- 1Vita-Salute San Raffaele University, Milan, Italy
- 2Division of Immunology, Transplantation and Infectious Diseases, IRCCS Ospedale San Raffaele, Milan, Italy
Regulated hemostasis, inflammation and innate immunity entail extensive interactions between platelets and neutrophils. Under physiological conditions, vascular inflammation offers a template for the establishment of effective intravascular immunity, with platelets providing neutrophils with an array of signals that increase their activation threshold, thus limiting collateral damage to tissues and promoting termination of the inflammatory response. By contrast, persistent systemic inflammation as observed in immune-mediated diseases, such as systemic vasculitides, systemic sclerosis, systemic lupus erythematosus or rheumatoid arthritis is characterized by platelet and neutrophil reciprocal activation, which ultimately culminates in the generation of thrombo-inflammatory lesions, fostering vascular injury and organ damage. Here, we discuss recent evidence regarding the multifaceted aspects of platelet-neutrophil interactions from bone marrow precursors to shed microparticles. Moreover, we analyse shared and disease-specific events due to an aberrant deployment of these interactions in human diseases. To restore communications between the pillars of the immune-hemostatic continuum constitutes a fascinating challenge for the near future.
Introduction
The Immune-Hemostatic Continuum
The circulatory system provides functional integration to tissues throughout the body and constitutes a dynamic platform for tasks, such as immune patrolling and defense against threats (1–3). Consistently, abnormalities in blood cells and cardiovascular manifestations are disproportionately represented in systemic autoimmune diseases, reflecting a network of interactions among circulating elements in the blood (4–7). Humoral moieties, such as complement, opsonins, components of the coagulation cascade and regulators of the vascular tone lie at the lowest level of complexity in this system and provide immediate, stereotyped responses to abnormal changes in the environment, such as volume loss, vascular injury or pathogen invasion, besides supporting more elaborate, long-term tasks performed by cells or subcellular elements (8–11).
Cellular membranes allow the segregation of selected information in compartments and modulate their subsequent effects on the environment by integrating multiple stimuli. Circulating membrane-endowed players in the immuno-hemostatic network encompass leukocytes, platelets and microparticles, with the endothelium as a fourth static counterpart (12, 13). Platelets and neutrophils play a crucial role in the maintenance of vascular and tissue integrity and interact extensively and productively. Consistently, alterations in platelet-neutrophil cross-talk have dramatic long-range effects on vascular and immune homeostasis (14–16) and are growingly appreciated as targets for therapeutic intervention (17, 18).
Pathophysiology of Interactions Between Platelets and Neutrophils
Characters on the Stage: Platelets, Neutrophils, and Microparticles
Hemostasis and inflammation counterbalance the effect of injuring external stimuli. Selective regulation and polarization of these pathways enhance homeostatic responses at sites of tissue or vascular injury and minimize the detrimental effects to the host. Multiple mechanisms have developed, including variability in the lifespan of players involved in the immune-hemostatic balance and availability of soluble or membrane-bound moieties. Emission of membrane-endowed subcellular particles fine-tunes cellular activation and converts locally concentrated high-intensity responses into a sum of smaller but widespread and reciprocally independent biological events. Generation of extracellular vesicles enables the extension of the total membrane area interacting with the environment as well as of the range of potential cellular targets. In addition, segregation of information in multiple signaling quanta discloses the possibility of independent interactions with distinct cellular counterparts according to the differential needs of target tissues. Platelets are anucleate cell fragments released by megakaryocytes. After a multi-stage process of cytoplasm compartmentalisation and concentration of bioactive compounds into granules taking place over the course of days, platelets are released as elongated precursors (proplatelets), which undergo multiple iterative fission events to reach their final size. Preplatelets are round-shaped precursors constituting a reversible intermediate stage in the transition from proplatelets to platelets (19). Small vessels of the bone marrow, spleen and lung might deliver signals that facilitate the final process of platelet maturation (20).
Increased platelet demand and/or consumption during acute systemic inflammation warrants adaptation of megakaryocytes. Inflammatory cytokines, such as IL6 promote megakaryocyte increase in ploidy and prompt thrombocytopoiesis through increased liver synthesis of thrombopoietin as part of the acute phase response (21). Platelets released under inflammatory stress are usually larger in volume, which correlates with an increased ischemic risk at a clinical level (22). Alternative sites of thrombocytopoiesis, such as the lung, might become activated under stress conditions in mice (23) and possibly in patients with lung cancer (24). In addition, stem-like megakaryocyte progenitors can be activated on demand during interferon-α-driven inflammatory responses (25). Platelets survive for 7–10 days in circulation, where they surrogate the damaged endothelium during vascular injury, recognize and control invading pathogens and release stimuli to promote tissue repair (12).
Controlled exocytosis or integration of bioactive compounds into platelet membrane is crucial for these tasks. Platelets are endowed with three classes of granules: alpha-granules; dense granules and few lysosomes. Some authors also described “T granules” equipped with Toll-like receptor 9 as a potential fourth platelet compartment (26–28). Besides being providers of bioactive compounds through exocytosis, platelets also produce microparticles. Platelet-derived microparticles (PDμP) constitute a substantial fraction of circulating microparticles in humans under physiological conditions (29). PDμP can present with a variety of sizes, contents and functions (30–32) that range from facilitation of coagulation through tissue factor (TF) and phospholipid (phosphatidylserine) scaffolds (33, 34) to angiogenesis, tissue repair (35–37) and defensive responses (38). Modulation of neutrophil behavior through delivery of nucleic acids (RNA) or inflammatory signal intercellular transfer also occurs under inflammatory conditions (39–42). In addition, mitochondria-enriched PDμP modulate target cell metabolism (32). Megakaryocytes release microparticles as well, influencing bone marrow homeostasis and synergising with PDμP (32, 43, 44).
Neutrophils constitute the most abundant leukocyte population in the blood and are in charge of the early innate effector response to noxious stimuli (45). Neutrophils express a vast array of oxidative and proteolytic enzymes, which are preformed and stored in ready-to-use granules. Activated neutrophils express TF, promoting isolation of injured tissues through thrombosis (46, 47) and contributing to immune-thrombosis upon interaction with platelets (2, 3). Neutrophils have a limited lifespan (45), which is mirrored by the timing of multiple acute or hyperacute clinical manifestations of infectious and immune-mediated diseases (48–50). Autophagy induction under inflammatory conditions might extend neutrophil survival, causing chronicization of tissue damage and facilitating autoimmunity (40, 51–55). In addition hematopoietic stem cells and myeloid progenitors respond to extreme inflammatory stimuli (thanks to the expression of innate germ-line encoded receptors, such as Toll-like receptors) causing massive granulopoiesis (56, 57).
Phagocytosis and digestion of invading pathogens constitutes the default-mode defensive task performed by neutrophils. However, frustrated microbial phagocytosis promotes the generation of extracellular traps (NETs) (58), i.e., the extracellular release of threads of decondensed chromatin and microbicidal moieties with or without loss of membrane integrity and cell vitality (suicidal vs. vital NET generation) (59). At least in cases leading to cell death, granule content is partially repurposed to facilitate chromatin remodeling and histone citrullination (60–63). Besides having a role in antimicrobial defense, NETs are also generated in response to unconventional stimuli, such as amorphous crystals, apoptotic bodies, cytokines, microparticles and changes in osmolarity (64, 65). Factors driving neutrophils to “choose” NET generation as opposed to phagocytosis are partially understood (66). Unsolicited formation and impaired clearance of NETs implies persistent exposure of self-antigens and inflammatory stimuli, which facilitates the development of autoimmunity (67–73).
Neutrophils also account for tissue and vascular damage in immune-mediated diseases including inflammatory bowel diseases, systemic lupus erythematosus (SLE) rheumatoid arthritis (RA) and vasculitides either directly or through NET-induced facilitation of thrombosis (2, 67, 68, 74–76). Neutrophils cooperate to regulate immune activation by secreting soluble pattern recognition receptors, such as pentraxin-3 (PTX3) or ficolins (3, 77, 78). Furthermore, they influence the activation and survival of other immunocompetent cells and tune the degree of systemic inflammation (45). Finally, neutrophils emit microparticles loaded with nucleic acids and/or digestive enzymes and armed with tissue factor (47, 79, 80). Neutrophil-derived microparticles prime endothelial cells, macrophages and neutrophils themselves to inflammatory activation (80, 81) and possibly destabilize genomic integrity in target tissues preventing resolution of the immune response (82). Elevated levels of such microparticles, possibly interacting with NET constituents are detectable in blood of patients with immune-mediated diseases (83, 84).
Weaving the Plot: General Features of Platelet-Neutrophil Interactions
Platelets interact productively with multiple cells types (12), although neutrophils constitute preferential partners (62) (Figure 1). P-selectin expression on platelet surface (which interacts with P-selectin granulocyte ligand 1, PSGL1, constitutively expressed by neutrophils) initiates platelet-neutrophil interaction (85, 86) and occurs after vessel injury/endothelial activation (87), pathogen recognition (88), or aging (89). P-selectin-dependent platelet-neutrophil interaction recruits downstream integrin-dependent pathways and culminates in neutrophil activation and facilitated extravasation (90). Neutrophils interacting with platelets can either: (a) phagocytose them, quenching their thrombogenic and inflammatory potential (85); (b) progressing to the generation of NETs (88), an event influenced by the neutrophil metabolic state (66, 91, 92).
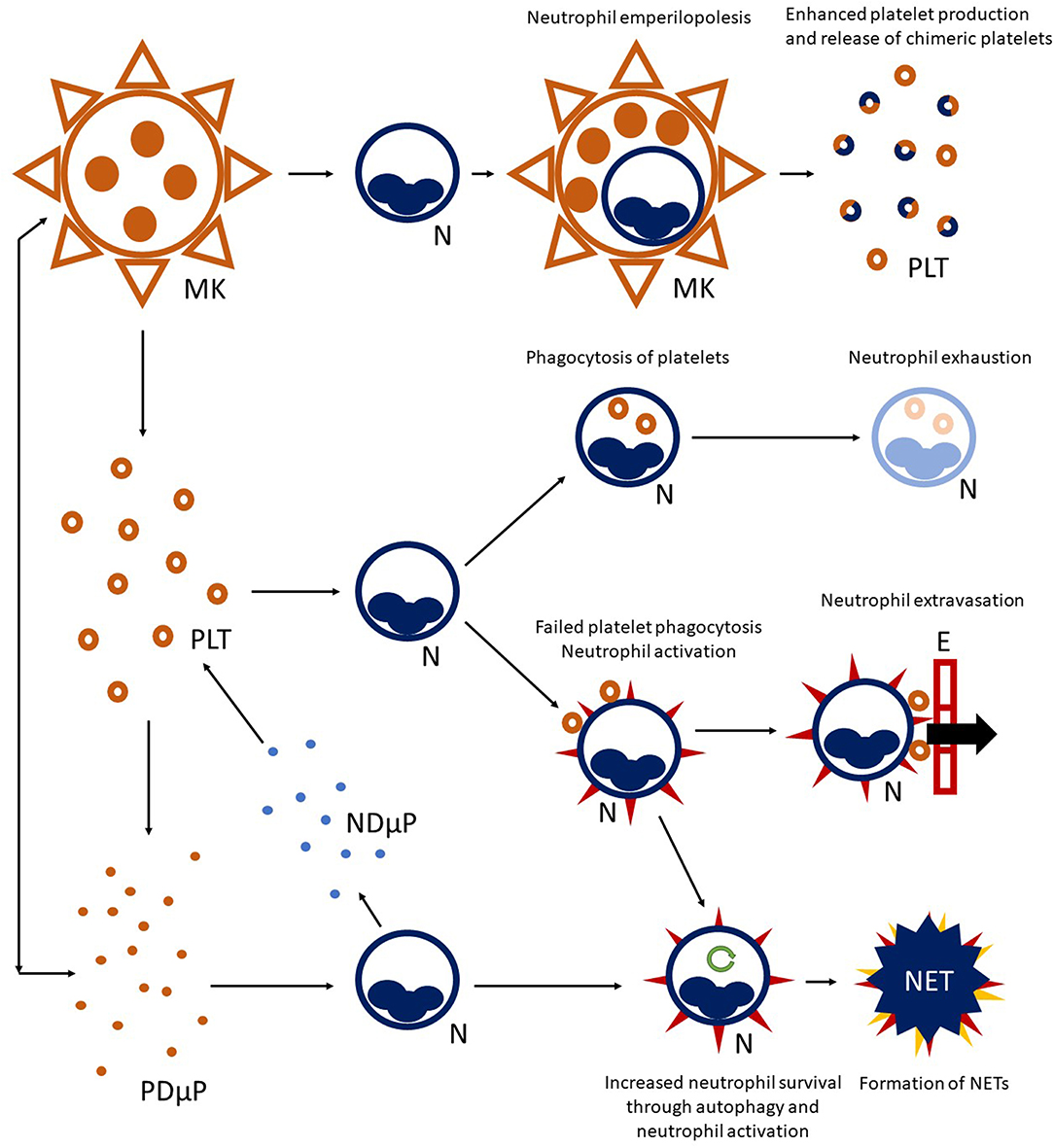
Figure 1. Platelet-neutrophil crosstalk. A complex network of interactions connects platelet (PLT) to neutrophil (N) biology. Megakaryocytes (MK) are able to interact with neutrophils residing in the bone marrow and engulf them, preserving their vitality (a phenomenon called emperilopolesis). Neutrophils may eventually escape megakaryocyte engulfment after donating membrane components to the host cell. This event causes enhanced platelet production and release of chimeric platelets. Activated platelets and neutrophils can further interact in the circulating blood, either directly through cell-cell contact and/or through the exchange of soluble compounds or microparticles. Engagement of platelets by neutrophils through the P-selectin/PSGL-1 axis, and later on, integrin-mediated bonds can lead to platelet-phagocytosis, resulting in neutrophil exhaustion. Alternatively, failed platelet clearance can promote neutrophil activation (heralded by expression of surface markers, such as tissue factor and activation of integrins—red spikes in the figure) and facilitate neutrophil extravasation through the endothelial wall (E). Activated neutrophils can also enter autophagy programmes (green circular arrow), extending their survival, and progress toward the formation of neutrophil extracellular traps (NET). Platelet-derived microparticles (PDμP), especially when loaded with the damage associated molecular pattern HMGB1, are potent inducers of NET generation. Neutrophil-derived microparticles (NDμP) constitute and additional channel for platelet-neutrophil interchanges and are thought to have a role in lipid metabolism.
