- 1Division of Medical Oncology, Department of Medicine, University of Washington, Seattle, WA, United States
- 2Division of Infectious Diseases, Department of Medicine, University of Washington, Seattle, WA, United States
- 3Vaccine and Infectious Diseases Division, Fred Hutchinson Cancer Research Center, Seattle, WA, United States
- 4Division of Global Oncology, Department of Medicine, Fred Hutchinson Cancer Research Center, Seattle, WA, United States
HIV infection alters the natural history of several cancers, in large part due to its effect on the immune system. Immune function in people living with HIV may vary from normal to highly dysfunctional and is largely dependent on the timing of initiation (and continuation) of effective antiretroviral therapy (ART). An individual's level of immune function in turn affects their cancer risk, management, and outcomes. HIV-associated lymphocytopenia and immune dysregulation permit immune evasion of oncogenic viruses and premalignant lesions and are associated with inferior outcomes in people with established cancers. Various types of immunotherapy, including monoclonal antibodies, interferon, cytokines, immunomodulatory drugs, allogeneic hematopoietic stem cell transplant, and most importantly ART have shown efficacy in HIV-related cancer. Emerging data suggest that checkpoint inhibitors targeting the PD-1/PD-L1 pathway can be safe and effective in people with HIV and cancer. Furthermore, some cancer immunotherapies may also affect HIV persistence by influencing HIV latency and HIV-specific immunity. Studying immunotherapy in people with HIV and cancer will advance clinical care of all people living with HIV and presents a unique opportunity to gain insight into mechanisms for HIV eradication.
Introduction
People living with HIV (PLWH) have an elevated risk of developing cancer compared to the general population. This increased risk is partially attributable to comorbid conditions and social factors such as smoking or poorer access to preventative services. However, there is strong evidence that immunologic factors such as decreased immunologic surveillance and increased susceptibility to oncogenic viral infection play a significant role (1–5). Historically, cancers developing in the setting of HIV have been classified as AIDS-defining malignancies (ADM; cancers that, when present, confer a diagnosis of AIDS) and non-AIDS defining malignancies (NADM; cancers whose presence does not necessarily indicate AIDS) (6). Many HIV-related cancers have a viral etiology (7). These include Kaposi sarcoma (KS) [Kaposi sarcoma herpes virus (KSHV)]; cervical, anal, penile and vulvar squamous cell cancer and oropharyngeal cancers [human papilloma virus (HPV)]; B cell non-Hodgkin lymphomas (NHL) including diffuse large B-cell lymphoma, Burkitt lymphoma, plasmablastic lymphoma, primary central nervous system lymphoma, primary effusion lymphoma, classic Hodgkin lymphoma, and lymphoproliferative disorders [in some cases, Epstein-Barr virus (EBV) and/or KSHV]; hepatocellular carcinoma [hepatitis B and C viruses (HBV/HCV)], and Merkel cell carcinoma [Merkel cell polyoma virus (MPV)]. In epidemiological studies of non-Hodgkin lymphoma, Kaposi sarcoma, and anal cancer, uncontrolled HIV viremia is an independent risk factor (4, 5, 8).
The introduction of antiretroviral therapy (ART) after 1996 resulted in a reduction in the incidence of many ADMs by 75–80% (9), largely due to reduced prevalence of profound immunodeficiency. NADMs including lung cancer, Hodgkin lymphoma, anal cancer, and oropharyngeal cancer now comprise an increasing proportion of total cancers in PLWH in North America (10, 11). A similar trend has been documented in Europe, Australia (12) and the Asia-Pacific region (11, 13). This epidemiological switch in prevalence away from ADMs and virally-associated malignancies corresponds with increasing life expectancy of PLWH, increased availability of ART and promotion of viral suppression (14–16).
HIV Leads to Partially Reversable Perturbation in T-Cell Function
HIV has multiple effects on T-cell immunity that may contribute to cancer risk. Absent effective ART, uncontrolled HIV infection leads to massive depletion of HIV-infected CD4 cells and uninfected bystander CD4s in both blood and tissue (17). In the same setting, CD8 counts often rise, leading to inverted CD4/CD8 ratios that are an independent measure of immune dysfunction. Moreover, HIV and other chronic viral infections lead to increased expression of immune checkpoint proteins (such as PD-1), exhaustion markers, and impaired CD8 T cell function (18–20), causing systemic immune dysfunction and dysregulation (21). Untreated HIV perturbs not only the quantity but also the breadth of T-cell immunity. HIV leads to decreased numbers of naïve T cells, less diversity of the T-cell repertoire in the blood (22, 23), and skewing of the T-cell receptor (TCR) repertoire secondary to CD4 depletion and expansion of oligoclonal CD8 populations (24). HIV viremia is rapidly suppressed with modern ART. Immune reconstitution after initiation of ART leads to CD4 recovery and CD8 decline over time (25). The likelihood of full immune recovery improves with earlier diagnosis and a younger age at ART initiation (26), although immune recovery is often incomplete (27). The heightened pro-inflammatory state associated with both untreated and treated HIV contributes to long-term adverse outcomes (28, 29).
Oncogenesis in the Setting of HIV-Induced Immune Dysfunction
Immunodeficiency is an established risk factor for the development of cancer, and the underlying causes are likely many, including uncontrolled proliferation of oncogenic viruses and inadequate immune surveillance. Many oncogenic viruses have been shown to cause cancer in other immunosuppressed states, including inherited immunodeficiencies and solid-organ transplantation (30). CD4 deficiency is strongly linked to malignancy (31), independent of HIV infection (32–35). The presence, number, and functionality of CD4 T cells are important in multiple steps of the oncogenic pathway, including recognition of tumor antigens, development of effective neutralizing antibody, and cellular responses to viral pathogens, and clearance of premalignant lesions. The risk of many HIV-associated malignancies decreases with improved CD4 count on ART (9, 12, 36–39) and cancer-specific mortality correlates inversely with CD4 count (12, 40). The link between reduced CD4 count and elevated cancer risk is profound in KS and NHL (41–43), but also present in other malignancies (37). An individual's risk of cancer (and long-term immune dysfunction) is likely influenced by the CD4 nadir, perhaps indicative of a synergistic relationship between chronic inflammation and impaired immune surveillance (10, 44–49).
CD4 lymphocytopenia, ineffective CD8 response, and associated immune dysregulation lead to a reduction in immunosurveillance, a key mechanism in HIV-associated oncogenesis (21, 50). This is illustrated in the link between HIV, immune status, and cervical cancer (37). PLWH are more likely to acquire high risk HPV (51, 52), less likely to clear HPV, and more likely to progress to higher-grade forms of dysplasia (53). PLWH with lower CD4 counts are also more likely to progress from dysplasia to invasive cancer (54). In an HPV vaccine trial in adolescents with HIV, the induced antibody titer correlated positively with CD4 count (55), supporting the importance of CD4 T cells in the production of high-affinity antibodies (51), the primary correlate of protection of the HPV vaccine (56). Tissue-localizing HPV-specific CD4 and CD8 T cells are also potentially important to tumor regression (57, 58).
Immune exhaustion and T-cell senescence are prominent features of both chronic viral infections and malignancies (59). In PLWH, T-cell dysfunction is most strongly implicated in the development of EBV-related lymphomas and KS (60). In HIV-associated B cell NHL, reduced T-cell polyfunctionality and TCR diversity is associated with poorer prognosis (61). These observations, among others (62), have led to interest in remedying immune dysfunction to treat malignancy in PLWH (63).
Antiretroviral Therapy and Other Forms of Immunotherapy in HIV-Related Cancer
ART is itself an effective form of immunotherapy for ADM. Improvements in ART in 1996 resulted in a decline in the incidence and severity of KS, as well as changes in its natural history (9, 64–66): the risk of death due to KS decreased at similar HIV RNA levels and CD4 count (66), suggesting that ART resulted not only in improved immune control of KSHV but also decreased immune dysregulation. ART-induced immune reconstitution results in regression of KS lesions in ~80% of PLWH with early KS (67). However, ART alone is often insufficient in advanced KS.
Several immunotherapies have shown efficacy in KS and other HIV-related cancers (Table 1). Interferon alpha (IFN-α), the first true immunotherapy used in HIV-associated KS, generated a 20–40% response rate (98–100). IL-12, which enhances Th-1 type immune responses (91), has been shown to have anti-KS activity in patients who are progressing despite ART (92) and is currently being developed as a tumor-targeted immunocytokine, NHS-IL12 (101). A recent trial of the immunomodulatory drug pomalidomide in 22 participants with heavily pretreated KS who were virally suppressed on ART noted an overall response rate of 60% among HIV-infected participants, which is comparable to traditional cytotoxic chemotherapy for KS. The investigators observed expansion of central memory cells and decreases in CD57+ immunosenescent T-cells (73, 74).
