- 1Department of Traumatology, Hand, Plastic, and Reconstructive Surgery, Center of Surgery, University of Ulm, Ulm, Germany
- 2Institute of Orthopedic Research and Biomechanics, University of Ulm, Ulm, Germany
- 3Department of Internal Medicine I, University of Ulm, Ulm, Germany
- 4Institute of General Physiology, University of Ulm, Ulm, Germany
- 5Department of Trauma, Hand and Reconstructive Surgery, Goethe University Frankfurt, Frankfurt, Germany
- 6Department of Trauma, University Hospital of Zurich, Zurich, Switzerland
- 7Department of Surgical Research, University Hospital of Zurich, Zurich, Switzerland
Background and Purpose: Post-traumatic cardiac dysfunction often occurs in multiply injured patients (ISS ≥ 16). Next to direct cardiac injury, post-traumatic cardiac dysfunction is mostly induced by the release of inflammatory biomarkers. One of these is the heparin-binding factor Midkine, which is elevated in humans after fracture, burn injury and traumatic spinal cord injury. Midkine is associated with cardiac pathologies but the exact role of Midkine in the development of those diseases is ambiguous. The systemic profile of Midkine after multiple trauma, its effects on cardiomyocytes and the association with post-traumatic cardiac dysfunction, remain unknown.
Experimental Approach: Midkine levels were investigated in blood plasma of multiply injured humans and pigs. Furthermore, human cardiomyocytes (iPS) were cultured in presence/absence of Midkine and analyzed regarding viability, apoptosis, calcium handling, metabolic alterations, and oxidative stress. Finally, the Midkine filtration capacity of the therapeutic blood absorption column CytoSorb ®300 was tested with recombinant Midkine or plasma from multiply injured patients.
Key Results: Midkine levels were significantly increased in blood plasma of multiply injured humans and pigs. Midkine acts on human cardiomyocytes, altering their mitochondrial respiration and calcium handling in vitro. CytoSorb®300 filtration reduced Midkine concentration ex vivo and in vitro depending on the dosage.
Conclusion and Implications: Midkine is elevated in human and porcine plasma after multiple trauma, affecting the functionality and metabolism of human cardiomyocytes in vitro. Further examinations are required to determine whether the application of CytoSorb®300 filtration in patients after multiple trauma is a promising therapeutic approach to prevent post-traumatic cardiac disfunction.
Introduction
According to the World Health Organization (WHO), trauma accounts for 10% of deaths and 16% of disabilities worldwide (1). Multiple trauma in humans (Injury Severity Score, ISS ≥ 16) are characterized by a massive release of different inflammatory biomarkers, such as cytokines, and damage associated molecular patterns (DAMPs). This damage affects different organs of the body and can trigger whole-body inflammation after trauma (2, 3). A substantial release of these trauma-dependent molecules is associated with the development of the so-called systemic inflammatory response syndrome (SIRS) and the multiple organ dysfunction syndrome (MODS), which are both associated with an increased mortality (4, 5). Many of the released inflammatory cytokines and DAMPs were recently shown to be cardio-depressive by acting on cardiomyocytes (CMs), altering their calcium handling, redox balance, signaling transduction, and finally resulting in post-traumatic cardiac dysfunction (6, 7). One inflammatory cytokine is the heparin-binding growth- and differentiation factor Midkine (Mdk). Increased Mdk expression is associated with different traumatic conditions such as bone fracture, burn injury, traumatic spinal cord injury, and sepsis (8–11). Increased Mdk in human blood can persist for overall 42 days after fracture (11). Furthermore, Mdk impairs fracture healing by reducing bone formation and increasing neutrophil infiltration during the fracture healing process (12, 13). However, the trauma-dependent elevation of Mdk in multiply injured patients as well as the exact impact of Mdk on the heart after trauma remains unclear. In patients with chronic heart failure, circulating Mdk increases significantly and is regarded as a novel marker, predicting different cardiac events (14, 15). Moreover, Mdk plays a role in ischemic heart injury, myocardial infarction and cardiac hypertrophy (16–18). Nevertheless, the function of Mdk in these different pathologies is still controversial, because in some cases such as ischemic heart injury, chronic heart failure and myocardial infarct, Mdk has positive effects by improving cell survival and cardiac function, inducing angiogenesis and reducing detrimental remodeling (17, 19, 20). In contrast, Mdk reduces cellular survival and induces pathological remodeling as well as fibrosis in patients with cardiac hypertrophy (18). Consequently, the exact effect of Mdk on the heart is ambiguous since Mdk can have beneficial and detrimental effects in cardiac pathology. The function of Mdk as an inflammatory cytokine on the heart during trauma especially requires clarification. After all, Mdk might be a potential therapeutic option in cardiac diseases as well as in the treatment and prevention of post-traumatic cardiac injury (21, 22). Mdk has been shown to play an important role in active myocarditis in patients and in experimental autoimmune myocarditis in mice (23). In these instances, Mdk promotes the recruitment of polymorphonuclear neutrophils (PMNs) and the production of neutrophil extracellular traps (NETs) in cardiac tissues, resulting in impaired systolic function (23). Increased activation and recruitment of neutrophils in cardiac tissue were also observed in humans after trauma and in experimental blunt chest trauma models in rats. In addition, it is linked to increased systemic levels of extracellular histones by NETosis, leading to cardiac dysfunction (24, 25).
In this study, we investigate the Mdk elevation in blood circulation after multiple trauma in pigs and humans. We further aim to thoroughly examine the effects of Mdk on human CMs. With regards to therapeutic options for posttraumatic cardiac dysfunction, the study aims to investigate the usage of CytoSorb® 300 hemadsorption. In clinical settings, CytoSorb® 300 hemadsorption improved the outcome of patients with endotoxemia, necrotizing fasciitis, septic shock, and cardiac surgery (26–29). Furthermore, CytoSorb® hemadsorption resulted in immediate hemodynamic stabilization and increased survival rates in patients with multiple organ failure (30). CytoSorb® 300 consists of highly porous (styrene-co-divinylbenzene) hemadsorbent polymer beads, which can remove substances within 10–60 kDa of molecular weight, such as complement factor 5a, cytokines DAMPs and pathogen associated molecular patterns (PAMPs), from circulating blood (26, 31). Similarly, the high-mobility group box 1 protein (HMGB1) can be removed from blood in a time dependent manner (31). Lastly, the study examines the capacity of CytoSorb® 300 to filtrate Mdk, which may be used as a therapeutic approach for preventing and handling post-traumatic cardiac dysfunction.
Materials and Methods
Human Blood Samples
Human plasma from 11 multiply injured patients with a history of acute blunt or penetrating trauma and an ISS ≥ 16 was collected after hospital admission in the University Hospital of the Goethe-University Frankfurt with institutional ethics committee approval (312/10), in accordance with the Declaration of Helsinki and following the Strengthening the Reporting of Observational studies in Epidemiology (STROBE)-guidelines (32). All enrolled patients either signed the written informed consent form or written informed consent was obtained from the nominated legally authorized representative of the participants in accordance with ethical standards. Exclusion criteria were the patients being younger than 18 or older than 80 years, presenting severe burn injury, acute myocardial stroke, cancer or chemotherapy, immunosuppressive drug therapy, HIV, infectious Hepatitis, acute CMV infection, and/or thromboembolic events. Control blood samples were collected from healthy volunteers (n = 6, 50:50 female male, no comorbidities). Randomization of the groups was not possible during the sample collection. Blood samples were withdrawn in ethylenediaminetetraacetic acid (EDTA) tubes (Sarstedt, Nürmbrecht, Germany) directly after admission. The samples were kept on ice until centrifugation at 2,100 g for 15 min. Then, the supernatant was collected and stored at −80°C until assay.
Animals
This study presents partial results obtained from a large animal porcine multiple trauma model, conducted by the TREAT research group.
The animal housing and experimental protocols were approved by the Cantonal Veterinary Department, Zurich, Switzerland, under license no. ZH 138/2017, and were in accordance with Swiss Animal Protection Law. Housing and experimental procedures also conformed to the European Directive 2010/63/EU of the European Parliament and of the Council on the Protection of vertebrate animals used for scientific purposes (Council of Europe no. 123, Strasbourg 1985) and to the Guide for the Care and Use of Laboratory Animals (Institute of Laboratory Animal Resources, National Research Council, National Academy of Sciences, 2011). Twenty-five male pigs weighting 50 ± 5 kg (Sus scrofa domestica) were included in the study (mean height, snout-tail length: 123,6 cm). Animals were held in a controlled environment with 21 ± 3°C room temperature (50% humidity), with a light/dark cycle of 12 h. Water was available for animals ad libitum. General instrumentation, anesthesia and trauma induction were described previously by Horst et al. (33).
Analgesia and Anesthesia
For premedication, pigs received an intramuscular injection with ketamine (20 mg/kg body weight), azaperone (1–2 mg/kg body weight) and atropine (0.1–0.2 mg/kg body weight). Anesthesia was performed by intravenous application of propofol (2,6-diisopropylphenol) (1–2 mg/kg body weight). Anesthesia was maintained during the study period with propofol (5–10 mg/kg/h). Pain medication was ensured by sufentanyl (1 μg/kg/h) perfusion over the whole observation period.
