- Institute of Animal Husbandry and Veterinary Medicine, Beijing Academy of Agriculture and Forestry Sciences, Beijing, China
Host defense peptides (HDPs) have antimicrobial and immunoregulatory activities and are involved in epithelial innate immune defense. Dietary modulation of endogenous HDP synthesis is an effective way to boost the host innate immune system. This study aimed to investigate the role of the probiotic Lactobacillus plantarum strain ZLP001 in porcine HDP induction and the underlying mechanism. To this end, we evaluated the stimulatory effect of L. plantarum ZLP001 on HDP expression in piglet intestinal tissue in vivo and porcine IPEC-J2 cells and 3D4/31 cells in vitro, and we examined the underlying intracellular signaling pathway in IPEC-J2 cells. L. plantarum ZLP001 treatment increased the mRNA expression of jejunal and ileal HDPs in weaned piglets. In IPEC-J2 and 3D4/31 cells, L. plantarum ZLP001 stimulated HDP expression, but different HDP induction patterns were observed, with the various HDPs exhibiting different relative mRNA levels in each cell line. L. plantarum ZLP001 induced porcine HDP expression through toll-like receptor (TLR)2 recognition as evidenced by the fact that HDP expression was suppressed in TLR2-knockdown IPEC-J2 cells. Furthermore, we found that L. plantarum ZLP001 activated the extracellular signal-regulated kinase (ERK)1/2 and c-jun N-terminal kinase (JNK) signaling pathways, as indicated by enhanced phosphorylation of both ERK1/2 and JNK and the fact that HDP expression was suppressed upon inhibition of ERK1/2 and JNK. Furthermore, L. plantarum ZLP001 activated c-fos and c-jun transcription factor phosphorylation and activity. We conclude that L. plantarum ZLP001 induces porcine HDP expression in vivo and in vitro, and the induction seems to be regulated via TLR2 as well as the ERK1/2/JNK and c-jun/c-fos signaling pathways. Modulation of endogenous HDPs mediated by L. plantarum ZLP001 might be a promising approach to improving intestinal health and enhancing diarrhea resistance in weaning piglets.
Introduction
Weaning stress in piglets can suppress immune responses, rendering the piglets vulnerable to infectious diseases, including diarrhea (1). Since mid-last century, antibiotics have been widely used at subtherapeutic levels in piglet production for disease control and growth promotion. The overuse of antibiotics has led to the development of antimicrobial-resistant bacterial strains, which threaten animal as well as human health. Because of this public safety concern, antibiotic additives will be phased out in China, as in many other countries. Therefore, novel methods to inhibit pathogen overgrowth in piglets and improve their host defense responses are urgently needed (2, 3).
Host defense peptides (HDPs), also known as antimicrobial peptides, are produced in various epithelial cells and immune cells lining the gut and play essential roles in mammalian innate immunity. HDPs have two main families (i.e., defensins and cathelicidins) in vertebrate animals. They have been widely studied for their broad-spectrum antimicrobial effects against bacteria, viruses, fungi, and protozoa (4). In addition to the well-described antimicrobial properties, HDPs display a wide range of immunomodulatory activities, including modulation of pro-, and anti-inflammatory responses (4, 5). Stimulating the innate immune system and elevating endogenous HPD synthesis through dietary supplementation has been suggested as a possible safe method to enhance bacterial balance and improve intestinal health (6, 7). In normal physiological conditions, HDPs are activated in response to the oscillating energy status of cells and tissues and help maintain and enhance defensive barriers without causing damage or inflammation (8). Certain nutritional components, such as VD3, butyrate, and zinc, induce HDP gene expression in animals, and humans without causing inflammatory damage (9–11). Likewise, certain probiotic strains can also induce the release of HDP from epithelial cells (12, 13), which may contribute to explain the beneficial effects of probiotics in infection preventing and gut barrier function stabilizing. Some human research suggests that toll-like receptors (TLRs) might participate in the regulating of probiotic associate HDPs induction effect, and this induction might also be mediated by proinflammatory pathways (14). However, the extent of modulation could be varied in different models and among different strains (15).
In our previous study, we demonstrated that Lactobacillus plantarum ZLP001, which we isolated from healthy piglet ileal mucosa (16), exert beneficial effects on growth performance and antioxidant status in weaning piglets (17), also can upregulate the expression of HDPs in IPEC-J2 cells but did not trigger an inflammatory response (18). However, little is known about the stimulatory effects of L. plantarum ZLP001 on macrophages and in vivo. Additionally, the mechanisms and possible cellular pathways of probiotic-mediated HDP induction have been investigated only in humans, never in pigs. In this study, we examined whether L. plantarum ZLP001 treatment stimulates HDP gene expression in weaning piglets. Additionally, different porcine cell lines, including 3D4/31 lung alveolar macrophages, were used to evaluate the potential regulatory effects of L. plantarum ZLP001 on HDP expression. Furthermore, a potential signaling pathway responsible for L. plantarum ZLP001 modulation of HDP expression was investigated.
Materials and Methods
Animals, Diets, and Experimental Design
Twenty piglets (Large White × Landrace) weaned at 28 days of age (body weight, 8.11 ± 0.35 kg) were used in this study. The experiment was conducted at the experimental animal farm of the Institute of Animal Husbandry and Veterinary Medicine of the Beijing Academy of Agriculture and Forestry Sciences (IAHVM-BAAFS) according to the guidelines established by the Animal Care and Use Committee of IAHVM-BAAFS. The protocol approval number was XM1706. The animals were given humane care throughout the trial. The piglets were raised in a room that was decontaminated prior to the study and maintained at 25–28°C. All piglets had free access to feed and water throughout the 28-days experimental period. They received a complete feed specially formulated according to the National Research Council (19) and the Feeding Standard of Swine (20). The ingredients and chemical composition of the diet are presented in Table 1. The experiment was designed as a contrast experiment including two dietary treatments, and piglets were randomly assigned to one of the treatments (10 piglets per treatment). In the control group, the basal diet was supplemented with a placebo (2 g/kg diet), and in the treatment group, it was supplemented with freeze-dried L. plantarum ZLP001 (109 CFU/g, 2 g/kg diet). The concentration of supplemented L. plantarum ZLP001 in the diet was determined based on the findings of our previous studies (16, 17) and post-extension practice results on the farm. On day 28, the piglets were euthanized. Segments of about 5-cm middle tissues from the duodenum, jejunum, and ileum were collected, rinsed with 0.9% (w/v) saline solution, immediately frozen in liquid nitrogen, and then stored at −80°C until use.
Probiotic Strain and Culture Conditions
L. plantarum ZLP001, originally isolated in our laboratory from ileal mucosa of healthy piglets 4 weeks after weaning, has been identified by the China Center of Industrial Culture Collection (Beijing, China) and is preserved in the China General Microbiological Culture Collection Center (CGMCC No. 7370). After thawing and overnight culture, L. plantarum ZLP001 was inoculated 1:100 in improved de Man, Rogosa, and Sharpe (MRS) liquid medium (10 g peptone, 5 g yeast powder, 20 g glucose, 10 g beef extract, 5 g sodium acetate, 2 g ammonium citrate dibasic, 2 g dipotassium phosphate, 0.58 g magnesium sulfate, 0.19 g manganese sulfate, 1 ml of Tween-80, and water to 1,000 ml; pH 6.5) and incubated at 37°C under anaerobic conditions for 18 h. For probiotic preparation, bacterial cells were collected by centrifugation at 10,000 × g for 10 min, the supernatant was discarded, and the bacterial sludge was mixed with protective medium (100 ml contained 12 g skimmed milk, 10 g dextrin, 4 g lactose, 1.5 g sodium glutamate, and 0.5 g L-cysteine). The mixture was vortexed for 30 min, added to a freeze-drying plate, incubated at −70°C for 2 h, and dried in a freeze-dryer at 1 Pa and −80°C for 24 h. The viable cell count of the freeze-dried powder was 109 CFU/g. The negative placebo sample consisted of protective medium without L. plantarum ZLP001 subjected to the same procedure.
Cell Lines and Culture Conditions
The porcine intestinal epithelial cell line IPEC-J2, which was originally derived from jejunal tissues of neonatal piglets (21), was kindly provided by Dr. Glenn Zhang at Oklahoma State University (Stillwater, OK, USA). IPEC-J2 cells were cultured in Dulbecco's modified Eagle medium/Nutrient Mixture F-12 (DMEM/F12; a 1:1 mixture of DMEM and Ham's F-12; Invitrogen, Carlsbad, CA, USA) supplemented with 10% fetal bovine serum (FBS; Invitrogen), streptomycin (100 μg/ml), penicillin (100 U/ml), and 1% insulin, transferrin, selenium (ScienCell, San Diego, CA, USA) at 37°C under a 5% CO2 and 95% air atmosphere with 90% humidity.
