- 1EA 7501 GICC Université de Tours, Tours, France
- 2CHRU de Tours, Tours, France
Low/intermediate affinity Fc-gamma receptors (FcγR) are crucial for the recognition of immune complexes and IgG-sensitized microorganisms by phagocytic and cytotoxic effector cells. In all mammalian species studied so far, their genes are clustered in a single locus. However, this locus differs between humans and mice, both in the number of genes and the structure/function of the encoded receptors. We show that murine fcgr3 evolved through several steps into FCGR2A, its ortholog, which is specific to primates. One of these steps was the insertion of a retroviral element bringing a new intracellular exon comprising a non-canonical ITAM motif. We also show that the fcgr3-hspa6-fcgr4-fcgr2b module in mammals that has evolved in a FCGR2A-HSPA6-FCGR4-FCGR2B module in primates, was subsequently duplicated in apes through a Non-Allelic Homologous Recombination (NAHR), giving birth to FCGR2C, a hybrid gene between FCGR2B and FCGR2A. The FCGR4 duplication, which occurred simultaneously, eventually resulted in the emergence of FCGR3B, while FCGR3A remained the true FCGR4 ortholog. FCGR2C and FCGR3B, markers of this NAHR, are present in gorillas and chimpanzees, whereas they are absent in orangutans and more distant primates, such as gibbons and macaques. These data need to be taken into account when testing IgG-based therapies in animal species.
Introduction
Receptors for the Fc portion of IgG (FcγR) play an important role by connecting innate and adaptive immunity. This is particularly true for receptors of low/intermediate affinity which are specialized in the recognition of immune complexes and IgG-sensitized microorganisms or target cells by phagocytic and cytotoxic effector cells. In all mammalian species studied so far, all the genes encoding these low/intermediate affinity FcγRs are clustered in a single locus. This clustering probably allows gene plasticity and rapid evolution to maintain adequate binding of the different FcγR to their different ligands (IgG subclasses), which are themselves in continuous evolution, and to maintain the immune functions conferred to the effector cells.
In mice, there are three different genes, gathered on distal chromosome 1: Fcgr2 and Fcgr3, coding for FcγRII and FcγRIII, respectively, and the more recently identified Fcgr4 coding for FcγRIV (1–3). FcγRIII and FcγRIV, as well as the high affinity FcγRI (gene located on chromosome 3), are associated with the FcR-γ chain, which carries an Immunoreceptor Tyrosine-based Activation Motif (ITAM) required for cell activation (2, 3). FcR-γ KO mice do not express these activating receptors and are deficient in antibody-mediated responses such as antibody-dependent cell-mediated cytotoxicity (ADCC) and antibody-dependent cell phagocytosis (4, 5). By contrast, FcγRII includes an Immunoreceptor Tyrosine-based Inhibitory Motif (ITIM) in its intracellular portion and can inhibit activating receptors when both types of receptors are coengaged (6). Accordingly, mice lacking Fcgr2 show augmented humoral responses and increased responses to therapeutic antibodies (4, 5).
In humans, there are five genes encoding low/intermediate affinity IgG Fc receptors, clustered on chromosome 1q21-q23: FCGR2A, FCGR2B, FCGR2C, FCGR3A, and FCGR3B. The situation therefore appears much more complex than in mice, with an increased number of genes, and also by the fact at least three of them (FCGR2A, FCGR2C, and FCGR3B) are coding for FcγRs displaying properties not existing in mice. FCGR2A encodes the activating receptor FcγRIIA, which is described as an equivalent to murine FcγRIII (7), although it does not associate with FcR-γ. Indeed, FcγRIIA possesses its own activation motif in its intracellular (IC) domain, a non-classical ITAM characterized by the presence of 12 residues between the two YXXL boxes, instead of 7 in classical ITAMs. This non-classical ITAM has been associated with differences in intracellular signaling pathways and functional activities (8). FCGR2C is a chimeric gene that is the result of an unequal crossing-over between FCGR2A and FCGR2B, having extracellular domains similar to FcγRIIB and an intracellular ITAM-like motif identical to that of FcγRIIA (9). FCGR3B codes for FcγRIIIB, an atypical receptor attached to the cell membrane by a glycosyl-phosphatidylinositol (GPI) anchor and having no intrinsic triggering capability even though it could contribute to cell activation by associating with other FcγRs (10). FCGR2B encodes the only ITIM-containing inhibitory receptor, in both human and mice, and is therefore likely the ortholog of murine Fcgr2. FcγRIIIA is the only human low/intermediate affinity FcγRs requiring FcR-γ as transduction subunit, like murine FcγRIII and FcγRIV, but sequence analyses suggest that FCGR3A could be the human ortholog of murine Fcgr4 (2).