Multiple Trauma in Pigs
Analgesia and Anesthesia of the animals was maintained during the whole procedure.
Pigs underwent either multiple trauma (n = 20) or sham-procedure (n = 5). Multiple trauma includes a combination of a penetrating thorax trauma, laparotomy, liver laceration, femur fracture, and hemorrhagic shock (ISS ≥ 27). Control animals underwent sham-procedure (n = 5). Femur fracture was induced by a bolt gun (Blitz-Kernen, turbocut JOBB GmbH, Germany), positioned on the mid third of the left femur. The gun was loaded with cattle-killing cartridges (9 x 17; DynamitNobel AG, Troisdorf, Germany). For introduction of blunt chest trauma, a pair of panels (steel 0.8 cm, lead 1.0 cm thickness) was placed on the right dorsal lower chest. A shock wave was induced by a bolt shot (Blitz-Kerner, turbocut JOBB GmbH, Germany), which was applied onto the panel using cattle-killing cartridges as previously described (34, 35). Midline-laparotomy was performed by exploring the right upper liver lobe. Penetrating hepatic injury was induced by cross-like incision halfway through the liver tissue. After a short period of uncontrolled bleeding (30 s), liver package was performed. Directly after the hepatic package, pressure-controlled and volume-limited hemorrhagic shock was induced by withdrawing blood until a mean arterial pressure (MAP) of 30 ± 5 mm Hg was reached. Maximal withdrawal amounts to 45% of total blood volume. The reached MAP was maintained for 60 min. At the end of the shock period, animals were resuscitated according to established trauma guidelines (ATLS®, AWMF-S3 guideline on Treatment of Patients with Severe and Multiple Injuries®) by adjusting FiO2 and an initial substitution of the withdrawn blood volume with Ringerfundin, fluid maintenance was performed by continuous infusing additional fluids (Ringerfundin, 2 ml/kg body weight/h). Moreover, pigs were rewarmed until normothermia (38.7–39.8°C) was reached. Sham procedure (n = 5) included instrumentation and anesthesia but without trauma or hemorrhage. The multiple trauma group (n = 20) was randomized in four therapy arms: pigs received either femoral nailing without reaming (n = 5), standard reaming (n = 5), reamed irrigation and aspiration (RIA I) (n = 5) or reamed irrigation and aspiration with reduced diameter and improved control of irrigation and suction (RIA II) (n = 5). In all groups a shortened conventional tibia nail was introduced.
Follow-Up and Euthanasia
Hemodynamic parameters were continuously monitored for 6 h. Pigs were euthanized under deep general anesthesia with intravenous Na-Pentobarbital.
This animal model represents a clinically relevant porcine model of severe multiple trauma (pulmonary contusion, extremity injury, liver laceration) with post-traumatic observation period under ICU conditions (33).
Sample Collection
Serum and plasma samples were collected at baseline, 4 and 6 h after multiple trauma and kept on ice. After centrifugation (1,500 g for 12 min at 4°C), serum and EDTA-plasma were removed and stored at −80°C until analysis. Heart tissue samples were obtained 6 h after resuscitation. Tissue of the superficial and the luminal left ventricle was fixed with 4% formalin, followed by embedding in paraffin. Furthermore, tissue was quick-frozen in liquid nitrogen, followed by storage at −80°C until analysis.
Midkine ELISA
For determination of Midkine in human and porcine plasma, as well as for the CytoSorb® 300 experiments, the human Midkine ELISA (R&D Systems, McKinley, MN, USA) was used. All procedures were performed according to manufacturers' instructions. Midkine ELISA was performed by a blinded investigator. Human plasma samples were diluted 1:4 and porcine plasma samples were diluted 1:2.
ips-Cardiomyocyte Cell Culture
Human cardiomyocytes (iPS) (Cellular Dynamics, Madison, WI, USA) were cultured for 10 days in maintenance medium at 37°C and in an atmosphere of 7% CO2, according to manufacturers' recommendations.
Binding Analysis of FITC-Labeled Midkine
Fluorescein isothiocyanate (FITC) (Sigma Aldrich, St. Louis, MO, USA) was dissolved in DMSO. Two mg/ml Midkine (Dianova, Hamburg, Germany), dissolved in 0.1 M NaHCO3 were added to 3 mg/ml fluorescein isothiocyanate (FITC) (Sigma Aldrich, St. Louis, MO, USA) solution and were incubated for 1 h at RT while continuously shaking. Unbound FITC was removed by using SnakeSkin® dialysis tube (ThermoScientific, Waltham, MA, USA). For dialysis, 1X phosphate buffered saline was used. Human CMs were seeded at a density of 6.3 × 104 cells/cm2 on ibidi 12-well chamber slides (ibidi, Germany). Afterwards, cells were incubated for 30 and 60 min with 100 ng/ml FITC-labeled Midkine. Cells were washed, fixed with 4% formalin and cell nuclei were counterstained using Hoechst (Sigma Aldrich, St. Louis, MO, USA). Cells were mounted with ProLong® Gold Antifade Mountant (ThermoScientific, Waltham, MA, USA). Cells were analyzed by blinded investigator by using Axio Imager M.2 microscope (Zeiss, Jena, Germany) and the Zeiss ZEN 2.3 software (Zeiss, Jena, Germany). Images were performed with 40x magnification (N.A. 0.75).
Cell Viability Assay
Cell viability was analyzed using Cell Titer-Glo® Luminescent Cell Viability Assay (Promega, Madison, WI, USA). Cells were seeded with a density of 6.3 × 104 cells/cm2 on a 96-well plate and treated with different Midkine concentrations (0.05, 0.1, 1 μg/ml) for 3 h, or with 1 μg/ml for different incubation times (0.5, 1, or 3 h). All procedures were performed according to manufacturers' instructions. For all experiments n = 6.
Troponin I ELISA
Human CMs were seeded with a density of 6.3 × 104 cells/cm2 on a 24-well plate and treated for 6 h with 100 ng/ml Midkine at 37°C and 7% CO2. Supernatant was collected and troponin I in supernatant was determined by using Human Cardiac Troponin I ELISA (Abcam, Cambridge, UK). All procedures were performed according to manufacturers' instructions. For all experiments n = 6.
Caspase-3/7 Assay
Human cardiomyocytes were seeded with a density of 6.3 × 104 cells/cm2 on a 96-well plate and treated with 100 ng/ml Midkine for 6 h at 37°C. Caspase-3/7 activity in human cardiomyocytes was examined by using Caspase-Glo® 3/7 Assay (Promega, Madison, WI, USA). All procedures were performed according to manufacturers' instructions. For all experiments n = 6.
Live Cell Imaging
Live cell imaging was performed using Leica Microscope SP8 and LAS X software (Leica, Wetzlar, Germany). Cells were seeded with a density of 6.3 × 104 cells/cm2 on a 96-well plate and were pre-loaded with 5 μM calcium indicator Fluo-3AM (Life Technologies, Carlsbad, CA, USA) and were incubated for 30 min at 37°C and 7% CO2. After incubation with Fluo-3AM, cells were analyzed immediately. For measurements, cells were placed in special live cell imaging chamber, adjusted at 37°C and 7% CO2. Cells were incubated with 100 ng/ml Mdk for 30 min and calcium signals were recorded and evaluated by using LAS X software. Cell culture medium was used during measurements. Live cell imaging was performed with 63x magnification (N.A. 1.2, water). Calcium peaks were determined and compared to baseline values. For all experiments n = 6.
Calcium Measurements
For calcium measurements, human cardiomyocytes (iPS) were seeded with a density of 6.3 × 104 cells/cm2 on ibidi 8-well chambers (ibidi, Germany). Before the measurements, cells were incubated with 100 ng/ml Midkine 60 min before the start of the experiments, as well as for the duration of the experiment. For measurement of changes in intracellular Ca2+ concentration, cells were loaded with 5 μM Fura-2 (ThermoScientific, Waltham, MA, USA) for 30 min (in presence of pharmacological compounds if needed). After incubation, cells were washed twice with bath solution (in mM: 140 NaCl; 5.4 KCl; MgCl2; 1.8 CaCl2; 5.5 Glucose; 5 Hepes; pH = 7.4). Fluorescence imaging was performed on a Cell Observer inverse microscope (Zeiss, Jena, Germany). Cells were illuminated for 90 min at a rate of 2 Hz at each excitation wavelength (340 and 380 nm). Images were acquired using MetaFluor (Molecular Devices, Ismaning, Germany). Cells were measured in bath solution using 40x magnification (N.A. 1.3) at room temperature. Fura-2 ratios were calculated with ImageJ and the data obtained were analyzed with the Matlab script PeakCaller (36). For all experiments n = 6.