The porcine lung alveolar macrophage cell line 3D4/31 (ATCC CRL-2844) was also kindly provided by Dr. Zhang. The 3D4/31 cells were cultured in RPMI 1640 medium (Invitrogen, Carlsbad, CA, USA) supplemented with 10% FBS, 100 μg/ml streptomycin, 100 U/ml penicillin, and 1 mM sodium pyruvate at 37°C under a 5% CO2 and 95% air atmosphere with 90% humidity.
Treatment of IPEC-J2 and 3D4/31 Cells With L. plantarum ZLP001
To prevent any influence of the FBS and antibiotics on the immune response, bacteria were collected by centrifugation and diluted in fresh DMEM/F12 without FBS and streptomycin/penicillin. The cells were seeded in six-well tissue-culture plates (Costar, Corning Inc., Corning, NY, USA) at 2.5 × 105 cells/well for IPEC-J2 cells and 1 × 106 cells/well for 3D4/31 cells. When the cells reached 70–80% confluence, they were exposed to L. plantarum ZLP001 (108 CFU/ml) in duplicate for 6 h. We used live L. plantarum ZLP001 in the present study, which exhibits the highest induction effect on HDP expression compared with other bacteria processing (heat-killed L. plantarum ZLP001, adhered L. plantarum ZLP001, undirect contact L. plantarum ZLP001, and bacterial free culture supernatant of L. plantarum ZLP001) (data not shown). The optimal bacteria concentration and treatment time were selected from our previous study based on a concentration-dependent (105, 106, 107, 108, and 109 CFU/ml L. plantarum ZLP001) and a time-dependent (3, 6, 9, and 12 h) experiment (18). Non-treated cells served as a control. After incubation, the cells were washed with PBS three times and collected for further assay. The culture media were also collected. All experiments were carried out in triplicate.
The involvement of different mitogen-activated protein kinase (MAPK) signaling pathways in L. plantarum ZLP001-induced expression of HDPs at both the transcriptional and translational levels was evaluated by using specific pharmacological inhibitors. The specific extracellular signal-regulated kinase (ERK)1/2 and c-jun N-terminal kinase (JNK) inhibitors U0126 and SB600125 were purchased from Cell Signaling Technology (Danvers, MA, USA) and were dissolved in dimethyl sulfoxide. IPEC-J2 cells were pretreated with the specific inhibitors for 1 h prior to stimulation with L. plantarum ZLP001. After the indicated incubation periods, cell samples were collected to examine mRNA expression by RT-qPCR and cell culture media were collected to analyze protein secretion by ELISA. Appropriate inhibitor concentration and incubation time were evaluated by Cell Counting Kit-8 (Dojindo Molecular Technologies, Kumamoto, Japan). All experiments were carried out in triplicate.
Quantitative Reverse Transcription (RT-q)PCR
HDP mRNA levels were determined by RT-qPCR. Cells and tissues were lysed directly in RNAzol (Molecular Research Center, Cincinnati, OH, USA), and total RNA was extracted according to the manufacturer's instructions. The RNA concentration was measured using a NanoDrop spectrophotometer (Thermo Fisher Scientific, Waltham, MA, USA), and the quality was ascertained by the A260:A280 and A260:A230 ratios. One microgram of RNA was reverse-transcribed to cDNA using an iScript™ cDNA Synthesis Kit (Bio-Rad, Hercules, CA, USA) according to the manufacturer's instructions. qPCR was conducted using iTaq™ Universal SYBR® Green Supermix (Bio-Rad) in a QuantStudio 3 Real-Time PCR System (Thermo Fisher Scientific). The thermal cycles were as follows: 95°C for 10 min followed by 40 cycles of 95°C for 30 s, 60°C for 30 s, and 72°C for 20 s. Porcine-specific primers were designed, and the sequences are listed in Supplementary Table S1. Target gene expression levels were normalized to that of glyceraldehyde-3-phosphate dehydrogenase (GAPDH), and relative gene expression was calculated using the ΔΔCt method.
Western Blotting
Proteins were extracted from IPEC-J2 cells using lysis buffer consisting of 150 mM NaCl, 1% Triton X-100, 0.5% sodium deoxycholate, 0.1% sodium dodecyl sulfate, 50 mM Tris-HCl at pH 7.4, and a protease inhibitor cocktail (Applygene, Beijing, China). Cells were mixed with 100 μl of ice-cold lysis buffer and incubated on ice for 30 min. The mixtures were centrifuged at 12,000 × g at 4°C for 5 min, and protein concentrations in the supernatants were determined using a BCA Protein Assay Kit (Pierce, Madison, WI, USA). Equal amounts of protein (30 μg/lane) were resolved by 10% sodium dodecyl sulfate polyacrylamide gel electrophoresis and electrophoretically transferred to polyvinylidene difluoride membranes (EMD Millipore, Bedford, MA, USA) at 90 V at 4°C for 60–100 min. After being blocked with 5% skim milk, the immunoblots were incubated with primary antibodies at 4°C overnight, then with the indicated horseradish peroxidase-conjugated secondary antibodies, and finally reacted with Western Blot Luminance Reagent (Santa Cruz Biotechnology, Dallas, TX, USA). The antibodies and dilutions used are shown in Supplementary Table S2. Immunoreactive proteins were imaged with a ChemiDoc XRS system (Bio-Rad, Hercules, CA, USA). Band densities were quantified using ImageJ (National Institutes of Health, Bethesda, MD, USA) and normalized to that of the loading control.
Enzyme-Linked Immunosorbent Assay (ELISA)
Five hundred microliters of cell culture media were centrifuged at 4,000 × g at room temperature for 10 min and then passed through 0.25-μm filters (Corning Inc., Corning, NY, USA). The concentration of porcine β-defensin 2 (pBD2) was determined using a commercial porcine-specific ELISA kit (CloudClone Corp. USCN Life Science, Inc., Wuhan, China) according to the manufacturer's instructions. We also tried to detect the concentration of pBD2 in the intestinal content and mucus, but we failed to get the valid data.
TLR2 Knockdown in IPEC-J2 Cells
TLR2 was knocked down in IPEC-J2 cells according to the method described by Murofushi et al. (22). IPEC-J2 cells were seeded in six-well tissue culture plates at 2.5 × 105 cells/well. When the cells reached 70–80% confluence, they were transfected with 300 pmol of TLR short interfering RNAs (siRNAs) using Lipofectamine RNAiMAX (Invitrogen, Carlsbad, CA, USA). The primers used for TLR2 gene knockdown were as follows: CAGAUGCCUCCUUUCUACCCAUGUU (sense) and AACAUGGGUAGAAAGGAGGCAUCUG (antisense). Inhibition of TLR2 expression in knockdown IPEC-J2 cells was detected by qPCR with TLR2-specific primers (sense: TTCAGGCCAAGGATTTCCAG; antisense: TCACTGTGCTGGTTCATTG).
Transcription Factor Activity Assay
DNA binding by c-jun and c-fos was detected using the TransAM transcription factor ELISA system (Active Motif, Carlsbad, CA, USA) according to the manufacturer's instructions. After stimulation with L. plantarum ZLP001, nuclear proteins were isolated from IPEC-J2 cells using a nuclear protein extraction kit (Active Motif, Carlsbad, CA, USA) according to the manufacturer's instructions. Ten micrograms of IPEC-J2 cell nuclear extract was incubated in oligonucleotide-coated wells at room temperature for 20 min. The plates were washed with PBS three times, and 100 μl of the corresponding transcription factor antibody (1:1,000) was added to each well and incubated for 1 h. After three washes with PBS, 100 μl of horseradish peroxidase-conjugated antibody (1:1,000) was added for 1 h. The plates were then incubated with developing solution for an additional 10 min, and the absorbance at 450 nm was measured using a spectrophotometer.
Statistical Analysis
Statistical analyses were performed using Prism version 6 (GraphPad Software, Inc., San Diego, CA, USA). Statistical significance between different treatments was determined using unpaired Student's two-tailed t-test. The results are expressed as means ± standard error of the mean (SEM). The minimal level of confidence at which experimental results were considered significant was set at P < 0.05.
