection (61). IL-17 also contributes to host immunopathology in response to experimental infection (62). The immunoproteomics analysis of three Eimeria species has identified several immunodominant antigens from these three species that could provide a useful breakthrough in exploring anticoccidial immunity, as some of these molecules cause profuse inflammatory and cellular immune response that contribute to pathogenesis and severity of infection (3, 63). Additionally, research on anticoccidial vaccines and natural alternatives has explored the immunobiology of coccidiosis in poultry (7). All these findings show the immunoregulatory effect of vaccines or several naturally occurring anti-inflammatory products such as curcumin and Allium hookeri. These findings also provide a useful insight into immunoregulation in avian coccidiosis, however, this is outside the scope of this review and has been reviewed previously (7). Much of this work has focused on immunomodulation by dietary ingredients in experimental Eimeria infections (64, 65).
Besides the above information, immunological variation among the different strains of the same Eimeria species has also been reported in chickens (66, 67). These findings show the characteristic intraspecific variations attributed to the biological features of Eimeria, such as morphology of oocysts, pathogenicity, and sensitivity to drugs (68). This inter- and intraspecies variation has been recently defined with the help of more advanced molecular approaches such as random amplification of polymorphic DNA–PCR, and restricted or amplified fragment length polymorphism (69). Analysis of these variations has led to the identification of several strain-specific immunoprotective antigens (70, 71). Similarly, more recent findings have also highlighted the variation in immune responses to Eimeria tenella infection in genetically distinct chicken lineages (72).
Among all the cytokines mentioned above, IFN-γ is a major cytokine that has anticoccidial effects (73). In mammals, parasitic infections are often characterized by increased levels of IFN-γ. Similarly, the functional role of this cytokine in Eimeria infections has been studied thoroughly (34, 74, 75). Until the cDNA cloning of chicken IFNs revealed the independent existence of type I (76) (IFN-α) and type II (77) (IFN-γ) IFNs, most of the findings on the role of IFN-like activity in Eimeria infections were believed to be associated with IFN-γ. All these IFN-dependent activities inhibit the invasion or development of Eimeria in cultured cells in vitro (73, 78). In vivo studies have also revealed the anticoccidial IFN-like activity in Eimeria-infected birds (79, 80). The specific involvement of IFN-γ in anti-Eimeria immunity was later described by Breed et al. who showed that IFN-γ was produced specifically after stimulation of peripheral blood lymphocytes from Eimeria-infected birds (31). It was then discovered that mitogen- or antigen-stimulated specific T cells circulating in the blood of Eimeria-infected chickens specifically produced IFN-γ (81). Based on these findings, it was proposed that T-cell priming might occur at the site of infection, resulting in production of IFN-γ at the infection site, thus regulating anticoccidial immunity (81). It was also hypothesized that CD8+ cells produce IFN-γ, which is involved in immunoregulation in primary coccidiosis (81). These findings were extended by Rothwell et al. who showed that IFN-γ-producing cells were present in blood and the spleen and may migrate from the spleen after secondary infection (82). In situ hybridization has shown that, following Eimeria challenge, IFN-γ is produced by the cells (predominantly T cells) at the site of infection (cecum) and by splenocytes (82). Several studies have shown the potential application of IFN-γ in protecting against Eimeria infections (36, 83, 84). Birds immunized with recombinant IFN-γ show increased body weight gain during infection with Eimeria acevirulina (36, 83). Also, the development of E. tenella is inhibited by IFN-γ in vitro (36, 84). When chicken cells are treated with recombinant IFN-γ, intracellular development of E. tenella is inhibited, with no significant effect on sporozoite invasion of the cells (36). Similarly, in vivo administration of recombinant IFN-γ protects against E. acevirulina characterized by reduced oocyst production and increased body weight gain (36, 83).
Besides its immunoregulatory or immunoprotective effect against chicken coccidiosis, IFN-γ has also been shown to have an adjuvant effect on coccidial vaccine in Eimeria-infected chickens (85). The adjuvant effect of IFN-γ is characterized by enhanced immune response to the vaccine antigen that induces a microbicidal effect to resolve the parasitic infection, thus increasing vaccine efficacy (85). Some DNA vaccines administered with IFN-γ increased the immunity at intestinal level and protected against avian coccidiosis (36, 86, 87). Recent studies have also indicated the beneficial effect of IFN-γ on anticoccidial DNA vaccine (88, 89). A chimeric vaccine constructed by fusion of genes encoding the E. tenella surface antigen, and IFN-γ alleviated the cecal lesions and improved the anticoccidial index in experimentally infected chickens, further suggesting the adjuvant effect of IFN-γ (89). Thus, all these efforts indicate the anticoccidial role of IFN-γ, direct, or as an adjuvant, and underline the significance of IFN-γ in anticoccidial immunity.