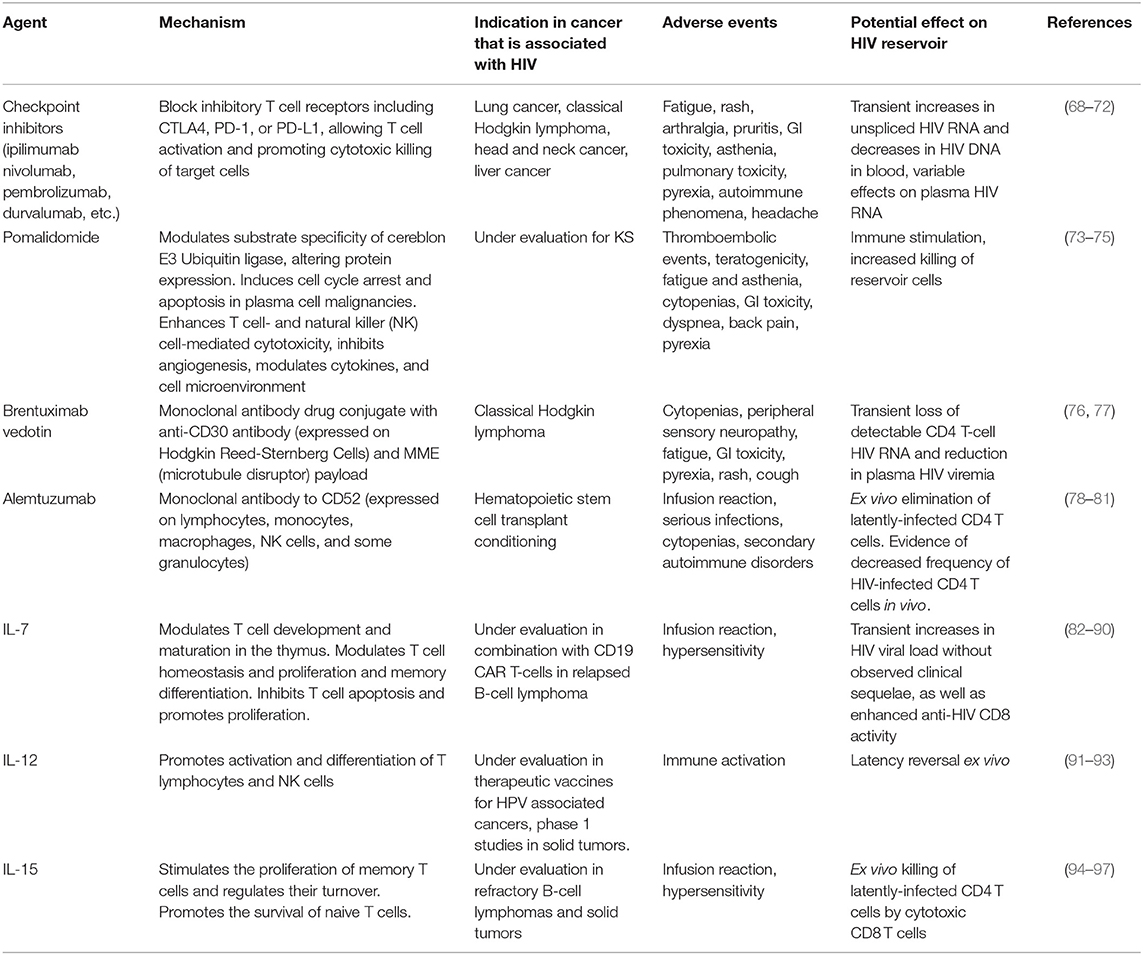
Table 1. Select immunotherapeutic agents used in cancers that occur at increased frequency in people with HIV and their demonstrated or hypothesized effect on measurements of the HIV reservoir.
Despite immune dysfunction due to HIV, cancer in PLWH is often responsive to immunotherapy. Thus far, the best-studied agents are tumor-targeting monoclonal antibodies in the management of HIV-associated lymphomas. Rituximab, a monoclonal antibody to the B-cell antigen CD20 that works in part through antibody-dependent cell-mediated cytotoxicity, is associated with improved overall survival in NHL when compared to chemotherapy alone (102–104). In people with HIV-associated lymphoma, a pooled analysis of over 1,500 patients noted that rituximab improved overall survival in those with a CD4 count >50 cells/μL (105). Brentuximab vedotin, an antibody-drug conjugate directed at CD30 on Reed-Sternberg cells, has been shown to have activity in HIV-associated Hodgkin lymphoma: in a study of 6 patients with HIV and classical Hodgkin lymphoma, all achieved a complete response with minimal hematologic toxicity or infectious complications (106).
More recently, immune checkpoint inhibitors (CPIs), monoclonal antibodies to cytotoxic lymphocyte associated protein 4 (CTLA-4) or programmed cell death 1 or its ligand (PD-1 and PD-L1), have gained widespread use due to their demonstrated activity and favorable toxicity profile in many malignancies. CPIs, which function by blocking T-cell inhibitory signaling, have performed well in clinical trials of many malignancies that are common in the setting of HIV, including lymphoma, lung cancer, cervical cancer, liver cancer, and head and neck cancers (107, 108). While nearly all these trials excluded PLWH (109), case reports and retrospective cohort studies from US and European collaborative groups have described an acceptable safety profile with the use of nivolumab, pembrolizumab, and ipilimumab in PLWH, with reported tumor responses in classical Hodgkin lymphoma, melanoma and lung cancer (68, 69, 110–116). A systematic review of CPIs in PLWH noted overall response and adverse event rates that were similar to the general population. In the subset of patients in whom viral load was measured, HIV remained suppressed in 93% of participants, and CD4 counts increased modestly. Notably, CPI use in KS was associated with an overall response rate of 63% (117). A prospective cohort study of 10 PLWH with NSCLC treated with nivolumab noted similar response rates to HIV-uninfected patients: 2 patients had a partial response, 4 had stable disease, and 4 progressed. All patients tolerated nivolumab well with no serious adverse events (70). A prospective phase 1 study of pembrolizumab in PLWH with a CD4 count >100 cells/μl and advanced cancer demonstrated evidence of safety and activity in KS, NHL, lung cancer, and liver cancer (118). A study of durvalumab in 20 aviremic PLWH with advanced solid tumors likewise reported no serious adverse events, nor evidence of HIV reactivation during durvalumab therapy (119). Ongoing studies evaluating CPIs in HIV-associated cancers include a phase 1 study of nivolumab (anti-PD-1) combined with ipilimumab (anti-CTLA-4) in relapsed classical Hodgkin lymphoma or solid tumors (NCT02408861), a phase 2 study of nivolumab in advanced non-small cell lung cancer (NCT03304093), a phase 2 study of durvalumab in advanced cancer (NCT03094286), a study of pembrolizumab as first systemic therapy in KS (NCT02595866), and intralesional nivolumab for limited cutaneous KS (NCT03316274).
Cancer Immunotherapy and HIV Persistance
Although HIV-infected individuals on ART may have undetectable plasma HIV RNA by standard clinical assays, a reservoir of latently HIV-infected cells (120, 121) persists from which the virus will resurface after discontinuation of ART (122). Persistence of the HIV reservoir is partly due to the inherent longevity of resting memory CD4 T cells; growing evidence suggests that its persistence is maintained by clonal expansion (123, 124). In whole genome-based studies, HIV integration favors sites of active gene transcription (125) which benefits HIV replication and establishment of latency (126, 127) and promotes pathways associated with oncogenesis (124). The HIV reservoir has been a major subject of research into a functional cure for HIV. One theory called “kick and kill” (Figure 1) (128, 129) proposes that HIV latency reversal in the setting of ART (meaning activation of HIV replication within a latently infected cell), can lead to increased immunogenicity of HIV infected cells, enhancement of anti-HIV immunity, and increased cell death of HIV reservoir cells.
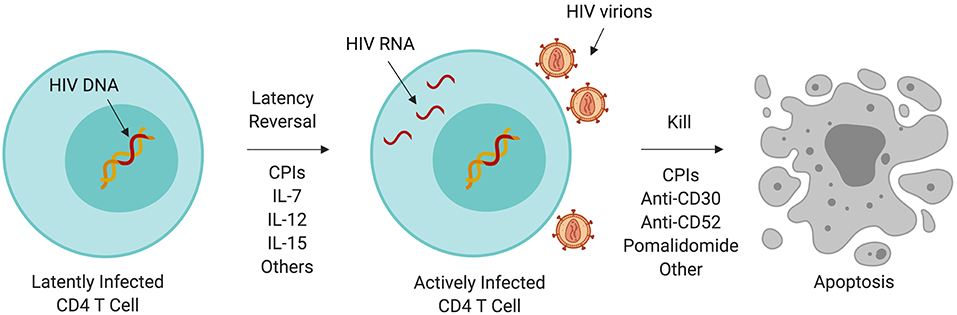
Figure 1. Immunotherapy and the HIV reservoir. A variety of immunotherapeutic agents used to treat cancer may perturb the HIV reservoir through induction of latency reversal or increased cell killing. Some of these agents are being evaluated in clinical trials targeting HIV persistence. CPI, immune checkpoint inhibitor; IL, interleukin.