Results
L. plantarum ZLP001 Stimulates Intestinal HDP Expression in vivo
L. plantarum ZLP001 was administered to weaned piglets at 2 g/kg diet for 28 days to determine whether it affects HDP expression in intestinal tissues. mRNA expression of six porcine HDPs, including pBD2, pBD3, PG1–5 (cysteine-rich protegrins 1–5), pEP2C (epididymis protein 2 splicing variant C), pBD114, and pBD129, was evaluated by RT-qPCR (Figure 1). Except for PG1-5, HDP mRNA expression levels in the duodenum did not differ (P > 0.05) between the L. plantarum ZLP001 treatment and control groups. For most HDPs, intragroup differences in mRNA expression levels in the duodenum were greater than intergroup differences. In the jejunum, dietary supplementation with L. plantarum ZLP001 resulted in significantly enhanced expression of the six HDPs (P < 0.05 vs. control group). In ileal tissues, L. plantarum ZLP001 supplementation resulted in significantly enhanced mRNA expression of all HDPs evaluated except pBD129. The evaluated genes responded differentially to L. plantarum ZLP001. in different intestinal tissue types. In the jejunum, pBD2 showed the strongest response, with an ~6-fold increase, whereas the other HDPs showed intermediate 3–4-fold induction. In the ileum, PG1-5 exhibited the strongest response, with an ~10-fold increase, and pBD2, pBD114, and pEP2C exhibited higher fold increases than did pBD3, which were only slightly induced (<3-fold).
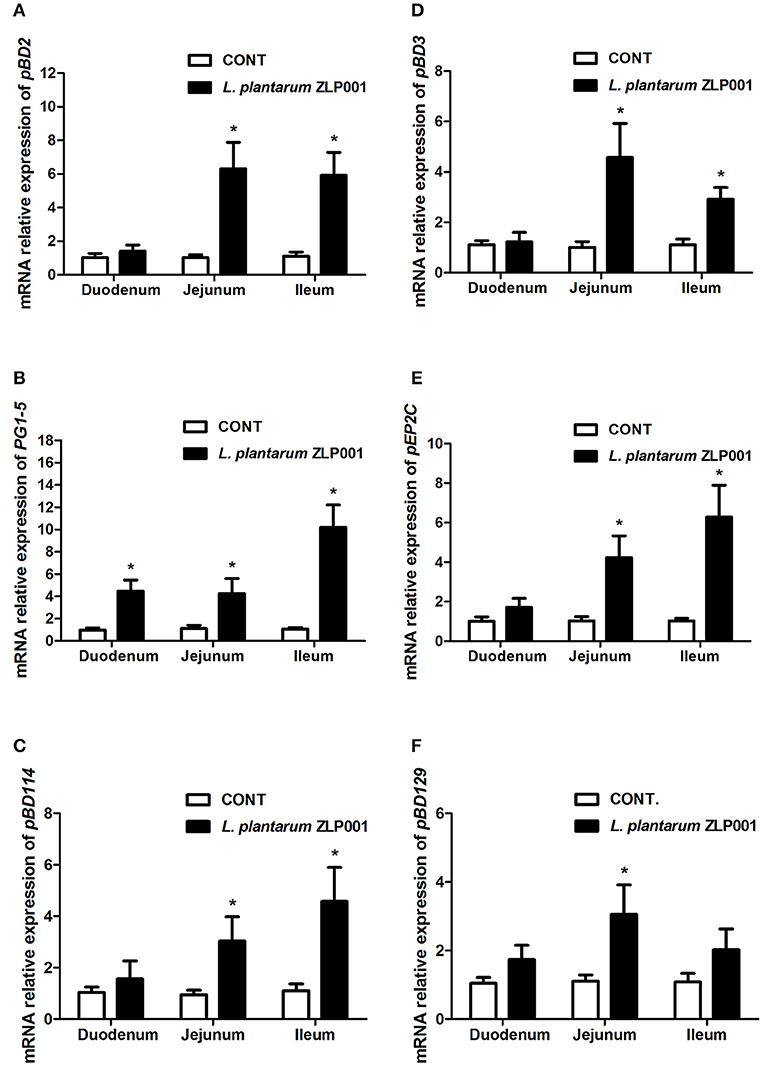
Figure 1. Relative mRNA expression of porcine host defense peptides (HDPs) in the duodena, jejuna, and ilea of piglets supplemented with L. plantarum ZLP001 for 4 weeks as determined by RT-qPCR. Average Ct values of each gene in duodenum, jejunum, and ileum were (A) pBD2 31.0/30.6, 30.9/28.6, 30.4/27.9; (B) PG1-5 30.8/28.8, 29.6/28.0, 31.2/27.5; (C) pBD114 31.1/31.0, 30.8/29.8, 31.9/30.3; (D) pBD3 30.5/30.4, 29.8/27.8, 29.8/29.4; (E) pEP2C 30.7/29.9, 31.5/29.4, 30.9/28.7; and (F) pBD129 30.2/30.3, 29.9/28.4, 31.0/29.9, respectively. mRNA expression was standardized to that of GAPDH. Relative fold changes vs. levels in non-stimulated controls were calculated by the ΔΔCt method. Data are the mean ± SEM of three independent experiments. *P < 0.05 vs. non-treated control group. White and black bars represent control and L. plantarum ZLP001 treatment, respectively.
L. plantarum ZLP001 Induces HDP Expression in IPEC-J2 and 3D4/31 Cells in vitro
First, we analyzed the mRNA expression of the above six porcine HDPs in IPEC-J2 intestinal epithelial cells exposed to L. plantarum ZLP001 by RT-qPCR. After 6-h exposure to L. plantarum ZLP001, mRNA expression of all six HDPs was significantly increased (Figure 2A). However, the magnitude of induction obviously differed among the six HDPs; pEP2C showed an ~4-fold increase; pBD2, PG1-5, and pBD129 exhibited ~3-fold induction; and pBD3 and pBD114 showed lower induction.
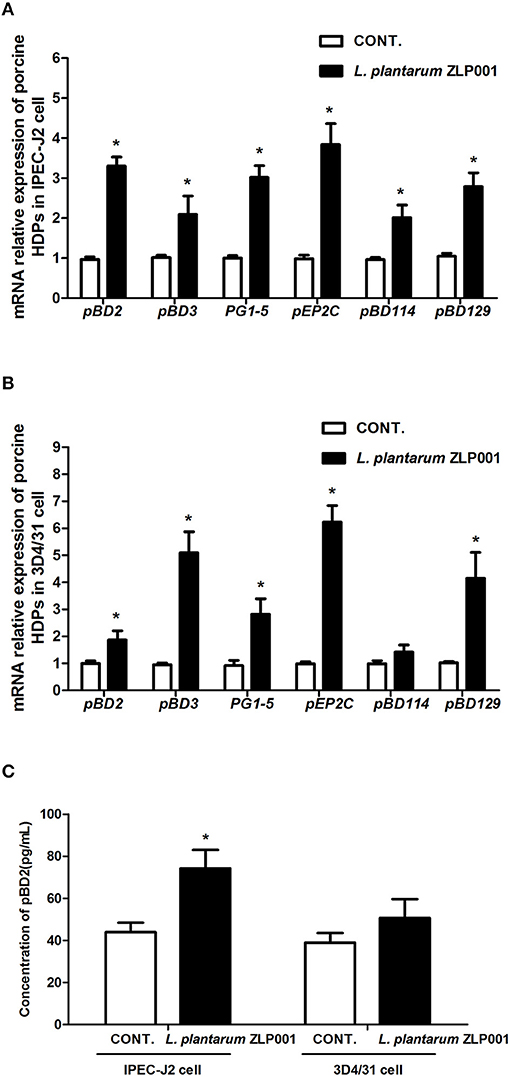
Figure 2. Relative mRNA expression and protein secretion of host defense peptides (HDPs) in different porcine cell lines after L. plantarum ZLP001 treatment. mRNA expression in (A) intestinal IPEC-J2 epithelial cells and (B) 3D4/31 lung alveolar macrophages as determined by RT-qPCR. Average Ct values of each gene in IPEC-J2 cell and 3D4/31 cell were (A) pBD2 31.7/29.6, pBD3 29.1/28.0, PG1-5 31.9/30.1, pEP2C 30.5/28.6, pBD114 32.0/31.0, pBD129 31.3/29.8; and (B) pBD2 30.3/29.5, pBD3 30.6/27.9, PG1-5 31.3/29.5, pEP2C 29.7/26.9, pBD114 31.2/30.7, pBD129 30.7/28.7. mRNA expression was standardized to that of GAPDH. Relative fold changes vs. levels in non-stimulated controls were calculated by the ΔΔCt method. (C) pBD2 secretion in both cell lines after L. plantarum ZLP001 treatment as determined by ELISA. Data are the mean ± SEM of three independent experiments. *P < 0.05 vs. non-treated control group. White and black bars represent control and L. plantarum ZLP001 treatment, respectively.
Next, we evaluated the effect of L. plantarum ZLP001 on porcine 3D4/31 lung alveolar macrophages (Figure 2B). mRNA levels of pBD2, pBD3, PG1-5, pEP2C, and pBD129 were significantly increased after incubation with L. plantarum ZLP001. The induction patterns of several genes in IPEC-J2 and 3D4/31 cells were in sharp contrast. pBD3 was substantially more strongly induced in 3D4/31 cells, with a nearly five-fold increase following L. plantarum ZLP001 treatment, whereas pBD2 exhibited a <2-fold increase. PG1-5 induction was similar in both cell lines. pEP2C expression exhibited a six-fold increase in 3D4/31 cells following L. plantarum ZLP001 treatment. pBD114 was not significantly induced in 3D4/31 cells.