RNA Isolation
For qPCR experiments, human CMs were seeded at a density of 6.3 × 104 cells/cm2 on a 24-well plate and were treated with 100 ng/ml Midkine for 6 h at 37°C and 7% CO2. Cells were lysed with RLY lysis buffer (Meridian Bioscience, Cincinnati, OH, USA), containing 10 μl/ml β-mercaptoethanol (Sigma Aldrich, St. Louis, MO, USA). RNA isolation from cell lysates was performed by using ISOLATE II RNA Mini Kit (Meridian Bioscience, Cincinnati, OH, USA). Remaining DNA was digested by DNase I (Meridian Bioscience, Cincinnati, OH, USA) for 15 min at RT as recommended by the manufacturer.
Reverse Transcribed Quantitative Polymerase Chain Reaction (RT-qPCR)
The respective RNA samples were reverse transcribed in cDNA using SuperScript® IV VILO® MasterMix (Life Technologies, Carlsbad, CA, USA). For cDNA transcription, 1–5 ng/ml mRNA were used, and experiment was performed according to manufacturer's instructions. For quantitative PCR, the PowerUp® SYBR® Green Master Mix (Applied Biosystems, Waltham, MA, USA) was used. All procedures were performed according to the manufacturers' instructions. For qPCR, the QuantStudio3 (Applied Biosystems, Waltham, MA, USA) system was utilized. Five-hundred to seven-hundred ng/ml cDNA were used for quantitative PCR. Quantitative mRNA expression of human troponin I (for: 5′-CCTCCAACTACCGCGCGCTTAT-3′, rev: 5′-CTGCAATTTTCTCGAGGCGG-3′), sarco/endoplasmic reticulum Ca2+-ATPase (SERCA2a) (for: 5′-CTCCTTGCCCGTGATTCTCA-3′, rev: 5′-CCAGTATTGCAGGTTCCAGGT-3′), ryanodine receptor 1 (RyR1) (for: 5′-GGGTTCCTGCCCGACATGAG-3′, rev: 5′-GCACAGGTAGCGGTTCACG-3′), Na+/Ca2+ exchanger (NCX) (for: 5′-GCCTGGTGGAGATGAGTGAG-3′, rev: 5′-ACAGGTTGGCCAAACAGGTA-3′), toll-like receptor 4 (TLR4) (for: 5′-CCTGCGTGGAGGTGTGAAAT-3′, rev: 5′-CTGGATGGGGTTTCCTGTCAA-3′), toll-like receptor 9 (TLR9) (for: 5′-AGACCTGAGGGTGGAAGTGT-3′, rev: 5′-CTGGATAGCACCAGTAGCGG-3′) and purigenic receptor subtype 7 (P2X7) (for: 5′-CACACCAAGGTGAAGGGGAT-3′, rev: 5′-GGTGTAGTCTGCGGTGTCAA-3′) was examined and calculated by the cycle threshold method ΔΔCt. Respective genes were normalized to expression of the housekeeping gene glutaraldehyde-phosphate dehydrogenase (GAPDH) (forward: 5′-TCTCTGCTCCTCCTGTTCGAC-3′, reverse: 5′-CCAATACGACCAAATCCGTTGA-3′) in order to exclude variations. Quantitative mRNA expression was determined by the double-threshold method (ΔΔCT). Results are presented as mean fold change. For all experiments n = 6.
Reactive Oxygen Species (ROS)
For analysis of cellular ROS, human CMs were seeded at a density of 6.3 × 104 cells/cm2 on ibidi 12-well slides (ibidi, Germany). Human CMs were treated with 100 ng/ml Midkine for 6 h at 37°C and 7% CO2. After treatment, cells were incubated for another 30 min with 5 μM CellROX® Deep Red Reagent (Life Technologies, Carlsbad, CA, USA) at 37°C and 7% CO2. Afterwards, cells were fixed with 4% formaldehyde and cell nuclei were stained with Hoechst. Cell were mounted with ProLong® Gold Antifade Mountant. Cells were investigated by blinded investigator by fluorescence microscopy using Axio Imager M.2 microscope and the Zeiss ZEN 2.3 software. Imaging was performed by using 20x magnification (N.A. 0.5). Relative amount of reactive oxygen species was determined by Zeiss ZEN 2.3 software in order to exclude variations. For all experiments n = 6.
Mitochondrial Respiration With Seahorse XF Analyzer
Mitochondrial respiration was analyzed by using the Seahorse XFe96 Analyzer (Agilent Technologies, Santa Clara, CA, USA). This extracellular flux analyzer makes it possible to perform highly accurate real-time measurements of cellular metabolism in living cells by simultaneously quantifying the rates of extracellular acidification (ECAR) and oxygen consumption (OCR), and measuring the glycolysis and the mitochondrial respiration of the cells. For the analysis of mitochondrial respiration, the Seahorse XF Cell Mito Stress Test Kit (Agilent Technologies, Santa Clara, CA, USA) was used. The Seahorse XF Cell Mito Stress Test Kit is an optimized solution for assessing mitochondrial function. During the experiment, the ECAR and the OCR were continuously measured, gaining the parameter for the basal (baseline) respiration of the mitochondria. Afterwards, 2 μM oligomycin, 1 μM carbonyl cyanide 4-(trifluoromethoxy) phenylhydrazone (FCCP), and 0.5 μM antimycin A and rotenone were pneumatically injected into the media of the cells. After automatically and gently mixing, the OCR and the ECAR were measured at multiple times after each injection. After the experiment, cells were fixed with 4% formalin at 4°C overnight. Then, cells were stained with 0.3% Janus-Green solution (Sigma Aldrich, St. Louis, MO, USA), washed and resolved with 0.5 M hydrochloric acid. Optical density was measured at 630 nm and OCR values were normalized to OD 630 nm values to exclude variations. Results were evaluated using Seahorse Wave 2.4 software (Agilent Technologies, Santa Clara, CA, USA), gaining the parameter for spare respiratory capacity of the mitochondria. For the analysis of mitochondrial respiration, cells were seeded with a density of 5 × 105 cells/cm2 on Seahorse XFe96 analyzer cell culture plates (Agilent Technologies, Santa Clara, CA, USA) and incubated for 6 h with 100 ng/ml Midkine and the above- mentioned procedure was performed. For all experiments n = 6.
CytoSorb® 300 Experiments
For the therapeutic experiments, the CytoSorb® 300 was used (CytosorbensInc., MonmouthJunction, NJ, USA). Therefore, small columns were prepared. An excess of CytoSorb® 300 at the ratio 2:1 (CytoSorb® 300 to plasma samples) was added on the column as recommended by the manufacturers. Human shock room blood plasma samples were added on the columns and were incubated for 6 or 3 h at RT while continuously shaking. For time-doses experiments, different Midkine concentrations (10,000, 5,000, 2,500, 2,000, 1,500, 1,000, 500, 1,000 pg/ml) diluted in PBS with 1% BSA were added on the columns and were also incubated for 6 and 3 h at RT, while continuously shaking. For all experiments n = 6.
Statistical Analysis
All values were expressed as means ± SEM. Data were analyzed by one-way ANOVA followed by Dunnett's or Tukey's multiple comparison test. For the statistical analysis of two groups, unpaired two-tailed students t-test was used. p ≤ 0.05 was considered statistically significant. GraphPad Prism 7.0 software was used for statistical analysis (GraphPad Software, Incorporated, San Diego, CA, USA).
Results
Midkine Plasma Levels in Multiply Injured Humans and Pigs
In humans as well as in pigs, the blood plasma concentrations of Midkine increased after multiple trauma compared to the healthy controls (Figures 1A,B). Animals submitted to reamed femoral nailing showed significantly higher Mdk levels when compared with pigs treated with conventional femoral nailing or with reamer irrigator aspirator treatment (RIA I/II; Figure 1B). This indicates that Mdk levels correlate with the invasiveness of the reaming method. In multiply injured pigs, plasma Mdk levels increased significantly after 6 h in the group with conventional reaming of the fracture compared to the control group.
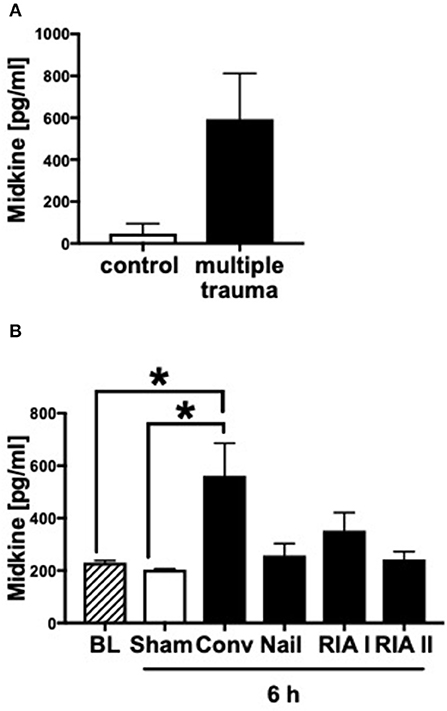
Figure 1. Midkine levels in blood plasma of multiply injured humans and pigs. Midkine levels (pg/ml) in shock room blood plasma from multiply injured patients compared to healthy control group (n = 10) (A). Midkine levels (pg/ml) in blood plasma of multiply injured pigs (B). The pigs' femur fracture was either treated with femoral nailing (nailing, n = 5), conventional (conv, n = 5), or with reamer irrigator aspirator 1 or 2 (RIA I or RIA II, each n = 5). Control animals received sham-procedure (n = 5). Evaluation of blood plasma at baseline (BL) and 6 h after trauma. Results are presented as mean ± SEM. Data were analyzed by one-way ANOVA followed by Dunnett's or Tukey's multiple comparison test. Results are significant *p < 0.05.