Vital neutrophil internalization (emperilopolesis) into megakaryocytes occurs in the bone marrow. Engulfed neutrophils provide megakaryocytes with activating stimuli (causing a rise in platelet production) and donate membrane segments causing enhanced phosphatidylserine expression by chimeric daughter platelets (93). It is tempting to speculate that emperilopolesis holds the key to understanding the mechanisms of the later interactions between neutrophils and platelets in the circulation.
Platelets and megakaryocytes communicate long-range with neutrophils through exocytosed mediators and microparticles with enhancing actions on neutrophil activation (94, 95). The prototypic alarmin/damage-associated molecular pattern HMGB1, either as a soluble moiety or loaded into microparticles, is a potent promoter of extended neutrophil survival and of NET generation (40, 51, 96–99). Cooperation and bidirectional exchange of lipid metabolites between neutrophils and platelets through microparticles maximize the synthesis of prostaglandins, such as the pro-coagulant and vasoconstrictor signal, thromboxane A2 (100, 101) and to the activation of the complement cascade, which in turn promotes neutrophil recruitment and activation (102).
An Anthology of Recurring Topoi: Shared Events Linked to Platelet and Neutrophil Biology
The interaction between platelets and neutrophils impacts on multiple stereotyped pathological manifestations (Table 1). A first hint can be found in laboratory tests, such as blood cell counts. In most cases, platelet and leukocyte numbers roughly correlate with systemic inflammation (147), either due to disease activity or infections. By contrast, reduced blood cell counts are a hallmark of a minority of autoimmune conditions, including SLE and overlap syndromes and Felty's syndrome, but might dominate during sepsis, affecting survival. No specific evidence is available about the reciprocal interactions between platelets and neutrophils during severe thrombocytopenia as an isolated phenomenon. This fact might also be due to difficulties in uncoupling variations in platelet counts and neutrophil responses from shared inciting stimuli underlying both events at an experimental level. Nonetheless, consistent evidence from sepsis, idiopathic thrombocytopenic purpura, thrombotic thrombocytopenic purpura and thrombocytopenia induced by heparin or other drugs suggests that neutrophils are intravascularly activated and tend to generate NETs in association with low platelet counts, possibly contributing to dysregulated hemostasis and adverse clinical outcomes (148–153). Interestingly, viral infections causing thrombocytopenia seem to associate with the expansion of low-density neutrophils (which are thought to have a higher propensity to form NETs), as observed in autoimmune diseases, such as SLE (154). Pancytopenia is a distinctive feature of hemophagocytic lymphohistiocytosis, a severe disorder developing either as a complication of multiple autoimmune diseases or as a standalone disorder (155). Variations in cell volume are thought to correlate with cytoskeletal remodeling and changes in cell function and metabolism (156–159). Mean platelet volume (MPV) is a variable provided in the context of blood count, significantly susceptible to the effects of multiple confounders at the analytical and preanalytical level. Altered MPV has been detected in SLE, RA, large- and small-vessel vasculitides, systemic sclerosis (SSc) and chronic spontaneous urticaria (103, 104, 117, 118, 123, 129, 135, 136, 160). However, disease activity in these settings has been associated with high and low MPV values, preventing a straightforward translation of this laboratory tool into clinical practice (161). Gasparyan et al. (137) and Scherlinger et al. (97) offer a possible syncretistic perspective: high platelet volume might reflect chronic low-grade platelet activation (156), whereas low MPV can be consequent to more severe inflammation, causing extensive shedding of platelet microparticles (32). Neutrophils showing increased cellular volume and consequent lower granular density have long been recognized as a hallmark of systemic immune-mediated diseases (159), although they are also detectable in sepsis (162) and cancer (163). This cell population is characterized by enhanced ability to extravasate, extended survival and by a tendency to form NETs (68, 158, 164). By contrast, consistent with the model of inverse association between NETosis and phagocytosis (Figure 1), low density neutrophils are less able to engulf substrates (159).
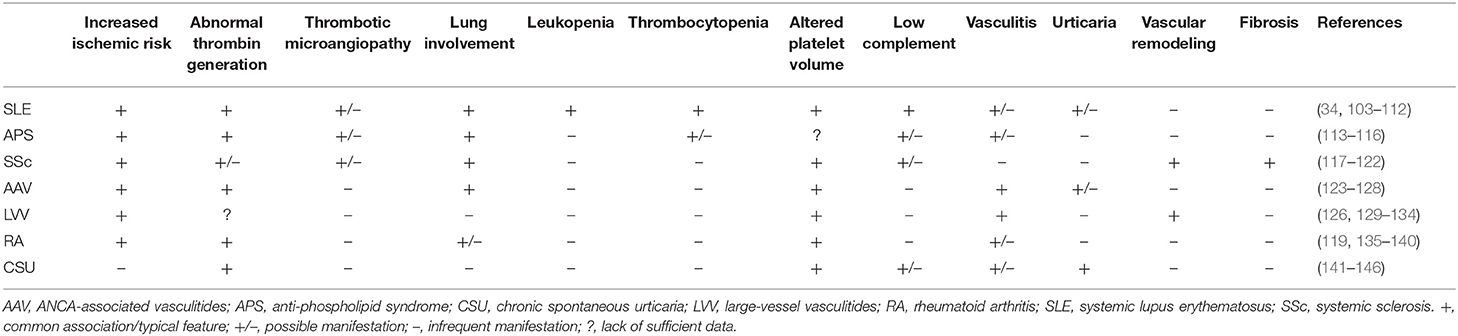
Table 1. Clinical and laboratory features potentially affected by altered platelet-leukocyte interactions in selected immune-mediated diseases.
Patients with inflammatory diseases of the blood vessels (124, 130, 131), connective tissue diseases and chronic arthritides have higher risks of ischemic events (105, 106, 113, 135, 138, 139, 165–167). Accelerated atherosclerosis is a common finding in SLE (7, 106, 168–170), RA (7, 139, 165, 171), or systemic vasculitides (170). Inflammation-related atherosclerotic mechanisms are only partially understood (172). NETs are major determinants of endothelial dysfunction and have been detected in atherosclerotic lesions (173). Platelets are also involved in the early phases of atherosclerosis, where they facilitate leukocyte egress toward the subendothelial vascular layers (174, 175). Antiphospholipid antibodies (aPL) constitute a major independent risk factor in the antiphospholipid syndrome (APS), a condition characterized by arterial or venous thrombosis and/or pregnancy complications occurring as a standalone disorder or secondary to SLE and other autoimmune diseases (176). aPL promote HMGB1-related response in platelets and monocytes (177) and, in cooperation with platelet Toll-like receptor 4, induce NETosis (178), possibly contributing to immunothrombosis (76). In addition, they might impair microparticle scavenging by glycoprotein I, increasing the likelihood of pro-coagulant platelet-neutrophil activation (179). Besides atherosclerotic lesions, NETs have been detected into coronary thrombi (96, 180).
Aberrant coagulation is detectable in immune mediated diseases (34, 107, 108, 119). Altered coagulation cascade and increased cardiovascular risk are common in asthmatic patients (181, 182), while patients with chronic spontaneous urticaria show aberrant thrombin generation but suffer no excess prevalence of cardiovascular disease (125, 141–146).
Thrombotic microangiopathy, consisting in diffuse deposition of thrombi along small vessels due to widespread endothelial activation with hemolysis and platelet consumption, might complicate SLE, SSc, antiphospholipid syndrome and other immune-mediated diseases (183–185). Under intravascular hemolytic conditions, free hemoglobin favors platelet activation. In turn, activated platelet boost neutrophil activation, possibly further feeding the inflammatory cascade (186). In addition, sera from patients with thrombotic microangiopathies fail to degrade NETs, which, in turn, can trigger thrombosis (76, 187).
The lung is a major inflammatory target (188). Neutrophils and platelets undergo unique pathophysiological interaction with the lung vasculature, a reservoir for neutrophils that can thus easily respond to airborne infectious or sterile inflammatory stimuli (189). Unleashed neutrophil effector functions contribute to acute lung injury/acute respiratory distress syndrome (ARDS) during sepsis or vasculitis (50), to the long-term effects on bronchial tissue of chronic inflammation in cystic fibrosis (190, 191) and to interstitial fibrosis in SSc (40, 192). NET generation has a central role in neutrophilic lung injury (193, 194). The lung vasculature influences platelets since lung microvessels are (a) a site of maturation of platelet precursors from the bone marrow (20); (b) a niche for human and mouse megakaryocyte homing (23, 195); (c) a site of detoxification of platelet mediators, such as serotonin (196). Conversely platelets contribute to persistent vasoconstriction (potentially leading to pulmonary hypertension), interstitial fibrosis (197), and NET generation in acute lung injury (198).
Abnormal Platelet-Neutrophil Interactions in Selected Disease Settings
Systemic Vasculitides
Systemic vasculitides encompass a very large set of immune-mediated diseases characterized by vascular injury and downstream organ ischemia as the core pathophysiological event (199). They can be roughly classified into two main subsets, large- and small-vessel vasculitides, based on (prominent) sites of vascular involvement. From a pathophysiological point of view, vascular remodeling with immune infiltration, disassembly of the physiological extracellular architecture and aberrant proliferation of macrophages, fibroblasts and endothelial cells predominate in large-vessel vasculitides, resulting in stroke-like acute failure of large portions of tissues or entire organ due to vessel occlusion. By contrast, small-vessel vasculitis are characterized by necrosis associated or not to granulomatous inflammation and increased thrombotic risk (200). Altered cross-talk among platelet, neutrophils and microparticles might contribute to vascular injury (201).
Neutrophils cause vascular damage in small-vessel vasculitis, possibly reflected by the accumulation of leukocyte cellular debris in peri-vasculitic lesions (leukocytoclasia). In anti-neutrophil cytoplasmic antibody (ANCA)-associated vasculitides (AAV), neutrophil mediated vascular injury is part of a vicious circle linking exposure of myeloperoxidase (MPO) and/or proteinase-3 from granules to neutrophil surface or into the setting of NETs (202) to the development of ANCA, which in turn activate neutrophils and the vascular endothelium (3, 67). In patients with AAV neutrophil responses couple with platelet activation (126). Consistently, patients with active AAV show increased plasmatic levels of P-selectin (127) and HMGB1 (203, 204). Elevation of HMGB1 has also been detected in other vasculitic settings, such as IgA-vasculitis (formerly Henoch-Schonlein's purpura) and Kawasaki disease (205).
In large vessel-vasculitides, activation of platelets may contribute to promote vascular remodeling through the release of signals, such as VEGF (206). Platelets in large-vessel vasculitides show signs of activation (129, 201), and in giant cell arteritis are significantly increased in number during active disease (147) and form hetero-aggregates with leukocytes, possibly contributing to exacerbate ischemic risk (132, 201). This evidence provides a rationale for the use of aspirin in primary prevention (207) and for the employment of platelets as diagnostic surrogates (126). Results in the literature (208) and reports on a potential cyclooxygenase-independent mechanism for aspirin in giant cell arteritis (209) suggest however that the role of platelets should be interpreted with caution.
Little is known on platelet-leukocyte interactions in large vessel vasculitides, although relative depletion of neutrophil granule content has been reported in giant cell arteritis in association with platelet activation (126, 132). Notably, items of small-vessel (peri)vasculitis have been reported in large series of temporal artery biopsies from patients with giant cell arteritis (210) and might be secondary to stereotyped events resembling those observed in small-vessel vasculitides, possibly targeting the vasa vasorum and entailing aberrant platelet-neutrophil cross-talk.