To evaluate the effect of L. plantarum ZLP001 on HDP secretion, we examined the concentration of pBD2 in cell culture media after L. plantarum ZLP001 treatment (commercial ELISA kits for other HDPs were unavailable). As shown in Figure 2C, L. plantarum ZLP001 significantly induced pBD2 production in IPEC-J2 but not 3D4/31 cells.
TLR2 Is Required for L. plantarum ZLP001-Induced HDP Upregulation in IPEC-J2 Cells
TLR2 is involved in the cellular response to probiotic bacteria (23). To examine whether TLR2 is involved in the upregulation of HDP expression by L. plantarum ZLP001 treatment, IPEC-J2 cells were transfected with siRNA to specifically knock down TLR2. Effective knockdown of TLR2 was confirmed by RT-qPCR and Western blot analysis (Figure 3A). pBD2 mRNA expression and pBD2 protein secretion were significantly decreased in TLR2-knockdown IPEC-J2 cells when compared with negative siRNA control cells after L. plantarum ZLP001 treatment, as indicated by RT-qPCR and Western blot analysis (Figure 3B). mRNA levels of the other HDPs were also suppressed in TLR2-knockdown cells treated with L. plantarum ZLP001 (Figure 3C). These results indicated that TLR2 is required for L. plantarum ZLP001-induced HDP upregulation in IPEC-J2 cells.
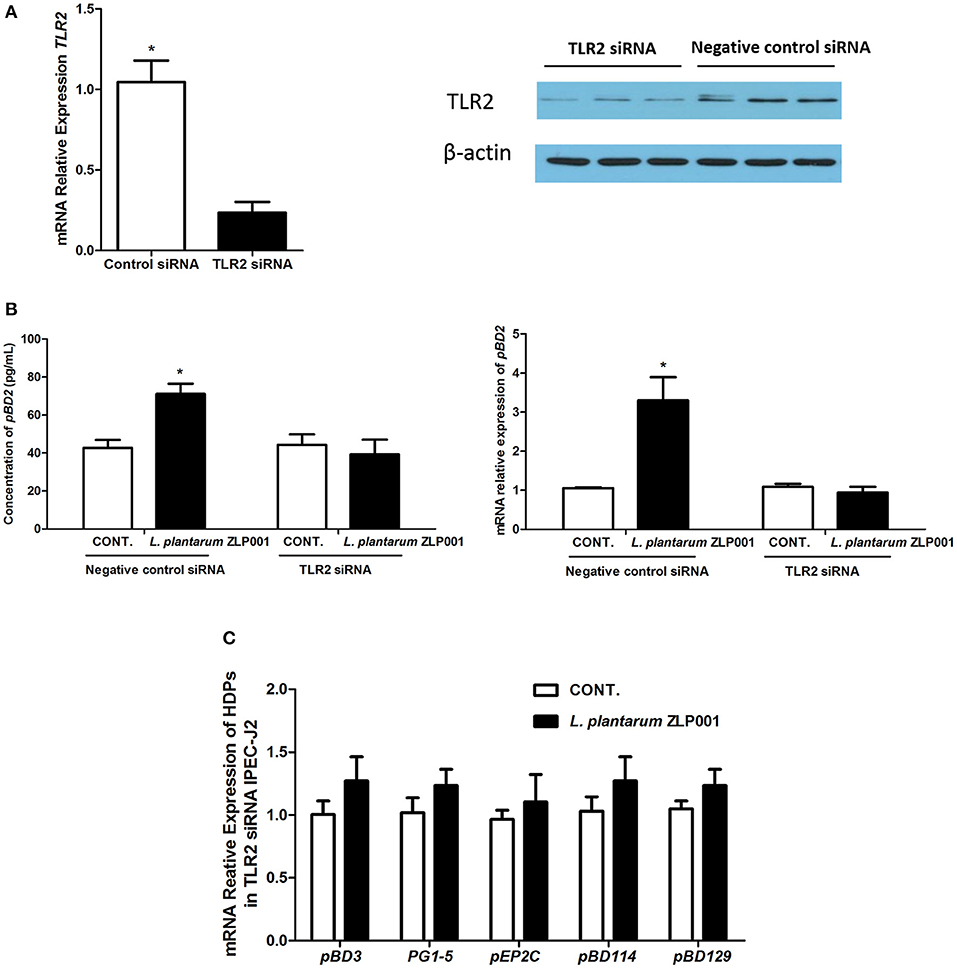
Figure 3. Toll-like receptor (TLR)2 is required for L. plantarum ZLP001-induced porcine host defense peptide (HDP) upregulation in IPEC-J2 cells. (A) TLR2 gene and protein expression in TLR2 siRNA-transfected IPEC-J2 cells was determined using RT-qPCR and Western blot analyses, respectively. Average Ct values of TLR2 gene were 30.3/32.7. (B) L. plantarum ZLP001 stimulates porcine pBD2 expression and secretion through TLR2 in IPEC-J2 cells. pBD2 expression and concentration were measured by RT-qPCR and ELISA, respectively. Average Ct values of pBD2 gene were 31.0/29.5 and 32.4/32.7 for negative control siRNA and TLR2 siRNA, respectively. Data are the mean ± SEM of three independent experiments. *P < 0.05 vs. non-treated control group. (C) TLR2 silencing suppresses porcine HDP expression induced by L. plantarum ZLP001 in IPEC-J2 cells. Average Ct values of each gene were pBD3 30.3/29.7, PG1-5 30.4/30.6, pEP2C 30.0/30.4, pBD114 31.1/30.3, pBD129 30.2/29.8, respectively.
L. plantarum ZLP001-Induced HDP Expression Is Regulated by MAPK Signaling in IPEC-J2 Cells
Activation of MAPK has been associated with HDP expression (12). Western blot analysis revealed increased ERK1/2 and JNK phosphorylation but not p38 phosphorylation after L. plantarum ZLP001 treatment (Figure 4), suggesting that L. plantarum ZLP001 activated both the ERK1/2 and JNK signaling pathways.
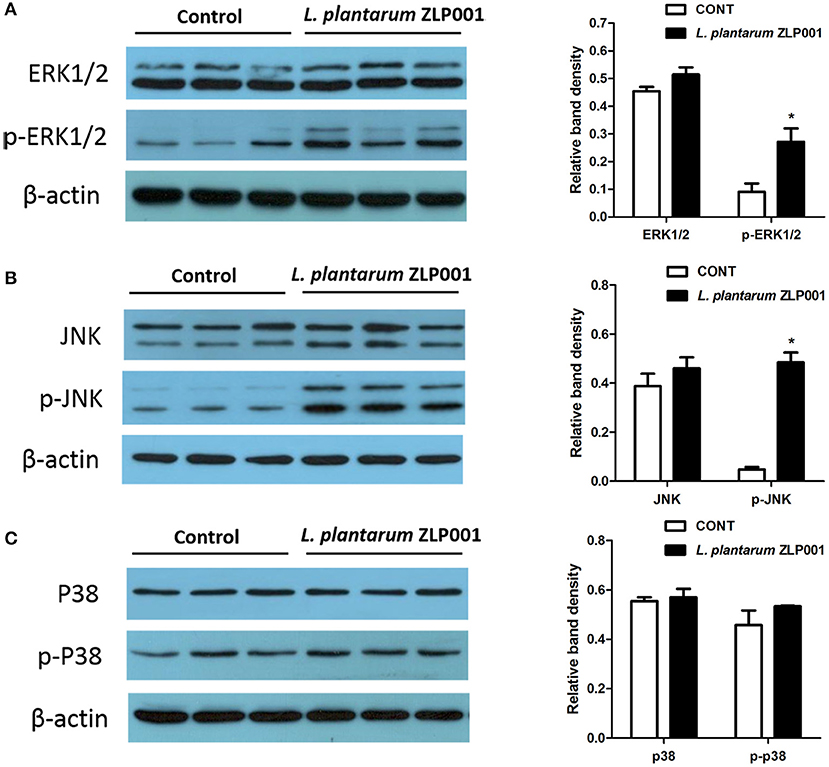
Figure 4. Role of mitogen-activated protein kinase (MAPK) signaling pathways in L. plantarum ZLP001-induced porcine host defense peptide (HDP) expression and secretion. IPEC-J2 cells were incubated with L. plantarum ZLP001 at 108 CFU/ml for 6 h, and protein expression and phosphorylation of extracellular signal-regulated kinase (ERK)1/2 (A), ERK (B), and p38 (C) in whole-cell lysates were assessed by Western blot analysis.