Several immunotherapeutic agents used in the treatment of cancer may have cause HIV latency reversal and/or have a targeted effect on HIV persistence. CPIs have been proposed to have latency reversal activity. Anti-PD-1 therapy is associated with changes in CD4 count and HIV RNA (130–132), perhaps due to direct targeting of the HIV reservoir. PD-1 and CTLA-4 expression are increased in the setting of chronic HIV infection, and HIV DNA and unspliced RNA are enriched in PD-1+ cells in blood and lymph nodes of individuals with HIV on ART (133–136). Multiple case reports and prospective studies have documented transient increases in HIV transcription in CD4 cells in people with HIV-associated malignancies on ART who are treated with anti-PD-(L)1 drugs, although many of these participants later experienced decreases in plasma HIV RNA (117, 128, 129, 132, 137). In one study, 2 of 28 patients who had undetectable HIV RNA prior to CPI therapy developed detectable HIV RNA, whereas 5 of 6 patients who had detectable viremia experienced a decrease in their viral load (117). A prospective study of the effect of ipilimumab in 24 PLWH with detectable viremia and without cancer, of whom 17 were on ART, also demonstrated a range of responses: 2 participants had slight decreases in HIV RNA but 14 had slight increases. None experienced significant change in CD4 or CD8 T cell count (138). These observations support the activity of CPIs to produce latency reversal. Additional studies are being performed to evaluate the effects of CPIs on anti-HIV T-cell function.
The effects of anti-CD30 monoclonal antibodies on HIV latency have also been investigated. Early work in HIV demonstrated that cross-linking of CD30 on latently-infected CD4 T cells induced HIV transcription (139). More recently, brentuximab vedotin has been associated with transient loss of detectable CD4 T-cell HIV RNA and reduction in plasma HIV viremia (76). CD30 is therefore speculated to be a marker of latent, but transcriptionally-active, HIV-infected cells and a potential therapeutic target for HIV eradication (140).
Alemtuzumab is a monoclonal antibody targeting CD52, which is expressed by T cells including HIV-infected T cells, regardless of CD4 count or plasma viremia. Latently-infected CD4 T cells have been eliminated in vitro with alemtuzumab (78). In vivo, a case report of alemtuzumab in an individual with HIV and Sezary syndrome described decreased frequency but not elimination of HIV-infected CD4 T cells (79). Alemtuzumab was also part of the conditioning regimen of one of the patients with sustained HIV aviremia after HSCT (141).
T-cell growth factors, many of which are being investigated for cancer indications, have also been shown to affect the HIV reservoir. Interleukin 7 (IL-7) is a homeostatic cytokine that increases T-cell repertoire diversity through expansion of naive T cells (82) and is being investigated in several malignancies. IL-7 levels increase in HIV-associated CD4 lymphocytopenia and decrease with immune reconstitution (142). Exogenous administration of IL-7 is associated with dose-dependent increases in CD4 and CD8 T cells in PLWH on ART (143), including HIV-specific CD8 T cells (83). In patients with suppressed HIV, administration of IL-7 led to transient increases in HIV viral load without observed clinical sequelae (84), as well as enhanced anti-HIV CD8 activity. Another T-cell growth factor, IL-15, induces antigen-specific T-cell proliferation, most pronounced in the CD8 compartment (94, 95, 144, 145). IL-15 is produced during acute HIV infection (95). Stimulating NK cells with IL-15 ex vivo from participants with suppressed HIV on ART led to ex vivo killing of latently-infected CD4 T cells by cytotoxic CD8 T cells (96). Early phase studies of IL-7 and IL-15 in several malignancies are underway.
Hematopoietic Stem Cell Transplantation in HIV
In 2007, an individual with HIV infection and leukemia underwent hematopoietic stem cell transplant (HSCT) in Berlin, using cells from a donor who was homozygous for CCR5-delta32, a mutation that renders CD4 cells resistant to CCR5-tropic HIV. After transplant, HIV was undetectable in blood and biopsy specimens, despite discontinuation of ART (146, 147). Recently, a second patient who underwent allogeneic HSCT for Hodgkin lymphoma using cells from a homozygous CCR5-delta32 donor and whose HIV remained undetectable 18 months after stopping ART (141) was described. Allogeneic stem cell transplant itself appears to substantially decrease the HIV reservoir. In the European IciStem cohort of PLWH on ART who underwent HSCT for hematologic malignancies from CCR5 wild-type donors with full donor engraftment and who remained on ART, 5 of 6 were found to have no detectable HIV DNA in CD4 cells from blood and tissues and no evidence of HIV in a humanized mouse viral outgrowth assay (148). However, ART interruption is required to demonstrate functional cure, and in cases of allotransplants from CCR5 wild-type donors, HSCT has failed to produce long-lasting viral suppression in the absence of ART. In an ART interruption study of 2 PLWH who underwent HSCT for hematologic malignancies from CCR5 wild-type donors and had undetectable HIV RNA for years post-transplant while on ART, both participants developed detectable viremia after ART interruption: patient A at day 84 and patient B at day 225 (149).
Given the success of allotransplants from homozygous CCR5-delta32 donors, CCR5-mutant cell products have been developed via gene editing and have been shown to be safe when infused into participants with chronic aviremic HIV. When ART was interrupted, the edited CD4 cells declined at a slower rate than endogenous CD4 cells. While these results are promising, additional work is required to develop a scalable approach to address HIV persistence on ART (150–153).
Improving Our Understanding of HIV-Related Cancer
As PLWH are living longer, cancer has become a major cause of morbidity and mortality, well above the burden faced by the general population. Although the incidence of AIDS-defining malignancies has decreased, mortality associated with NADMs is rising. Given the persistent immune abnormalities despite ART and the implications for cancer risk, immunotherapy is uniquely poised to improve outcomes in HIV-associated cancers. In order to advance our understanding, PLWH must be included in immuno-oncology studies. Recent recommendations from ASCO and the FDA provide guidance for appropriate inclusion of PLWH and cancer in clinical trials (109, 154). Furthermore, studying cancer immunotherapy in this population represents an opportunity to gain a better understanding of HIV itself. Investigation of the immunologic and viral responses to cancer immunotherapy in PLWH will lead to novel insights into HIV elimination and, above all, improve the outcomes of people with HIV and cancer.
Author Contributions
All authors listed have made a substantial, direct and intellectual contribution to the work, and approved it for publication.
Funding
CP was supported by the Jacob Green Fund. EF was supported by U19 AI113173.
Conflict of Interest Statement
TU reports research support for investigator initiated research from Merck, Celgene, and Roche outside the submitted work. In addition TU has a patent 10,001,483 B2 issued.
The remaining authors declare that the research was conducted in the absence of any commercial or financial relationships that could be construed as a potential conflict of interest.
References
1. Coghill AE, Han X, Suneja G, Lin CC, Jemal A, Shiels MS. Advanced stage at diagnosis and elevated mortality among US patients with cancer infected with HIV in the National Cancer Data Base. Cancer. (2019) 125:2868–76. doi: 10.1002/cncr.32158
2. Shepherd L, Ryom L, Law M, Petoumenos K, Hatleberg CI, d'Arminio Monforte A, et al. Cessation of cigarette smoking and the impact on cancer incidence in human immunodeficiency virus-infected persons: the data collection on adverse events of anti-HIV drugs study. Clin Infect Dis. (2019) 68:650–7. doi: 10.1093/cid/ciy508
3. Grover S, Desir F, Jing Y, Bhatia RK, Trifiletti DM, Swisher-McClure S, et al. Reduced cancer survival among adults with HIV and AIDS-defining illnesses despite no difference in cancer stage at diagnosis. J Acquir Immune Defic Syndr. (2018) 79:421–9. doi: 10.1097/QAI.0000000000001842
4. Hernandez-Ramirez RU, Qin L, Lin H, Leyden W, Neugebauer RS, Althoff KN, et al. Association of immunosuppression and HIV viremia with anal cancer risk in persons living with HIV in the United States and Canada. Clin Infect Dis. (2019) 2019:ciz329. doi: 10.1093/cid/ciz329
5. Hernández-Ramírez RU, Qin L, Lin H, Leyden W, Neugebauer RS, Althoff KN, et al. Association of immunosuppression and HIV viraemia with non-Hodgkin lymphoma risk overall and by subtype in people living with HIV in Canada and the USA: a multicentre cohort study. Lancet HIV. (2019) 6:e240–e9.