Since plasma Mdk levels increased after multiple trauma, we investigated whether Mdk affects human cardiomyocytes (CMs). After 30 and 60 min the Mdk was actively absorbed into the human CMs and was primarily located around their nucleus in vitro (Figure 2A).
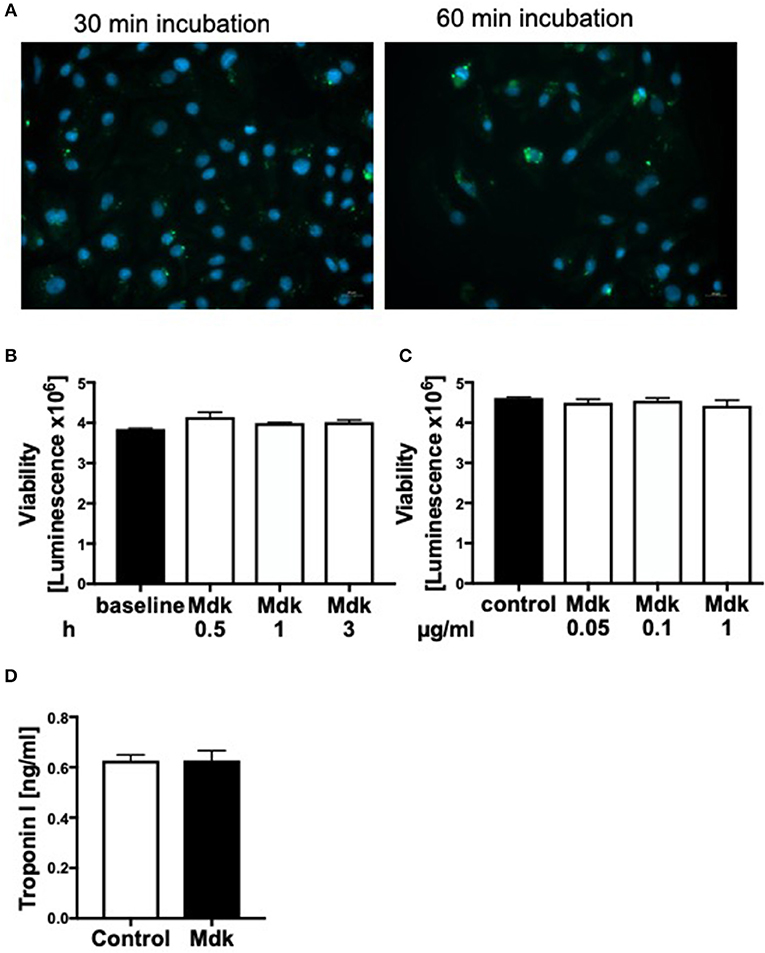
Figure 2. Effects of Midkine on human cardiomyocytes. Immunofluorescence staining of human cardiomyocytes (A). Human cardiomyocytes were treated for 30 and 60 min with 100 ng/ml fluorescein isothiocyanate (FITC)-labeled Midkine (green). Cell nuclei were counterstained with Hoechst (blue). Cell viability of human cardiomyocytes (Luminescence in counts/sec) treated for 0.5, 1, and 3 h with 100 ng/ml Midkine (B). Cell viability of human cardiomyocytes (Luminescence in counts/sec) treated for 3 h with 0.05, 0.1, and 1 μg/ml Midkine (C). Troponin I (ng/ml) in supernatant of human cardiomyocytes, treated for 6 h with 100 ng/ml Midkine (D). Results are presented as mean ± SEM. For all experiments n = 6. Data were analyzed by two-tailed, unpaired students t-test.
Cell Viability, Cell Damage, and Calcium Handling of Human Cardiomyocytes
Given that Mdk is actively taken into the cells, we examined whether it then affects the cell viability of the human CMs. The cell viability of the human CMs was neither affected by different Mdk concentrations nor by different incubation times (Figures 2B,C). Furthermore, there were no differences in troponin I concentrations in supernatant of the humans CMs treated with Mdk compared to control cells after 6 h (Figure 2D). However, the calcium handling of the human CMs was altered after Mdk treatment, which is exemplified by the significant increase in their delta calcium peaks (Figure 3A), meaning the cells beat slower in presence of Mdk. Moreover, the frequency of calcium signals in human CMs decreased significantly in presence of Mdk, developing bradycardic conditions (Figure 3B), which is also demonstrated the traces of the calcium signals of the cells (Figures 3C–F).
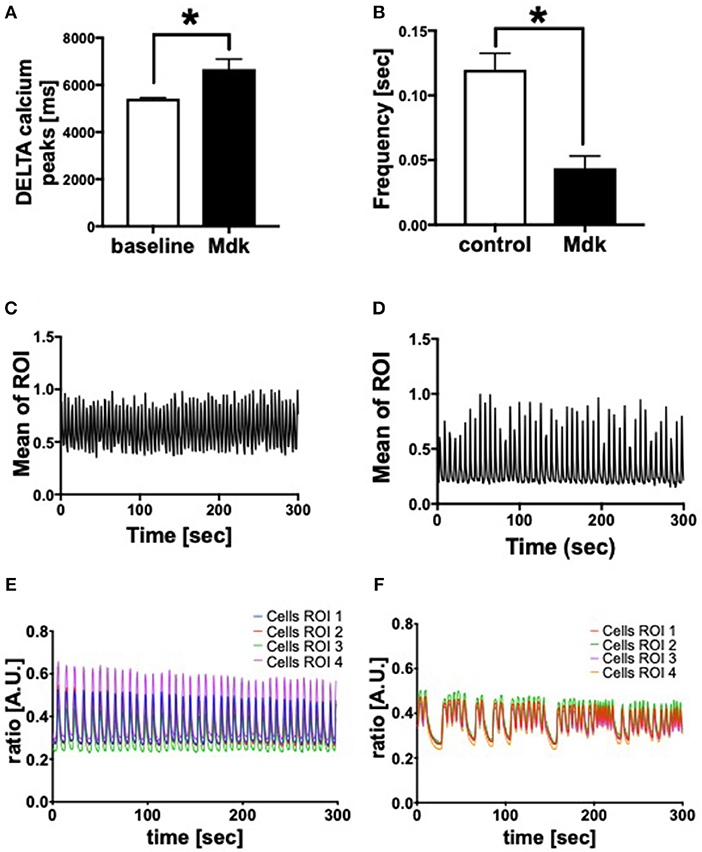
Figure 3. Calcium handling of human cardiomyocytes. Delta calcium peaks (msec) of human cardiomyocytes treated for 30 min with 100 ng/ml Midkine (A). Frequency of calcium signals (sec) of human cardiomyocytes treated for 60 min with 100 ng/ml Midkine (B). Traces of calcium signals of human cardiomyocytes (Mean Calcium peaks of ration of interest (ROI) vs. time in sec) (C,D). Traces of calcium signals of human cardiomyocytes (Ratio of Fura-2 signals in A.U. vs. time in sec) (E,F). Different colors for selected ROI of calcium signals of the cells. For all experiments n = 6. Results are presented as mean ± SEM. Data were analyzed by one-way ANOVA followed by Dunnett's or Tukey's multiple comparison test. Results are significant *p < 0.05.
Gene Expression of Human Cardiomyocytes
We showed that Mdk alters the calcium handling in human CMs. Next, we investigated the gene expression of specific cardiac calcium pumps as well as the expression of different receptors, which might be involved in Mdk signaling. In human CMs, the mRNA expression of SERCA2a, NCX, TLR4, TLR9, and P2X7 increased significantly in presence of Mdk compared to control (Figures 4A,C–F), indicating for direct effects of Mdk on gene expression of calcium handling proteins. Moreover, the effects of Mdk might be mediated via TLR-P2X7 signaling. The mRNA expression of RyR1 was unaffected (Figure 4B).