We also examined whether these two pathways are required for L. plantarum ZLP001-induced porcine HPD expression using specific inhibitors (Figure 5A). Neither the ERK1/2 inhibitor U0126 nor the JNK inhibitor SP600125 significantly induced HDP expression in IPEC-J2 cells. U0126 and SP600125 treatments partially suppressed the increases in pBD2, pBD3, and pEP2C mRNA expression induced by L. plantarum ZLP001. U0126 failed to inhibit PG1-5 induction and SP600125 failed to inhibit pBD114 and pBD129 induction by L. plantarum ZLP001 at the mRNA level. These results suggested that different HDPs are regulated by different signaling pathways.
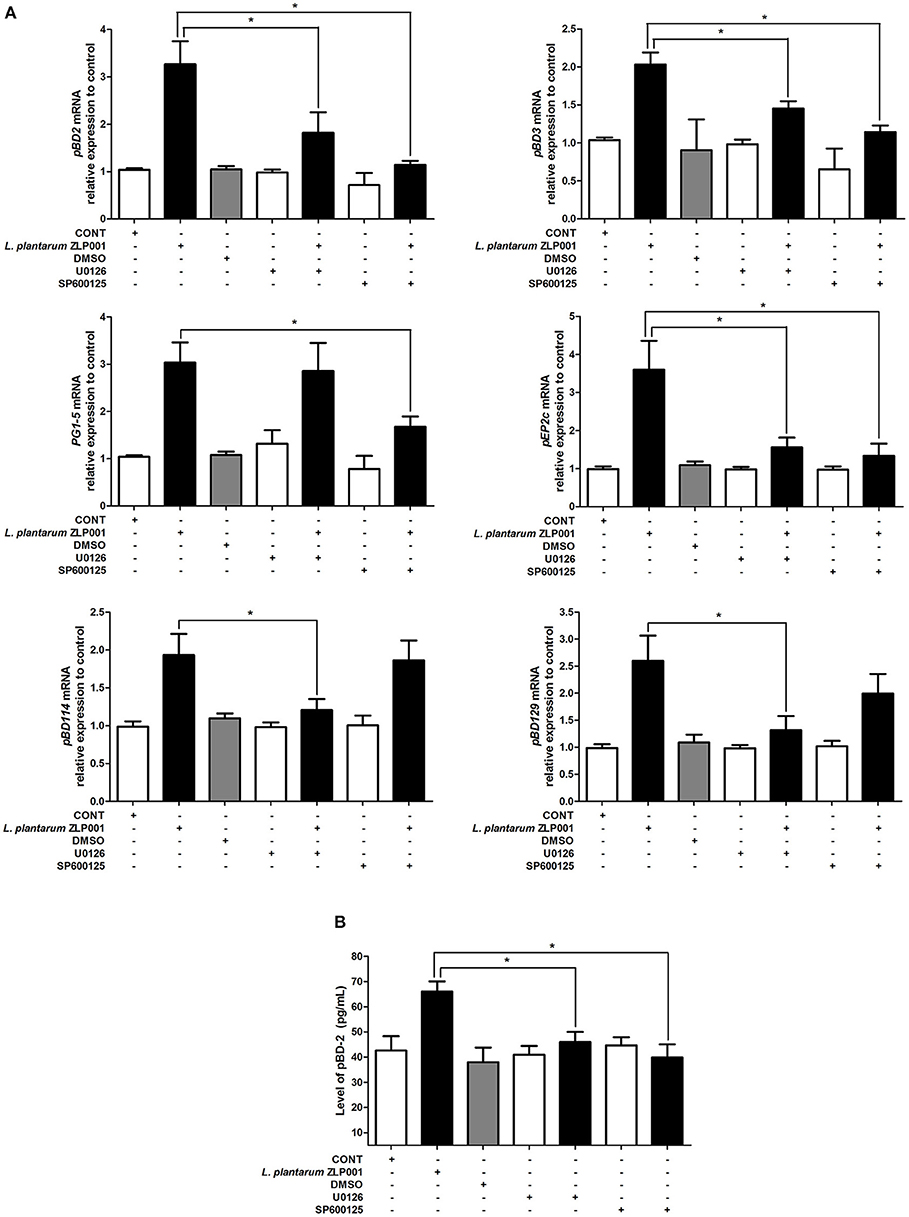
Figure 5. Blocking the key proteins of mitogen-activated protein kinase (MAPK) signaling pathway affects porcine host defense peptide (HDP) expression and production. (A) Inhibition of extracellular signal-regulated kinase (ERK)1/2 and c-jun N-terminal kinase (JNK) blocks porcine HDP mRNA expression. IPEC-J2 cells were preincubated with the specific ERK1/2 inhibitor U0126 (10 μM) and the specific JNK inhibitor SP600125 (10 μM) 1 h before incubation with 108 CFU/ml L. plantarum ZLP001 for 6 h. Average Ct values of each gene were pBD2 30.9/29.3/31.6/31.1/30.0/31.5/30.5, PG1-5 30.1/28.5/29.9/30.2/28.2/31.0/29.3, pBD114 31.6/30.5/31.3/32.0/31.2/31.3/30.5, pBD3 30.3/29.1/31.6/30.0/29.4/31.1/29.9, pEP2C 30.1/28.7/29.7/30.2/29.6/30.7/30.3, and pBD129 30.7/29.0/30.1/30.6/30.0/30.4/29.4, respectively. (B) Inhibition of ERK1/2 and JNK blocks porcine pBD2 production. The concentration of pBD2 in the supernatant of L. plantarum ZLP001-treated IPEC-J2 cells was analyzed by ELISA. Gray level, mRNA expression data, and ELISA data are presented as the mean ± SEM of three independent experiments. *P < 0.05 vs. non-treated control group.
When we measured pBD2 secretion by IPEC-J2 cells upon U0126 and SP600125 treatment, we found that pBD2 concentrations in culture media were significantly decreased after inhibitor treatment compared with those in cells treated with L. plantarum ZLP001 alone (Figure 5B). These findings suggested that pBD2 production induced by L. plantarum ZLP001 was blocked by the ERK1/2 and JNK inhibitors.
Activator Protein (AP)-1 Regulates L. plantarum ZLP001-Induced HDP Upregulation in IPEC-J2 Cells
The AP-1 signaling pathway plays an important role in the innate immune response. AP-1 is composed of hetero- and homodimers of c-jun and c-fos family members (24). Therefore, we evaluated whether L. plantarum ZLP001-induced HDP upregulation is regulated by c-fos and c-jun, which are the main downstream effectors activated by ERK1/2 and JNK signaling. Treatment of IPEC-J2 cells with L. plantarum ZLP001 for 6 h increased protein expression of c-fos and c-jun in IPEC-J2 cells (Figure 6A). In addition, c-fos and c-jun activities were induced by L. plantarum ZLP001 when compared to the levels in non-treated IPEC-J2 cells (P < 0.05; Figure 6B). These results suggested a role for c-fos and c-jun in regulating the stimulatory effects of L. plantarum ZLP001 on HDP expression in IPEC-J2 cells.
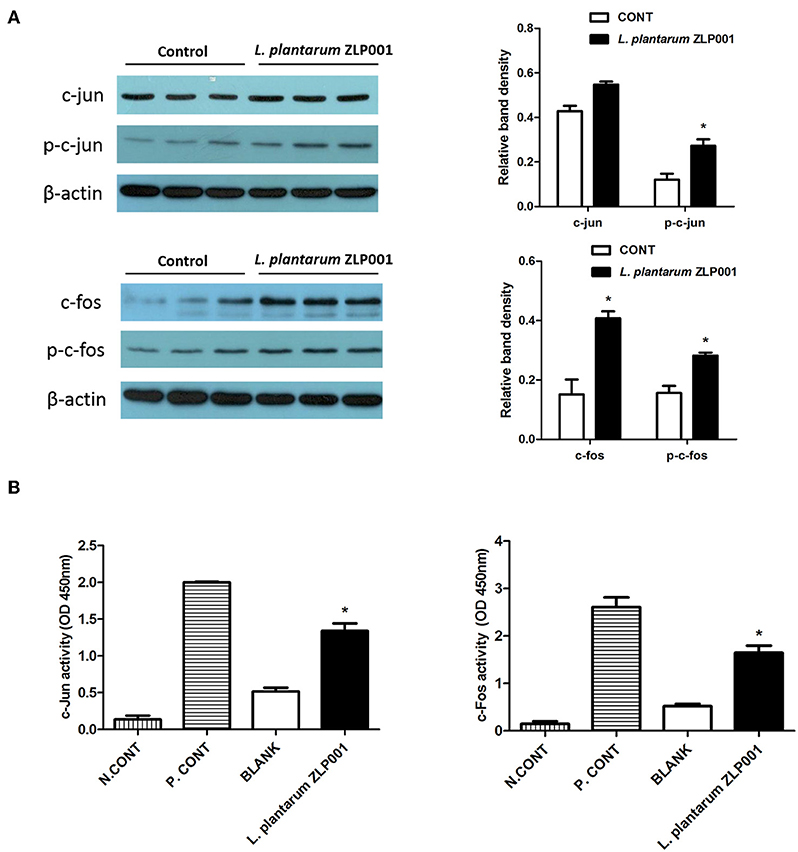
Figure 6. Role of transcription factor mitogen-activated protein kinase (MAPK)/activator protein (AP)-1 in L. plantarum ZLP001-induced host defense peptide (HDP) expression. (A) L. plantarum ZLP001 induces c-fos and c-jun protein expression. IPEC-J2 cells were incubated with 108 CFU/ml L. plantarum ZLP001 for 6 h, and protein expression and phosphorylation of c-jun and c-fos were assessed by Western blot analysis in whole-cell lysates. (B) L. plantarum ZLP001 increased AP-1 subunit c-jun and c-fos activities. c-jun and c-fos activities in nuclear extracts were assessed by TransAM assay. A nuclear extract provided from the supplier served as a positive control, and a negative control was incubated without nuclear extract. Gray level and activity data are presented as the mean ± SEM of three independent experiments. *P < 0.05 vs. non-treated control group.