Systemic Lupus Erythematosus
SLE is a multi-organ autoimmune disease with a wide spectrum of clinical manifestations and pathogenic mechanisms (211). Failure of clearance mechanisms and/or exposure of cell death debris in an inflammatory setting promotes autoimmunity and subsequent tissue damage (212). Hematological manifestations constitute a hallmark of SLE and are detected in >80% of patients (213). Cytopenia is the most frequent modality of presentation and affects either red blood cells, platelets and leukocytes. Bone marrow abnormalities are frequent, although no clear correlation can be established with disease activity (214–217). Accordingly, primary bone marrow failure is a rare cause of cytopenia (218), with most relevant mechanisms (besides drugs) being inflammation-induced iron deficiency and cytolysis. Neutropenia occurs in up to one third of SLE cases, in most cases due to antibodies (219), which is not apparently associated to infectious risk (109). Thrombocytopenia is also common in patients with SLE (220). Megakaryocyte number is generally increased during disease activity, reflecting extensive platelet production (214, 220). Patients with SLE and thrombocytopenia have an increased risk of a severe disease course and of mortality in large cohort studies (221).
Cardiovascular manifestations are frequent in patients with SLE and a cause of morbidity and mortality (222). Accelerated atherosclerosis, aPL and dysfunctional coagulation likely converge to determine this risk (106). Despite low absolute platelet counts, patients with SLE frequently show extensive platelet activation (223–227). Higher levels of P-selectin are detectable in urines from patients with lupus nephritis (228). Platelets also contribute to mesangial remodeling and renal vascular damage (229, 230).
Endothelial derived microparticles constitute the most abundant microparticle subset in patients with SLE and correlate with endothelial dysfunction and interferon-α signature (231, 232). However, PDμP also accumulate during active SLE (233, 234) and might impact on inflammation and hemostasis (34, 234). PDμP facilitate coagulation by providing phosphatidylserine scaffolds and intravascularly expressed TF. In addition, they promote neutrophil activation and NET generation being reservoirs of HMGB1 (96) and CD40L (234). Finally PDμP synergise with NETs as inducers of anti-nuclear immunity by constituting a source of mitochondria, which behave as potent damage-associated molecular patterns due to their bacterial origin (235).
Mechanistically, platelet activation in SLE might depend on circulating immunocomplexes, which are abundant in SLE patients biological fluids and are recognized on platelet surface by FcγRIIA and Toll-like receptor 4,7 (236). PDμP themselves could take part in immunocomplexes, enforcing an inflammatory/immunogenic self-sustaining loop (235). Ensuing complement activation in turn amplifies and propagates neutrophil and platelet activation (102, 231, 234).
Systemic Sclerosis
SSc is a systemic autoimmune disease, characterized by unrelenting inflammation with a wound repair response consisting in mesenchymal extracellular matrix deposition leading to fibrosis, and by microvascular dysfunction and aberrant neoangiogenesis (120, 237, 238). Platelets and aberrant platelet-neutrophil interactions play a role in SSc (239). Possibly in response to microvascular damage, platelets of patients with SSc are constitutively activated and express signals driving neutrophil interaction (240, 241). P-selectin dependent cell-cell interactions seem to be relatively less represented in SSc, due to the lower leukocyte expression of PSGL-1 (242). Neutrophils have a pericellular distribution of granules and of their content, causing enhanced degradation of fibrinogen by exposed neutrophil proteases and eventually impairing fibrinogen dependent interactions between neutrophil CD11b/CD18 (also known as Mac-1 or αMβ2 integrin) and platelet glycoprotein IIbIIIa (40, 96). Indeed, platelet-neutrophil heterotypic aggregates are less frequently detected in SSc compared to other inflammatory conditions (40, 96, 242).
Activated platelets in SSc contribute to impaired vascular tone [due to altered arachidonic acid metabolism (197, 239)] and to fibrosis. In fact, platelets release multiple fibrogenic mediators, such as transforming growth factor beta, platelet-derived growth factor, CXCL4 (also known as platelet factor 4), beta-thromboglobulin, serotonin and HMGB1 (27, 97, 242–244). NETs promote fibroblast differentiation and function and might synergise with platelet in supporting fibrosis (192), also in light of the abundance of NET byproducts in the blood of patients with SSc (40). Synergistic NET/platelet-induced fibrosis is expected to be particularly significant in lung tissue, where neutrophil and platelets are abundant (189). Consistently, PDμP (retrieved from the plasma of patients with SSc) induce neutrophil granule mobilization and autophagy, culminating in extended neutrophil survival and generation of NETs through a HMGB1-dependent mechanism. Furthermore, neutrophils stimulated by SSc platelet microparticles migrate in murine lungs, associate with interstitial endothelial damage and promote lung fibrosis (40).
Rheumatoid Arthritis
Rheumatoid arthritis is a relatively frequent autoimmune disease characterized by prominent involvement of the synovial joints. Although extra-skeletal manifestations are relatively less frequent compared to other immune-mediated diseases, patients with RA show an increased ischemic risk, pointing to the existence of a core pathophysiological event linking inflammatory manifestations to vascular dysfunction (245).
Neutrophils undergoing NET generation might provide autoantigens in RA. Patients in fact frequently develop antibodies against citrullinated peptides (ACPA). Citrullination occurs thanks to the activity of deiminating enzymes, such as protein-arginine deiminase 4 (PAD4), abundantly expressed in neutrophils (246). Citrullinated histones constitute a fundamental component of chromatin threads within NETs (73, 247). Platelets might also contribute to ACPA formation due to their expression of vimentin, a preferential target of citrullination (248). ACPA sustain joint inflammation by perpetuating macrophage activation within the synovia, eventually causing chronically elevated levels of tumor necrosis factor alpha (TNFα), which in turn promotes synovial proliferation, bone reabsorption, neoangiogenesis and enhanced synovial infiltration through activated endothelium (249). Enhanced expression of TF on activated platelets and neutrophils, which coexist in the synovial fluid of inflamed joints in patients with RA, provides an interesting hint on potential mechanisms involved in RA-associated enhanced ischemic risk. Platelets and leukocytes from patients with RA are activated due increased plasmatic concentration of TNFα (140). Indeed, platelet respond to TNFα thanks to the expression of TNF receptors 1 and 2 (250) resulting in increased P-selectin expression, platelet degranulation, phosphatidylserine up-regulation and TF expression. TNFα-activated platelets prompt thrombin generation and activation of leukocytes due to P-selectin. Consistently, platelet and leukocyte activation is reduced in patients treated with anti-TNF agents (140), who also face relatively lower rates of cardiovascular events in the long-term (251, 252).
Platelets can be activated by collagen through the megakaryocyte lineage-specific glycoprotein VI and thus prompted to generate microparticles. Boilard and colleagues (253) showed that, following this mechanism, high concentrations of PDμP (possibly shuttled by engaging leukocytes) are detectable in synovial fluid of patients with RA and are required for arthritis development in a murine model. PDμP contain significant amounts of interleukin 1α and β, promoting synoviocyte proliferation, and of IL8, enhancing neutrophil recruitment and ensuring maintenance of inflammation (253).
Conclusion
Platelets and neutrophils are major determinants of the immune-hemostatic continuum and extensively interact based on cell-cell contact and/or exchange of soluble signals and microparticles to synergise in contrasting the noxious effects of endogenous or environmental stimuli toward vessel and tissue integrity and to promote physiological tissue renewal and homeostasis. These events, part of a set of simple, innate, but evolutionarily preserved stereotyped responses, are disproportionately active and self-sustained in patients with immune-mediated diseases, such as systemic vasculitides, SLE, SSc, RA and possibly allergic disorders and might account for the development of either some inflammatory manifestations and of cardiovascular complications. Patients with immune-mediated diseases consistently show signs of platelet (and/or PDμP) activation, possibly prompting either the formation of heterotypic aggregates with neutrophils (as in giant cell arteritis) or neutrophil activation toward enhanced survival and eventually NET generation (as observed in small-vessel vasculitis, SLE, RA and to a higher extent SSc). Diagnostic and therapeutic strategies currently employed in the setting of autoimmune diseases to prevent disease progression and the occurrence of secondary complications are generally not targeted on these pathogenic mechanisms, suggesting the existence of a largely unexplored window of opportunity to improve survival and quality of life for patients by dampening sustained neutrophil-platelet interactions.
Author Contributions
GR and NM collected the literature data, drafted, and revised the manuscript. AM revised the manuscript and provided critical analysis of intellectual content. All authors approved the final version of the manuscript and agree to be accountable for all aspects of the work in ensuring that questions related to the accuracy or integrity of any part of the work will appropriately be investigated and resolved.
Funding
This work of the authors was supported by grants from the Associazione Italiana Ricerca sul Cancro (AIRC IG 2017-2035) and the Ministero della Salute (RF-2013-02358715).
Conflict of Interest
The authors declare that the research was conducted in the absence of any commercial or financial relationships that could be construed as a potential conflict of interest.
References
1. Keragala CB, Draxler DF, McQuilten ZK, Medcalf RL. Haemostasis and innate immunity–a complementary relationship: a review of the intricate relationship between coagulation and complement pathways. Br J Haematol. (2018) 180:782–98. doi: 10.1111/bjh.15062
2. Engelmann B, Massberg S. Thrombosis as an intravascular effector of innate immunity. Nat Rev Immunol. (2013) 13:34–45. doi: 10.1038/nri3345
3. Ramirez GA, Rovere-Querini P, Sabbadini MG, Manfredi AA. Parietal and intravascular innate mechanisms of vascular inflammation. Arthritis Res Ther. (2015) 17:16. doi: 10.1186/s13075-015-0528-2
4. Mason JC, Libby P. Cardiovascular disease in patients with chronic inflammation: mechanisms underlying premature cardiovascular events in rheumatologic conditions. Eur Heart J. (2015) 36:482–9. doi: 10.1093/eurheartj/ehu403
5. Fernandez-Gutierrez B, Perrotti PP, Gisbert JP, Domenech E, Fernandez-Nebro A, Canete JD, et al. Cardiovascular disease in immune-mediated inflammatory diseases: a cross-sectional analysis of 6 cohorts. Medicine (Baltimore). (2017) 96:e7308. doi: 10.1097/MD.0000000000007308
6. Libby P, Nahrendorf M, Swirski FK. Leukocytes link local and systemic inflammation in ischemic cardiovascular disease: an expanded “Cardiovascular Continuum”. J Am Coll Cardiol. (2016) 67:1091–103. doi: 10.1016/j.jacc.2015.12.048
7. Symmons DP, Gabriel SE. Epidemiology of CVD in rheumatic disease, with a focus on RA and SLE. Nat Rev Rheumatol. (2011) 7:399–408. doi: 10.1038/nrrheum.2011.75
8. Manfredi AA, Rovere-Querini P, Bottazzi B, Garlanda C, Mantovani A. Pentraxins, humoral innate immunity and tissue injury. Curr Opin Immunol. (2008) 20:538–44. doi: 10.1016/j.coi.2008.05.004
9. Fischetti F, Tedesco F. Cross-talk between the complement system and endothelial cells in physiologic conditions and in vascular diseases. Autoimmunity. (2006) 39:417–28. doi: 10.1080/08916930600739712
10. de Bont CM, Boelens WC, Pruijn GJM. NETosis, complement, and coagulation: a triangular relationship. Cell Mol Immunol. (2019) 16:19–27. doi: 10.1038/s41423-018-0024-0
11. Cicardi M, Zuraw BL. Angioedema due to bradykinin dysregulation. J Allergy Clin Immunol Pract. (2018) 6:1132–41. doi: 10.1016/j.jaip.2018.04.022
12. Semple JW, Italiano JE Jr, Freedman J. Platelets and the immune continuum. Nat Rev Immunol. (2011) 11:264–74. doi: 10.1038/nri2956