6. Patel P, Hanson DL, Sullivan PS, Novak RM, Moorman AC, Tong TC, et al. Incidence of types of cancer among HIV-infected persons compared with the general population in the United States, 1992–2003. Ann Intern Med. (2008) 148:728–36. doi: 10.7326/0003-4819-148-10-200805200-00005
7. Park LS, Hernández-Ramírez RU, Silverberg MJ, Crothers K, Dubrow R. Prevalence of non-HIV cancer risk factors in persons living with HIV/AIDS: a meta-analysis. AIDS. (2016) 30:273–91. doi: 10.1097/QAD.0000000000000922
8. Achenbach CJ, Buchanan AL, Cole SR, Hou L, Mugavero MJ, Crane HM, et al. HIV viremia and incidence of non-Hodgkin lymphoma in patients successfully treated with antiretroviral therapy. Clin Infect Dis. (2014) 58:1599–606. doi: 10.1093/cid/ciu076
9. Franceschi S, Lise M, Clifford GM, Rickenbach M, Levi F, Maspoli M, et al. Changing patterns of cancer incidence in the early- and late-HAART periods: the Swiss HIV Cohort Study. Br J Cancer. (2010) 103:416–22. doi: 10.1038/sj.bjc.6605756
10. Franzetti M, Ricci E, Bonfanti P. The pattern of non-AIDS-defining cancers in the HIV population: epidemiology, risk factors and prognosis. A review. Curr HIV Res. (2019) 17:1–12. doi: 10.2174/1570162X17666190327153038
11. Nagata N, Nishijima T, Niikura R, Yokoyama T, Matsushita Y, Watanabe K, et al. Increased risk of non-AIDS-defining cancers in Asian HIV-infected patients: a long-term cohort study. BMC Cancer (2018) 18:1066. doi: 10.1186/s12885-018-4963-8
12. Worm SW, Bower M, Reiss P, Bonnet F, Law M, Fätkenheuer G, et al. Non-AIDS defining cancers in the D:A:D Study–time trends and predictors of survival: a cohort study. BMC Infect Dis. (2013) 13:471. doi: 10.1186/1471-2334-13-471
13. Jung IY, Rupasinghe D, Woolley I, O'Connor CC, Giles M, Azwa RI, et al. Trends in mortality among ART-treated HIV-infected adults in the Asia-Pacific region between 1999 and 2017: results from the TREAT Asia HIV Observational Database (TAHOD) and Australian HIV Observational Database (AHOD) of IeDEA Asia-Pacific. J Int AIDS Soc. (2019) 22:e25219. doi: 10.1002/jia2.25219
14. Shiels MS, Pfeiffer RM, Gail MH, Hall HI, Li J, Chaturvedi AK, et al. Cancer burden in the HIV-infected population in the United States. J Natl Cancer Inst. (2011) 103:753–62. doi: 10.1093/jnci/djr076
15. Cobucci RN, Lima PH, de Souza PC, Costa VV, Cornetta Mda C, Fernandes JV, et al. Assessing the impact of HAART on the incidence of defining and non-defining AIDS cancers among patients with HIV/AIDS: a systematic review. J Infect Public Health. (2015) 8:1–10. doi: 10.1016/j.jiph.2014.08.003
16. Coghill AE, Shiels MS, Suneja G, Engels EA. Elevated cancer-specific mortality among HIV-infected patients in the United States. J Clin Oncol. (2015) 33:2376–83. doi: 10.1200/JCO.2014.59.5967
17. Doitsh G, Cavrois M, Lassen KG, Zepeda O, Yang Z, Santiago ML, et al. Abortive HIV infection mediates CD4 T cell depletion and inflammation in human lymphoid tissue. Cell. (2010) 143:789–801. doi: 10.1016/j.cell.2010.11.001
18. Cockerham LR, Jain V, Sinclair E, Glidden DV, Hartogenesis W, Hatano H, et al. Programmed death-1 expression on CD4? and CD8? T cells in treated and untreated HIV disease. AIDS. (2014) 28:1749–58. doi: 10.1097/QAD.0000000000000314
19. Khoury G, Fromentin R, Solomon A, Hartogensis W, Killian M, Hoh R, et al. Human immunodeficiency virus persistence and T-cell activation in blood, rectal, and lymph node tissue in human immunodeficiency virus-infected individuals receiving suppressive antiretroviral therapy. J Infect Dis. (2017) 215:911–9. doi: 10.1093/infdis/jix039
20. Betts MR, Nason MC, West SM, De Rosa SC, Migueles SA, Abraham J, et al. HIV nonprogressors preferentially maintain highly functional HIV-specific CD8+ T cells. Blood. (2006) 107:4781–9. doi: 10.1182/blood-2005-12-4818
21. Porichis F, Hart MG, Massa A, Everett HL, Morou A, Richard J, et al. Immune checkpoint blockade restores HIV-specific CD4 T cell help for NK cells. J Immunol. (2018) 201:971–81. doi: 10.4049/jimmunol.1701551
22. Baum PD, Young JJ, Schmidt D, Zhang Q, Hoh R, Busch M, et al. Blood T-cell receptor diversity decreases during the course of HIV infection, but the potential for a diverse repertoire persists. Blood. (2012) 119:3469–77. doi: 10.1182/blood-2011-11-395384
23. Connors M, Kovacs JA, Krevat S, Gea-Banacloche JC, Sneller MC, Flanigan M, et al. HIV infection induces changes in CD4+ T-cell phenotype and depletions within the CD4+ T-cell repertoire that are not immediately restored by antiviral or immune-based therapies. Nat Med. (1997) 3:533–40. doi: 10.1038/nm0597-533
24. Heather JM, Best K, Oakes T, Gray ER, Roe JK, Thomas N, et al. Dynamic Perturbations of the T-cell receptor repertoire in chronic HIV infection and following antiretroviral therapy. Front Immunol. (2015) 6:644. doi: 10.3389/fimmu.2015.00644
25. Funderburg NT, Andrade A, Chan ES, Rosenkranz SL, Lu D, Clagett B, et al. Dynamics of immune reconstitution and activation markers in HIV+ treatment-naive patients treated with raltegravir, tenofovir disoproxil fumarate and emtricitabine. PLoS ONE. (2013) 8:e83514. doi: 10.1371/journal.pone.0083514
26. Wilson EM, Sereti I. Immune restoration after antiretroviral therapy: the pitfalls of hasty or incomplete repairs. Immunol Rev. (2013) 254:343–54. doi: 10.1111/imr.12064
27. Kelley CF, Kitchen CM, Hunt PW, Rodriguez B, Hecht FM, Kitahata M, et al. Incomplete peripheral CD4+ cell count restoration in HIV-infected patients receiving long-term antiretroviral treatment. Clin Infect Dis. (2009) 48:787–94. doi: 10.1086/597093
28. Castillo-Mancilla JR, Phillips AN, Neaton JD, Neuhaus J, Sharma S, Baker JV, et al. Incomplete ART adherence is associated with higher inflammation in individuals who achieved virologic suppression in the START study. J Int AIDS Soc. (2019) 22:e25297. doi: 10.1002/jia2.25297
29. Nasi M, De Biasi S, Gibellini L, Bianchini E, Pecorini S, Bacca V, et al. Ageing and inflammation in patients with HIV infection. Clin Exp Immunol. (2017) 187:44–52. doi: 10.1111/cei.12814
30. Bonnet F, Lewden C, May T, Heripret L, Jougla E, Bevilacqua S, et al. Malignancy-related causes of death in human immunodeficiency virus-infected patients in the era of highly active antiretroviral therapy. Cancer. (2004) 101:317–24. doi: 10.1002/cncr.20354
31. Clifford GM, Franceschi S. Cancer risk in HIV-infected persons: influence of CD4+ count. Future Oncol. (2009) 5:669–78. doi: 10.2217/fon.09.28