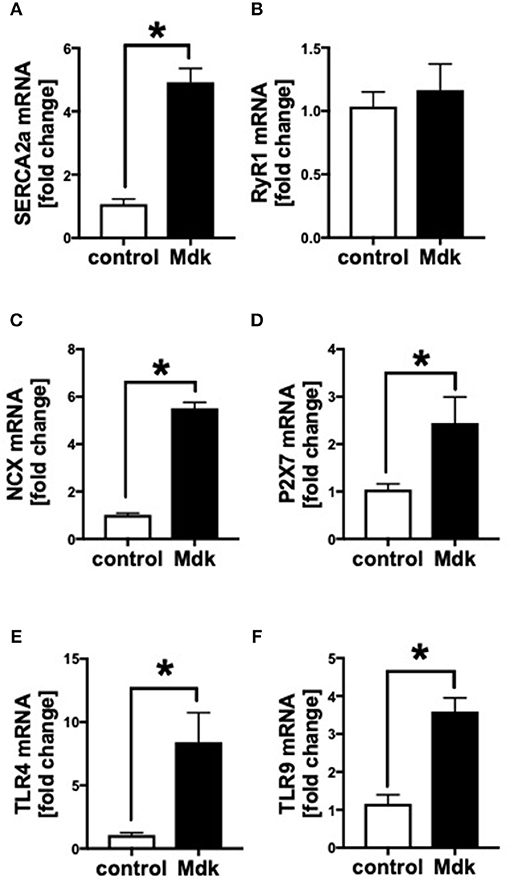
Figure 4. Gene expression of human cardiomyocytes treated for 6 h with 100 ng/ml Midkine. mRNA expression (in fold change) of sarco/endoplasmatic reticulum Ca2+-ATPase (SERCA2a) (A), ryanodine receptor-1 (RyR1) (B), sodium-calcium exchanger (NCX) (C), purigenic P2X receptor subtype 7 (P2X7) (D), toll-like receptor 4 (TLR4) (E), toll-like receptor 9 (TLR9) (F). Results are presented as mean ± SEM. Data were analyzed by two-tailed, unpaired students t-test. For all experiments n = 6. Results are significant *p < 0.05.
Mitochondrial Respiration of Human Cardiomyocytes
In addition, we analyzed the effects of Mdk on the mitochondrial respiration of CMs Figure 5A. The basal respiration as well as the spare respiratory capacity of the human CMs decreased significantly after the Mdk treatment (Figures 5B,C), indicating detrimental effects of Mdk on mitochondrial respiration.
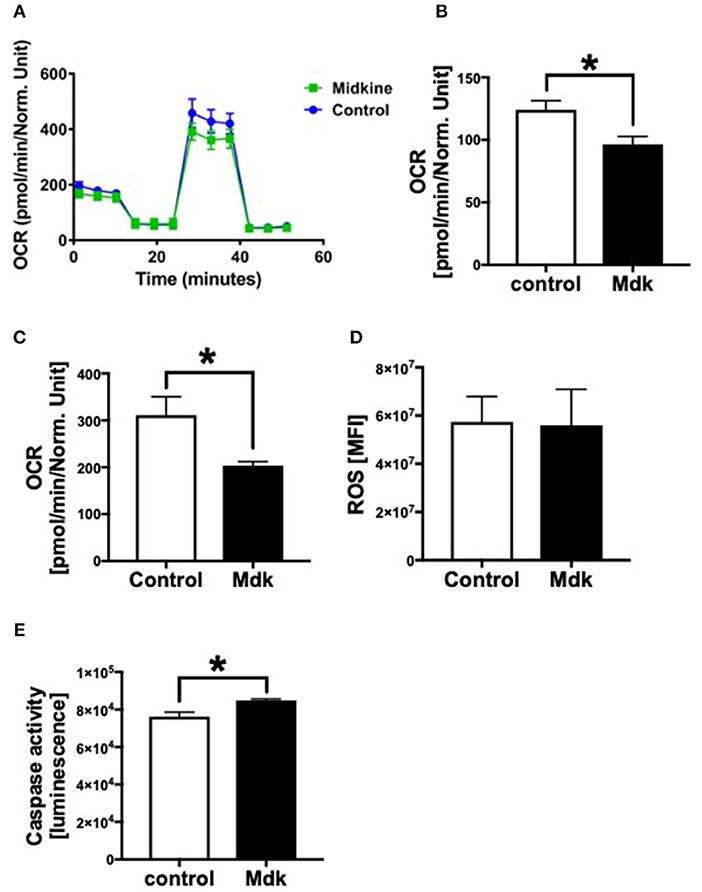
Figure 5. Mitochondrial respiration, cellular reactive oxygen species (ROS) and Caspase 3/7 activity of human cardiomyocytes treated for 6 h with 100 ng/ml Midkine. Oxygen consumption rate (OCR) of human cardiomyocytes (Control, Midkine) during Seahorse MitoStress Assay (OCR in pmol/min/E630 vs. time in min) (A). Basal respiration of human cardiomyocytes (OCR in pmol/min/E630) (B). Spare respiratory capacity of human cardiomyocytes (OCR in pmol/min/E630) (C). Amount of reactive oxygen species (mean fluorescence intensity, MFI) (D). Caspase 3/7 activity (Luminescence in counts/sec) (E). Results are presented as mean ± SEM. For all experiments n = 6. Data were analyzed by two-tailed, unpaired students t-test. Results are significant *p < 0.05.
Intracellular Reactive Oxygen Species (ROS) and Caspase3/7 Activity
As Mdk alters mitochondrial respiration and ATP production of the cells, we next investigated whether Mdk also affects the redox balance of the human CMs. The amount of ROS did not change in human CMs after being treated with Mdk compared to control cells (Figure 5D). Although Caspase3/7 activity increased significantly in human CMs in presence of Mdk (Figure 5E), indicating for enhanced apoptosis in the cells.
Filtration of Midkine by CytoSorb® 300
Because Mdk is elevated in plasma of multiply injured humans and pigs and acts on human CMs, we examined the potential of a therapeutic approach: the absorption capacity of Mdk from human blood by CytoSorb® 300. After incubation of different Mdk concentrations with CytoSorb® 300, the Mdk levels decreased between 45 and 95% within 6 h (Figure 6A). Especially high Mdk concentrations (10,000 pg/ml) were significantly reduced up to 95% after filtration with CytoSorb® 300 after 6 h compared to the 3 h incubation (Figure 6A). Moreover, Mdk levels in plasma from multiply injured patients were significantly reduced after incubation with CytoSorb® 300 (Figure 6B).
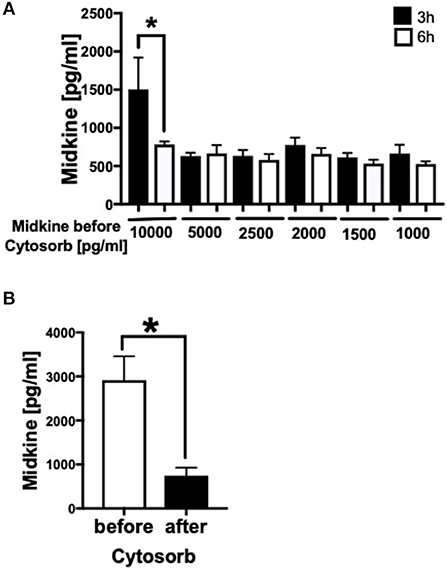
Figure 6. Filtration of Midkine by CytoSorb® 300. Filtration of different Midkine concentrations (10,000, 5,000, 2,500, 2,000, 1,500, 1,000 pg/ml) (n = 6) with CytoSorb® 300 after 3 h (black bars) and 6 h (white bars) (A). Midkine concentration before and after filtration with CytoSorb® 300 on the x-axis in pg/ml. Midkine levels (pg/ml) in human shock room blood plasma of multiply injured patients before and after filtration with CytoSorb® 300 (n = 11) (B). Results are presented as mean ± SEM. Data were analyzed by one-way ANOVA followed by Dunnett's or Tukey's multiple comparison test. Results are significant *p < 0.05.
Discussion
Our study shows for the first time that Mdk is elevated in blood circulation after multiple trauma. This elevation is similar to other traumatic injuries, suggesting that circulating Mdk may act as a novel inflammatory marker for polytrauma (8, 9, 11). Furthermore, we demonstrated that Mdk acts directly on human cardiomyocytes in vitro and is actively taken up by these cells, altering their functionality without affecting their viability. We found that Mdk affects the functionality of the human CMs by altering their calcium handling. The delta calcium peaks of the human CMs increased significantly after Mdk treatment, meaning the cells became bradycardic. Moreover, the frequency of the calcium signals in human CMs decreased significantly after Mdk treatment, confirming the bradycardic effect of Mdk. The mRNA expression of the specific cardiac calcium pumps SERCA2a and NCX also increased significantly after Mdk treatment, suggesting direct effects of Mdk on calcium handling in the cells. The location of Mdk around the cell nucleus of the human CMs confirmed the regulatory effects on cellular gene expression of the calcium handling proteins. Alterations in calcium signals as well as in mRNA expression of SERCA2a and NCX were also described previously in presence of other trauma-related inflammatory biomarkers and DAMPs as well as in different trauma models and during sepsis, nominating Mdk as a powerful cardio-depressive mediator after trauma and during sepsis (25, 37–42). However, cardiac overexpression of SERCA2a in rodents improved cardiac contractility and relaxation, which might also indicate potential protective effects of Mdk in the heart, which would require to be investigated in future studies (43, 44). We also novelly showed that the basal respiration as well as the spare respiratory capacity of the mitochondria of the human CMs decreased significantly, indicating detrimental effects of Mdk on cellular mitochondrial respiration and energy production. Nevertheless, the amount of cytosolic reactive oxygen species (ROS) was not altered in the human CMs in presence of Mdk. Mitochondrial dysfunction was also depicted previously for other trauma-related biomarkers (7, 45, 46). The detrimental and cardio-depressive effects of Mdk on the human CMs might be mediated via the toll-like receptor (TLR) 4, TLR9, and the pyrogenic receptor subtype 7 (P2X7) since the mRNA expression of these receptors was significantly upregulated. All of these receptors have been demonstrated to be involved in the DAMP-associated cardiac signaling pathways in different trauma models (47, 48). The activation of the TLRs results in increased cardiac inflammation, mediated via the nuclear factor κ B (NFκB) (47). This TLR-mediated cardiac inflammation leads to cardiac injury and finally results in cardiac contractile dysfunction (47–49). Since the mRNA expression of the TLRs was upregulated, the protein expression of these receptors might be increased after Mdk treatment. This might lead to sensitization of the CMs for other systemic circulating DAMPs, such as HMGB1 and extracellular histones, which were elevated after polytrauma, leading to cardiomyocyte dysfunction (7, 24, 50). The P2X7 receptor was also shown to be involved in cardiac contractile dysfunction (51). Interestingly, the Caspase 3/7 activity increased in human CMs after treatment with Mdk, which was demonstrated previously in cardiac tissue in vivo in an experimental polytrauma model in pigs (41). So far, Mdk was described as an anti-apoptotic factor by decreasing caspase activity in other cells, such as neurons and HepG2 cells, which is in accordance to unaffected cell viability in the present study (52, 53). The effects of Mdk on cellular apoptosis of human CMs has not been described, so far. In contrast to other cells, human CMs seem to follow different cellular processes and various signal cascades might be involved in Caspase-3/7 activation and activity when these cells were treated with Mdk. Moreover, this phenomenon could also be time-dependent as we solely investigated the Caspase-3/7 activity after 6 h of Mdk exposure. This observation should be the subject of future studies in order to understand the specific effects of Mdk on apoptosis of human CMs.