Little is known about the evolution of the FCGR2/4 gene cluster and the mechanisms which led to the acquisition of FCGR2C and FCGR3B in human. Moreover, despite the functional consequences of the ITAM-like motif, no hypothesis has been proposed to explain its presence in FcγRIIA and FcγRIIC so far.
Amongst non-human primates, FcγRIIA has been identified in macaque, baboon and mangabey (11). Rhesus, cynomolgus and pigtail macaques are also known to express FcγRIIA and IIB (12). As for other mammals, FcγRII is known to be expressed cows, and FcγRIII by cows and pigs, without any further detail as to which isoform (A or B) these receptors correspond to (13).
In order to gain insight into the evolutional history of the FCGR2/4 cluster, we studied the genes coding for low-affinity FcγRs in several mammalian species, including several primates. Nucleotide alignments were performed on the genomes to find low/medium affinity FCGR orthologs, which were amplified using long PCR and further characterized through sequencing and alignment with orthologs. A sequence suggesting a genetic recombination event was found and compared with known viral retroelements. We found that the cluster is composed of 5 genes in humans, chimpanzees and gorillas but by only three in macaques, gibbons, orangutans and other mammals. We also confirm that macaques are characterized by a unique FcγRIIA receptor with an ITAM-like motif, and extend this feature to other primates (14).
Materials and Methods
DNA
Genomic DNA from chimpanzee (ECACC No. 89072704), gorilla (ECACC No. 89072703), orangutan (ECACC No. 89072705), gibbon (ECACC No. 86102901) and cynomologous monkey (ECACC No. 90071809) cell lines was purchased from European Collection of Cell Cultures. DNA was extracted from buccal samples of six Gorillas and 8 Orangutans, using Isohelix DNA isolation kit. One additional gorilla DNA was obtained by blood extraction using QIAGEN DNA extraction kit (Qiagen, ref. 51104).
Identification of the Medium/Low Affinity Loci in Other Mammals
The mammalian genomes were systematically screened for Fcgr2/3 orthologs using BLAST for nucleotidic alignment, with default parameters (15). The contiguous sequences, the versions used and the updated versions where appropriate, are described in (Supplementary Table 1).
Amplification and Sequence Analysis of FCGR2A/FCGR2B/FCGR2C Genes
Long-PCR was performed with Elongase Amplification System (Invitrogen, ref. 10480010) according to manufacturer procedure, with 1.5 mM of MgCl2. Primers were designed on highly conserved coding sequences observed between primates in order to amplify genes of interest. Special care was taken in order to design primers with high gene specificity and with sequence compatibility including mammals other than primates when possible. For FCGR2A amplification, an 8 kb fragment was obtained using a 2aMfe2-S forward primer located in exon 2 (5′GCTTCTGCAGACAGTCAAACTG3′) and a 2aMfe7-AS reverse primer located in exon 7 (5′TCGGGCAGCCTTCACAGGATC3′). For FCGR2B amplification a 7.5 kb fragment was obtained using a 2bMfHse2-S forward primer located in exon 2 (5′CTCCTGTTGCTGGGACACCTG3′) and a 2bMfHse8-AS reverse primer located in exon 8 (5′TGAATAGGTGATTGTGTTCTCAGC3′). For FCGR2C, which is a chimeric gene between FCGR2A and FCGR2B, a 7.2 kb fragment was obtained using 2bMfHse2-S forward primer and 2aMfe7-AS reverse primer. For gibbon, due to divergence, 2aGibbon-S primer (5′CTTCTGCAGACAGTCAAGCTG3′) was used instead of 2aMfe2-S. For all species, 100 ng of genomic DNA sample were amplified in 20 μL final volume on a Bio-Rad MJ Mini Thermal Cycler with an initial denaturation at 94°C for 5 min, followed by 35 cycles of: 94°C for 30 s, 63.1°C for 30 s, 68°C for 10 min. Amplification was verified by loading PCR products on a 0.8% agarose gel, which was revealed using ethidium bromide. All the PCR products where secondly sequenced using the primers described in Supplementary Table 2.