Discussion
The mucosal immune system plays an important role in maintaining gastrointestinal function in pigs (25). The intestinal epithelium of piglets is immature and is weakened under weaning stress. The establishment of a strong gastrointestinal immune system after weaning is crucial to enhancing tolerance to harmful pathogens (26). Nutritional modulation has been proposed as an effective strategy to stimulate the mucosal innate immune system (27). Probiotics may induce endogenous functional molecules, including HDPs, through microbe–host cell signaling, thus improving the intestinal microecology and modulating the immune response (28). There are two major HDP families in pigs, defensins and cathelicidins, and most members of both families are secreted by gastrointestinal epithelial cells (10). The stimulatory potential of probiotic strains on HDP expression in different species, including pigs, has been demonstrated (12, 13). However, the underlying signaling mechanisms remained not completely understood.
We investigated the inductive effects of L. plantarum ZLP001 on porcine HDP expression using in vivo and in vitro models. In piglets, the effects were tissue-specific; the L. plantarum ZLP001-treated group had significantly higher mRNA levels of pBD2, pBD3, pEP2C, and pBD114 in the jejunum and ileum than those in the control group, whereas no significant changes were observed in the duodenum after L. plantarum ZLP001 treatment. In line with our findings, a previous study showed that Lactobacillus reuteri I5007 significantly inducted pBD2 expression in the jejunum but not in the ileum or colon (13). In addition, we found significant induction of PG1-5 in the duodenum and no induction of pBD129 in the ileum besides others segments. This indicated that different genes respond differentially to L. plantarum ZLP001 treatment in various intestinal tissue types, which might be due to gene- and cell type-specific differences in various porcine intestinal tissue types (10). Gene- and cell type-specific HDP induction by L. plantarum ZLP001 was verified by in vitro findings in IPEC-J2 and 3D4/31 cells. Although pEP2C showed the highest mRNA induction response among the six HDPs detected in both cell types, the other HDPs showed markedly different responses. Such specific regulation has also been demonstrated in other species and with other stimulators (10, 29, 30). In addition, it is well-known that the effect of probiotics is strain-specific in most cases. Different Lactobacillus strains also showed varying capacities in inducing a human HDP (hBD-2) mRNA expression (12). Comparative studies of multiple Lactobacillus strains on porcine HDP induction are needed in the future.
The innate immune system initiates a response to microorganisms via pattern recognition receptors (PRRs), which have been extensively studied to explain the regulatory effects of immunobiotic bacteria in both immune and intestinal epithelial cells (31). TLR2 is an important PRR that plays an essential role in immune regulation by probiotics. It has a broad recognition profile that includes products from gram-positive bacteria, including lipoteichoic acid, peptidoglycan, lipoproteins, and so on (32). TLR2 is essential to human HDP DEFB131 production after lipoteichoic acid treatment, as evidenced by the result that TLR2-silenced RWPE-1 cells exhibited diminished DEFB131 production (33). We found that TLR2-silenced IPEC-J2 cells show decreased pBD2 mRNA expression and secretion upon L. plantarum ZLP001 treatment, which indicates that L. plantarum ZLP001 exerts an HDP production-inductive effect through TLR2 recognition of its products. Similarly, Schlee et al. (12) suggested that Lactobacillus strains induce human hBD-2 likely via a bacterial cell wall component, as Lactobacillus pellets showed similar hBD-2-inductive capacity as culture media. In contrast, in a study using the probiotic strain Escherichia coli Nissle 1917, culture supernatant exhibited a 13-fold stronger hBD-2-inductive effect than pelleted bacteria (34). We have confirmed that live bacteria of L. plantarum ZLP001 exhibit highest induction effect on HDP expression compared with other different processing bacteria, including bacterial free culture supernatant, in our preliminary experiment. However, which bacterial cell component or products of L. plantarum ZLP001 are recognized by TLR2 and provoked subsequent responses responsible for the stimulation of HDP still need to be clarified in further studies. In addition, whether TLR2 exerts its actions by forming heterodimers with other TLRs (e.g., TLR1 or TLR6) (35, 36) and cooperating with TLR2 coreceptors (37) remains to be investigated.
TLR2 activation triggers signaling pathways involving MAPKs and transcription factors. MAPKs, including the three major MAPKs, ERK, p38, and JNK, regulate various processes associated with innate immunity and the proinflammatory response, as well as with cell metabolism and transcription (38, 39). The family of MAPK pathways is linked to HDP expression by various stimuli in different species (34, 40, 41). For example, human cathelicidin LL-37 expression induced by short-chain fatty acids is mediated by the MEK/ERK pathway, and branched-chain amino acids can activate ERK1/2 and the downstream transcription factor 90RSK to induce porcine β-defensin expression (41, 42). On the other hand, epigallocatechin-3-gallate induced pBD2 expression via p38, not ERK or JNK signaling (43). In the present study, ERK1/2 and JNK were clearly activated during L. plantarum ZLP001-induced HDP expression but not p38, and the involvement of these two MAPKs in HDP expression induction was corroborated by pharmacological inhibition experiments. In line with our findings, a previous study on human hBD-2 gene expression upon Caco-2 cells after treatment with the probiotics Lactobacillus fermentum, Lactobacillus acidophilus, and Pediococcus pentosaceus revealed that ERK1/2, JNK, and p38 were apparently activated by these strains, although p38 seemed less important to the regulation of hBD-2 (12). However, studies of the probiotic strain Escherichia coli 1917 demonstrated that its inductive effect on hBD-2 was mediated by the JNK pathway, not the ERK1/2 or p38 signaling (34). Our and previous findings clearly indicate that probiotics modulate immune functions against pathogens in a species- and strain-specific manner (44). Furthermore, the present study revealed that ERK1/2 and JNK inhibitors had differential effects on different HDP genes, indicating that both ERK1/2 and JNK separately contribute to each porcine HDP regulation. This suggests that the mechanisms underlying the regulation of different HDP genes are different perhaps principally owing to different promoter structures and transcription factor-binding sites. Certainly, the possibility that other signaling pathways participate in HDP induction cannot be excluded based on our present results. Specific recognition mechanisms and other pathways should be addressed in future investigations.
L. plantarum ZLP001-induced upregulation of HDPs in pigs may involve a transcriptional mechanism. However, the molecular mechanisms involved in the transcriptional modulation of HDPs are not well-understood. The AP-1 transcription factor complex is the main transcription factor downstream of MAPK pathways and can bind with specific DNA sequences (45, 46). In the present study, the AP-1 subunits c-fos and c-jun were activated in IPEC-J2 cells incubated with L. plantarum ZLP001. They may bind to specific porcine HDP promoter DNA consensus sequences to initiate transcription. DNA-binding activity is an important capacity of transcription factors for transcriptional regulation. Our further studies corroborated that L. plantarum ZLP001 promotes c-fos and c-jun DNA-binding activities. These results confirmed the important role of AP-1 in the stimulation of porcine HDP expression in response to L. plantarum ZLP001. The study by Schlee et al. (12) also suggested that binding of AP-1 to the hBD-2 promoter plays an essential role in hBD-2 promoter activation initiated by L. fermentum and P. pentosaceus. Further investigations will be needed to explore the promoter region of each porcine HDP gene and find its specific binding site for transcription factors downstream of MAPK or other signaling pathways that play essential roles in the regulation of HDP expression.