Therapeutic approaches treating post-traumatic cardiac dysfunction are still limited. In this study, we clearly showed that Mdk is elevated in plasma after multiple trauma and is predominantly detrimental on human CMs, causing the development of post-traumatic cardiac dysfunction. As a consequence, we investigated the efficiency of CytoSorb® 300 in filtering Mdk from human blood plasma. CytoSorb® 300 is an absorption column, composed of porous polymer beads, which is normally used in the intensive care unit (ICU) for septic patients or for patients with SIRS. The filtration potential of CytoSorb® 300 for various trauma-associated cytokines and DAMPs was already demonstrated by others (31, 54). Here, we showed for the first time that CytoSorb® 300 is able to absorb Mdk dose-dependently, filtering high Mdk concentrations (10,000 pg/ml) up to 95%. Moreover, CytoSorb® 300 filtered Mdk from human plasma obtained on admission to the emergency room, making it a very promising therapeutic approach for treatment and prevention of post-traumatic cardiac dysfunction. One huge benefit of using CytoSorb® 300 instead of single antibodies for therapy is that CytoSorb® 300 is able to filter a high amount of many miscellaneous damage- and inflammation molecules after trauma and not only a single molecule, which is the case of antibody treatment. Furthermore, filtration of Mdk by CytoSorb® 300 might limit other negative effects of Mdk on polytrauma patients, since it was shown that Mdk acts as an inhibitor of fracture healing and that high Mdk serum levels were associated with poor outcome in septic patients. Finally, we found that systemic Mdk is higher after conventional reaming, compared to nailing without reaming and to RIA I/II. Consequently, treatment of the fracture with RIA I/II might be better for fracture outcome as well as for fracture healing after trauma (55). In addition, conventional reaming of the fracture might have other negative effects after trauma (e.g., pulmonary embolism).
One limitation of the study might be the small sample size (n = 6) to investigate different treatment approaches for the femur fracture. Consequently, more experiments are needed to find the best and the least invasive treatment approach. The same applies for a possible correlation between fracture treatment approaches and systemic Mdk levels. Because investigated groups were heterogenous, a bigger number of samples might be helpful to extrapolate the results to a clinical population. Another limitation might be that we only used small columns with Cytosorb® 300 polymer beads in our study, trying to mimic the clinical application in ICU. However, as our study was only an experimental approach, clinical studies should be performed, including more patients and larger application approaches of Cytosorb® 300. This may help to- confirm the therapeutic potential of Cytosorb® 300 for the prevention of post-traumatic cardiac dysfunction by filtering Mdk from human blood in vivo. Furthermore, it is not possible to mimic in vitro the real in vivo inflammatory conditions, which occur after trauma. The presence of many different inflammatory mediators and DAMPs and the activation of different signal cascades in the cells lead to post-traumatic cardiac dysfunction. Consequently, it is not possible to specify these detrimental effects on one single mediator like Mdk.
Taken together, in our study we observed for the first time that Mdk is elevated systemically after multiple trauma in humans and pigs, acting cardio-depressive on human CMs by impairing their calcium handling and mitochondrial respiration capacity in vitro. PlX27/TLR might be involved in mediating these detrimental effects of MdK (Figure 7). In the clinical setting, the hemadsorption filter Cytosorb® 300 might be a powerful tool to remove cardio-depressive mediators from patients' circulation and therefore help to improve cardiac function.
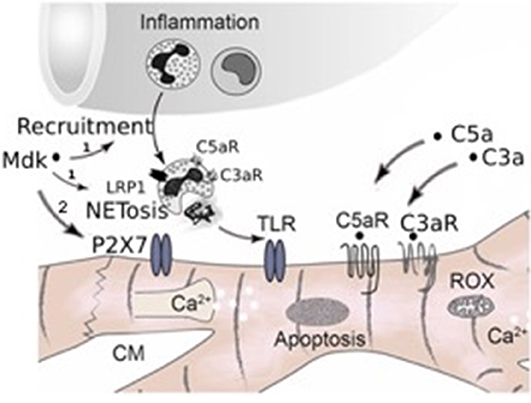
Figure 7. Schematic representation of systemic inflammation after trauma and during sepsis. After trauma Midkine is released systemically. Midkine recruits polymorphonuclear neutrophils (PMNs) and induces the release of extracellular traps (NETosis) via the receptor-related protein 1 (LRP1) on the neutrophils, which was demonstrated by Weckbach et al. (23), (1). The neutrophil extracellular traps (NETs) include extracellular histones, which act via toll-like receptors (TLRs) on the surface of cardiomyocytes, inducing cardiac dysfunction and cardiac damage. Furthermore, Midkine is able to act detrimental on human cardiomyocytes by direct interactions via TLR4, TLR9, and pyrogenic receptor subtype 7 (P2X7), inducing enhanced apoptosis, disturbing calcium signaling and impairing mitochondrial respiration (2, Hypothesis of this manuscript). Additionally, after trauma and during sepsis the complement factors C5a and C3a are released systemically. Both act directly via their receptors (C5aR, C3aR) on cardiomyocytes, leading to cardiac dysfunction. Moreover, the complement factors also induce NETosis of neutrophils via their receptors.
Data Availability
All datasets generated for this study are included in the manuscript and/or the supplementary files.
Ethics Statement
Human plasma from 11 multiply injured patients with a history of acute blunt or penetrating trauma and an ISS ≥ 16 was collected after hospital admission in the University Hospital of the Goethe-University Frankfurt with institutional ethics committee approval (312/10), in accordance with the Declaration of Helsinki and following the Strengthening the Reporting of Observational studies in Epidemiology (STROBE)-guidelines (32). All enrolled patients signed the written informed consent form themselves or written informed consent was obtained from the nominated legally authorized representative on the behalf of participants in accordance with ethical standards.
The animal housing and experimental protocols were approved by the Cantonal Veterinary Department, Zurich, Switzerland, under license no. ZH 138/2017, and were in accordance with Swiss Animal Protection Law. Housing and experimental procedures also conformed to the European Directive 2010/63/EU of the European Parliament and of the Council on the Protection of vertebrate animals used for scientific purposes (Council of Europe no. 123, Strasbourg 1985) and to the Guide for the Care and Use of Laboratory Animals (Institute of Laboratory Animal Resources, National Research Council, National Academy of Sciences, 2011).
Author Contributions
IL, BW, MB, TE, GF, SH, ML, and NC performed the experiments including animal studies, cell culture experiments, microscopic studies, and ELISAs. IL primarily wrote the paper. MH-L, FG, BR, IM, RP, H-CP, and MK contributed to experimental design and data analysis and coordinated the study and supervised financial support for the studies. All authors made substantial contributions to conception and design of the study, participated in drafting the article, and gave final approval of the version to be published.
Funding
This work was conducted in the framework of the CRC 1149 funded by the Deutsche Forschungsgemeinschaft (DFG, German Research Foundation)—Project number 251293561. This work was also funded by the AO Grant S-16-133T: Effects of standard reaming and RIA techniques on local soft tissue and systemic homeostasis in a porcine trauma model.
Conflict of Interest Statement
The authors declare that the research was conducted in the absence of any commercial or financial relationships that could be construed as a potential conflict of interest.
Acknowledgments
We kindly acknowledge the Department of Children and Adolescent Medicine, Division of Pediatric Endocrinology and Diabetes for the provision of the Agilent Seahorse XFe96 analyzer. Thanks to all members of the TREAT research group.