Amplification and Sequence Analysis of FCGR3A and FCGR3B Genes
PCR was performed with Taq Polymerase (Eurobio, ref. GAETAQ004D) following the manufacturer's instructions. Primers were designed as described above. We referred to a genomic alignment to ensure that primers would amplify the intended fragments in all considered species. For co-amplification of FCGR3A and FCGR3B, a 176 bp product was obtained with a 3Mfe5-S forward primer (5′TTTGGCAGTGTCATCCATCTC3′) and a 3Mfe5(2)-AS reverse primer (5′ATTTGTCTTGAGGGTCCTTGCTCC3′), both located in exon 5. PCR was conducted as described above, except that initial denaturation was followed by 35 cycles of: 94°C for 30 s, 55.7°C for 30 s, 68°C for 10 min. Amplification was verified by loading PCR products on an 8% acrylamide gel, which was revealed using ethidium bromide. All the PCR products where secondly sequenced.
Bioinformatic Analysis
Sequences were collected in genomic and cDNA databases by similarity searching using the BLAST tools (15), all available on the NCBI website (http://www.ncbi.nlm.nih.gov/). Sequences for endogenous retrovirus were extracted from REPBASE using CENSOR tool (16) available on GIRI web site (http://www.girinst.org/) and from RepeatMasker (http://www.repeatmasker.org/). Multiple alignments for the genomic analysis were done using CLUSTALW (17) followed by visual inspection and manual editing when necessary. The Neighbor-Joining trees were obtained using Kalign algorithm (18).
Results
Genesis of FCGR2A, a New Activating Receptor Gene in Primates
Analysis of human and mouse FCGR sequences was performed and confirmed orthology between human FCGR3A and mouse Fcgr4 and between human FCGR2B and mouse Fcgr2b. Alignment of mouse Fcgr3-HspA6 segment with human FCGR2A-HSPA6 showed extensive homology, notably in S1 and S2 exons, from the EC1 to the EC2 exons, including the third intron, and the whole HSPA6 gene, including upstream and downstream regions (Figure 1A).
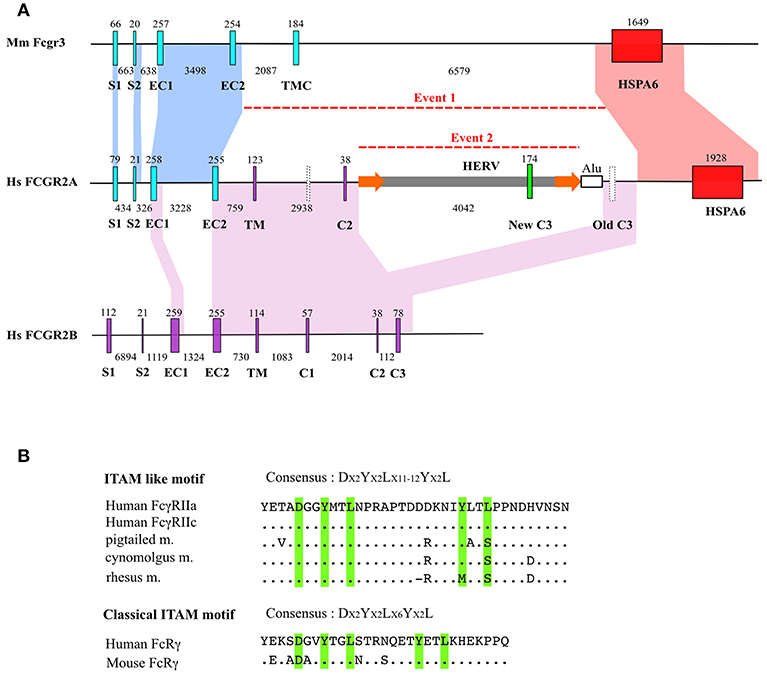
Figure 1. FCGR2A genesis. (A) Intron/exon organization of mouse Fcgr3 and human FCGR2B and FCGR2A genes. Homologous regions (defined by default BLAST E-value) were indicated by areas of identical colors. Major insertion events are indicated by dotted lines. Exons are abbreviated as follows: signal peptide 1 (S1) and 2 (S2), extracellular 1 (EC1) and 2 (EC2), transmembrane (TM) and intracytoplasmic 1 (C1), 2 (C2), and 3 (C3) domains. HSPA6 stands for Heat Shock Protein Family A (Hsp70) Member 6. HERV stands for Human Endogeneous RetroVirus. Exon and intron size are indicated above and below each gene. For clarity purposes, there is no correspondence between nucleotidic size of the represented genetic elements in kb and scale in the figure. Empty vertical bars represent non-coding exons. Flanking LTRs around HERV are shown as orange arrows. (B) Comparison of FCGR2A ITAM-like motif coding by retroelement with canonical ITAM motif. Dots indicate identity and dashes indicate gaps.