13. Arraud N, Linares R, Tan S, Gounou C, Pasquet JM, Mornet S, et al. Extracellular vesicles from blood plasma: determination of their morphology, size, phenotype and concentration. J Thromb Haemost. (2014) 12:614–27. doi: 10.1111/jth.12554
14. Manfredi AA, Rovere-Querini P, Maugeri N. Dangerous connections: neutrophils and the phagocytic clearance of activated platelets. Curr Opin Hematol. (2010) 17:3–8. doi: 10.1097/MOH.0b013e3283324f97
15. Totani L, Evangelista V. Platelet-leukocyte interactions in cardiovascular disease and beyond. Arterioscler Thromb Vasc Biol. (2010) 30:2357–61. doi: 10.1161/ATVBAHA.110.207480
16. McGregor L, Martin J, McGregor JL. Platelet-leukocyte aggregates and derived microparticles in inflammation, vascular remodelling and thrombosis. Front Biosci. (2006) 11:830–7. doi: 10.2741/1840
17. Burnouf T, Burnouf PA, Wu YW, Chuang EY, Lu LS, Goubran H. Circulatory-cell-mediated nanotherapeutic approaches in disease targeting. Drug Discov Today. (2018) 23:934–43. doi: 10.1016/j.drudis.2017.08.012
18. Baldini M, Manfredi AA, Maugeri N. Targeting platelet-neutrophil interactions in giant-cell arteritis. Curr Pharma Des. (2014) 20:567–74. doi: 10.2174/138161282004140213144840
19. Thon JN, Montalvo A, Patel-Hett S, Devine MT, Richardson JL, Ehrlicher A, et al. Cytoskeletal mechanics of proplatelet maturation and platelet release. J Cell Biol. (2010) 191:861–74. doi: 10.1083/jcb.201006102
20. Machlus KR, Italiano JE Jr. The incredible journey: from megakaryocyte development to platelet formation. J Cell Biol. (2013) 201:785–96. doi: 10.1083/jcb.201304054
21. Kaushansky K. The molecular mechanisms that control thrombopoiesis. J Clin Invest. (2005) 115:3339–47. doi: 10.1172/JCI26674
22. Slavka G, Perkmann T, Haslacher H, Greisenegger S, Marsik C, Wagner OF, et al. Mean platelet volume may represent a predictive parameter for overall vascular mortality and ischemic heart disease. Arterioscler Thromb Vasc Biol. (2011) 31:1215–8. doi: 10.1161/ATVBAHA.110.221788
23. Lefrancais E, Ortiz-Munoz G, Caudrillier A, Mallavia B, Liu F, Sayah DM, et al. The lung is a site of platelet biogenesis and a reservoir for haematopoietic progenitors. Nature. (2017) 544:105–9. doi: 10.1038/nature21706
24. Dejima H, Nakanishi H, Kuroda H, Yoshimura M, Sakakura N, Ueda N, et al. Detection of abundant megakaryocytes in pulmonary artery blood in lung cancer patients using a microfluidic platform. Lung Cancer. (2018) 125:128–35. doi: 10.1016/j.lungcan.2018.09.011
25. Haas S, Hansson J, Klimmeck D, Loeffler D, Velten L, Uckelmann H, et al. Inflammation-Induced emergency megakaryopoiesis driven by hematopoietic stem cell-like megakaryocyte progenitors. Cell Stem Cell. (2015) 17:422–34. doi: 10.1016/j.stem.2015.07.007
26. Sharda A, Flaumenhaft R. The life cycle of platelet granules. F1000Res. (2018) 7:236. doi: 10.12688/f1000research.13283.1
27. Nurden AT. Platelets, inflammation and tissue regeneration. Thromb Haemost. (2011) 105:S13–33. doi: 10.1160/THS10-11-0720
28. Flaumenhaft R. Molecular basis of platelet granule secretion. Arterioscler Thromb Vasc Biol. (2003) 23:1152–60. doi: 10.1161/01.ATV.0000075965.88456.48
29. Scherlinger M, Sisirak V, Richez C, Lazaro E, Duffau P, Blanco P. New insights on platelets and platelet-derived microparticles in systemic lupus erythematosus. Curr Rheumatol Rep. (2017) 19:48. doi: 10.1007/s11926-017-0678-0
30. Pienimaeki-Roemer A, Kuhlmann K, Bottcher A, Konovalova T, Black A, Orso E, et al. Lipidomic and proteomic characterization of platelet extracellular vesicle subfractions from senescent platelets. Transfusion. (2015) 55:507–21. doi: 10.1111/trf.12874
31. Dean WL, Lee MJ, Cummins TD, Schultz DJ, Powell DW. Proteomic and functional characterisation of platelet microparticle size classes. Thromb Haemost. (2009) 102:711–8. doi: 10.1160/TH09-04-243
32. Boilard E, Duchez AC, Brisson A. The diversity of platelet microparticles. Curr Opin Hematol. (2015) 22:437–44. doi: 10.1097/MOH.0000000000000166
33. Key NS, Mackman N. Tissue factor and its measurement in whole blood, plasma, and microparticles. Semin Thromb Hemost. (2010) 36:865–75. doi: 10.1055/s-0030-1267040
34. Pereira J, Alfaro G, Goycoolea M, Quiroga T, Ocqueteau M, Massardo L, et al. Circulating platelet-derived microparticles in systemic lupus erythematosus. Association with increased thrombin generation and procoagulant state. Thromb Haemost. (2006) 95:94–9. doi: 10.1160/TH05-05-0310
35. Varon D, Hayon Y, Dashevsky O, Shai E. Involvement of platelet derived microparticles in tumor metastasis and tissue regeneration. Thromb Res. (2012) 130:S98–9. doi: 10.1016/j.thromres.2012.08.289
36. Brill A, Dashevsky O, Rivo J, Gozal Y, Varon D. Platelet-derived microparticles induce angiogenesis and stimulate post-ischemic revascularization. Cardiovasc Res. (2005) 67:30–8. doi: 10.1016/j.cardiores.2005.04.007
37. Gaetani E, Del Zompo F, Marcantoni M, Gatto I, Giarretta I, Porfidia A, et al. Microparticles produced by activated platelets carry a potent and functionally active angiogenic signal in subjects with Crohn's disease. Int J Mol Sci. (2018) 19:2921. doi: 10.3390/ijms19102921
38. Sung PS, Huang TF, Hsieh SL. Extracellular vesicles from CLEC2-activated platelets enhance dengue virus-induced lethality via CLEC5A/TLR2. Nat Commun. (2019) 10:2402. doi: 10.1038/s41467-019-10360-4
39. Laffont B, Corduan A, Rousseau M, Duchez AC, Lee CH, Boilard E, et al. Platelet microparticles reprogram macrophage gene expression and function. Thromb Haemost. (2016) 115:311–23. doi: 10.1160/th15-05-0389
40. Maugeri N, Capobianco A, Rovere-Querini P, Ramirez GA, Tombetti E, Valle PD, et al. Platelet microparticles sustain autophagy-associated activation of neutrophils in systemic sclerosis. Sci Transl Med. (2018) 10:eaao3089. doi: 10.1126/scitranslmed.aao3089
41. Provost P. The clinical significance of platelet microparticle-associated microRNAs. Clin Chem Lab Med. (2017) 55:657–66. doi: 10.1515/cclm-2016-0895
42. Duchez AC, Boudreau LH, Naika GS, Bollinger J, Belleannee C, Cloutier N, et al. Platelet microparticles are internalized in neutrophils via the concerted activity of 12-lipoxygenase and secreted phospholipase A2-IIA. Proc Natl Acad Sci USA. (2015) 112:E3564–73. doi: 10.1073/pnas.1507905112
43. Cunin P, Nigrovic PA. Megakaryocytes as immune cells. J Leukoc Biol. (2019) 105:1111–21. doi: 10.1002/JLB.MR0718-261RR
44. Jiang J, Kao CY, Papoutsakis ET. How do megakaryocytic microparticles target and deliver cargo to alter the fate of hematopoietic stem cells? J Control Release. (2017) 247:1–18. doi: 10.1016/j.jconrel.2016.12.021
45. Mantovani A, Cassatella MA, Costantini C, Jaillon S. Neutrophils in the activation and regulation of innate and adaptive immunity. Nat Rev Immunol. (2011) 11:519–31. doi: 10.1038/nri3024
46. Maugeri N, Brambilla M, Camera M, Carbone A, Tremoli E, Donati MB, et al. Human polymorphonuclear leukocytes produce and express functional tissue factor upon stimulation. J Thromb Haemost. (2006) 4:1323–30. doi: 10.1111/j.1538-7836.2006.01968.x
47. Kambas K, Chrysanthopoulou A, Vassilopoulos D, Apostolidou E, Skendros P, Girod A, et al. Tissue factor expression in neutrophil extracellular traps and neutrophil derived microparticles in antineutrophil cytoplasmic antibody associated vasculitis may promote thromboinflammation and the thrombophilic state associated with the disease. Ann Rheum Dis. (2014) 73:1854–63. doi: 10.1136/annrheumdis-2013-203430
48. Schett G, Schauer C, Hoffmann M, Herrmann M. Why does the gout attack stop? A roadmap for the immune pathogenesis of gout. RMD Open. (2015) 1:e000046. doi: 10.1136/rmdopen-2015-000046
49. Hu Q, Shi H, Zeng T, Liu H, Su Y, Cheng X, et al. Increased neutrophil extracellular traps activate NLRP3 and inflammatory macrophages in adult-onset Still's disease. Arthritis Res Ther. (2019) 21:9. doi: 10.1186/s13075-018-1800-z
50. Rebetz J, Semple JW, Kapur R. The pathogenic involvement of neutrophils in acute respiratory distress syndrome and transfusion-related acute lung injury. Transf Med Hemother. (2018) 45:290–8. doi: 10.1159/000492950
51. Manfredi AA, Rovere-Querini P, D'Angelo A, Maugeri N. Low molecular weight heparins prevent the induction of autophagy of activated neutrophils and the formation of neutrophil extracellular traps. Pharmacol Res. (2017) 123:146–56. doi: 10.1016/j.phrs.2016.08.008
52. Mitroulis I, Kambas K, Chrysanthopoulou A, Skendros P, Apostolidou E, Kourtzelis I, et al. Neutrophil extracellular trap formation is associated with IL-1beta and autophagy-related signaling in gout. PLoS ONE. (2011) 6:e29318. doi: 10.1371/journal.pone.0029318
53. Pan Q, Gao C, Chen Y, Feng Y, Liu WJ, Liu HF. Update on the role of autophagy in systemic lupus erythematosus: a novel therapeutic target. Biomed Pharmacother. (2015) 71:190–3. doi: 10.1016/j.biopha.2015.02.017
54. Remijsen Q, Vanden Berghe T, Wirawan E, Asselbergh B, Parthoens E, De Rycke R, et al. Neutrophil extracellular trap cell death requires both autophagy and superoxide generation. Cell Res. (2011) 21:290–304. doi: 10.1038/cr.2010.150
55. Tang S, Zhang Y, Yin S, Gao X, Shi W, Wang Y, et al. Neutrophil extracellular trap formation is associated with autophagy-related signaling in ANCA-associated vasculitis. Clin Exp Immunol. (2015) 180:408–18. doi: 10.1111/cei.12589
56. Manz MG, Boettcher S. Emergency granulopoiesis. Nat Rev Immunol. (2014) 14:302–14. doi: 10.1038/nri3660
57. Chavakis T, Mitroulis I, Hajishengallis G. Hematopoietic progenitor cells as integrative hubs for adaptation to and fine-tuning of inflammation. Nat Immunol. (2019) 20:802–11. doi: 10.1038/s41590-019-0402-5
58. Wartha F, Beiter K, Normark S, Henriques-Normark B. Neutrophil extracellular traps: casting the NET over pathogenesis. Curr Opin Microbiol. (2007) 10:52–6. doi: 10.1016/j.mib.2006.12.005
59. Boeltz S, Amini P, Anders HJ, Andrade F, Bilyy R, Chatfield S, et al. To NET or not to NET:current opinions and state of the science regarding the formation of neutrophil extracellular traps. Cell Death Differ. (2019) 26:395–408. doi: 10.1038/s41418-018-0261-x
60. Yipp BG, Kubes P. NETosis: how vital is it? Blood. (2013) 122:2784–94. doi: 10.1182/blood-2013-04-457671
61. Zhao W, Fogg DK, Kaplan MJ. A novel image-based quantitative method for the characterization of NETosis. J Immunol Methods. (2015) 423:104–10. doi: 10.1016/j.jim.2015.04.027
62. Maugeri N, Rovere-Querini P, Manfredi AA. Disruption of a regulatory network consisting of neutrophils and platelets fosters persisting inflammation in rheumatic diseases. Front Immunol. (2016) 7:182. doi: 10.3389/fimmu.2016.00182
63. Metzler KD, Fuchs TA, Nauseef WM, Reumaux D, Roesler J, Schulze I, et al. Myeloperoxidase is required for neutrophil extracellular trap formation: implications for innate immunity. Blood. (2011) 117:953–9. doi: 10.1182/blood-2010-06-290171
64. Ramirez GA, Manfredi AA, Rovere-Querini P, Maugeri N. Bet on NETs! Or on how to translate basic science into clinical practice. Front Immunol. (2016) 7:417. doi: 10.3389/fimmu.2016.00417
65. Podolska MJ, Mahajan A, Knopf J, Hahn J, Boeltz S, Munoz L, et al. Autoimmune, rheumatic, chronic inflammatory diseases: neutrophil extracellular traps on parade. Autoimmunity. (2018) 51:281–7. doi: 10.1080/08916934.2018.1519804
66. Manfredi AA, Ramirez GA, Rovere-Querini P, Maugeri N. The neutrophil's choice: phagocytose vs make neutrophil extracellular traps. Front Immunol. (2018) 9:288. doi: 10.3389/fimmu.2018.00288
67. Sangaletti S, Tripodo C, Chiodoni C, Guarnotta C, Cappetti B, Casalini P, et al. Neutrophil extracellular traps mediate transfer of cytoplasmic neutrophil antigens to myeloid dendritic cells toward ANCA induction and associated autoimmunity. Blood. (2012) 120:3007–18. doi: 10.1182/blood-2012-03-416156
68. Villanueva E, Yalavarthi S, Berthier CC, Hodgin JB, Khandpur R, Lin AM, et al. Netting neutrophils induce endothelial damage, infiltrate tissues, and expose immunostimulatory molecules in systemic lupus erythematosus. J Immunol. (2011) 187:538–52. doi: 10.4049/jimmunol.1100450
69. Hakkim A, Furnrohr BG, Amann K, Laube B, Abed UA, Brinkmann V, et al. Impairment of neutrophil extracellular trap degradation is associated with lupus nephritis. Proc Natl Acad Sci USA. (2010) 107:9813–8. doi: 10.1073/pnas.0909927107
70. Chapman EA, Lyon M, Simpson D, Mason D, Beynon RJ, Moots RJ, et al. Caught in a trap? Proteomic analysis of neutrophil extracellular traps in rheumatoid arthritis and systemic lupus erythematosus. Front Immunol. (2019) 10:423. doi: 10.3389/fimmu.2019.00423
71. Bruschi M, Bonanni A, Petretto A, Vaglio A, Pratesi F, Santucci L, et al. Neutrophil extracellular traps (NETs) profiles in patients with incident SLE and lupus nephritis. J Rheumatol. (2019). doi: 10.3899/jrheum.181232. [Epub ahead of print].