TREAT Research Group
Auner B, Stormann P, Simon TP, Marx G, Haug A, Egerer L, Giensven MV, Huber-Lang M, Tolba R, Reiss K, Uhlig S, Horst K, Teuben M, Almahoud K, Kalbas Y, Luken H, Almahoud K, and Hildebrand F.
References
1. WHO. Global Health Estimates 2015 Summary Tables: Deaths by Cause, Age and Sex, 2000–2015 [Online]. Available online at: http://www.who.int/healthinfo/global_burden_disease?GHE2015_Deaths_Global_2000_2015.xls?ua=1 (2016) (accessed October 1, 2017).
2. Sapan HB, Paturusi I, Jusuf I, Patellongi I, Massi MN, Pusponegoro AD, et al. Pattern of cytokine (IL-6 and IL-10) level as inflammation and anti-inflammation mediator of multiple organ dysfunction syndrome (MODS) in polytrauma. Int J Burns Trauma. (2016) 6:37–43.
3. Timmermans K, Kox M, Scheffer GJ, Pickkers P. Danger in the intensive care unit: damps in critically ill patients. Shock. (2016) 45:108–16. doi: 10.1097/SHK.0000000000000506
4. Dewar D, Moore FA, Moore EE, Balogh Z. Postinjury multiple organ failure. Injury. (2009) 40:912–8. doi: 10.1016/j.injury.2009.05.024
5. Guisasola MC, Alonso B, Bravo B, Vaquero J, Chana F. An overview of cytokines and heat shock response in polytraumatized patients. Cell Stress Chaperones. (2018) 23:483–9. doi: 10.1007/s12192-017-0859-9
6. Kumar A, Thota V, Dee L, Olson J, Uretz E, Parrillo JE. Tumor necrosis factor alpha and interleukin 1beta are responsible for in vitro myocardial cell depression induced by human septic shock serum. J Exp Med. (1996) 183:949–58. doi: 10.1084/jem.183.3.949
7. Kalbitz M, Grailer JJ, Fattahi F, Jajou L, Herron TJ, Campbell KF, et al. Role of extracellular histones in the cardiomyopathy of sepsis. FASEB J. (2015) 29:2185–93. doi: 10.1096/fj.14-268730
8. Iwashita N, Muramatsu H, Toriyama K, Torii S, Muramatsu T. Expression of midkine in normal and burn sites of rat skin. Burns. (1999) 25:119–24. doi: 10.1016/S0305-4179(98)00120-X
9. Sakakima H, Yoshida Y, Muramatsu T, Yone K, Goto M, Ijiri K, et al. Traumatic injury-induced midkine expression in the adult rat spinal cord during the early stage. J Neurotrauma. (2004) 21:471–7. doi: 10.1089/089771504323004610
10. Krzystek-Korpacka M, Mierzchala M, Neubauer K, Durek G, Gamian A. Midkine, a multifunctional cytokine, in patients with severe sepsis and septic shock: a pilot study. Shock. (2011) 35:471–7. doi: 10.1097/SHK.0b013e3182086001
11. Fischer V, Kalbitz M, Muller-Graf F, Gebhard F, Ignatius A, Liedert A, et al. Influence of menopause on inflammatory cytokines during murine and human bone fracture healing. Int J Mol Sci. (2018) 19:2070. doi: 10.3390/ijms19072070
12. Haffner-Luntzer M, Heilmann A, Rapp AE, Roessler R, Schinke T, Amling M, et al. Antagonizing midkine accelerates fracture healing in mice by enhanced bone formation in the fracture callus. Br J Pharmacol. (2016) 173:2237–49. doi: 10.1111/bph.13503
13. Haffner-Luntzer M, Kemmler J, Heidler V, Prystaz K, Schinke T, Amling M, et al. Inhibition of midkine augments osteoporotic fracture healing. PLoS ONE. (2016) 11:e0159278. doi: 10.1371/journal.pone.0159278
14. Kitahara T, Shishido T, Suzuki S, Katoh S, Sasaki T, Ishino M, et al. Serum midkine as a predictor of cardiac events in patients with chronic heart failure. J Card Fail. (2010) 16:308–13. doi: 10.1016/j.cardfail.2009.12.014
15. Przybylowski P, Malyszko J, Malyszko JS. Serum midkine is related to NYHA class and cystatin C in heart transplant recipients. Transplant Proc. (2010) 42:3704–7. doi: 10.1016/j.transproceed.2010.08.026
16. Reynolds PR, Mucenski ML, Le Cras TD, Nichols WC, Whitsett JA. Midkine is regulated by hypoxia and causes pulmonary vascular remodeling. J Biol Chem. (2004) 279:37124–32. doi: 10.1074/jbc.M405254200
17. Fukui S, Kitagawa-Sakakida S, Kawamata S, Matsumiya G, Kawaguchi N, Matsuura N, et al. Therapeutic effect of midkine on cardiac remodeling in infarcted rat hearts. Ann Thorac Surg. (2008) 85:562–70. doi: 10.1016/j.athoracsur.2007.06.002
18. Netsu S, Shishido T, Kitahara T, Honda Y, Funayama A, Narumi T, et al. Midkine exacerbates pressure overload-induced cardiac remodeling. Biochem Biophys Res Commun. (2014) 443:205–10. doi: 10.1016/j.bbrc.2013.11.083
19. Horiba M, Kadomatsu K, Yasui K, Lee JK, Takenaka H, Sumida A, et al. Midkine plays a protective role against cardiac ischemia/reperfusion injury through a reduction of apoptotic reaction. Circulation. (2006) 114:1713–20. doi: 10.1161/CIRCULATIONAHA.106.632273
20. Sumida A, Horiba M, Ishiguro H, Takenaka H, Ueda N, Ooboshi H, et al. Midkine gene transfer after myocardial infarction in rats prevents remodelling and ameliorates cardiac dysfunction. Cardiovasc Res. (2010) 86:113–21. doi: 10.1093/cvr/cvp386
21. Kadomatsu K, Bencsik P, Gorbe A, Csonka C, Sakamoto K, Kishida S, et al. Therapeutic potential of midkine in cardiovascular disease. Br J Pharmacol. (2014) 171:936–44. doi: 10.1111/bph.12537
22. Badila E, Daraban AM, Tintea E, Bartos D, Alexandru N, Georgescu A. Midkine proteins in cardio-vascular disease. Where do we come from and where are we heading to? Eur J Pharmacol. (2015) 762:464–71. doi: 10.1016/j.ejphar.2015.06.040
23. Weckbach LT, Grabmaier U, Uhl A, Gess S, Boehm F, Zehrer A, et al. Midkine drives cardiac inflammation by promoting neutrophil trafficking and NETosis in myocarditis. J Exp Med. (2019) 216:350–68. doi: 10.1084/jem.20181102
24. Abrams ST, Zhang N, Manson J, Liu T, Dart C, Baluwa F, et al. Circulating histones are mediators of trauma-associated lung injury. Am J Respir Crit Care Med. (2013) 187:160–9. doi: 10.1164/rccm.201206-1037OC
25. Kalbitz M, Amann EM, Bosch B, Palmer A, Schultze A, Pressmar J, et al. Experimental blunt chest trauma-induced myocardial inflammation and alteration of gap-junction protein connexin 43. PLoS ONE. (2017) 12:e0187270. doi: 10.1371/journal.pone.0187270
26. Kellum JA, Song M, Venkataraman R. Hemoadsorption removes tumor necrosis factor, interleukin-6, and interleukin-10, reduces nuclear factor-κB DNA binding, and improves short-term survival in lethal endotoxemia. Crit Care Med. (2004) 32:801–5. doi: 10.1097/01.CCM.0000114997.39857.69
27. Born F, Pichmaeier M, Peter S. Systemic inflammatory response syndrome in heart surgery: new possibilities for treatment through the use of a cytokine adsorber during ECC? Kardiotechnik. (2014) 14:1–10.
28. Hetz H, Berger R, Recknagel P, Steltzer H. Septic shock secondary to beta-hemolytic streptococcus-induced necrotizing fasciitis treated with a novel cytokine adsorption therapy. Int J Artif Organs. (2014) 37:422–6. doi: 10.5301/ijao.5000315
29. Hinz B, Jauch O, Noky T, Friesecke S, Abel P, Kaiser R. CytoSorb, a novel therapeutic approach for patients with septic shock: a case report. Int J Artif Organs. (2015) 38:461–4. doi: 10.5301/ijao.5000429
30. Kogelmann K, Drüner M, Jarczak D. Case study of 8 patients with multiple organ failure treated additionally with CytoSorbents haemadsorption as adjunctive therapy in septic shock and severe SIRS in cardiac failure. Germany Infect. (2015) 43:1–73.