Conclusion
This study revealed that L. plantarum ZLP001 supplementation induces porcine HDP expression and production in vivo and in vitro. Furthermore, our study provided insights into the mechanism underlying L. plantarum ZLP001-induced porcine HDP expression (Figure 7). Upon L. plantarum ZLP001 stimulation, TLR2 recruits signaling molecules to intracellular signaling pathways within IPEC-J2 cells, leading to ERK1/2/JNK and c-fos/c-jun activation. We believe that the induction of defensins by probiotics such as L. plantarum ZLP001 might be an interesting new dietary strategy for strengthening innate defense function in weaning piglets. Further studies should focus on identifying bacterial cell wall components that play major roles in this inductive process and the specific signaling pathways regulating specific HDPs.
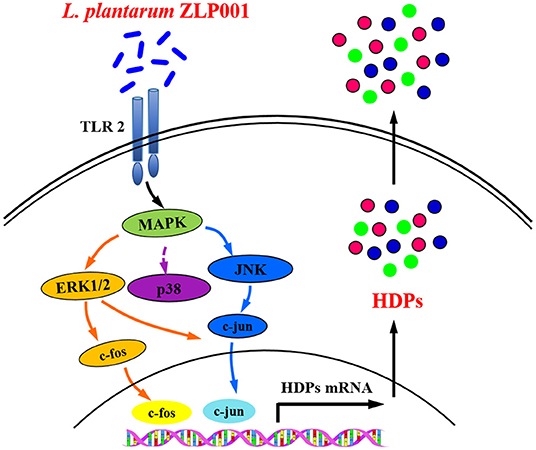
Figure 7. Suggested mechanism by which L. plantarum ZLP001 modulates porcine intestinal endogenous host defense peptide (HDP) synthesis. L. plantarum ZLP001 may induce porcine HDP expression and secretion through toll-like receptor (TLR)2 recognition as well as extracellular signal-regulated kinase (ERK)1/2/c-jun N-terminal kinase (JNK) and c-jun/c-fos pathway activation.
Data Availability Statement
All datasets generated for this study are included in the article/Supplementary Material.
Ethics Statement
The animal study was reviewed and approved by Animal Care and Use Committee of Institute of Animal Husbandry and Veterinary Medicine of the Beijing Academy of Agriculture and Forestry Sciences. Written informed consent was obtained from the owners for the participation of their animals in this study.
Author Contributions
JW and HJ conceived and designed the study. JW, WZ, SW, HL, and DZ performed the experiments. JW and WZ analyzed the data and drafted the manuscript. YW contributed reagents and materials. All authors read and approved the final manuscript.
Funding
This study was financially supported by the Special Program on Science and Technology Innovation Capacity Building of BAAFS (KJCX20180414 and KJCX201914), the National Natural Science Foundation of China (31972576 and 31702303), and the International Scientific and Technological Cooperation Funding of BAAFS (GJHZ2018-06).
Conflict of Interest
The authors declare that the research was conducted in the absence of any commercial or financial relationships that could be construed as a potential conflict of interest.
Supplementary Material
The Supplementary Material for this article can be found online at: https://www.frontiersin.org/articles/10.3389/fimmu.2019.02691/full#supplementary-material
References
1. Campbell JM, Crenshaw JD, Polo J. The biological stress of early weaned piglets. J Anim Sci Biotechnol. (2013) 4:19. doi: 10.1186/2049-1891-4-19
2. Heo JM, Opapeju FO, Pluske JR, Kim JC, Hampson DJ, Nyachoti CM. Gastrointestinal health and function in weaned pigs: a review of feeding strategies to control post-weaning diarrhoea without using in-feed antimicrobial compounds. J Anim Physiol Anim Nutr. (2013) 97:207–37. doi: 10.1111/j.1439-0396.2012.01284.x
3. Gresse R, Chaucheyras-Durand F, Fleury MA, Van de Wiele T, Forano E, Blanquet-Diot S. Gut microbiota dysbiosis in postweaning piglets: understanding the keys to health. Trends Microbiol. (2017) 25:851–73. doi: 10.1016/j.tim.2017.05.004
4. Moravej H, Moravej Z, Yazdanparast M, Heiat M, Mirhosseini A, Moosazadeh, et al. Antimicrobial peptides: features, action, and their resistance mechanisms in bacteria. Microb Drug Resist. (2018) 24:747–67. doi: 10.1089/mdr.2017.0392
5. Hancock RE, Haney EF, Gill EE. The immunology of host defence peptides: beyond antimicrobial activity. Nat Rev Immunol. (2016) 16:321–34. doi: 10.1038/nri.2016.29
6. Mandal SM, Manna S, Mondal S, Ghosh AK, Chakraborty R. Transcriptional regulation of human defense peptides: a new direction in infection control. Biol Chem. (2018) 399:1277–84. doi: 10.1515/hsz-2018-0182
7. Robinson K, Ma X, Liu Y, Qiao S, Hou Y, Zhang G. Dietary modulation of endogenous host defense peptide synthesis as an alternative approach to in-feed antibiotics. Anim Nutr. (2018) 4:160–9. doi: 10.1016/j.aninu.2018.01.003
8. Becker T, Loch G, Beyer M, Zinke I, Aschenbrenner A, Carrea P, et al. FOXO-dependent regulation of innate immune homeostasis. Nature. (2010) 463:369–73. doi: 10.1038/nature08698
9. Talukder P, Satho T, Irie K, Sharmin T, Hamady D, Nakashima Y, et al. Trace metal zinc stimulates secretion of antimicrobial peptide LL-37 from Caco-2 cells through ERK and p38 MAP kinase. Int Immunopharmacol. (2011) 11:141–4. doi: 10.1016/j.intimp.2010.10.010
10. Zeng X, Sunkara LT, Jiang W, Bible M, Carter S, Ma X, et al. Induction of porcine host defense peptide gene expression by short-chain fatty acids and their analogs. PLoS ONE. (2013) 8:e72922. doi: 10.1371/journal.pone.0072922
11. Merriman KE, Kweh MF, Powell JL, Lippolis JD, Nelson CD. Multiple β- defensin genes are upregulated by the vitamin D pathway in cattle. J Steroid Biochem Mol Biol. (2015) 154:120–9. doi: 10.1016/j.jsbmb.2015.08.002
12. Schlee M, Harder J, Koten B, Stange EF, Wehkamp J, Fellermann K. Probiotic lactobacilli and VSL#3 induce enterocyte β-defensin 2. Clin Exp Immunol. (2008) 151:528–35. doi: 10.1111/j.1365-2249.2007.03587.x
13. Liu H, Hou C, Wang G, Jia H, Yu H, Zeng X, et al. Lactobacillus reuteri I5007 modulates intestinal host defense peptide expression in the model of IPEC-J2 cells and neonatal piglets. Nutrients. (2017) 9:559. doi: 10.3390/nu9060559
14. Wan LY, Chen ZJ, Shah NP, El-Nezami H. Modulation of intestinal epithelial defense responses by probiotic bacteria. Crit Rev Food Sci Nutr. (2016) 56:2628–41. doi: 10.1080/10408398.2014.905450
15. Möndel M, Schroeder B, Zimmermann K, Huber H, Nuding S, Beisner J, et al. Probiotic, E. coli treatment mediates antimicrobial human beta-defensin synthesis and fecal excretion in humans. Mucosal Immunol. (2009) 2:166–72. doi: 10.1038/mi.2008.77
16. Wang J, Ji H, Zhang D, Liu H, Wang S, Shan D, et al. Assessment of probiotic properties of Lactobacillus plantarum ZLP001 isolated from gastrointestinal tract of weaning pigs. Afr J Biotechnol. (2011) 10:11303–8. doi: 10.5897/AJB11.255
17. Wang J, Ji H, Wang S, Zhang D, Liu H, Shan D, et al. Lactobacillus plantarum ZLP001: in vitro assessment of antioxidant capacity and effect on growth performance and antioxidant status in weaning piglets. Asian Austral J Anim Sci. (2012) 25:1153–8. doi: 10.5713/ajas.2012.12079
18. Wang J, Zeng YX, Wang SX, Liu H, Zhang DY, Zhang W, et al. Swine-derived probiotic Lactobacillus plantarum inhibits growth and adhesion of enterotoxigenic Escherichia coli and mediates host defense. Front Microbiol. (2018) 9:1364. doi: 10.3389/fmicb.2018.01364
19. National Research Council (2012). Nutrient Requirements of Swine, 11th Edn. Washington, DC: National Academy Press.
20. Agricultural industry standards of the People's Republic of China. (2004). Feeding Standard of Swine. Beijing, China Agriculture Press.