Besides the response elicited by IFN-γ-mediated Th1 cells against avian coccidiosis, the other CD4 T-cell subsets have also been studied since the discovery of their homologs in mammals (42). A lineage of IL-17-producing CD4+ T helper (Th)17 cells that are distinct from the previously well-characterized Th1/Th2 paradigm, has emerged and is involved in proinflammatory responses in various autoimmune diseases and infections (90). The biological activities of IL-17 as a signature cytokine of Th17 cells include recruitment of neutrophils, stimulation of antimicrobial peptide production, such as β-defensins and mucins, as well as induction of cytokines and chemokines, in particular IL-6, CXCL8 and GM-CSF (91). Chicken IL-17 isolated from Eimeria-infected IELs exerts a proinflammatory role in coccidiosis (8). The exact role of Th17 cells in chicken is poorly understood due to the lack of immunological reagents. This section describes studies that focused on the role of IL-17 as a signature cytokine in Th17 cells in chicken coccidiosis. Following infection by E. acervulina or E. maxima, IL-17 mRNA levels were increased in IELs compared to uninfected controls (40). In E. tenella infection, IL-17 expression in IELs was downregulated, except in the latter stage of infection (39). Similarly, Kim et al. reported that chicken IL-17 expression was downregulated in inflamed intestinal tissue following E. tenella infection, and treatment with IL-17 or IL-17F induced expression of proinflammatory cytokines in chicken fibroblasts (92). These results suggest that chicken coccidiosis induces IL-17 expression in the gut and is dependent on the species of Eimeria. Th17 response can play both protective and pathological roles in protozoan infections. The cloning of IL-17 receptor A (IL-17RA), which binds IL-17A and IL-17F in chickens, has revealed that Eimeria infection downregulates expression of IL-17RA, and modulation of this receptor facilitates the host to reduce intestinal pathogenesis amplified by IL-17/IL-17RA signaling. Several authors have proposed that Th17 cells or IL-17 promote pathogenesis in leishmaniasis, toxoplasmosis, and Eimeria falciformis infection (93–95), whereas others have demonstrated that they are involved in protective immunity against trypanosomiasis, toxoplasmosis and Pneumocystis carinii infection (96, 97). Recent evidence seems to support a role for Th17 cytokines in host immunopathology in coccidiosis in chickens. Treatment with IL-17 neutralizing antibody in E. tenella infection induces lower heterophil recruitment, inflammatory cytokine expression, and parasite burden in the intestinal tract, resulting in enhanced body weight gain, reduced oocyst production in feces, and intestinal lesions (62). IL-17 is also involved in the initiation and migratory response of epithelial cells during intracellular development, and maturation of parasites, contributing to pathogenesis in the intestinal tract. Following E. tenella infection, chickens treated with IL-17 neutralizing antibody have a reduced number of second-generation schizonts and cecal lesions (98).
Treg cells are a subset of T cells involved in immunosuppression. Mammalian Treg cells have the phenotype CD4+CD25+FoxP3+ (99). In chickens, the ortholog of mammalian FoxP3 has yet to be identified, although there is a report of an avian foxp3 gene (100, 101). Thus, CD4+CD25+ T cells in chickens have been characterized as Treg cells showing suppression of activated immune cells (102). These cells produce high amounts of IL-10, TGF-β, CTLA-4, and LAG-3, as in mammals (103). IL-10 showed 29-fold higher expression in CD4+CD25+ cells compared to CD4+CD25− cells and its immunosuppression in chickens has been extensively studied (102). In coccidiosis, IL-10 is considered to play an important role in evasion of the host immune response. One possible mechanism to explain its role in coccidiosis is that coccidial parasites have evolved to stimulate Treg cells to express IL-10, and it helps parasites to facilitate invasion and survival in chickens through suppression of the IFN-γ-related Th1 response that is critical for protective immunity against coccidial parasites. Two inbred lines of chickens that differ in their resistance or susceptibility to Eimeria infection have revealed that expression of IL-10 is the major difference between the two lines. Expression of IL-10 is highly induced in susceptible chickens among the genes related to different Th lineages, such as IFN-γ for Th1, IL-4 for Th2, and IL-10 and TGF-β for Treg cells, while IL-10 is suppressed in the age-matched resistant line (46). Eimeria-infected chickens treated with IL-10 neutralizing antibody show improved growth rate compared to those with control antibody but it has no effect on fecal oocyst production (104, 105). Morris et al. reported that supplementation of vitamin D induced IL-10 expression as well as Treg cells and showed decreased production losses associated with coccidial infection (106). These results indicate that regulation of the protective immune response to Eimeria infection by Treg cells is critical, and IL-10 plays a role in pathogenesis in chicken coccidiosis. We recently identified that Treg cells could help to reduce pathology in Eimeria-infected intestine by suppression of Th17 cells that induce tissue inflammation. Increased expression of CD4+CD25+ Treg cells has been found in E. tenella-infected chickens with increased IL-10 expression. After treatment with aryl hydrocarbon receptor such as 3,3′-diindolylmethane, Treg cells are increased in the intestine, whereas CD4+IL-17+ Th17 cells are suppressed. We have also found that generation of Th17 cells is suppressed by Treg cells, which leads to reduced pathogenicity in chicken coccidiosis (107).