72. Pratesi F, Dioni I, Tommasi C, Alcaro MC, Paolini I, Barbetti F, et al. Antibodies from patients with rheumatoid arthritis target citrullinated histone 4 contained in neutrophils extracellular traps. Ann Rheum Dis. (2014) 73:1414–22. doi: 10.1136/annrheumdis-2012-202765
73. Sur Chowdhury C, Giaglis S, Walker UA, Buser A, Hahn S, Hasler P. Enhanced neutrophil extracellular trap generation in rheumatoid arthritis: analysis of underlying signal transduction pathways and potential diagnostic utility. Arthritis Res Ther. (2014) 16:R122. doi: 10.1186/ar4579
74. Wright HL, Moots RJ, Edwards SW. The multifactorial role of neutrophils in rheumatoid arthritis. Nat Rev Rheumatol. (2014) 10:593–601. doi: 10.1038/nrrheum.2014.80
75. Nakazawa D, Masuda S, Tomaru U, Ishizu A. Pathogenesis and therapeutic interventions for ANCA-associated vasculitis. Nat Rev Rheumatol. (2019) 15:91–101. doi: 10.1038/s41584-018-0145-y
76. Jimenez-Alcazar M, Rangaswamy C, Panda R, Bitterling J, Simsek YJ, Long AT, et al. Host DNases prevent vascular occlusion by neutrophil extracellular traps. Science. (2017) 358:1202–6. doi: 10.1126/science.aam8897
77. Jaillon S, Peri G, Delneste Y, Fremaux I, Doni A, Moalli F, et al. The humoral pattern recognition receptor PTX3 is stored in neutrophil granules and localizes in extracellular traps. J Exp Med. (2007) 204:793–804. doi: 10.1084/jem.20061301
78. Jaillon S, Ponzetta A, Magrini E, Barajon I, Barbagallo M, Garlanda C, et al. Fluid phase recognition molecules in neutrophil-dependent immune responses. Semin Immunol. (2016) 28:109–18. doi: 10.1016/j.smim.2016.03.005
79. Polgar J, Matuskova J, Wagner DD. The P-selectin, tissue factor, coagulation triad. J Thromb Haemost. (2005) 3:1590–6. doi: 10.1111/j.1538-7836.2005.01373.x
80. Moraes JA, Frony AC, Barcellos-de-Souza P, Menezes da Cunha M, Brasil Barbosa Calcia T, Benjamim CF, et al. Downregulation of microparticle release and pro-inflammatory properties of activated human polymorphonuclear neutrophils by LMW fucoidan. J Innate Immun. (2019) 11:330–46. doi: 10.1159/000494220
81. Mesri M, Altieri DC. Endothelial cell activation by leukocyte microparticles. J Immunol. (1998) 161:4382–7.
82. Butin-Israeli V, Bui TM, Wiesolek HL, Mascarenhas L, Lee JJ, Mehl LC, et al. Neutrophil-induced genomic instability impedes resolution of inflammation and wound healing. J Clin Invest. (2019) 129:712–26. doi: 10.1172/JCI122085
83. Sellam J, Proulle V, Jungel A, Ittah M, Miceli Richard C, Gottenberg JE, et al. Increased levels of circulating microparticles in primary Sjogren's syndrome, systemic lupus erythematosus and rheumatoid arthritis and relation with disease activity. Arthritis Res Ther. (2009) 11:R156. doi: 10.1186/ar2833
84. Wang Y, Luo L, Braun OO, Westman J, Madhi R, Herwald H, et al. Neutrophil extracellular trap-microparticle complexes enhance thrombin generation via the intrinsic pathway of coagulation in mice. Sci Rep. (2018) 8:4020. doi: 10.1038/s41598-018-22156-5
85. Maugeri N, Rovere-Querini P, Evangelista V, Covino C, Capobianco A, Bertilaccio MT, et al. Neutrophils phagocytose activated platelets in vivo: a phosphatidylserine, P-selectin, and {beta}2 integrin-dependent cell clearance program. Blood. (2009) 113:5254–65. doi: 10.1182/blood-2008-09-180794
86. Maugeri N, Evangelista V, Celardo A, Dell'Elba G, Martelli N, Piccardoni P, et al. Polymorphonuclear leukocyte-platelet interaction: role of P-selectin in thromboxane B2 and leukotriene C4 cooperative synthesis. Thromb Haemost. (1994) 72:450–6. doi: 10.1055/s-0038-1648888
87. Page C, Pitchford S. Neutrophil and platelet complexes and their relevance to neutrophil recruitment and activation. Int Immunopharmacol. (2013) 17:1176–84. doi: 10.1016/j.intimp.2013.06.004
88. Clark SR, Ma AC, Tavener SA, McDonald B, Goodarzi Z, Kelly MM, et al. Platelet TLR4 activates neutrophil extracellular traps to ensnare bacteria in septic blood. Nat Med. (2007) 13:463–9. doi: 10.1038/nm1565
89. Brown SB, Clarke MC, Magowan L, Sanderson H, Savill J. Constitutive death of platelets leading to scavenger receptor-mediated phagocytosis. A caspase-independent cell clearance program. J Biol Chem. (2000) 275:5987–96. doi: 10.1074/jbc.275.8.5987
90. Kornerup KN, Salmon GP, Pitchford SC, Liu WL, Page CP. Circulating platelet-neutrophil complexes are important for subsequent neutrophil activation and migration. J Appl Physiol (1985). (2010) 109:758–67. doi: 10.1152/japplphysiol.01086.2009
91. Han CZ, Ravichandran KS. Metabolic connections during apoptotic cell engulfment. Cell. (2011) 147:1442–5. doi: 10.1016/j.cell.2011.12.006
92. Zhang S, Bories G, Lantz C, Emmons R, Becker A, Liu E, et al. Immunometabolism of phagocytes and relationships to cardiac repair. Front Cardiovasc Med. (2019) 6:42. doi: 10.3389/fcvm.2019.00042
93. Cunin P, Bouslama R, Machlus KR, Martinez-Bonet M, Lee PY, Wactor A, et al. Megakaryocyte emperipolesis mediates membrane transfer from intracytoplasmic neutrophils to platelets. Elife. (2019) 8:e44031. doi: 10.7554/eLife.44031
94. Kuravi SJ, Harrison P, Rainger GE, Nash GB. Ability of platelet-derived extracellular vesicles to promote neutrophil-endothelial cell interactions. Inflammation. (2019) 42:290–305. doi: 10.1007/s10753-018-0893-5
95. Jy W, Mao WW, Horstman L, Tao J, Ahn YS. Platelet microparticles bind, activate and aggregate neutrophils in vitro. Blood Cells Mol Dis. (1995) 21:217–31; discussion 31a. doi: 10.1006/bcmd.1995.0025
96. Maugeri N, Campana L, Gavina M, Covino C, De Metrio M, Panciroli C, et al. Activated platelets present high mobility group box 1 to neutrophils, inducing autophagy and promoting the extrusion of neutrophil extracellular traps. J Thromb Haemost. (2014) 12:2074–88. doi: 10.1111/jth.12710
97. Scherlinger M, Guillotin V, Truchetet ME, Contin-Bordes C, Sisirak V, Duffau P, et al. Systemic lupus erythematosus and systemic sclerosis: all roads lead to platelets. Autoimmun Rev. (2018) 17:625–35. doi: 10.1016/j.autrev.2018.01.012
98. Zhou H, Deng M, Liu Y, Yang C, Hoffman R, Zhou J, et al. Platelet HMGB1 is required for efficient bacterial clearance in intra-abdominal bacterial sepsis in mice. Blood Adv. (2018) 2:638–48. doi: 10.1182/bloodadvances.2017011817
99. Kim SW, Lee H, Lee HK, Kim ID, Lee JK. Neutrophil extracellular trap induced by HMGB1 exacerbates damages in the ischemic brain. Acta Neuropathol Commun. (2019) 7:94. doi: 10.1186/s40478-019-0747-x
100. Rossaint J, Kuhne K, Skupski J, Van Aken H, Looney MR, Hidalgo A, et al. Directed transport of neutrophil-derived extracellular vesicles enables platelet-mediated innate immune response. Nat Commun. (2016) 7:13464. doi: 10.1038/ncomms13464
101. Maugeri N, Evangelista V, Piccardoni P, Dell'Elba G, Celardo A, de Gaetano G, et al. Transcellular metabolism of arachidonic acid: increased platelet thromboxane generation in the presence of activated polymorphonuclear leukocytes. Blood. (1992) 80:447–51. doi: 10.1016/0049-3848(92)90486-T
102. Eriksson O, Mohlin C, Nilsson B, Ekdahl KN. The human platelet as an innate immune cell: interactions between activated platelets and the complement system. Front Immunol. (2019) 10:1590. doi: 10.3389/fimmu.2019.01590
103. Bai M, Xing L, Feng J, Cui C, Huang L, Liang G. Mean platelet volume could reflect disease activity of adult patients with systemic lupus erythematosus. Clin Lab. (2016) 62:1317–22. doi: 10.7754/Clin.Lab.2015.151134
104. Yavuz S, Ece A. Mean platelet volume as an indicator of disease activity in juvenile SLE. Clin Rheumatol. (2014) 33:637–41. doi: 10.1007/s10067-014-2540-3
105. Fernandez-Nebro A, Rua-Figueroa I, Lopez-Longo FJ, Galindo-Izquierdo M, Calvo-Alen J, Olive-Marques A, et al. Cardiovascular events in systemic lupus erythematosus: a nationwide study in Spain from the RELESSER registry. Medicine (Baltimore). (2015) 94:e1183. doi: 10.1097/MD.0000000000001183
106. Ramirez GA, Efthymiou M, Isenberg DA, Cohen H. Under crossfire: thromboembolic risk in systemic lupus erythematosus. Rheumatology (Oxf). (2019) 58:940–52. doi: 10.1093/rheumatology/key307
107. Mehta BM, Kiani AN, Chen C, Jani J, Kickler TS, Petri M. Endogenous thrombin potential in the assessment of hypercoagulability in systemic lupus erythematosus. Am J Hematol. (2010) 85:83–5. doi: 10.1002/ajh.21566
108. Kern A, Barabas E, Balog A, Burcsar S, Kiszelak M, Vasarhelyi B. Characterization of the thrombin generation profile in systemic lupus erythematosus. Physiol Int. (2017) 104:35–41. doi: 10.1556/2060.104.2017.1.5
109. Carli L, Tani C, Vagnani S, Signorini V, Mosca M. Leukopenia, lymphopenia, and neutropenia in systemic lupus erythematosus: prevalence and clinical impact–a systematic literature review. Semin Arthritis Rheum. (2015) 45:190–4. doi: 10.1016/j.semarthrit.2015.05.009
110. Ferriani MP, Silva MF, Pereira RM, Terreri MT, Saad Magalhaes C, Bonfa E, et al. Chronic spontaneous urticaria: a survey of 852 cases of childhood-onset systemic lupus erythematosus. Int Arch Allergy Immunol. (2015) 167:186–92. doi: 10.1159/000438723
111. Kolkhir P, Pogorelov D, Olisova O, Maurer M. Comorbidity and pathogenic links of chronic spontaneous urticaria and systemic lupus erythematosus–a systematic review. Clin Exp Allergy. (2016) 46:275–87. doi: 10.1111/cea.12673
112. Lood C, Tyden H, Gullstrand B, Nielsen CT, Heegaard NH, Linge P, et al. Decreased platelet size is associated with platelet activation and anti-phospholipid syndrome in systemic lupus erythematosus. Rheumatology. (2017) 56:408–16. doi: 10.1093/rheumatology/kex216
113. Ruiz-Irastorza G, Crowther M, Branch W, Khamashta MA. Antiphospholipid syndrome. Lancet. (2010) 376:1498–509. doi: 10.1016/S0140-6736(10)60709-X
114. Cervera R, Serrano R, Pons-Estel GJ, Ceberio-Hualde L, Shoenfeld Y, de Ramon E, et al. Morbidity and mortality in the antiphospholipid syndrome during a 10-year period: a multicentre prospective study of 1000 patients. Ann Rheum Dis. (2015) 74:1011–8. doi: 10.1136/annrheumdis-2013-204838
115. Arachchillage DR, Efthymiou M, Mackie IJ, Lawrie AS, Machin SJ, Cohen H. Anti-protein C antibodies are associated with resistance to endogenous protein C activation and a severe thrombotic phenotype in antiphospholipid syndrome. J Thromb Haemost. (2014) 12:1801–9. doi: 10.1111/jth.12722
116. Pierangeli SS, Girardi G, Vega-Ostertag M, Liu X, Espinola RG, Salmon J. Requirement of activation of complement C3 and C5 for antiphospholipid antibody-mediated thrombophilia. Arthritis Rheum. (2005) 52:2120–4. doi: 10.1002/art.21157
117. Soydinc S, Turkbeyler IH, Pehlivan Y, Soylu G, Goktepe MF, Bilici M, et al. Mean platelet volume seems to be a valuable marker in patients with systemic sclerosis. Inflammation. (2014) 37:100–6. doi: 10.1007/s10753-013-9716-x
118. Maeda M, Kachi H, Mori S. Ultrastructural observation of platelets from patients with progressive systemic sclerosis (PSS). J Dermatol. (1998) 25:222–30. doi: 10.1111/j.1346-8138.1998.tb02385.x
119. Solfietti L, Binello GB, Stella S, Bazzan M, Salierno M, Roccatello D. Thrombin generation assay: interactions between chronic inflammation and haemostasis in patients with autoimmune diseases. Clin Exp Rheumatol. (2016) 34:925–8.