31. Gruda MC, Ruggeberg KG, O'Sullivan P, Guliashvili T, Scheirer AR, Golobish TD, et al. Broad adsorption of sepsis-related PAMP and DAMP molecules, mycotoxins, and cytokines from whole blood using CytoSorb(R) sorbent porous polymer beads. PLoS ONE. (2018) 13:e0191676. doi: 10.1371/journal.pone.0191676
32. von Elm E, Altman DG, Egger M, Pocock SJ, Gotzsche PC, Vandenbroucke JP. The Strengthening the Reporting of Observational Studies in Epidemiology (STROBE) statement: guidelines for reporting observational studies. J Clin Epidemiol. (2008) 61:344–9. doi: 10.1016/j.jclinepi.2007.11.008
33. Horst K, Simon TP, Pfeifer R, Teuben M, Almahmoud K, Zhi Q, et al. Characterization of blunt chest trauma in a long-term porcine model of severe multiple trauma. Sci Rep. (2016) 6:39659. doi: 10.1038/srep39659
34. Eschbach D, Steinfeldt T, Hildebrand F, Frink M, Scholler K, Sassen M, et al. A porcine polytrauma model with two different degrees of hemorrhagic shock: outcome related to trauma within the first 48 h. Eur J Med Res. (2015) 20:73. doi: 10.1186/s40001-015-0162-0
35. Horst K, Eschbach D, Pfeifer R, Hubenthal S, Sassen M, Steinfeldt T, et al. Local inflammation in fracture hematoma: results from a combined trauma model in pigs. Mediators Inflamm. (2015) 2015:126060. doi: 10.1155/2015/126060
36. Artimovich E, Jackson RK, Kilander MBC, Lin YC, Nestor MW. PeakCaller: an automated graphical interface for the quantification of intracellular calcium obtained by high-content screening. BMC Neurosci. (2017) 18:72. doi: 10.1186/s12868-017-0391-y
37. Ballard-Croft C, Carlson D, Maass DL, Horton JW. Burn trauma alters calcium transporter protein expression in the heart. J Appl Physiol. (2004) 97:1470–6. doi: 10.1152/japplphysiol.01149.2003
38. Tanaka T, Kanda T, Takahashi T, Saegusa S, Moriya J, Kurabayashi M. Interleukin-6-induced reciprocal expression of SERCA and natriuretic peptides mRNA in cultured rat ventricular myocytes. J Int Med Res. (2004) 32:57–61. doi: 10.1177/147323000403200109
39. Duncan DJ, Yang Z, Hopkins PM, Steele DS, Harrison SM. TNF-α and IL-1β increase Ca2+ leak from the sarcoplasmic reticulum and susceptibility to arrhythmia in rat ventricular myocytes. Cell Calcium. (2010) 47:378–86. doi: 10.1016/j.ceca.2010.02.002
40. Zhang C, Mo M, Ding W, Liu W, Yan D, Deng J, et al. High-mobility group box 1 (HMGB1) impaired cardiac excitation-contraction coupling by enhancing the sarcoplasmic reticulum (SR) Ca(2+) leak through TLR4-ROS signaling in cardiomyocytes. J Mol Cell Cardiol. (2014) 74:260–73. doi: 10.1016/j.yjmcc.2014.06.003
41. Kalbitz M, Schwarz S, Weber B, Bosch B, Pressmar J, Hoenes FM, et al. Cardiac depression in pigs after multiple trauma - characterization of posttraumatic structural and functional alterations. Sci Rep. (2017) 7:17861. doi: 10.1038/s41598-017-18088-1
42. Fattahi F, Frydrych LM, Bian G, Kalbitz M, Herron TJ, Malan EA, et al. Role of complement C5a and histones in septic cardiomyopathy. Mol Immunol. (2018) 102:32–41. doi: 10.1016/j.molimm.2018.06.006
43. He H, Giordano FJ, Hilal-Dandan R, Choi DJ, Rockman HA, McDonough PM, et al. Overexpression of the rat sarcoplasmic reticulum Ca2+ ATPase gene in the heart of transgenic mice accelerates calcium transients and cardiac relaxation. J Clin Invest. (1997) 100:380–9. doi: 10.1172/JCI119544
44. Vetter R, Rehfeld U, Reissfelder C, Weiss W, Wagner KD, Gunther J, et al. Transgenic overexpression of the sarcoplasmic reticulum Ca2+ ATPase improves reticular Ca2+ handling in normal and diabetic rat hearts. FASEB J. (2002) 16:1657–9. doi: 10.1096/fj.01-1019fje
45. Tatsumi T, Matoba S, Kawahara A, Keira N, Shiraishi J, Akashi K, et al. Cytokine-induced nitric oxide production inhibits mitochondrial energy production and impairs contractile function in rat cardiac myocytes. J Am Coll Cardiol. (2000) 35:1338–46. doi: 10.1016/S0735-1097(00)00526-X
46. Shen YL, Shi YZ, Chen GG, Wang LL, Zheng MZ, Jin HF, et al. TNF-α induces Drp1-mediated mitochondrial fragmentation during inflammatory cardiomyocyte injury. Int J Mol Med. (2018) 41:2317–27. doi: 10.3892/ijmm.2018.3385
47. Boyd JH, Mathur S, Wang Y, Bateman RM, Walley KR. Toll-like receptor stimulation in cardiomyoctes decreases contractility and initiates an NF-κB dependent inflammatory response. Cardiovasc Res. (2006) 72:384–93. doi: 10.1016/j.cardiores.2006.09.011
48. Bruns B, Maass D, Barber R, Horton J, Carlson D. Alterations in the cardiac inflammatory response to burn trauma in micelacking a functional Toll-like receptor 4 gene. Shock. (2008) 30:740–6. doi: 10.1097/SHK.0b013e318173f329
49. Avlas O, Fallach R, Shainberg A, Porat E, Hochhauser E. Toll-like receptor 4 stimulation initiates an inflammatory response that decreases cardiomyocyte contractility. Antioxid Redox Signal. (2011) 15:1895–909. doi: 10.1089/ars.2010.3728
50. Darrabie MD, Cheeseman J, Limkakeng AT, Borawski J, Sullenger BA, Elster EA, et al. Toll-like receptor activation as a biomarker in traumatically injured patients. J Surg Res. (2018) 231:270–7. doi: 10.1016/j.jss.2018.05.059
51. Gao H, Yin J, Shi Y, Hu H, Li X, Xue M, et al. Targeted P2X7 R shRNA delivery attenuates sympathetic nerve sprouting and ameliorates cardiac dysfunction in rats with myocardial infarction. Cardiovasc Ther. (2017) 35. doi: 10.1111/1755-5922.12245
52. Owada K, Sanjo N, Kobayashi T, Mizusawa H, Muramatsu H, Muramatsu T, et al. Midkine inhibits caspase-dependent apoptosis via the activation of mitogen-activated protein kinase and phosphatidylinositol 3-kinase in cultured neurons. J Neurochem. (1999) 73:2084–92. doi: 10.1046/j.1471-4159.1999.02084.x
53. Ohuchida T, Okamoto K, Akahane K, Higure A, Todoroki H, Abe Y, et al. Midkine protects hepatocellular carcinoma cells against TRAIL-mediated apoptosis through down-regulation of caspase-3 activity. Cancer. (2004) 100:2430–6. doi: 10.1002/cncr.20266
54. Houschyar KS, Pyles MN, Rein S, Nietzschmann I, Duscher D, Maan ZN, et al. Continuous hemoadsorption with a cytokine adsorber during sepsis - a review of the literature. Int J Artif Organs. (2017) 40:205–11. doi: 10.5301/ijao.5000591
Keywords: polytrauma, cardiac dysfunction, fracture treatment, damage associated molecular pattern, toll-like receptor, toll-like receptor signaling, prevention cardiac injury, CytoSorb® 300
Citation: Lackner I, Weber B, Baur M, Haffner-Luntzer M, Eiseler T, Fois G, Gebhard F, Relja B, Marzi I, Pfeifer R, Halvachizadeh S, Lipiski M, Cesarovic N, Pape H-C, Kalbitz M and TREAT Research Group (2019) Midkine Is Elevated After Multiple Trauma and Acts Directly on Human Cardiomyocytes by Altering Their Functionality and Metabolism. Front. Immunol. 10:1920. doi: 10.3389/fimmu.2019.01920
Received: 29 April 2019; Accepted: 29 July 2019;
Published: 21 August 2019.
Edited by:
Lukas Martin, University Hospital RWTH Aachen, GermanyReviewed by:
Juerg Hamacher, Lindenhofspital, SwitzerlandSergio Iván Valdés-Ferrer, Instituto Nacional de Ciencias Médicas y Nutrición Salvador Zubirán (INCMNSZ), Mexico
Sandra Kraemer, University Hospital RWTH Aachen, Germany
Copyright © 2019 Lackner, Weber, Baur, Haffner-Luntzer, Eiseler, Fois, Gebhard, Relja, Marzi, Pfeifer, Halvachizadeh, Lipiski, Cesarovic, Pape, Kalbitz and TREAT Research Group. This is an open-access article distributed under the terms of the Creative Commons Attribution License (CC BY). The use, distribution or reproduction in other forums is permitted, provided the original author(s) and the copyright owner(s) are credited and that the original publication in this journal is cited, in accordance with accepted academic practice. No use, distribution or reproduction is permitted which does not comply with these terms.
*Correspondence: Miriam Kalbitz, miriam.kalbitz@uniklinik-ulm.de