These observations confirm an orthologous relationship between Fcgr3 and FCGR2A.
Interestingly, although mouse Fcgr3 and Fcgr2b show no homology except between their EC1 and EC2 exons (data not shown), human FCGR2A aligns with FCGR2B not only at the level of EC1 and EC2 exons, but also on a large stretch extending for the 5′ part of EC2 to the 3′ part of IC3 of FCGR2B. This alignment is interrupted by a ~5 kb segment characterized by long terminal repeats (LTR) suggesting a retroviral origin (Figure 1A). Annotation from Repeatmasker showed that these LTR present high homology with LTR from MER57F, a class I human endogenous retrovirus. No homology with the classical retrovirus genes gag, pol and env was found in the sequence. Analysis of the internal part of the sequence revealed a high homology with MER34B, a non-autonomous retroelement (Supplementary Table 3). The presence of this element was tested among other mammals by LTR signature to evaluate the age of this Fcgr3 insertion. Traces of this retroelement were found in Otolemur garnetii (Lemuriformes), Callithrix jacchus (Callitrichidae), and in Macaca mulatta (Cercopithecidae) which revealed that its acquisition is probably specific to primates. A new FCGR2A IC3 exon appeared as being encoded by the endogenous retrovirus segment homologous to MER34B. The non-canonical ITAM motif contained in FcγRIIA and FcγRIIC, unique to primates and encoded by the new IC3 exon, possesses a DX2YX2LX12YX2L sequence in human and chimpanzee FcγRIIA and FcγRIIC, replaced by a DX2YX2LX11YX2L sequence in cynomolgus monkey (Figure 1B). A nucleotide sequence potentially encoding DX2YX2LX11YX2L in another composite endogenous retrovirus is located in chromosome 11p14.3, between the NELL1 and TMEM16E genes. This sequence is not associated to any known transcript. The vestigial IC3 exon downstream the endogenous retrovirus, which was homologous to FCGR2B IC3, has never been found in FCGR2A (or FCGR2C) mRNA, even in the EST database.
Genomic Organization of FCGR2/3 Cluster in Mammals
Segmental Duplication of Cluster FCGR2/3 in Primates
Sequences drawn from the Chimpanzee Genome Project were reassembled using the human FCGR2/3 cluster as reference. It appears that the chimpanzee's cluster comprises orthologs of all the human FCGR genes as well as of the HSPA6 and HSPA7 genes. However, it should be noted that, through this approach, the FCGR2C gene could not be unambiguously assigned. Similarly, we analyzed the FCGR2/3 cluster in macaque (Macaca mulatta) using sequences from Rhesus Macaque Genome Resources. We found traces of sequences that suggest that macaque cluster is composed by only three FCGR genes and one HSPA6 gene (FCGR2A-HSPA6-FCGR4-FCGR2B) corresponding to orthologous genes of human FCGR2A, HSPA6, FCGR3A, and FCGR2B. However, based on the available sequences and reassembled contigs, it cannot be totally excluded that FCGR3B and FCGR2C genes do exist in this species. Sequences available for rat, cow and partial sequence from dog revealed a genomic organization close to that of mouse cluster which demonstrates that fcgr3-hspA6fcgr4-fcgr2b is the standard cluster for non-primate mammals (Figure 2A). Close inspection of the whole human cluster allowed us to define two duplication modules of approximately ~80 kb with the same architecture, on either side of the breaking point region already associated with FCGR2C genesis (9). The upstream module (b1), from FCGR2A to FCGR2C, encompasses HSPA6 and FCGR3A, whereas the downstream module (b2), from FCGR2C to FCGR2B, encompasses HSPA7, and FCGR3B (Figure 2A). The b1 and b2 modules are colinear with a remarkable identity score (94%), raising the hypothesis of a tandem duplication of the b module in a former FCGR2A-FCGR3-HSPA6-FCGR2B cluster, which will be referred to as FCGR2A-FCGR4-HSPA6-FCGR2B with respect to the nomenclature already in use in other species. This event was simultaneously responsible for the duplication of FCGR4 (which subsequently evolved in FCGR3A and FCGR3B), HSPA6 (duplicated in HSPA6 and HSPA7), and for the emergence of FCGR2C, made of the 5′ part of FCGR2B and of the 3′part of FCGR2A (Figure 2B).