120. Nicolosi PA, Tombetti E, Maugeri N, Rovere-Querini P, Brunelli S, Manfredi AA. Vascular remodelling and mesenchymal transition in systemic sclerosis. Stem Cells Int. (2016) 2016:4636859. doi: 10.1155/2016/4636859
121. Denton CP, Khanna D. Systemic sclerosis. Lancet. (2017) 390:1685–99. doi: 10.1016/S0140-6736(17)30933-9
122. Ames PR, Lupoli S, Alves J, Atsumi T, Edwards C, Iannaccone L, et al. The coagulation/fibrinolysis balance in systemic sclerosis: evidence for a haematological stress syndrome. Br J Rheumatol. (1997) 36:1045–50. doi: 10.1093/rheumatology/36.10.1045
123. Kim HJ, Jung SM, Song JJ, Park YB, Lee SW. Mean platelet volume can estimate the current vasculitis activity of microscopic polyangiitis. Rheumatol Int. (2018) 38:1095–101. doi: 10.1007/s00296-018-4011-7
124. Merkel PA, Lo GH, Holbrook JT, Tibbs AK, Allen NB, Davis JC Jr, et al. Brief communication: high incidence of venous thrombotic events among patients with Wegener granulomatosis: the Wegener's Clinical Occurrence of Thrombosis (WeCLOT) Study. Ann Intern Med. (2005) 142:620–6. doi: 10.7326/0003-4819-142-8-200505030-00011
125. Maino A, Rossio R, Cugno M, Marzano AV, Tedeschi A. Hypereosinophilic syndrome, Churg-Strauss syndrome and parasitic diseases: possible links between eosinophilia and thrombosis. Curr Vasc Pharmacol. (2012) 10:670–5. doi: 10.2174/157016112801784594
126. Maugeri N, Rovere-Querini P, Evangelista V, Godino C, Demetrio M, Baldini M, et al. An intense and short-lasting burst of neutrophil activation differentiates early acute myocardial infarction from systemic inflammatory syndromes. PLoS ONE. (2012) 7:e39484. doi: 10.1371/journal.pone.0039484
127. Tomasson G, Lavalley M, Tanriverdi K, Finkielman JD, Davis JC Jr, Hoffman GS, et al. Relationship between markers of platelet activation and inflammation with disease activity in Wegener's granulomatosis. J Rheumatol. (2011) 38:1048–54. doi: 10.3899/jrheum.100735
128. Ma TT, Huang YM, Wang C, Zhao MH, Chen M. Coagulation and fibrinolysis index profile in patients with ANCA-associated vasculitis. PLoS ONE. (2014) 9:e97843. doi: 10.1371/journal.pone.0097843
129. Peng YF, Guo J, Deng YB. The role of mean platelet volume in patients with Takayasu arteritis. Ann Clin Biochem. (2017) 54:273–8. doi: 10.1177/0004563216658312
130. Weyand CM, Goronzy JJ. Medium- and large-vessel vasculitis. N Engl J Med. (2003) 349:160–9. doi: 10.1056/NEJMra022694
131. Tombetti E, Mason JC. Takayasu arteritis: recent advances and hopes for the future. Rheumatology. (2019) 17:238–47. doi: 10.1111/1756-185X.12309
132. Maugeri N, Baldini M, Rovere-Querini P, Maseri A, Sabbadini MG, Manfredi AA. Leukocyte and platelet activation in patients with giant cell arteritis and polymyalgia rheumatica: a clue to thromboembolic risks? Autoimmunity. (2009) 42:386–8. doi: 10.1080/08916930902832629
133. Akkececi NS, Cetin GY, Gogebakan H, Acipayam C. The C-reactive protein/albumin ratio and complete blood count parameters as indicators of disease activity in patients with Takayasu Arteritis. Med Sci Monit. (2019) 25:1401. doi: 10.12659/MSM.912495
134. Kaiser M, Weyand CM, Bjornsson J, Goronzy JJ. Platelet-derived growth factor, intimal hyperplasia, and ischemic complications in giant cell arteritis. Arthritis Rheum. (1998) 41:623–33.
135. Maden M, Pamuk GE, Pamuk ON. Development of atherosclerotic cardiovascular mortality in gouty arthritis and rheumatoid arthritis patients: are they associated with mean platelet volume and neutrophil-lymphocyte ratio? A comparative study. Arch Rheumatol. (2017) 32:39–45. doi: 10.5606/ArchRheumatol.2017.6033
136. Yazici S, Yazici M, Erer B, Erer B, Calik Y, Ozhan H, et al. The platelet indices in patients with rheumatoid arthritis: mean platelet volume reflects disease activity. Platelets. (2010) 21:122–5. doi: 10.3109/09537100903474373
137. Gasparyan AY, Ayvazyan L, Mikhailidis DP, Kitas GD. Mean platelet volume: a link between thrombosis and inflammation? Curr Pharma Des. (2011) 17:47–58. doi: 10.2174/138161211795049804
138. Gasparyan AY, Stavropoulos-Kalinoglou A, Mikhailidis DP, Douglas KM, Kitas GD. Platelet function in rheumatoid arthritis: arthritic and cardiovascular implications. Rheumatol Int. (2011) 31:153–64. doi: 10.1007/s00296-010-1446-x
139. Lauper K, Gabay C. Cardiovascular risk in patients with rheumatoid arthritis. Semin Immunopathol. (2017) 39:447–59. doi: 10.1007/s00281-017-0632-2
140. Manfredi AA, Baldini M, Camera M, Baldissera E, Brambilla M, Peretti G, et al. Anti-TNFalpha agents curb platelet activation in patients with rheumatoid arthritis. Ann Rheum Dis. (2016) 75:1511–20. doi: 10.1136/annrheumdis-2015-208442
141. Chandrashekar L, Rajappa M, Sundar I, Munisamy M, Ananthanarayanan PH, Thappa DM, et al. Platelet activation in chronic urticaria and its correlation with disease severity. Platelets. (2014) 25:162–5. doi: 10.3109/09537104.2013.786822
142. Sakurai Y, Morioke S, Takeda T, Takahagi S, Hide M, Shima M. Increased thrombin generation potential in patients with chronic spontaneous urticaria. Allergol Int. (2015) 64:96–8. doi: 10.1016/j.alit.2014.07.006
143. Takeda T, Sakurai Y, Takahagi S, Kato J, Yoshida K, Yoshioka A, et al. Increase of coagulation potential in chronic spontaneous urticaria. Allergy. (2011) 66:428–33. doi: 10.1111/j.1398-9995.2010.02506.x
144. Tedeschi A, Kolkhir P, Asero R, Pogorelov D, Olisova O, Kochergin N, et al. Chronic urticaria and coagulation: pathophysiological and clinical aspects. Allergy. (2014) 69:683–91. doi: 10.1111/all.12389
145. Yanase Y, Takahagi S, Hide M. Chronic spontaneous urticaria and the extrinsic coagulation system. Allergol Int. (2018) 67:191–4. doi: 10.1016/j.alit.2017.09.003
146. Egeberg A, Kofoed K, Gislason GH, Vestergaard C, Thyssen JP. Cardiovascular risk is not Increased in patients with chronic urticaria: a retrospective population-based cohort study. Acta Derm Venereol. (2017) 97:261–2. doi: 10.2340/00015555-2516
147. Chan FLY, Lester S, Whittle SL, Hill CL. The utility of ESR, CRP and platelets in the diagnosis of GCA. BMC Rheumatol. (2019) 3:14. doi: 10.1186/s41927-019-0061-z
148. Claushuis TA, van Vught LA, Scicluna BP, Wiewel MA, Klein Klouwenberg PM, Hoogendijk AJ, et al. Thrombocytopenia is associated with a dysregulated host response in critically ill sepsis patients. Blood. (2016) 127:3062–72. doi: 10.1182/blood-2015-11-680744
149. Hillgruber C, Poppelmann B, Weishaupt C, Steingraber AK, Wessel F, Berdel WE, et al. Blocking neutrophil diapedesis prevents hemorrhage during thrombocytopenia. J Exp Med. (2015) 212:1255–66. doi: 10.1084/jem.20142076
150. Alzubiedi S, Saleh MI. Predictors of severe thrombocytopenia secondary to peginterferon Alfa-2a treatment in subjects with hepatitis C virus infection. Am J Ther. (2017) 24:e670–5. doi: 10.1097/MJT.0000000000000356
151. Gollomp K, Kim M, Johnston I, Hayes V, Welsh J, Arepally GM, et al. Neutrophil accumulation and NET release contribute to thrombosis in HIT. JCI Insight. (2018) 3:99445. doi: 10.1172/jci.insight.99445
152. Leffler J, Prohaszka Z, Mikes B, Sinkovits G, Ciacma K, Farkas P, et al. Decreased neutrophil extracellular trap degradation in Shiga toxin-associated haemolytic uraemic syndrome. J Innate Immun. (2017) 9:12–21. doi: 10.1159/000450609
153. Perdomo J, Leung HHL, Ahmadi Z, Yan F, Chong JJH, Passam FH, et al. Neutrophil activation and NETosis are the major drivers of thrombosis in heparin-induced thrombocytopenia. Nat Commun. (2019) 10:1322. doi: 10.1038/s41467-019-09160-7
154. Li Y, Wang H, Peng C, Li H, Jie S. Low-density neutrophils in severe fever with thrombocytopenia syndrome (SFTS) display decreased function to phagocytose SFTS virus and enhanced capacity to synthesize cytokines. Int J Clin Exp Pathol. (2017) 4:4069–78.
155. Esteban YM, de Jong JLO, Tesher MS. An overview of hemophagocytic lymphohistiocytosis. Pediatr Ann. (2017) 46:e309–13. doi: 10.3928/19382359-20170717-01
156. Thompson CB, Eaton KA, Princiotta SM, Rushin CA, Valeri CR. Size dependent platelet subpopulations: relationship of platelet volume to ultrastructure, enzymatic activity, and function. Br J Haematol. (1982) 50:509–19. doi: 10.1111/j.1365-2141.1982.tb01947.x
157. Thompson CB, Scher I, Schaefer ME, Lindsten T, Finkelman FD, Mond JJ. Size-dependent B lymphocyte subpopulations: relationship of cell volume to surface phenotype, cell cycle, proliferative response, and requirements for antibody production to TNP-Ficoll and TNP-BA. J Immunol. (1984) 133:2333–42.