32. Dutta A, Uno H, Lorenz DR, Wolinsky SM, Gabuzda D. Low T-cell subsets prior to development of virus-associated cancer in HIV-seronegative men who have sex with men. Cancer Causes Control. (2018) 29:1131–42. doi: 10.1007/s10552-018-1090-4
33. Grossman D, Lewis DE, Ballas ZK, Duvic M. Idiopathic CD4+ T lymphocytopenia in a patient with mycosis fungoides. J Am Acad Dermatol. (1994) 31:275–6. doi: 10.1016/S0190-9622(08)81978-0
34. Paolini R, D'Andrea E, Poletti A, Del Mistro A, Zerbinati P, Girolami A. B Non-Hodgkin's Lymphoma in a Haemophilia Patient with Idiopathic CD4+ T-lymphocytopenia. Leuk Lymph. (1996) 21:177–80. doi: 10.3109/10428199609067597
35. Menetrier-Caux C, Ray-Coquard I, Blay JY, Caux C. Lymphopenia in cancer patients and its effects on response to immunotherapy: an opportunity for combination with cytokines? J Immunother Cancer. (2019) 7:85. doi: 10.1186/s40425-019-0549-5
36. Asgari MM, Ray GT, Quesenberry CP, Katz KA, Silverberg MJ. Association of multiple primary skin cancers with human immunodeficiency virus infection, CD4 count, and viral load. JAMA Dermatol. (2017) 153:892–6. doi: 10.1001/jamadermatol.2017.1716
37. Hernández-Ramírez RU, Shiels MS, Dubrow R, Engels EA. Cancer risk in HIV-infected people in the USA from 1996 to 2012: a population-based, registry-linkage study. Lancet HIV. (2017) 4:e495–504. doi: 10.1016/S2352-3018(17)30125-X
38. Ryom L, Lundgren JD, De Wit S, Kovari H, Reiss P, Law M, et al. Use of antiretroviral therapy and risk of end-stage liver disease and hepatocellular carcinoma in HIV-positive persons. AIDS. (2016) 30:1731–43. doi: 10.1097/QAD.0000000000001018
39. Yarchoan R, Uldrick TS. HIV-associated cancers and related diseases. N Engl J Med. (2018) 378:1029–41. doi: 10.1056/NEJMra1615896
40. Ebogo-Belobo JT, Kagoué Simeni LA, Mbassa Nnouma G, Lawan Loubou M, Abamé I, Tchuisseu Hapi A, et al. Incidence of cancer in people living with HIV and prognostic value of current CD4. Bull Cancer. (2019) 106:201–5. doi: 10.1016/j.bulcan.2018.11.003
41. Dubrow R, Qin L, Lin H, Hernández-Ramírez RU, Neugebauer RS, Leyden W, et al. Association of CD4+ T-cell Count, HIV-1 RNA viral load, and antiretroviral therapy with kaposi sarcoma risk among HIV-infected persons in the United States and Canada. J Acquir Immune Defic Syndr. (2017) 75:382–90. doi: 10.1097/QAI.0000000000001394
42. Shepherd L, Ryom L, Law M, Hatleberg CI, de Wit S, Monforte AD, et al. Differences in virological and immunological risk factors for non-Hodgkin and Hodgkin lymphoma. J Natl Cancer Inst. (2018) 110:598–607. doi: 10.1093/jnci/djx249
43. Rohner E, Bütikofer L, Schmidlin K, Sengayi M, Maskew M, Giddy J, et al. Comparison of Kaposi sarcoma risk in human immunodeficiency virus-positive adults across 5 continents: a multiregional multicohort study. Clin Infect Dis. (2017) 65:1316–26. doi: 10.1093/cid/cix480
44. Ostroumov D, Fekete-Drimusz N, Saborowski M, Kühnel F, Woller N. CD4 and CD8 T lymphocyte interplay in controlling tumor growth. Cell Mol Life Sci. (2018) 75:689–713. doi: 10.1007/s00018-017-2686-7
45. Serrano-Villar S, Pérez-Elías MJ, Dronda F, Casado JL, Moreno A, Royuela A, et al. Increased risk of serious non-AIDS-related events in HIV-infected subjects on antiretroviral therapy associated with a low CD4/CD8 ratio. PLoS ONE. (2014) 9:e85798. doi: 10.1371/journal.pone.0085798
46. Serrano-Villar S, Sainz T, Lee SA, Hunt PW, Sinclair E, Shacklett BL, et al. HIV-infected individuals with low CD4/CD8 ratio despite effective antiretroviral therapy exhibit altered T cell subsets, heightened CD8+ T cell activation, and increased risk of non-AIDS morbidity and mortality. PLoS Pathog. (2014) 10:e1004078. doi: 10.1371/journal.ppat.1004078
47. igel K, Makinson A, Thaler J. Lung cancer in persons with HIV. Curr Opin HIV AIDS. (2017) 12:31–8. doi: 10.1097/COH.0000000000000326
48. Clifford GM, Rickenbach M, Lise M, Dal Maso L, Battegay M, Bohlius J, et al. Hodgkin lymphoma in the Swiss HIV cohort study. Blood. (2009) 113:5737–42. doi: 10.1182/blood-2009-02-204172
49. Hema MN, Ferry T, Dupon M, Cuzin L, Verdon R, Thiébaut R, et al. Low CD4/CD8 ratio is associated with non AIDS-defining cancers in patients on antiretroviral therapy: ANRS CO8 (Aproco/Copilote) prospective cohort study. PLoS ONE. (2016) 11:e0161594. doi: 10.1371/journal.pone.0161594
50. Okoye IS, Houghton M, Tyrrell L, Barakat K, Elahi S. Coinhibitory receptor expression and immune checkpoint blockade: maintaining a balance in CD8(+) T cell responses to chronic viral infections and cancer. Front Immunol. (2017) 8:1215. doi: 10.3389/fimmu.2017.01215
51. Konopnicki D, Manigart Y, Gilles C, Barlow P, de Marchin J, Feoli F, et al. High-risk human papillomavirus infection in HIV-positive African women living in Europe. J Int AIDS Soc. (2013) 16:18023. doi: 10.7448/IAS.16.1.18023
52. Luchters SM, Vanden Broeck D, Chersich MF, Nel A, Delva W, Mandaliya K, et al. Association of HIV infection with distribution and viral load of HPV types in Kenya: a survey with 820 female sex workers. BMC Infect Dis. (2010) 10:18. doi: 10.1186/1471-2334-10-18
53. Massad LS, Seaberg EC, Wright RL, Darragh T, Lee YC, Colie C, et al. Squamous cervical lesions in women with human immunodeficiency virus. Obstet Gynecol. (2008) 111:1388–93. doi: 10.1097/AOG.0b013e3181744619
54. Abraham SN, Miao Y. The nature of immune responses to urinary tract infections. Nat Rev Immunol. (2015) 15:655–63. doi: 10.1038/nri3887
55. Mugo NR, Eckert L, Magaret AS, Cheng A, Mwaniki L, Ngure K, et al. Quadrivalent HPV vaccine in HIV-1-infected early adolescent girls and boys in Kenya: Month 7 and 12 post vaccine immunogenicity and correlation with immune status. Vaccine. (2018) 36:7025–32. doi: 10.1016/j.vaccine.2018.09.059
56. Scherer EM, Smith RA, Simonich CA, Niyonzima N, Carter JJ, Galloway DA. Characteristics of memory B cells elicited by a highly efficacious HPV vaccine in subjects with no pre-existing immunity. PLoS Pathog. (2014) 10:e1004461. doi: 10.1371/journal.ppat.1004461
57. Kenter GG, Welters MJ, Valentijn AR, Lowik MJ, Berends-van der Meer DM, Vloon AP, et al. Vaccination against HPV-16 oncoproteins for vulvar intraepithelial neoplasia. N Engl J Med. (2009) 361:1838–47. doi: 10.1056/NEJMoa0810097
58. Maldonado L, Teague JE, Morrow MP, Jotova I, Wu TC, Wang C, et al. Intramuscular therapeutic vaccination targeting HPV16 induces T cell responses that localize in mucosal lesions. Sci Transl Med. (2014) 6:221ra13. doi: 10.1126/scitranslmed.3007323
59. Saeidi A, Zandi K, Cheok YY, Saeidi H, Wong WF, Lee CYQ, et al. T-cell exhaustion in chronic infections: reversing the state of exhaustion and reinvigorating optimal protective immune responses. Front Immunol. (2018) 9:2569. doi: 10.3389/fimmu.2018.02569