It is the consensus that the Th1 response is the most efficient host response in avian coccidiosis. However, studies on other aspects like Th17 and Treg responses are also important because the immune responses are not independent, but rather they are connected and work together in an integrated immune system. It is becoming clear that the outcome of an inflammatory process caused by infection depends on the balance of responses by several components of the immune system of particular relevance is the interplay between Treg and Th17 cells during immunoinflammatory events (108). Compared to mammalian immunology, little is known about the role of T cells in chickens, although the number of reports on coccidiosis is steadily growing. To understand better immunity against chicken coccidiosis, it is necessary to know how T cells are modulated and how they interplay since this intracellular pathogen predominantly induces a T-cell-associated immune response that involves several types of T cells. In regard to controlling coccidiosis, the best way might be development of alternatives to antibiotics because most effective anticoccidial drugs that produce resistance or residues will be banned from the market in the future. Understanding the mechanism of how chickens respond to Eimeria will lead to new approaches to control coccidiosis.
All authors listed have made a substantial, direct and intellectual contribution to the work, and approved it for publication.
This research was supported, in part, by an ARS CRIS project 8042-32000-106-00D and by Development of Poultry Immune Reagent program (Award No: 2017-67015-26793) funded by USDA/NIFA.
The authors declare that the research was conducted in the absence of any commercial or financial relationships that could be construed as a potential conflict of interest.
1. Railliet ALA. Developpement experimental des coccidies de l'epithelium intestinal du lapin et de la poule. C R Soc Biol Paris. (1891) 36:820–3.
2. Rose ME, Hesketh P. Immunity to coccidiosis : t-lymphocyte- deficient animals B-lymphocyte-. Immunology. (1979) 26:630–7.
3. Lal K, Bromley E, Oakes R, Prieto JH, Sanderson SJ, Kurian D, et al. Proteomic comparison of four Eimeria tenella life-cycle stages: Unsporulated oocyst, sporulated oocyst, sporozoite and second-generation merozoite. Proteomics. (2009) 9:4566–76. doi: 10.1002/pmic.200900305
4. Norton CC, Chard MJ. The oocyst sporulation time of Eimeria species from the fowl. Parasitology. (1983) 86:193–8. doi: 10.1017/S0031182000050368
5. Dalloul RA, Lillehoj HS. Recent advances in immunomodulation and vaccination strategies against coccidiosis. Avian Dis. (2005) 49:1–8. doi: 10.1637/7306-11150R
6. Gilbert ER, Cox CM, Williams PM, McElroy AP, Dalloul RA, Keith Ray W, et al. Eimeria species and genetic background influence the serum protein profile of broilers with coccidiosis. PLoS ONE. (2011) 6:e14636. doi: 10.1371/journal.pone.0014636
7. Quiroz-Castañeda RE, Dantán-González E. Control of avian coccidiosis: future and present natural alternatives. BioMed Res Int. (2015) 2015:430610. doi: 10.1155/2015/430610
8. Min W, Kim WH, Lillehoj EP, Lillehoj HS. Recent progress in host immunity to avian coccidiosis: IL-17 family cytokines as sentinels of the intestinal mucosa. Dev Compar Immunol. (2013) 41:418–28. doi: 10.1016/j.dci.2013.04.003
9. Kumar H, Kawai T, Akira S. Pathogen recognition by the innate immune system. Int Rev Immunol. (2011) 30:16–34. doi: 10.3109/08830185.2010.529976
10. Brownlie R, Allan B. Avian toll-like receptors. Cell Tissue Res. (2011) 343:121–30. doi: 10.1007/s00441-010-1026-0
11. Fetterer RH, Miska KB, Jenkins MC, Barfield RC. A conserved 19-kDa Eimeria tenella antigen is a profilin-like protein. J Parasitol. (2004) 90:1321–8. doi: 10.1645/GE-307R
12. Sun H-W, Bernhagentt J, Bucalat R, Lolis E. Crystal structure at 2.6-A resolution of human macrophage migration inhibitory factor. Proc Natl Acad Sci USA. (1996) 93:5191–6. doi: 10.1073/pnas.93.11.5191
13. Girard F, Fort G, Yvore P, Quere P. Kinetics of specific immunoglobulin A, M and G production in the duodenal and caecal mucosa of chickens infected with Eimeria acervulina or Eimeria tenella. Int J Parasitol. (1997) 27:803–9. doi: 10.1016/S0020-7519(97)00044-1
14. Lillehoj HS. Effects of immunosuppression on avian coccidiosis: cyclosporin A but not hormonal bursectomy abrogates host protective immunity. Infect Immun. (1987) 55:1616–21.
15. Wallach M, Halabi A, Pillemer G, Sar-Shalom O, Mencher D, Gilad M, et al. Maternal immunization with gametocyte antigens as a means of providing protective immunity against Eimeria maxima in chickens. Infect Immun. (1992) 60:2036–9.