158. Denny MF, Yalavarthi S, Zhao W, Thacker SG, Anderson M, Sandy AR, et al. A distinct subset of proinflammatory neutrophils isolated from patients with systemic lupus erythematosus induces vascular damage and synthesizes type I IFNs. J Immunol. (2010) 184:3284–97. doi: 10.4049/jimmunol.0902199
159. Hacbarth E, Kajdacsy-Balla A. Low density neutrophils in patients with systemic lupus erythematosus, rheumatoid arthritis, and acute rheumatic fever. Arthritis Rheum. (1986) 29:1334–42. doi: 10.1002/art.1780291105
160. Vena GA, Cassano N, Marzano AV, Asero R. The role of platelets in chronic urticaria. Int Arch Allergy Immunol. (2016) 169:71–9. doi: 10.1159/000444085
161. Korniluk A, Koper-Lenkiewicz OM, Kaminska J, Kemona H, Dymicka-Piekarska V. Mean platelet volume (MPV): new perspectives for an old marker in the course and prognosis of inflammatory conditions. Mediat Inflamm. (2019) 2019:9213074. doi: 10.1155/2019/9213074
162. Park DH, Park K, Park J, Park HH, Chae H, Lim J, et al. Screening of sepsis using leukocyte cell population data from the Coulter automatic blood cell analyzer DxH800. Int J Lab Hematol. (2011) 33:391–9. doi: 10.1111/j.1751-553X.2011.01298.x
163. Sagiv JY, Michaeli J, Assi S, Mishalian I, Kisos H, Levy L, et al. Phenotypic diversity and plasticity in circulating neutrophil subpopulations in cancer. Cell Rep. (2015) 10:562–73. doi: 10.1016/j.celrep.2014.12.039
164. Worthen GS, Henson PM, Rosengren S, Downey GP, Hyde DM. Neutrophils increase volume during migration in vivo and in vitro. Am J Respir Cell Mol Biol. (1994) 10:1–7. doi: 10.1165/ajrcmb.10.1.8292373
165. Chung CP, Giles JT, Kronmal RA, Post WS, Gelber AC, Petri M, et al. Progression of coronary artery atherosclerosis in rheumatoid arthritis: comparison with participants from the Multi-Ethnic Study of Atherosclerosis. Arthritis Res Ther. (2013) 15:R134. doi: 10.1186/ar4314
166. Ruiz-Irastorza G, Egurbide MV, Ugalde J, Aguirre C. High impact of antiphospholipid syndrome on irreversible organ damage and survival of patients with systemic lupus erythematosus. Arch Intern Med. (2004) 164:77–82. doi: 10.1001/archinte.164.1.77
167. Palatinus A, Adams M. Thrombosis in systemic lupus erythematosus. Semin Thromb Hemost. (2009) 35:621–9. doi: 10.1055/s-0029-1242716
168. Kay SD, Poulsen MK, Diederichsen AC, Voss A. Coronary, carotid, and lower-extremity atherosclerosis and their interrelationship in danish patients with systemic lupus erythematosus. J Rheumatol. (2016) 43:315–22. doi: 10.3899/jrheum.150488
169. Roman MJ, Crow MK, Lockshin MD, Devereux RB, Paget SA, Sammaritano L, et al. Rate and determinants of progression of atherosclerosis in systemic lupus erythematosus. Arthritis Rheum. (2007) 56:3412–9. doi: 10.1002/art.22924
170. Seyahi E, Ugurlu S, Cumali R, Balci H, Seyahi N, Yurdakul S, et al. Atherosclerosis in Takayasu arteritis. Ann Rheum Dis. (2006) 65:1202–7. doi: 10.1136/ard.2005.047498
171. Salmon JE, Roman MJ. Subclinical atherosclerosis in rheumatoid arthritis and systemic lupus erythematosus. Am J Med. (2008) 121:S3–8. doi: 10.1016/j.amjmed.2008.06.010
172. Bruce IN. ‘Not only … but also’: factors that contribute to accelerated atherosclerosis and premature coronary heart disease in systemic lupus erythematosus. Rheumatology. (2005) 44:1492–502. doi: 10.1093/rheumatology/kei142
173. Qi H, Yang S, Zhang L. Neutrophil extracellular traps and endothelial dysfunction in atherosclerosis and thrombosis. Front Immunol. (2017) 8:928. doi: 10.3389/fimmu.2017.00928
174. Mezger M, Nording H, Sauter R, Graf T, Heim C, von Bubnoff N, et al. Platelets and immune responses during thromboinflammation. Front Immunol. (2019) 10:1731. doi: 10.3389/fimmu.2019.01731
175. Massberg S, Brand K, Gruner S, Page S, Muller E, Muller I, et al. A critical role of platelet adhesion in the initiation of atherosclerotic lesion formation. J Exp Med. (2002) 196:887–96. doi: 10.1084/jem.20012044
176. Bertolaccini ML, Sanna G. Recent advances in understanding antiphospholipid syndrome. F1000Res. (2016) 5:2908. doi: 10.12688/f1000research.9717.1
177. Manganelli V, Truglia S, Capozzi A, Alessandri C, Riitano G, Spinelli FR, et al. Alarmin HMGB1 and soluble RAGE as new tools to evaluate the risk stratification in patients with the antiphospholipid syndrome. Front Immunol. (2019) 10:460. doi: 10.3389/fimmu.2019.00460
178. Yalavarthi S, Gould TJ, Rao AN, Mazza LF, Morris AE, Nunez-Alvarez C, et al. Release of neutrophil extracellular traps by neutrophils stimulated with antiphospholipid antibodies: a newly identified mechanism of thrombosis in the antiphospholipid syndrome. Arthritis Rheumatol. (2015) 67:2990–3003. doi: 10.1002/art.39247
179. de Groot PG, Meijers JC. beta(2)-Glycoprotein I: evolution, structure and function. J Thromb Haemost. (2011) 9:1275–84. doi: 10.1111/j.1538-7836.2011.04327.x
180. Stakos DA, Kambas K, Konstantinidis T, Mitroulis I, Apostolidou E, Arelaki S, et al. Expression of functional tissue factor by neutrophil extracellular traps in culprit artery of acute myocardial infarction. Eur Heart J. (2015) 36:1405–14. doi: 10.1093/eurheartj/ehv007
181. Bazan-Socha S, Mastalerz L, Cybulska A, Zareba L, Kremers R, Zabczyk M, et al. Asthma is associated with enhanced thrombin formation and impaired fibrinolysis. Clin Exp Allergy. (2016) 46:932–44. doi: 10.1111/cea.12734
182. Tattersall MC, Guo M, Korcarz CE, Gepner AD, Kaufman JD, Liu KJ, et al. Asthma predicts cardiovascular disease events: the multi-ethnic study of atherosclerosis. Arterioscler Thromb Vasc Biol. (2015) 35:1520–5. doi: 10.1161/ATVBAHA.115.305452
183. Liszewski MK, Atkinson JP. Too much of a good thing at the site of tissue injury: the instructive example of the complement system predisposing to thrombotic microangiopathy. Hematol Am Soc Hematol Educ Program. (2011) 2011:9–14. doi: 10.1182/asheducation-2011.1.9
184. Ruggenenti P, Noris M, Remuzzi G. Thrombotic microangiopathy, hemolytic uremic syndrome, and thrombotic thrombocytopenic purpura. Kidney Int. (2001) 60:831–46. doi: 10.1046/j.1523-1755.2001.060003831.x
185. Song D, Wu LH, Wang FM, Yang XW, Zhu D, Chen M, et al. The spectrum of renal thrombotic microangiopathy in lupus nephritis. Arthritis Res Ther. (2013) 15:R12. doi: 10.1186/ar4142
186. Bhasym A, Annarapu GK, Saha S, Shrimali N, Gupta S, Seth T, et al. Neutrophils develop rapid proinflammatory response after engulfing Hb-activated platelets under intravascular hemolysis. Clin Exp Immunol. (2019) 197:131–40. doi: 10.1111/cei.13310
187. Jimenez-Alcazar M, Napirei M, Panda R, Kohler EC, Kremer Hovinga JA, Mannherz HG, et al. Impaired DNase1-mediated degradation of neutrophil extracellular traps is associated with acute thrombotic microangiopathies. J Thromb Haemost. (2015) 13:732–42. doi: 10.1111/jth.12796
188. Doyle TJ, Dellaripa PF. Lung manifestations in the rheumatic diseases. Chest. (2017) 152:1283–95. doi: 10.1016/j.chest.2017.05.015
189. Aulakh GK. Neutrophils in the lung: “the first responders”. Cell Tissue Res. (2018) 371:577–88. doi: 10.1007/s00441-017-2748-z
190. Yang C, Chilvers M, Montgomery M, Nolan SJ. Dornase alfa for cystic fibrosis. Cochrane Database Syst Rev. (2016) 4:CD001127. doi: 10.1002/14651858.CD001127.pub3
191. Yoo DG, Winn M, Pang L, Moskowitz SM, Malech HL, Leto TL, et al. Release of cystic fibrosis airway inflammatory markers from Pseudomonas aeruginosa-stimulated human neutrophils involves NADPH oxidase-dependent extracellular DNA trap formation. J Immunol. (2014) 192:4728–38. doi: 10.4049/jimmunol.1301589
192. Chrysanthopoulou A, Mitroulis I, Apostolidou E, Arelaki S, Mikroulis D, Konstantinidis T, et al. Neutrophil extracellular traps promote differentiation and function of fibroblasts. J Pathol. (2014) 233:294–307. doi: 10.1002/path.4359
193. Liu S, Su X, Pan P, Zhang L, Hu Y, Tan H, et al. Neutrophil extracellular traps are indirectly triggered by lipopolysaccharide and contribute to acute lung injury. Sci Rep. (2016) 6:37252. doi: 10.1038/srep37252
194. Gregoire M, Uhel F, Lesouhaitier M, Gacouin A, Guirriec M, Mourcin F, et al. Impaired efferocytosis and neutrophil extracellular trap clearance by macrophages in ARDS. Eur Respir J. (2018) 52:1702590. doi: 10.1183/13993003.02590-2017
195. Kosaki G. In vivo platelet production from mature megakaryocytes: does platelet release occur via proplatelets? Int J Hematol. (2005) 81:208–19. doi: 10.1532/IJH97.04177
196. Yamamoto Y, Hasegawa H, Inoue F, Ikeda K, Ichiyama A. Serotonin in the lung. Demonstration of a close correlation to blood platelet. Agents Actions. (1986) 18:351–8. doi: 10.1007/BF01964996
197. Pattanaik D, Brown M, Postlethwaite BC, Postlethwaite AE. Pathogenesis of systemic sclerosis. Front Immunol. (2015) 6:272. doi: 10.3389/fimmu.2015.00272
198. Caudrillier A, Kessenbrock K, Gilliss BM, Nguyen JX, Marques MB, Monestier M, et al. Platelets induce neutrophil extracellular traps in transfusion-related acute lung injury. J Clin Invest. (2012) 122:2661–71. doi: 10.1172/JCI61303
199. Jennette JC, Falk RJ, Bacon PA, Basu N, Cid MC, Ferrario F, et al. 2012 Revised International Chapel Hill consensus conference nomenclature of vasculitides. Arthritis Rheum. (2013) 65:1–11. doi: 10.1002/art.37715
200. Ramirez GA, Maugeri N, Sabbadini MG, Rovere-Querini P, Manfredi AA. Intravascular immunity as a key to systemic vasculitis: a work in progress, gaining momentum. Clin Exp Immunol. (2014) 175:150–66. doi: 10.1111/cei.12223
201. Maugeri N, Baldini M, Ramirez GA, Rovere-Querini P, Manfredi AA. Platelet-leukocyte deregulated interactions foster sterile inflammation and tissue damage in immune-mediated vessel diseases. Thromb Res. (2012) 129:267–73. doi: 10.1016/j.thromres.2011.12.001
202. Kessenbrock K, Krumbholz M, Schonermarck U, Back W, Gross WL, Werb Z, et al. Netting neutrophils in autoimmune small-vessel vasculitis. Nat Med. (2009) 15:623–5. doi: 10.1038/nm.1959
203. Bruchfeld A, Wendt M, Bratt J, Qureshi AR, Chavan S, Tracey KJ, et al. High-mobility group box-1 protein (HMGB1) is increased in antineutrophilic cytoplasmatic antibody (ANCA)-associated vasculitis with renal manifestations. Mol Med. (2011) 17:29–35. doi: 10.2119/molmed.2010.00132
204. Wibisono D, Csernok E, Lamprecht P, Holle JU, Gross WL, Moosig F. Serum HMGB1 levels are increased in active Wegener's granulomatosis and differentiate between active forms of ANCA-associated vasculitis. Ann Rheum Dis. (2010) 69:1888–9. doi: 10.1136/ard.2009.119172
205. Wang C, de Souza AW, Westra J, Bijl M, Chen M, Zhao MH, et al. Emerging role of high mobility group box 1 in ANCA-associated vasculitis. Autoimmun Rev. (2015) 14:1057–65. doi: 10.1016/j.autrev.2015.07.010
206. Baldini M, Maugeri N, Ramirez GA, Giacomassi C, Castiglioni A, Prieto-Gonzalez S, et al. Selective up-regulation of the soluble pattern-recognition receptor pentraxin 3 and of vascular endothelial growth factor in giant cell arteritis: relevance for recent optic nerve ischemia. Arthritis Rheum. (2012) 64:854–65. doi: 10.1002/art.34515
207. Nesher G, Berkun Y, Mates M, Baras M, Rubinow A, Sonnenblick M. Low-dose aspirin and prevention of cranial ischemic complications in giant cell arteritis. Arthritis Rheum. (2004) 50:1332–7. doi: 10.1002/art.20171
208. Berger CT, Wolbers M, Meyer P, Daikeler T, Hess C. High incidence of severe ischaemic complications in patients with giant cell arteritis irrespective of platelet count and size, and platelet inhibition. Rheumatology. (2009) 48:258–61. doi: 10.1093/rheumatology/ken480
209. Weyand CM, Kaiser M, Yang H, Younge B, Goronzy JJ. Therapeutic effects of acetylsalicylic acid in giant cell arteritis. Arthritis Rheum. (2002) 46:457–66. doi: 10.1002/art.10071
210. Cavazza A, Muratore F, Boiardi L, Restuccia G, Pipitone N, Pazzola G, et al. Inflamed temporal artery: histologic findings in 354 biopsies, with clinical correlations. Am J Surg Pathol. (2014) 38:1360–70. doi: 10.1097/PAS.0000000000000244
211. Bengtsson AA, Ronnblom L. Systemic lupus erythematosus: still a challenge for physicians. J Intern Med. (2017) 281:52–64. doi: 10.1111/joim.12529
212. Tsokos GC. Systemic lupus erythematosus. N Engl J Med. (2011) 365:2110–21. doi: 10.1056/NEJMra1100359
213. Aleem A, Al Arfaj AS, khalil N, Alarfaj H. Haematological abnormalities in systemic lupus erythematosus. Acta Reumatol Port. (2014) 39:236–41.