60. Unemori P, Leslie KS, Hunt PW, Sinclair E, Epling L, Mitsuyasu R, et al. Immunosenescence is associated with presence of Kaposi's sarcoma in antiretroviral treated HIV infection. AIDS. (2013) 27:1735–42. doi: 10.1097/QAD.0b013e3283601144
61. Hernández DM, Valderrama S, Gualtero S, Hernández C, López M, Herrera MV, et al. Loss of T-cell multifunctionality and TCR-Vβ repertoire against Epstein- Barr virus is associated with worse prognosis and clinical parameters in HIV+patients. Front Immunol. (2018) 9:2291. doi: 10.3389/fimmu.2018.02291
62. Zheng J, Wang L, Cheng Z, Pei Z, Zhang Z, Li Z, et al. Molecular changes of lung malignancy in HIV infection. Sci Rep. (2018) 8:13128. doi: 10.1038/s41598-018-31572-6
63. Bender Ignacio RA, Lin LL, Rajdev L, Chiao E. Evolving paradigms in HIV malignancies: review of ongoing clinical trials. J Natl Compr Canc Netw. (2018) 16:1018–26. doi: 10.6004/jnccn.2018.7064
64. Mocroft A, Kirk O, Clumeck N, Gargalianos-Kakolyris P, Trocha H, Chentsova N, et al. The changing pattern of Kaposi sarcoma in patients with HIV, 1994–2003: the EuroSIDA Study. Cancer. (2004) 100:2644–54. doi: 10.1002/cncr.20309
65. Franceschi S, Maso LD, Rickenbach M, Polesel J, Hirschel B, Cavassini M, et al. Kaposi sarcoma incidence in the Swiss HIV Cohort Study before and after highly active antiretroviral therapy. Brit J Cancer. (2008) 99:800–4. doi: 10.1038/sj.bjc.6604520
66. Gallafent JH, Buskin SE, De Turk PB, Aboulafia DM, Gallafent JH, Buskin SE, et al. Profile of patients with Kaposi's sarcoma in the era of highly active antiretroviral therapy. J Clin Oncol. (2005) 23:1253–60. doi: 10.1200/JCO.2005.04.156
67. Antman K, Chang Y. Kaposi's sarcoma. N Engl J Med. (2000) 342:1027–38. doi: 10.1056/NEJM200004063421407
68. Heppt MV, Schlaak M, Eigentler TK, Kähler KC, Kiecker F, Loquai C, et al. Checkpoint blockade for metastatic melanoma and Merkel cell carcinoma in HIV-positive patients. Ann Oncol. (2017):3104–6. doi: 10.1093/annonc/mdx538
69. Tio M, Rai R, Ezeoke OM, Zimmer L, Khoo C, Park JJ, et al. Anti-PD-1/PD-L1 immunotherapy in patients with solid organ transplant, HIV or hepatitis B/C infection. Eur J Cancer. (2018) 104:137–44. doi: 10.1016/j.ejca.2018.09.017
70. Lavolé A, Guihot A, Veyri M, Lambotte O, Autran B, Cloarec N, et al. PD-1 blockade in HIV-infected patients with lung cancer: a new challenge or already a strategy? Ann Oncol. (2018) 29:1065–6. doi: 10.1093/annonc/mdx817
73. Polizzotto MN, Uldrick TS, Wyvill KM, Aleman K, Peer CJ, Bevans M, et al. Pomalidomide for Symptomatic Kaposi's Sarcoma in People With and Without HIV Infection: a phase I/II study. J Clin Oncol. (2016) 34:4125–31. doi: 10.1200/JCO.2016.69.3812
74. Polizzotto MN, Uldrick T, Wyvill KM, Aleman K, Peer CJ, Bevans M, et al. Pomalidomide induces expansion of activated and central memory CD4+ and CD8+ T cells in vivo in patients with and without HIV infection. Blood. (2014) 124:4128–31. doi: 10.1200/JCO.2016.69.3812t
76. Wang CC, Thanh C, Gibson EA, Ball-Burack M, Hogan LE, Descours B, et al. Transient loss of detectable HIV-1 RNA following brentuximab vedotin anti-CD30 therapy for Hodgkin lymphoma. Blood Adv. (2018) 2:3479–82. doi: 10.1182/bloodadvances.2018024364
78. Ruxrungtham K, Sirivichayakul S, Buranapraditkun S, Krause W. Alemtuzumab-induced elimination of HIV-1-infected immune cells. J Virus Erad. (2016) 2:12–8.
79. Rasmussen TA, McMahon J, Chang JJ, Symons J, Roche M, Dantanarayana A, et al. Impact of alemtuzumab on HIV persistence in an HIV-infected individual on antiretroviral therapy with Sezary syndrome. AIDS. (2017) 31:1839–45. doi: 10.1097/QAD.0000000000001540
81. Guarnera C, Bramanti P, Mazzon E. Alemtuzumab: a review of efficacy and risks in the treatment of relapsing remitting multiple sclerosis. Ther Clin Risk Manag. (2017) 13:871–9. doi: 10.2147/TCRM.S134398
82. Mackall CL, Fry TJ, Gress RE. Harnessing the biology of IL-7 for therapeutic application. Nat Rev Immunol. (2011) 11:330–42. doi: 10.1038/nri2970
83. Wang C, Edilova MI, Wagar LE, Mujib S, Singer M, Bernard NF, et al. Effect of IL-7 Therapy on phospho-ribosomal protein S6 and TRAF1 expression in HIV-specific CD8 T cells in patients receiving antiretroviral therapy. J Immunol. (2018) 200:558–64. doi: 10.4049/jimmunol.1601254
84. Logerot S, Rancez M, Charmeteau-de Muylder B, Figueiredo-Morgado S, Rozlan S, Tambussi G, et al. HIV reservoir dynamics in HAART-treated poor immunological responder patients under IL-7 therapy. AIDS. (2018) 32:715–20. doi: 10.1097/QAD.0000000000001752
85. Fry TJ, Connick E, Falloon J, Lederman MM, Liewehr DJ, Spritzler J, et al. A potential role for interleukin-7 in T-cell homeostasis. Blood. (2001) 97:2983–90. doi: 10.1182/blood.V97.10.2983
86. Managlia EZ, Landay A, Al-Harthi L. Interleukin-7 induces HIV replication in primary naive T cells through a nuclear factor of activated T cell (NFAT)-dependent pathway. Virology. (2006) 350:443–52. doi: 10.1016/j.virol.2006.02.019
87. Chahroudi A, Silvestri G. Interleukin-7 in HIV pathogenesis and therapy. Eur Cytokine Netw. (2010) 21:202–7. doi: 10.1684/ecn.2010.0205
88. Le Saout C, Luckey MA, Villarino AV, Smith M, Hasley RB, Myers TG, et al. IL-7-dependent STAT1 activation limits homeostatic CD4+ T cell expansion. JCI insight (2017) 2:96228. doi: 10.1172/jci.insight.96228
89. Ponchel F, Cuthbert RJ, Goëb V. IL-7 and lymphopenia. Clin Chim Acta. (2011) 412:7–16. doi: 10.1016/j.cca.2010.09.002
90. Sportès C, Hakim FT, Memon SA, Zhang H, Chua KS, Brown MR, et al. Administration of rhIL-7 in humans increases in vivo TCR repertoire diversity by preferential expansion of naive T cell subsets. J Exp Med. (2008) 205:1701–14. doi: 10.1084/jem.20071681
92. Little RF, Pluda JM, Wyvill KM, Rodriguez-Chavez IR, Tosato G, Catanzaro AT, et al. Activity of subcutaneous interleukin-12 in AIDS-related Kaposi sarcoma. Blood. (2006) 107:4650–7. doi: 10.1182/blood-2005-11-4455
93. Vandergeeten C, Fromentin R, Chomont N. The role of cytokines in the establishment, persistence and eradication of the HIV reservoir. Cytokine Growth Factor Rev (2012) 23:143–9. doi: 10.1016/j.cytogfr.2012.05.001
94. Berard M, Brandt K, Bulfone-Paus S, Tough DF. IL-15 promotes the survival of naive and memory phenotype CD8+ T cells. J Immunol. (2003) 170:5018–26. doi: 10.4049/jimmunol.170.10.5018
95. Mueller YM, Katsikis PD. IL-15 in HIV infection: pathogenic or therapeutic potential? Eur Cytokine Netw. (2010) 21:219–21.