16. Wallach M. Role of antibody in immunity and control of chicken coccidiosis. Trends Parasitol. (2010) 26:382–7. doi: 10.1016/j.pt.2010.04.004
17. Lee SH, Lillehoj HS, Park DW, Jang SI, Morales A, García D, et al. Induction of passive immunity in broiler chickens against Eimeria acervulina by hyperimmune egg yolk immunoglobulin Y. Poultry Science. (2009) 88:562–6. doi: 10.3382/ps.2008-00340
18. Lee SH, Lillehoj HS, Park DW, Jang SI, Morales A, García D, et al. Protective effect of hyperimmune egg yolk IgY antibodies against Eimeria tenella and Eimeria maxima infections. Vet Parasitol. (2009) 163:123–6. doi: 10.1016/j.vetpar.2009.04.020
19. Lillehoj HS. Role of T lymphocytes and cytokines in coccidiosis. Int J Parasitol. (1998) 28:1071–81. doi: 10.1016/S0020-7519(98)00075-7
20. Lillehoj HS, Lillehoj EP. Avian coccidiosis. A review of acquired intestinal immunity and vaccination strategies. Avian Dis. (2000) 44:408–25. doi: 10.2307/1592556
21. Trout JM, Lillehoj HS. T lymphocyte roles during Eimeria acervulina and Eimeria tenella infections. Vet Immunol Immunopathol. (1996) 53:163–72. doi: 10.1016/0165-2427(95)05544-4
22. Yun CH, Lillehoj HS, Lillehoj EP. Intestinal immune responses to coccidiosis. Dev Compar Immunol. (2000) 24:303–24. doi: 10.1016/S0145-305X(99)00080-4
24. Allen PC, Fetterer RH. Recent advances in biology and immunobiology of Eimeria species and in diagnosis and control of infection with these coccidian parasites of poultry. Clin Microbiol Rev. (2002) 15:58–65. doi: 10.1128/CMR.15.1.58-65.2002
25. Shirley MW, Lillehoj HS. The long view: a selective review of 40 years of coccidiosis research. Avian Pathol. (2012) 41:111–21. doi: 10.1080/03079457.2012.666338
26. Rose ME, Ogilvie BM, Hesketh P, Festing MFW. Failure of nude. (athymic) rats to become resistant to reinfection with the intestinal coccidian parasite Eimeria nieschulzi or the nematode Nippostrongylus brasiliensis. Parasite Immunol. (1979) 1:125–32. doi: 10.1111/j.1365-3024.1979.tb00700.x
27. Wakelin D, Rose ME, Hesketh P, Else KJ, Grencis RK. Immunity to coccidiosis: genetic influences on lymphocyte and cytokine responses to infection with Eimeria vermiformis in inbred mice. Parasite Immunol. (1993) 15:11–9. doi: 10.1111/j.1365-3024.1993.tb00567.x
28. Rose ME, Hesketh P, Ogilvie BM. Peripheral blood leucocyte response to coccidial infection: a comparison of the response in rats and chickens and its correlation with resistance to reinfection. Immunology. (1979) 36:71–9.
29. Lillehoj HS, Bacon LD. Increase of intestinal intraepithelial lymphocytes expressing CD8 antigen following challenge infection with Eimeria acervulina. Avian Dis. (1991) 35:294–301. doi: 10.2307/1591179
30. Breed DGJ, Dorrestein J, Vermeulen AN. Immunity to Eimeria tenella in chickens: phenotypical and functional changes in peripheral blood T-cell subsets. Avian Dis. (1996) 40:37–48. doi: 10.2307/1592369
31. Breed DGJ, Dorrestein J, Schetters TPM, Waart LVD, Rijke E, Vermeulen AN. Peripheral blood lymphocytes from Eimeria tenella infected chickens produce gamma-interferon after stimulation in vitro. Parasite Immunol. (1997) 19:127–35. doi: 10.1046/j.1365-3024.1997.d01-191.x
32. Rose ME, Hesketh P, Wakelin D. Immune control of murine coccidiosis: CD4+ and CD8+ T lymphocytes contribute differentially in resistance to primary and secondary infections. Parasitology. (1992) 105:349–354. doi: 10.1017/S0031182000074515
33. Rothwell L, Gramzinski RA, Rose ME, Kaiser P. Avian coccidiosis: changes in intestinal lymphocyte populations associated with the development of immunity to Eimeria maxima. Parasite Immunol. (1995) 17:525–33. doi: 10.1111/j.1365-3024.1995.tb00883.x
34. Rose ME, Wakelin D, Hesketh P. Interferon-gamma-mediated effects upon immunity to coccidial infections in the mouse. Parasite Immunol. (1991) 13:63–74. doi: 10.1111/j.1365-3024.1991.tb00263.x
35. Choi KD, Lillehoj HS, Song KD, Han JY. Molecular and functional characterization of chicken IL-15. Dev Compar Immunol. (1999) 23:165–77. doi: 10.1016/S0145-305X(98)00046-9