21. Rhoads JM, Chen W, Chu P, Berschneider HM, Argenzio RA, Paradiso AM. L-Glutamine and L-asparagine stimulate Na+/H+ exchange in porcine jejunal enterocytes. Am J Physiol. (1994) 266:G828–38. doi: 10.1152/ajpgi.1994.266.5.G828
22. Murofushi Y, Villena J, Morie K, Kanmani P, Tohno M, Shimazu T, et al. The toll-like receptor family protein RP105/MD1 complex is involved in the immunoregulatory effect of exopolysaccharides from Lactobacillus plantarum N14. Mol Immunol. (2015) 64:63–75. doi: 10.1016/j.molimm.2014.10.027
23. Bermudez-Brito M, Plaza-Díaz J, Muñoz-Quezada S, Gómez-Llorente C, Gil A. Probiotic mechanisms of action. Ann Nutr Metab. (2012) 61:160–74. doi: 10.1159/000342079
24. Vesely PW, Staber PB, Hoefler G, Kenner L. Translational regulation mechanisms of AP-1 proteins. Mutat Res. (2009) 682:7–12. doi: 10.1016/j.mrrev.2009.01.001
25. Burkey TE, Skjolaas KA, Minton JE. Board-invited review: porcine mucosal immunity of the gastrointestinal tract. J Anim Sci. (2009) 87:1493–501. doi: 10.2527/jas.2008-1330
26. Stokes CR. The development and role of microbial-host interactions in gut mucosal immune development. J Anim Sci Biotechnol. (2017) 8:12. doi: 10.1186/s40104-016-0138-0
27. Wu D, Lewis ED, Pae M, Meydani SN. Nutritional modulation of immune function: analysis of evidence, mechanisms, and clinical relevance. Front Immunol. (2019) 9:3160. doi: 10.3389/fimmu.2018.03160
28. Fischbach MA, Segre JA. Signaling in host-associated microbial communities. Cell(2016) 164:1288–300. doi: 10.1016/j.cell.2016.02.037
29. Schauber J, Dorschner RA, Yamasaki K, Brouha B, Gallo RL. Control of the innate epithelial antimicrobial response is cell-type specific and dependent on relevant microenvironmental stimuli. Immunology. (2006) 118:509–19. doi: 10.1111/j.1365-2567.2006.02399.x
30. Sunkara LT, Achanta M, Schreiber NB, Bommineni YR, Dai G, Jiang W, et al. Butyrate enhances disease resistance of chickens by inducing antimicrobial host defense peptide gene expression. PLoS ONE. (2011) 6:e27225. doi: 10.1371/journal.pone.0027225
31. Kanmani P, Albarracin L, Kobayashi H, Iida H, Komatsu R, Humayun Kober AKM, et al. Exopolysaccharides from Lactobacillus delbrueckii OLL1073R-1 modulate innate antiviral immune response in porcine intestinal epithelial cells. Mol Immunol. (2018) 93:253–65. doi: 10.1016/j.molimm.2017.07.009
32. Zähringer U, Lindner B, Inamura S, Heine H, Alexander C. TLR2 - promiscuous or specific? A critical re-evaluation of a receptor expressing apparent broad specificity. Immunobiology. (2008) 213:205–24. doi: 10.1016/j.imbio.2008.02.005
33. Kim JH, Kim KH, Kim HJ, Lee J, Myung SC. Expression of beta-defensin 131 promotes an innate immune response in human prostate epithelial cells. PLoS ONE. (2015) 10:e0144776. doi: 10.1371/journal.pone.0144776
34. Wehkamp J, Harder J, Wehkamp K, Wehkamp-von Meissner B, Schlee M, Enders C, et al. NF-kappaB- and AP-1-mediated induction of human beta defensin-2 in intestinal epithelial cells by Escherichia coli Nissle 1917: a novel effect of a probiotic bacterium. Infect Immun. (2004) 72:5750–8. doi: 10.1128/IAI.72.10.5750-5758.2004
35. Jin MS, Kim SE, Heo JY, Lee ME, Kim HM, Paik SG, et al. Crystal structure of the TLR1-TLR2 heterodimer induced by binding of a tri-acylated lipopeptide. Cell. (2007) 130:1071–82. doi: 10.1016/j.cell.2007.09.008
36. Kang JY, Nan X, Jin MS, Youn SJ, Ryu YH, Mah S, et al. Recognition of lipopeptide patterns by Toll-like receptor 2-Toll-like receptor 6 heterodimer. Immunity. (2009) 31:873–84. doi: 10.1016/j.immuni.2009.09.018
37. Nilsen NJ, Deininger S, Nonstad U, Skjeldal F, Husebye H, Rodionov D, et al. Cellular trafficking of lipoteichoic acid and Toll-like receptor 2 in relation to signaling: role of CD14 and CD36. J Leukoc Biol. (2008) 84:280–91. doi: 10.1189/jlb.0907656
38. Tiedje C, Holtmann H, Gaestel M. The role of mammalian MAPK signaling in regulation of cytokine mRNA stability and translation. J Interferon Cytokine Res. (2014) 34:220–32. doi: 10.1089/jir.2013.0146
39. Sun Y, Liu WZ, Liu T, Feng X, Yang N, Zhou HF. Signaling pathway of MAPK/ERK in cell proliferation, differentiation, migration, senescence and apoptosis. J Recept Signal Transduct Res. (2015) 35:600–4. doi: 10.3109/10799893.2015.1030412
40. Sunkara LT, Zeng X, Curtis AR, Zhang G. Cyclic AMP synergizes with butyrate in promoting beta-defensin 9 expression in chickens. Mol Immunol. (2014) 57:171–80. doi: 10.1016/j.molimm.2013.09.003
41. Ren M, Zhang S, Liu X, Li S, Mao X, Zeng X, et al. Different lipopolysaccharide branched-chain amino acids modulate porcine intestinal endogenous β-defensin expression through the Sirt1/ERK/90RSK pathway. J Agric Food Chem. (2016) 64:3371–9. doi: 10.1021/acs.jafc.6b00968
42. Schauber J, Svanholm C, Termén S, Iffland K, Scheppach W, Melcher R, et al. Expression of the cathelicidin LL-37 is modulated by short chain fatty acids in colonocytes: relevance of signalling pathways. Gut. (2003) 52:735–41. doi: 10.1136/gut.52.5.735
43. Wan ML, Ling KH, Wang MF, El-Nezami H. Green tea polyphenol epigallocatechin-3-gallate improves epithelial barrier function by inducing the production of antimicrobial peptide pBD-1 and pBD-2 in monolayers of porcine intestinal epithelial IPEC-J2 cells. Mol Nutr Food Res. (2016) 60:1048–58. doi: 10.1002/mnfr.201500992
44. Kandasamy S, Vlasova AN, Fischer DD, Chattha KS, Shao L, Kumar A, et al. Unraveling the differences between gram-positive and gram-negative probiotics in modulating protective immunity to enteric infections. Front Immunol. (2017) 8:334. doi: 10.3389/fimmu.2017.00334
45. Li JK, Nie L, Zhao YP, Zhang YQ, Wang X, Wang SS. IL-17 mediates inflammatory reactions via p38/c-Fos and JNK/c-Jun activation in an AP-1-dependent manner in human nucleus pulposus cells. J Transl Med. (2016) 14:77. doi: 10.1186/s12967-016-0833-9
46. Priyamvada S, Anbazhagan AN, Kumar A, Soni V, Alrefai WA, Gill RK, et al. Lactobacillus acidophilus stimulates intestinal P-glycoprotein expression via a c-Fos/c-Jun-dependent mechanism in intestinal epithelial cells. Am J Physiol Gastrointest Liver Physiol. (2016) 310:G599–608. doi: 10.1152/ajpgi.00210.2015
Keywords: Lactobacillus plantarum ZLP001, host defense peptide, toll-like receptor 2, mitogen-activated protein kinase, activator protein 1, weaning piglet
Citation: Wang J, Zhang W, Wang S, Liu H, Zhang D, Wang Y and Ji H (2019) Swine-Derived Probiotic Lactobacillus plantarum Modulates Porcine Intestinal Endogenous Host Defense Peptide Synthesis Through TLR2/MAPK/AP-1 Signaling Pathway. Front. Immunol. 10:2691. doi: 10.3389/fimmu.2019.02691
Received: 18 June 2019; Accepted: 31 October 2019;
Published: 19 November 2019.
Edited by:
Fabrizio Ceciliani, University of Milan, ItalyReviewed by:
Henk Peter Haagsman, Utrecht University, NetherlandsEric Cox, Ghent University, Belgium
Shiyan Qiao, China Agricultural University (CAU), China
Zheng Ruan, Nanchang University, China
Shuai Chen, Institute of Subtropical Agriculture, Chinese Academy of Sciences, China
Copyright © 2019 Wang, Zhang, Wang, Liu, Zhang, Wang and Ji. This is an open-access article distributed under the terms of the Creative Commons Attribution License (CC BY). The use, distribution or reproduction in other forums is permitted, provided the original author(s) and the copyright owner(s) are credited and that the original publication in this journal is cited, in accordance with accepted academic practice. No use, distribution or reproduction is permitted which does not comply with these terms.
*Correspondence: Haifeng Ji, jhf207@126.com
†These authors have contributed equally to this work