96. Garrido C, Abad-Fernandez M, Tuyishime M, Pollara JJ, Ferrari G, Soriano-Sarabia N, et al. Interleukin-15-stimulated natural killer cells clear HIV-1-infected cells following latency reversal ex vivo. J Virol. (2018) 92:e00235–18. doi: 10.1128/JVI.00235-18
97. Xu A, Bhanumathy KK, Wu J, Ye Z, Freywald A, Leary SC, et al. IL-15 signaling promotes adoptive effector T-cell survival and memory formation in irradiation-induced lymphopenia. Cell Biosci (2016) 6:30. doi: 10.1186/s13578-016-0098-2
98. Krown SE, Li P, Von Roenn JH, Paredes J, Huang J, Testa MA, et al. Efficacy of low-dose interferon with antiretroviral therapy in Kaposi's sarcoma: a randomized phase II AIDS clinical trials group study. J Interferon Cytokine Res. (2002) 22:295–303. doi: 10.1089/107999002753675712
99. Shepherd FA, Beaulieu R, Gelmon K, Thuot CA, Sawka C, Read S, et al. Prospective randomized trial of two dose levels of interferon alfa with zidovudine for the treatment of Kaposi's sarcoma associated with human immunodeficiency virus infection: a Canadian HIV Clinical Trials Network study. J Clin Oncol. (1998) 16:1736–42. doi: 10.1200/JCO.1998.16.5.1736
100. Krown SE, Gold JW, Niedzwiecki D, Bundow D, Flomenberg N, Gansbacher B, et al. Interferon-alpha with zidovudine: safety, tolerance, and clinical and virologic effects in patients with Kaposi sarcoma associated with the acquired immunodeficiency syndrome (AIDS). Ann Inten Med. (1990) 112:812–21. doi: 10.7326/0003-4819-112-11-812
101. Strauss J, Heery CR, Kim JW, Jochems C, Donahue RN, Montgomery AS, et al. First-in-human phase i trial of a tumor-targeted cytokine (NHS-IL12) in subjects with metastatic solid tumors. Clin Cancer Res. (2019) 25:99–109. doi: 10.1158/1078-0432.CCR-18-1512
102. Pfreundschuh M, Kuhnt E, Trümper L, Osterborg A, Trneny M, Shepherd L, et al. CHOP-like chemotherapy with or without rituximab in young patients with good-prognosis diffuse large-B-cell lymphoma: 6-year results of an open-label randomised study of the MabThera International Trial (MInT) Group. Lancet Oncol. (2011) 12:1013–22. doi: 10.1016/S1470-2045(11)70235-2
103. Coiffier B, Thieblemont C, Van Den Neste E, Lepeu G, Plantier I, Castaigne S, et al. Long-term outcome of patients in the LNH-98.5 trial, the first randomized study comparing rituximab-CHOP to standard CHOP chemotherapy in DLBCL patients: a study by the Groupe d'Etudes des Lymphomes de l'Adulte. Blood. (2010) 116:2040–5. doi: 10.1182/blood-2010-03-276246
104. Coiffier B, Lepage E, Briere J, Herbrecht R, Tilly H, Bouabdallah R, et al. CHOP chemotherapy plus rituximab compared with CHOP alone in elderly patients with diffuse large-B-cell lymphoma. N Engl J Med. (2002) 346:235–42. doi: 10.1056/NEJMoa011795
105. Barta SK, Xue X, Wang D, Tamari R, Lee JY, Mounier N, et al. Treatment factors affecting outcomes in HIV-associated non-Hodgkin lymphomas: a pooled analysis of 1546 patients. Blood. (2013) 122:3251–62. doi: 10.1182/blood-2013-04-498964
106. Rubinstein PG, Moore PC, Rudek MA, Henry DH, Ramos JC, Ratner L, et al. Brentuximab vedotin with AVD shows safety, in the absence of strong CYP3A4 inhibitors, in newly diagnosed HIV-associated Hodgkin lymphoma. AIDS. (2018) 32:605–11. doi: 10.1097/QAD.0000000000001729
107. Sundar R, Cho BC, Brahmer JR, Soo RA. Nivolumab in NSCLC: latest evidence and clinical potential. Ther Adv Med Oncol. (2015) 7:85–96. doi: 10.1177/1758834014567470
108. Brahmer JR, Tykodi SS, Chow LQ, Hwu WJ, Topalian SL, Hwu P, et al. Safety and activity of anti–PD-L1 antibody in patients with advanced cancer. N Engl J Med. (2012) 366:2455–65. doi: 10.1056/NEJMoa1200694
109. Uldrick TS, Ison G, Rudek MA, Noy A, Schwartz K, Bruinooge S, et al. Modernizing clinical trial eligibility criteria: recommendations of the american society of clinical oncology-friends of cancer research HIV working group. J Clin Oncol. (2017) 35:3774–80. doi: 10.1200/JCO.2017.73.7338
110. Sandoval-Sus JD, Mogollon-Duffo F, Patel A, Visweshwar N, Laber DA, Kim R, et al. Nivolumab as salvage treatment in a patient with HIV-related relapsed/refractory Hodgkin lymphoma and liver failure with encephalopathy. J Immunother Cancer. (2017) 5:49. doi: 10.1186/s40425-017-0252-3
111. Davar D, Wilson M, Pruckner C, Kirkwood JM. PD-1 blockade in advanced melanoma in patients with hepatitis C and/or HIV. Case Rep Oncol Med. (2015) 2015:737389. doi: 10.1155/2015/737389
112. Husnain M, Park W, Ramos JC, Johnson TE, Chan J, Dasari A, et al. Complete response to ipilimumab and nivolumab therapy in a patient with extensive extrapulmonary high-grade small cell carcinoma of the pancreas and HIV infection. J Immunother Cancer. (2018) 6:66. doi: 10.1186/s40425-018-0379-x
113. Burke MM, Kluger HM, Golden M, Heller KN, Hoos A, Sznol M. Case Report: response to ipilimumab in a patient with HIV with metastatic melanoma. J ClinOncol. (2011) 29:e792–4. doi: 10.1200/JCO.2011.36.9199
114. Spano JP, Veyri M, Gobert A, Guihot A, Perré P, Kerjouan M, et al. Immunotherapy for cancer in people living with HIV: safety with an efficacy signal from the series in real life experience on behalf of the French CANCERVIH network. AIDS. (2019) 33:F13–9. doi: 10.1097/QAD.0000000000002298
115. Chang E, Sabichi AL, Kramer JR, Hartman C, Royse KE, White DL, et al. Nivolumab treatment for cancers in the HIV-infected population. J Immunother. (2018) 41:379–83. doi: 10.1097/CJI.0000000000000240
116. Evans VA, van der Sluis RM, Solomon A, Dantanarayana A, McNeil C, Garsia R, et al. Programmed cell death-1 contributes to the establishment and maintenance of HIV-1 latency. AIDS. (2018) 32:1491–7. doi: 10.1097/QAD.0000000000001849
117. Cook MR, Kim C. Safety and efficacy of immune checkpoint inhibitor therapy in patients with HIV infection and advanced-stage cancer: a systematic review. JAMA Oncol. (2019) 5:1049–54. doi: 10.1001/jamaoncol.2018.6737
118. Uldrick TS, Gonçalves PH, Abdul-Hay M, Claeys AJ, Emu B, Ernstoff MS, et al. Assessment of the safety of pembrolizumab in patients with HIV and advanced cancer-A phase 1 study. JAMA Oncol. (2019). doi: 10.1001/jamaoncol.2019.2244. [Epub ahead of print].
119. González-Cao M, Moran T, Dalmau J, Garcia-Corbacho J, Bernabé R, Juan O, et al. Phase II study of durvalumab (MEDI4736) in cancer patients HIV-1-infected. J Clin Oncol. (2019) 37:2501. doi: 10.1200/JCO.2019.37.15_suppl.2501
120. Finzi D, Hermankova M, Pierson T, Carruth LM, Buck C, Chaisson RE, et al. Identification of a reservoir for HIV-1 in patients on highly active antiretroviral therapy. Science. (1997) 278:1295–300. doi: 10.1126/science.278.5341.1295
121. Wong JK, Hezareh M, Günthard HF, Havlir DV, Ignacio CC, Spina CA, et al. Recovery of replication-competent HIV despite prolonged suppression of plasma viremia. Science. (1997) 278:1291–5. doi: 10.1126/science.278.5341.1291
122. Chun TW, Stuyver L, Mizell SB, Ehler LA, Mican JA, Baseler M, et al. Presence of an inducible HIV-1 latent reservoir during highly active antiretroviral therapy. Proc Natl Acad Sci USA. (1997) 94:13193–7. doi: 10.1073/pnas.94.24.13193
123. Simonetti FR, Sobolewski MD, Fyne E, Shao W, Spindler J, Hattori J, et al. Clonally expanded CD4+ T cells can produce infectious HIV-1 in vivo. Proc Natl Acad Sci USA. (2016) 113:1883–8. doi: 10.1073/pnas.1522675113
124. Wagner TA, McLaughlin S, Garg K, Cheung CYK, Larsen BB, Styrchak S, et al. HIV latency. Proliferation of cells with HIV integrated into cancer genes contributes to persistent infection. Science. (2014) 345:570–3. doi: 10.1126/science.1256304
125. Schröder ARW, Shinn P, Chen H, Berry C, Ecker JR, Bushman F. HIV-1 integration in the human genome favors active genes and local hotspots. Cell. (2002) 110:521–9. doi: 10.1016/S0092-8674(02)00864-4
126. Haworth KG, Schefter LE, Norgaard ZK, Ironside C, Adair JE, Kiem HP. HIV infection results in clonal expansions containing integrations within pathogenesis-related biological pathways. JCI insight. (2018) 3:99127. doi: 10.1172/jci.insight.99127
127. Maldarelli F, Wu X, Su L, Simonetti FR, Shao W, Hill S, et al. HIV latency. Specific HIV integration sites are linked to clonal expansion and persistence of infected cells. Science. (2014) 345:179–83. doi: 10.1126/science.1254194
128. Wightman F, Solomon A, Kumar SS, Urriola N, Gallagher K, Hiener B, et al. Effect of ipilimumab on the HIV reservoir in an HIV-infected individual with metastatic melanoma. AIDS. (2015) 29:504–6. doi: 10.1097/QAD.0000000000000562
129. Offersen R, Nissen SK, Rasmussen TA, Østergaard L, Denton PW, Søgaard OS, et al. Pembrozlizumab Induces HIV latency reversal in HIV+ Individuals on ART with Cancer. In: Conference on Retroviruses and Opportunistic Infections (CROI). Seattle, WA (2019). p. Abstract 27.