36. Lillehoj HS, Choi KD. Recombinant chicken interferon-gamma-mediated inhibition of Eimeria tenella development in vitro and reduction of oocyst production and body weight loss following Eimeria acervulina challenge infection. Avian Dis. (1998) 42:307–14. doi: 10.2307/1592481
37. Lillehoj HS. Lymphocytes involved in cell-mediated immune responses and methods to assess cell-mediated immunity. Poultry Sci. (1991) 70:1154–64. doi: 10.3382/ps.0701154
38. Lillehoj HS. Analysis of Eimeria acervulina-induced changes in the intestinal T lymphocyte subpopulations in two chicken strains showing different levels of susceptibility to coccidiosis. Res Vet Sci. (1994) 56:1–7. doi: 10.1016/0034-5288(94)90188-0
39. Hong YH, Lillehoj HS, Lillehoj EP, Lee SH. Changes in immune-related gene expression and intestinal lymphocyte subpopulations following Eimeria maxima infection of chickens. Vet Immunol Immunopathol. (2006) 114:259–72. doi: 10.1016/j.vetimm.2006.08.006
40. Hong YH, Lillehoj HS, Lee SH, Dalloul RA, Lillehoj EP. Analysis of chicken cytokine and chemokine gene expression following Eimeria acervulina and Eimeria tenella infections. Vet Immunol Immunopathol. (2006) 114:209–23. doi: 10.1016/j.vetimm.2006.07.007
41. Avery S, Rothwell L, Degen WDJ, Schijns VEJC, Young J, Kaufman J, et al. Characterization of the first nonmammalian T2 cytokine gene cluster: the cluster contains functional single-copy genes for IL-3, IL-4, IL-13, and GM-CSF, a gene for IL-5 that appears to be a pseudogene, and a gene encoding another cytokinelike transcript. J Interf Cytokine Res. (2004) 24:600–10. doi: 10.1089/jir.2004.24.600
42. Min W, Lillehoj HS. Isolation and characterization of chicken interleukin-17 cDNA. J Interf Cytokine Res. (2002) 22:1123–8. doi: 10.1089/10799900260442548
43. Min W, Lillehoj HS. Identification and characterization of chicken interleukin-16 cDNA. Dev Compar Immunol. (2004) 28:153–62. doi: 10.1016/S0145-305X(03)00133-2
44. Min W, Lillehoj HS, Fetterer RH. Identification of an alternatively spliced isoform of the common cytokine receptor γ chain in chickens. Biochem Biophys Res Commun. (2002) 299:321–7. doi: 10.1016/S0006-291X(02)02636-0
45. Pan H, Halper J. Cloning, expression, and characterization of chicken transforming growth factor β4. Biochem Biophys Res Commun. (2003) 303:24–30. doi: 10.1016/S0006-291X(03)00300-0
46. Rothwell L, Young JR, Zoorob R, Whittaker CA, Hesketh P, Archer A, et al. Cloning and characterization of chicken IL-10 and its role in the immune response to Eimeria maxima. J Immunol. (2004) 173:2675–82. doi: 10.4049/jimmunol.173.4.2675
47. Schneider K, Puehler F, Baeuerle D, Elvers S, Staeheli P, Kaspers B, et al. cDNA cloning of biologically active chicken interleukin-18. J Interf Cytokine Res. (2000) 20:879–83. doi: 10.1089/10799900050163244
48. Schneider K, Klaas R, Kaspers B, Staeheli P. Chicken interleukin-6: cDNA structure and biological properties. Eur J Biochem. (2001) 268:4200–6. doi: 10.1046/j.1432-1327.2001.02334.x
49. Song KD, Lillehoj HS, Choi KD, Zarlenga D, Han JY. Expression and functional characterization of recombinant chicken interferon-gamma. Vet Immunol Immunopathol. (1997) 58:321–33. doi: 10.1016/S0165-2427(97)00034-2
50. Yoo J, Jang SI, Kim S, Cho JH, Lee HJ, Rhee MH, et al. Molecular characterization of duck interleukin-17. Vet Immunol Immunopathol. (2009) 132:318–22. doi: 10.1016/j.vetimm.2009.06.003
51. Zhang S, Lillehoj HS, Ruff MD. Chicken tumor necrosis-like factor. I. In vitro production by macrophages stimulated with Eimeria tenella or bacterial lipopolysaccharide. Poultry Sci. (1995) 74:1304–10. doi: 10.3382/ps.0741304
52. Degen WGJ, van Daal N, van Zuilekom HI, Burnside J, Schijns VEJC. Identification and molecular cloning of functional chicken IL-12. J Immunol. (2004) 172:4371–80. doi: 10.4049/jimmunol.172.7.4371
53. Hong YH, Lillehoj HS, Hyen Lee S, Woon Park D, Lillehoj EP. Molecular cloning and characterization of chicken lipopolysaccharide-induced TNF-A factor. (LITAF). Dev Compar Immunol. (2006) 30:919–29. doi: 10.1016/j.dci.2005.12.007
54. Jakowlew SB, Dillard PJ, Winokur TS, Flanders KC, Sporn MB, Roberts AB. Expression of transforming growth factor-βs 1-4 in chicken embryo chondrocytes and myocytes. Dev Biol. (1991) 143:135–48. doi: 10.1016/0012-1606(91)90061-7