214. Barrera-Vargas A, Campos-Guzmán J, Govea-Peláez S, García-Ramos A, Demichelis-Gómez R, Bourlon C, et al. THU0260 Systemic Lupus Erythematosus and Cytopenias: The Key Findings in Bone Marrow. Madrid: BMJ Publishing Group Ltd (2019).
215. Pereira RM, Velloso ER, Menezes Y, Gualandro S, Vassalo J, Yoshinari NH. Bone marrow findings in systemic lupus erythematosus patients with peripheral cytopenias. Clin Rheumatol. (1998) 17:219–22. doi: 10.1007/BF01451051
216. Martinez-Banos D, Crispin JC, Lazo-Langner A, Sanchez-Guerrero J. Moderate and severe neutropenia in patients with systemic lupus erythematosus. Rheumatology. (2006) 45:994–8. doi: 10.1093/rheumatology/kel016
217. Hepburn AL, Narat S, Mason JC. The management of peripheral blood cytopenias in systemic lupus erythematosus. Rheumatology. (2010) 49:2243–54. doi: 10.1093/rheumatology/keq269
218. Del Porto F, Tatarelli C, Di Napoli A, Proietta M. Systemic lupus erythematosus and myelofibrosis: a case report and revision of literature. Leuk Res Rep. (2018) 9:58–64. doi: 10.1016/j.lrr.2018.04.004
219. Velo-Garcia A, Castro SG, Isenberg DA. The diagnosis and management of the haematologic manifestations of lupus. J Autoimmun. (2016) 74:139–60. doi: 10.1016/j.jaut.2016.07.001
220. Ziakas PD, Giannouli S, Zintzaras E, Tzioufas AG, Voulgarelis M. Lupus thrombocytopenia: clinical implications and prognostic significance. Ann Rheum Dis. (2005) 64:1366–9. doi: 10.1136/ard.2004.033100
221. Fernandez M, Alarcon GS, Apte M, Andrade RM, Vila LM, Reveille JD, et al. Systemic lupus erythematosus in a multiethnic US cohort: XLIII. The significance of thrombocytopenia as a prognostic factor. Arthritis Rheum. (2007) 56:614–21. doi: 10.1002/art.22376
222. Liu Y, Kaplan MJ. Cardiovascular disease in systemic lupus erythematosus: an update. Curr Opin Rheumatol. (2018) 30:441–8. doi: 10.1097/BOR.0000000000000528
223. Joseph JE, Harrison P, Mackie IJ, Isenberg DA, Machin SJ. Increased circulating platelet-leucocyte complexes and platelet activation in patients with antiphospholipid syndrome, systemic lupus erythematosus and rheumatoid arthritis. Br J Haematol. (2001) 115:451–9. doi: 10.1046/j.1365-2141.2001.03101.x
224. Nagahama M, Nomura S, Ozaki Y, Yoshimura C, Kagawa H, Fukuhara S. Platelet activation markers and soluble adhesion molecules in patients with systemic lupus erythematosus. Autoimmunity. (2001) 33:85–94. doi: 10.3109/08916930108995993
225. Duffau P, Seneschal J, Nicco C, Richez C, Lazaro E, Douchet I, et al. Platelet CD154 potentiates interferon-alpha secretion by plasmacytoid dendritic cells in systemic lupus erythematosus. Sci Transl Med. (2010) 2:47ra63. doi: 10.1126/scitranslmed.3001001
226. Tanaka A, Ito T, Kibata K, Inagaki-Katashiba N, Amuro H, Nishizawa T, et al. Serum high-mobility group box 1 is correlated with interferon-α and may predict disease activity in patients with systemic lupus erythematosus. Lupus. (2019) 28:1120–7. doi: 10.1177/0961203319862865
227. Lood C, Tyden H, Gullstrand B, Jonsen A, Kallberg E, Morgelin M, et al. Platelet-derived S100A8/A9 and cardiovascular disease in systemic lupus erythematosus. Arthritis Rheumatol. (2016) 68:1970–80. doi: 10.1002/art.39656
228. Wu T, Xie C, Wang HW, Zhou XJ, Schwartz N, Calixto S, et al. Elevated urinary VCAM-1, P-selectin, soluble TNF receptor-1, and CXC chemokine ligand 16 in multiple murine lupus strains and human lupus nephritis. J Immunol. (2007) 179:7166–75. doi: 10.4049/jimmunol.179.10.7166
229. Delmas Y, Viallard JF, Solanilla A, Villeneuve J, Pasquet JM, Belloc F, et al. Activation of mesangial cells by platelets in systemic lupus erythematosus via a CD154-dependent induction of CD40. Kidney Int. (2005) 68:2068–78. doi: 10.1111/j.1523-1755.2005.00663.x
230. Schwarzenberger C, Sradnick J, Lerea KM, Goligorsky MS, Nieswandt B, Hugo CP, et al. Platelets are relevant mediators of renal injury induced by primary endothelial lesions. Am J Physiol Renal Physiol. (2015) 308:F1238–46. doi: 10.1152/ajprenal.00535.2014
231. Tyden H, Lood C, Gullstrand B, Nielsen CT, Heegaard NHH, Kahn R, et al. Endothelial dysfunction is associated with activation of the type I interferon system and platelets in patients with systemic lupus erythematosus. RMD Open. (2017) 3:e000508. doi: 10.1136/rmdopen-2017-000508
232. Ostergaard O, Nielsen CT, Tanassi JT, Iversen LV, Jacobsen S, Heegaard NHH. Distinct proteome pathology of circulating microparticles in systemic lupus erythematosus. Clin Proteomics. (2017) 14:23. doi: 10.1186/s12014-017-9159-8
233. McCarthy EM, Moreno-Martinez D, Wilkinson FL, McHugh NJ, Bruce IN, Pauling JD, et al. Microparticle subpopulations are potential markers of disease progression and vascular dysfunction across a spectrum of connective tissue disease. BBA Clin. (2017) 7:16–22. doi: 10.1016/j.bbacli.2016.11.003
234. Mobarrez F, Vikerfors A, Gustafsson JT, Gunnarsson I, Zickert A, Larsson A, et al. Microparticles in the blood of patients with systemic lupus erythematosus (SLE): phenotypic characterization and clinical associations. Sci Rep. (2016) 6:36025. doi: 10.1038/srep36025
235. Mobarrez F, Fuzzi E, Gunnarsson I, Larsson A, Eketjall S, Pisetsky DS, et al. Microparticles in the blood of patients with SLE: size, content of mitochondria and role in circulating immune complexes. J Autoimmun. (2019) 102:142–9. doi: 10.1016/j.jaut.2019.05.003
236. Linge P, Fortin PR, Lood C, Bengtsson AA, Boilard E. The non-haemostatic role of platelets in systemic lupus erythematosus. Nat Rev Rheumatol. (2018) 14:195–213. doi: 10.1038/nrrheum.2018.38
237. Denton CP, Khanna D. Systemic sclerosis. Lancet. (2017) 390:1685–99. doi: 10.1093/med/9780199642489.003.0121_update_001
238. Kuwana M, Okazaki Y, Yasuoka H, Kawakami Y, Ikeda Y. Defective vasculogenesis in systemic sclerosis. Lancet. (2004) 364:603–10. doi: 10.1016/S0140-6736(04)16853-0
239. Ramirez GA, Franchini S, Rovere-Querini P, Sabbadini MG, Manfredi AA, Maugeri N. The role of platelets in the pathogenesis of systemic sclerosis. Front Immunol. (2012) 3:160. doi: 10.3389/fimmu.2012.00160
240. Agache I, Radoi M, Duca L. Platelet activation in patients with systemic scleroderma–pattern and significance. Roman J Intern Med. (2007) 45:183–91.
241. Yoshizaki A, Komura K, Iwata Y, Ogawa F, Hara T, Muroi E, et al. Clinical significance of serum HMGB-1 and sRAGE levels in systemic sclerosis: association with disease severity. J Clin Immunol. (2009) 29:180–9. doi: 10.1007/s10875-008-9252-x
242. Maugeri N, Rovere-Querini P, Baldini M, Baldissera E, Sabbadini MG, Bianchi ME, et al. Oxidative stress elicits platelet/leukocyte inflammatory interactions via HMGB1: a candidate for microvessel injury in sytemic sclerosis. Antioxid Redox Signal. (2014) 20:1060–74. doi: 10.1089/ars.2013.5298
243. Dees C, Akhmetshina A, Zerr P, Reich N, Palumbo K, Horn A, et al. Platelet-derived serotonin links vascular disease and tissue fibrosis. J Exp Med. (2011) 208:961–72. doi: 10.1084/jem.20101629
244. Maugeri N, Franchini S, Campana L, Baldini M, Ramirez GA, Sabbadini MG, et al. Circulating platelets as a source of the damage-associated molecular pattern HMGB1 in patients with systemic sclerosis. Autoimmunity. (2012) 45:584–7. doi: 10.3109/08916934.2012.719946
245. Smolen JS, Aletaha D, McInnes IB. Rheumatoid arthritis. Lancet. (2016) 388:2023–38. doi: 10.1016/S0140-6736(16)30173-8
246. Kusunoki Y, Nakazawa D, Shida H, Hattanda F, Miyoshi A, Masuda S, et al. Peptidylarginine deiminase inhibitor suppresses neutrophil extracellular trap formation and MPO-ANCA production. Front Immunol. (2016) 7:227. doi: 10.3389/fimmu.2016.00227
247. Khandpur R, Carmona-Rivera C, Vivekanandan-Giri A, Gizinski A, Yalavarthi S, Knight JS, et al. NETs are a source of citrullinated autoantigens and stimulate inflammatory responses in rheumatoid arthritis. Sci Transl Med. (2013) 5:178ra40. doi: 10.1126/scitranslmed.3005580
248. Habets KL, Huizinga TW, Toes RE. Platelets and autoimmunity. Eur J Clin Invest. (2013) 43:746–57. doi: 10.1111/eci.12101
249. Farrugia M, Baron B. The role of TNF-alpha in rheumatoid arthritis: a focus on regulatory T cells. J Clin Transl Res. (2016) 2:84–90. doi: 10.18053/jctres.02.201603.005
250. Pignatelli P, De Biase L, Lenti L, Tocci G, Brunelli A, Cangemi R, et al. Tumor necrosis factor-alpha as trigger of platelet activation in patients with heart failure. Blood. (2005) 106:1992–4. doi: 10.1182/blood-2005-03-1247
251. Greenberg JD, Kremer JM, Curtis JR, Hochberg MC, Reed G, Tsao P, et al. Tumour necrosis factor antagonist use and associated risk reduction of cardiovascular events among patients with rheumatoid arthritis. Ann Rheum Dis. (2011) 70:576–82. doi: 10.1136/ard.2010.129916
252. Westlake SL, Colebatch AN, Baird J, Curzen N, Kiely P, Quinn M, et al. Tumour necrosis factor antagonists and the risk of cardiovascular disease in patients with rheumatoid arthritis: a systematic literature review. Rheumatology. (2011) 50:518–31. doi: 10.1093/rheumatology/keq316
Keywords: platelets, neutrophil, inflammation, autoimmunity, systemic lupus erythematosus, systemic sclerosis, rheumatoid arthritis, vasculitis
Citation: Ramirez GA, Manfredi AA and Maugeri N (2019) Misunderstandings Between Platelets and Neutrophils Build in Chronic Inflammation. Front. Immunol. 10:2491. doi: 10.3389/fimmu.2019.02491
Received: 04 August 2019; Accepted: 07 October 2019;
Published: 22 October 2019.
Edited by:
Craig N. Jenne, University of Calgary, CanadaReviewed by:
Rostyslav Bilyy, Danylo Halytsky Lviv National Medical University, UkraineHadi Goubran Messiha, University of Saskatchewan, Canada
Copyright © 2019 Ramirez, Manfredi and Maugeri. This is an open-access article distributed under the terms of the Creative Commons Attribution License (CC BY). The use, distribution or reproduction in other forums is permitted, provided the original author(s) and the copyright owner(s) are credited and that the original publication in this journal is cited, in accordance with accepted academic practice. No use, distribution or reproduction is permitted which does not comply with these terms.
*Correspondence: Norma Maugeri, maugeri.norma@hsr.it