130. Hentrich M, Schipek-Voigt K, Jäger H, Schulz S, Schmid P, Stötzer O, et al. Nivolumab in HIV-related non-small-cell lung cancer. Ann Oncol. (2017) 28:2890. doi: 10.1093/annonc/mdx321
131. McCullar B, Alloway T, Martin M. Durable complete response to nivolumab in a patient with HIV and metastatic non-small cell lung cancer. J Thorac Dis. (2017) 9:E540–2. doi: 10.21037/jtd.2017.05.32
132. Le Garff G, Samri A, Lambert-Niclot S, Even S, Lavolé A, Cadranel J, et al. Transient HIV-specific T cells increase and inflammation in an HIV-infected patient treated with nivolumab. AIDS. (2017) 31:1048–51. doi: 10.1097/QAD.0000000000001429
133. Macatangay BJ, Rinaldo CR. PD-1 blockade: a promising immunotherapy for HIV? Cell Sci. (2009) 5:61–5.
134. Chomont N, El-Far M, Ancuta P, Trautmann L, Procopio FA, Yassine-Diab B, et al. HIV reservoir size and persistence are driven by T cell survival and homeostatic proliferation. Nat Med. (2009) 15:893–900. doi: 10.1038/nm.1972
135. Fromentin R, DaFonseca S, Costiniuk CT, El-Far M, Procopio FA, Hecht FM, et al. PD-1 blockade potentiates HIV latency reversal ex vivo in CD4(+) T cells from ART-suppressed individuals. Nat Commun. (2019) 10:814. doi: 10.1038/s41467-019-08798-7
136. Banga R, Procopio FA, Noto A, Pollakis G, Cavassini M, Ohmiti K, et al. PD-1(+) and follicular helper T cells are responsible for persistent HIV-1 transcription in treated aviremic individuals. Nat Med. (2016) 22:754–61. doi: 10.1038/nm.4113
137. Guihot A, Marcelin AG, Massiani MA, Samri A, Soulié C, Autran B, et al. Drastic decrease of the HIV reservoir in a patient treated with nivolumab for lung cancer. Ann Oncol. (2018) 29:517–8. doi: 10.1093/annonc/mdx696
138. Colston E, Grasela D, Gardiner D, Bucy RP, Vakkalagadda B, Korman AJ, et al. An open-label, multiple ascending dose study of the anti-CTLA-4 antibody ipilimumab in viremic HIV patients. PLoS ONE. (2018) 13:e0198158. doi: 10.1371/journal.pone.0198158
139. Biswas P, Smith CA, Goletti D, Hardy EC, Jackson RW, Fauci AS. Cross-linking of CD30 induces HIV expression in chronically infected T cells. Immunity. (1995) 2:587–96. doi: 10.1016/1074-7613(95)90003-9
140. Hogan LE, Vasquez J, Hobbs KS, Hanhauser E, Aguilar-Rodriguez B, Hussien R, et al. Increased HIV-1 transcriptional activity and infectious burden in peripheral blood and gut-associated CD4+ T cells expressing CD30. PLoS Pathog. (2018) 14:e1006856. doi: 10.1371/journal.ppat.1006856
141. Gupta RK, Abdul-Jawad S, McCoy LE, Mok HP, Peppa D, Salgado M, et al. HIV-1 remission following CCR5Delta32/Delta32 haematopoietic stem-cell transplantation. Nature. (2019) 568:244–8. doi: 10.1038/s41586-019-1027-4
142. Hodge JN, Srinivasula S, Hu Z, Read SW, Porter BO, Kim I, et al. Decreases in IL-7 levels during antiretroviral treatment of HIV infection suggest a primary mechanism of receptor-mediated clearance. Blood. (2011) 118:3244–53. doi: 10.1182/blood-2010-12-323600
143. Lévy Y, Sereti I, Tambussi G, Routy JP, Lelièvre JD, Delfraissy JF, et al. Effects of recombinant human interleukin 7 on T-cell recovery and thymic output in HIV-infected patients receiving antiretroviral therapy: results of a phase I/IIa randomized, placebo-controlled, multicenter study. Clin Infect Dis. (2012) 55:291–300. doi: 10.1093/cid/cis383
144. Mueller YM, Bojczuk PM, Halstead ES, Kim AH, Witek J, Altman JD, et al. IL-15 enhances survival and function of HIV-specific CD8+ T cells. Blood. (2003) 101:1024–9. doi: 10.1182/blood-2002-07-1957
145. Hasan MS, Kallas EG, Thomas EK, Looney J, Campbell M, Evans TG. Effects of interleukin-15 on in vitro human T cell proliferation and activation. J Interferon Cytokine Res. (2000) 20:119–23. doi: 10.1089/107999000312513
146. Hütter G, Nowak D, Mossner M, Ganepola S, Müssig A, Allers K, et al. Long-term control of HIV by CCR5 Delta32/Delta32 stem-cell transplantation. N Engl J Med. (2009) 360:692–8. doi: 10.1056/NEJMoa0802905
147. Hutter G, Ganepola S. Eradication of HIV by transplantation of CCR5-deficient hematopoietic stem cells. Sci World J. (2011) 11:1068–76. doi: 10.1100/tsw.2011.102
148. Salgado M, Kwon M, Gálvez C, Badiola J, Nijhuis M, Bandera A, et al. Mechanisms that contribute to a profound reduction of the HIV-1 reservoir after allogeneic stem cell transplant. Ann Intern Med. (2018) 169:674–83. doi: 10.7326/M18-0759
149. Henrich TJ, Hanhauser E, Marty FM, Sirignano MN, Keating S, Lee TH, et al. Antiretroviral-free HIV-1 remission and viral rebound after allogeneic stem cell transplantation: report of 2 cases. Ann Intern Med. (2014) 161:319–27. doi: 10.7326/M14-1027
150. Macpherson JL, Boyd MP, Arndt AJ, Todd AV, Fanning GC, Ely JA, et al. Long-term survival and concomitant gene expression of ribozyme-transduced CD4+ T-lymphocytes in HIV-infected patients. J Gene Med. (2005) 7:552–64. doi: 10.1002/jgm.705
151. Mitsuyasu RT, Merigan TC, Carr A, Zack JA, Winters MA, Workman C, et al. Phase 2 gene therapy trial of an anti-HIV ribozyme in autologous CD34+ cells. Nat Med. (2009) 15:285–92. doi: 10.1038/nm.1932
152. Burnett JC, Zaia JA, Rossi JJ. Creating genetic resistance to HIV. Curr Opin Immunol. (2012) 24:625–32. doi: 10.1016/j.coi.2012.08.013
153. Durand CM, Blankson JN, Siliciano RF. Developing strategies for HIV-1 eradication. Trends Immunol. (2012) 33:554–62. doi: 10.1016/j.it.2012.07.001
Keywords: HIV, immunotherapy, HIV reservoir, cancer, PD-1, Kaposi sarcoma, lymphoma
Citation: Puronen CE, Ford ES and Uldrick TS (2019) Immunotherapy in People With HIV and Cancer. Front. Immunol. 10:2060. doi: 10.3389/fimmu.2019.02060
Received: 15 May 2019; Accepted: 15 August 2019;
Published: 28 August 2019.
Edited by:
Steven Grant Deeks, University of California, San Francisco, United StatesReviewed by:
Zong Sheng Guo, University of Pittsburgh, United StatesChristine Marie Durand, The Johns Hopkins Hospital, United States
Copyright © 2019 Puronen, Ford and Uldrick. This is an open-access article distributed under the terms of the Creative Commons Attribution License (CC BY). The use, distribution or reproduction in other forums is permitted, provided the original author(s) and the copyright owner(s) are credited and that the original publication in this journal is cited, in accordance with accepted academic practice. No use, distribution or reproduction is permitted which does not comply with these terms.
*Correspondence: Thomas S. Uldrick, tuldrick@fredhutch.org