55. Jeong J, Lee C, Yoo J, Koh PO, Kim YH, Chang HH, et al. Molecular identification of duck and quail common cytokine receptor γ chain genes. Vet Immunol Immunopathol. (2011) 140:159–65. doi: 10.1016/j.vetimm.2010.11.023
56. Jeong J, Kim WH, Yoo J, Lee C, Kim S, Cho J-H, et al. Identification and comparative expression analysis of interleukin 2/15 receptor β chain in chickens infected with E. tenella. PLoS ONE. (2012) 7:e37704. doi: 10.1371/journal.pone.0037704
57. Koskela K, Kohonen P, Salminen H, Uchida T, Buerstedde JM, Lassila O. Identification of a novel cytokine-like transcript differentially expressed in avian γδ T cells. Immunogenetics. (2004) 55:845–54. doi: 10.1007/s00251-004-0643-8
58. Kaiser P, Poh TY, Rothwell L, Avery S, Balu S, Pathania US, et al. A genomic analysis of chicken cytokines and chemokines. J Interf Cytokine Res. (2005) 25:467–84. doi: 10.1089/jir.2005.25.467
59. Lillehoj HS, Min W, Dalloul RA. Recent progress on the cytokine regulation of intestinal immune responses to Eimeria. Poultry Sci. (2004) 83:611–23. doi: 10.1093/ps/83.4.611
60. Gadde U, Rathinam T, Erf GF, Chapman HD. Acquisition of immunity to the protozoan parasite Eimeria adenoeides in turkey poults and cellular responses to infection. Poultry Sci. (2013) 92:3149–57. doi: 10.3382/ps.2013-03406
61. Zhou Z, Wang Z, Cao L, Hu S, Zhang Z, Qin B, et al. Upregulation of chicken TLR4, TLR15 and MyD88 in heterophils and monocyte-derived macrophages stimulated with Eimeria tenella in vitro. Exp Parasitol. (2013) 133:417–33. doi: 10.1016/j.exppara.2013.01.002
62. Zhang L, Liu R, Song M, Hu Y, Pan B, Cai J, et al. Eimeria tenella: interleukin 17 contributes to host immunopathology in the gut during experimental infection. Exp Parasitol. (2013) 133:121–30. doi: 10.1016/j.exppara.2012.11.009
63. Liu L, Huang X, Liu J, Li W, Ji Y, Tian D, et al. Identification of common immunodominant antigens of Eimeria tenella, Eimeria acervulina and Eimeria maxima by immunoproteomic analysis. Oncotarget. (2017) 8:34935–45. doi: 10.18632/oncotarget.16824
64. Allen PC. Dietary supplementation with Echinacea and development of immunity to challenge infection with coccidia. Parasitol Res. (2003) 91:74–8. doi: 10.1007/s00436-003-0938-y
65. Lee SH, Lillehoj HS, Jang SI, Lee KW, Bravo D, Lillehoj EP. Effects of dietary supplementation with phytonutrients on vaccine-stimulated immunity against infection with Eimeria tenella. Vet Parasitol. (2011) 181:97–105. doi: 10.1016/j.vetpar.2011.05.003
66. Joyner LP. Immunological variation between two strains of Eimeria acervulina. Parasitology. (1969) 59L725–732. doi: 10.1017/S0031182000031243
67. Norton CC, Hein EH. Eimeria maxima: a comparison of two laboratory strains with a fresh isolate. Parasitology. (1976) 72:345–54. doi: 10.1017/S0031182000049544
68. Jeffers TK. Genetic transfer of anticoccidial drug resistance in Eimeria tenella. J Parasitol. (1974) 60:900–4. doi: 10.2307/3278505
69. Chapman HD, Barta JR, Blake D, Gruber A, Jenkins M, Smith NC, et al. A selective review of advances in coccidiosis research. Adv Parasitol. (2013) 83:93–171. doi: 10.1016/B978-0-12-407705-8.00002-1
70. Blake DP, Oakes R, Smith AL. A genetic linkage map for the apicomplexan protozoan parasite Eimeria maxima and comparison with Eimeria tenella. Int J Parasitol. (2011) 41:263–70. doi: 10.1016/j.ijpara.2010.09.004
71. Blake DP, Billington KJ, Copestake SL, Oakes RD, Quail MA, Wan KL, et al. Genetic mapping identifies novel highly protective antigens for an apicomplexan parasite. PLoS Pathog. (2011) 7:e1001279. doi: 10.1371/journal.ppat.1001279
72. Lee SH, Dong X, Lillehoj HS, Lamont SJ, Suo X, Kim DK, et al. Comparing the immune responses of two genetically B-complex disparate Fayoumi chicken lines to Eimeria tenella. British Poultry Sci. (2016) 57:165–71. doi: 10.1080/00071668.2016.1141172
73. Kogut M, Lange C. Interferon-g-mediated inhibition of the development of Eimeria tenella in cultured cells. J Parasitol. (1989) 75:313–7. doi: 10.2307/3282782
74. Rose ME, Wakelin D, Hesketh P. Gamma imerferon controls Eimeria vermiformis primary infection in BALB/c mice. Infect Immun. (1989) 57:1599–603.
75. Smith AL, Hayday AC. Genetic analysis of the essential components of the immunoprotective response to infection with Eimeria vermiformis. Int J Parasitol. (1998) 28:1061–9. doi: 10.1016/S0020-7519(98)00081-2
76. Sekellick MJ, Ferrandino AF, Hopkins DA, Marcus PI. Chicken interferon gene: cloning, expression, and analysis. J Interf Res. (1994) 14:71–9. doi: 10.1089/jir.1994.14.71
77. Digby M, Lowenthal J. Cloning and expression of the chicken interferon-gamma gene. J Interf Cytokine Res. (1995) 15:939–45. doi: 10.1089/jir.1995.15.939
78. Kogut MH, Lange C. Recombinant interferon-γ inhibits cell invasion by Eimeria tenella. J Interf Res. (1989) 9:67–77. doi: 10.1089/jir.1989.9.67
79. Prowse SJ, Pallister J. Interferon release as a measure of the T-cell response to coccidial antigens in chickens. Avian Pathol. (1989) 18:619–30. doi: 10.1080/03079458908418637
80. Byrnes S, Emerson K, Kogut M. Dynamics of cytokine production during coccidial infections in chickens: colony-stimulating factors and interferon. FEMS Immunol Med Microbiol. (1993) 6:45–52. doi: 10.1111/j.1574-695X.1993.tb00302.x
81. Breed DGJ, Schetters TPM, Verhoeven NAP, Vermeulen AN. Characterization of phenotype related responsiveness of peripheral blood lymphocytes from Eimeria tenella infected chickens. Parasite Immunol. (1997) 19:536–69. doi: 10.1046/j.1365-3024.1997.d01-174.x
82. Rothwell L, Muir W, Kaiser P. Interferon-γ is expressed in both gut and spleen during Eimeria tenella infection. Avian Pathol. (2000) 29:333–42. doi: 10.1080/03079450050118467
83. Lowenthal JW, York JJ, O'Neil TE, Rhodes S, Prowse SJ, Strom DG, et al. In vivo effects of chicken interferon-γ during infection with Eimeria. J Interf Cytokine Res. (1997) 17:551–8. doi: 10.1089/jir.1997.17.551
84. Dimier IH, Quéré P, Naciri M, Bout DT. Inhibition of Eimeria tenella development in vitro mediated by chicken macrophages and fibroblasts treated with chicken cell supernatants with IFN-gamma activity. Avian Dis. (1998) 42:239–47. doi: 10.2307/1592473
85. Takehara K, Kobayashi K, Ruttanapumma R, Kamikawa M, Nagata T, Yokomizo Y, et al. Adjuvant effect of chicken interferon-gamma for inactivated Salmonella Enteritidis antigen. J Vet Med Sci. (2003) 65:1337–41. doi: 10.1292/jvms.65.1337
86. Lillehoj HS, Ding X, Quiroz MA, Bevensee E, Lillehoj EP. Resistance to intestinal coccidiosis following DNA immunization with the cloned 3-1E Eimeria gene plus IL-2, IL-15, and IFN-gamma. Avian Dis. (2005) 49:112–7. doi: 10.1637/7249-073004R
87. Min W, Lillehoj HS, Burnside J, Weining KC, Staeheli P, Zhu JJ. Adjuvant effects of IL-1β, IL-2, IL-8, IL-15, IFN-α, IFN-γ TGF-β4 and lymphotactin on DNA vaccination against Eimeria acervulina. Vaccine. (2001) 20:267–74. doi: 10.1016/S0264-410X(01)00270-5
88. Shah MAA, Song X, Xu L, Yan R, Song H, Ruirui Z, et al. The DNA-induced protective immunity with chicken interferon gamma against poultry coccidiosis. Parasitol Res. (2010) 107:747–50. doi: 10.1007/s00436-010-1940-9
89. Song X, Huang X, Yan R, Xu L, Li X. Efficacy of chimeric DNA vaccines encoding Eimeria tenella 5401 and chicken IFN-γ or IL-2 against coccidiosis in chickens. Exp Parasitol. (2015) 156:19–25. doi: 10.1016/j.exppara.2015.05.003
90. Martinez GJ, Nurieva RI, Yang XO, Dong C. Regulation and function of proinflammatory TH17 cells. Annals N Y Acad Sci. (2008) 1143:188–211. doi: 10.1196/annals.1443.021
91. Pappu R, Rutz S, Ouyang W. Regulation of epithelial immunity by IL-17 family cytokines. Trends Immunol. (2012) 33:343–9. doi: 10.1016/j.it.2012.02.008
92. Kim WH, Jeong J, Park AR, Yim D, Kim Y-H, Kim KD, et al. Chicken IL-17F: Identification and comparative expression analysis in Eimeria-infected chickens. Dev Compar Immunol. (2012) 38:401–9. doi: 10.1016/j.dci.2012.08.002
93. Bacellar O, Faria D, Nascimento M, Cardoso TM, Gollob KJ, Dutra WO, et al. Interleukin 17 production among patients with american cutaneous leishmaniasis. J Infect Dis. (2009) 200:75–8. doi: 10.1086/599380
94. Guiton R, Vasseur V, Charron S, Arias MT, Van Langendonck N, Buzoni-Gatel D, et al. Interleukin 17 receptor signaling is deleterious during Toxoplasma gondii infection in susceptible BL6 mice. J Infect Dis. (2010) 202:427–35. doi: 10.1086/653738
95. Stange J, Hepworth MR, Rausch S, Zajic L, Kühl AA, Uyttenhove C, et al. IL-22 mediates host defense against an intestinal intracellular parasite in the absence of IFN-γ at the cost of Th17-driven immunopathology. J Immunol. (2012) 188:2410–8. doi: 10.4049/jimmunol.1102062
96. Miyazaki Y, Hamano S, Wang S, Shimanoe Y, Iwakura Y, Yoshida H. IL-17 is necessary for host protection against acute-phase Trypanosoma cruzi infection. J Immunol. (2010) 185:1150–7. doi: 10.4049/jimmunol.0900047
97. Kelly MN, Kolls JK, Happel K, Schwartzman JD, Schwarzenberger P, Combe C, et al. Interleukin-17/interleukin-17 receptor-mediated signaling is important for generation of an optimal polymorphonuclear response against Toxoplasma gondii infection. Infect Immun. (2005) 73:617–21. doi: 10.1128/IAI.73.1.617-621.2005
98. Del Cacho E, Gallego M, Lillehoj HS, Quílez J, Lillehoj EP, Ramo A, et al. IL-17A regulates Eimeria tenella schizont maturation and migration in avian coccidiosis. Vet Res. (2014) 45:1–9. doi: 10.1186/1297-9716-45-25
99. Tiemessen MM, Jagger AL, Evans HG, van Herwijnen MJC, John S, Taams LS. CD4+CD25+Foxp3+ regulatory T cells induce alternative activation of human monocytes/macrophages. Proc Natl Acad Sci USA. (2007) 104:19446–51. doi: 10.1073/pnas.0706832104
100. Shack LA, Buza JJ, Burgess SC. The neoplastically transformed. (CD30hi) Marek's disease lymphoma cell phenotype most closely resembles T-regulatory cells. Cancer Immunol Immunother. (2008) 57:1253–62. doi: 10.1007/s00262-008-0460-2
101. Denyer MP, Pinheiro DY, Garden OA, Shepherd AJ. Missed, not missing: phylogenomic evidence for the existence of avian foxP3. PLoS ONE. (2016) 11:e0150988. doi: 10.1371/journal.pone.0150988
102. Shanmugasundaram R, Selvaraj RK. Regulatory T cell properties of chicken CD4+CD25+ cells. J Immunol. (2011) 186:1997–2002. doi: 10.4049/jimmunol.1002040
103. Selvaraj RK. Avian CD4+CD25+ regulatory T cells: properties and therapeutic applications. Dev Compar Immunol. (2013) 41:397–402. doi: 10.1016/j.dci.2013.04.018
104. Sand JM, Arendt MK, Repasy A, Deniz G, Cook ME. Oral antibody to interleukin-10 reduces growth rate depression due to Eimeria spp. infection in broiler chickens. Poultry Sci. (2016) 95:439–46. doi: 10.3382/ps/pev352
105. Arendt MK, Sand JM, Marcone TM, Cook ME. Interleukin-10 neutralizing antibody for detection of intestinal luminal levels and as a dietary additive in Eimeria challenged broiler chicks. Poultry Sci. (2016) 95:430–8. doi: 10.3382/ps/pev365
106. Morris A, Shanmugasundaram R, McDonald J, Selvaraj RK. Effect of in vitro and in vivo 25-hydroxyvitamin D treatment on macrophages, T cells, and layer chickens during a coccidia challenge. J Anim Sci. (2015) 93:2894–903. doi: 10.2527/jas.2014-8866
107. Kim WH, Lillehoj HS, Min W. Indole treatment alleviates intestinal tissue damage induced by chicken coccidiosis through activation of the aryl hydrocarbon receptor. Front Immunol. (2019) 10:560. doi: 10.3389/fimmu.2019.00560
Keywords: chicken, coccidiosis, T cells, avian immunology, host immunity
Citation: Kim WH, Chaudhari AA and Lillehoj HS (2019) Involvement of T Cell Immunity in Avian Coccidiosis. Front. Immunol. 10:2732. doi: 10.3389/fimmu.2019.02732
Received: 31 July 2018; Accepted: 07 November 2019;
Published: 22 November 2019.
Edited by:
Armin Saalmueller, University of Veterinary Medicine Vienna, AustriaReviewed by:
Renato Augusto DaMatta, Universidade Estadual Do Norte Fluminense Darcy Ribeiro, BrazilCopyright © 2019 Kim, Chaudhari and Lillehoj. This is an open-access article distributed under the terms of the Creative Commons Attribution License (CC BY). The use, distribution or reproduction in other forums is permitted, provided the original author(s) and the copyright owner(s) are credited and that the original publication in this journal is cited, in accordance with accepted academic practice. No use, distribution or reproduction is permitted which does not comply with these terms.
*Correspondence: Hyun S. Lillehoj, SHl1bi5MaWxsZWhvakBhcnMudXNkYS5nb3Y=
†These authors have contributed equally to this work
Disclaimer: All claims expressed in this article are solely those of the authors and do not necessarily represent those of their affiliated organizations, or those of the publisher, the editors and the reviewers. Any product that may be evaluated in this article or claim that may be made by its manufacturer is not guaranteed or endorsed by the publisher.
Research integrity at Frontiers
Learn more about the work of our research integrity team to safeguard the quality of each article we publish.