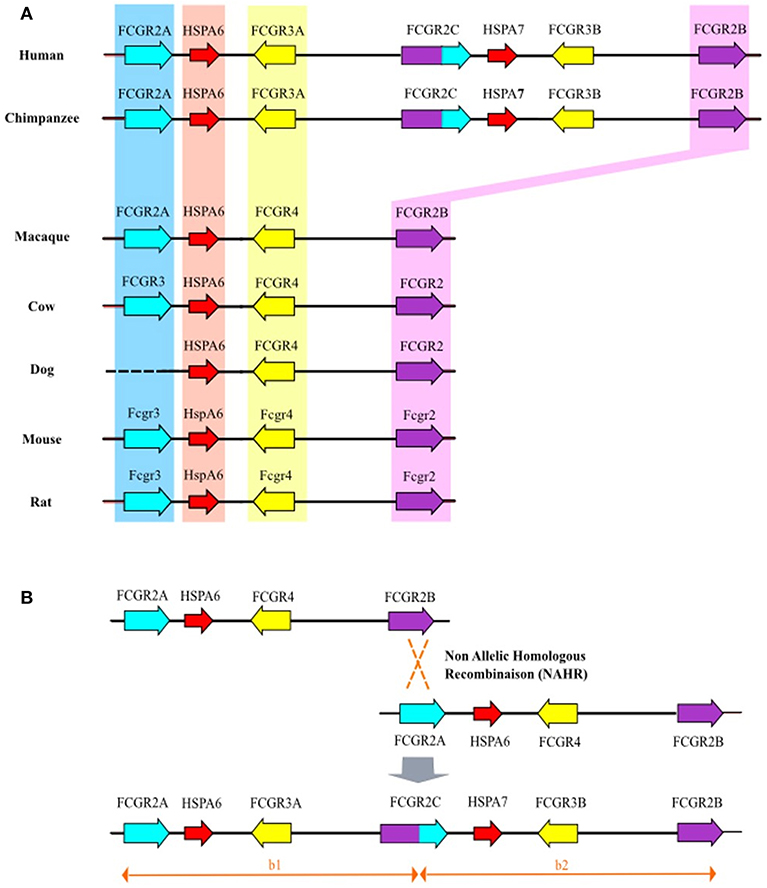
Figure 2. (A) Genomic organization of the FCGR2/3 cluster in mammals. Bioinformatic analysis of orthologous genes of human FCGR2A, FCGR2B, and FCGR3A (blue, yellow, and purple backgrounds, respectively). For gorilla and chimpanzee, the FCGR2/3 cluster is characterized by additional human orthologous FCGR2C and FCGR3B genes. Similarly, orthologous genes of human HSPA6 (red background) were found in all studied species whereas only gorilla and chimpanzee possess human orthologous HSPA7. (B) Non allelic homologous recombination between FCGR2A and FCGR2B leading to duplication of FCGR4 (giving birth to FCGR3B and FCGR3A) and emergence of FCGR2C.
PCR Screening for Orthologous Genes of Human FCGR2 and FCGR3
We were then interested to know whether the intermediary species between Cercopithecids (macaques and baboons) and Hominids (chimpanzees and humans), i.e., Hylobates and Pongids, possess both the FCGR2C and FCGR3B genes, or not. In this purpose, two primer pairs were first designed to specifically amplify FCGR2A and FCGR2B whatever the primate species, in a representative set of Old World primates. Using the FCGR2B-specific forward and the FCGR2A-specific reverse primers, FCGR2C is specifically amplified if present (Supplementary Figure 1). An amplification product was obtained with human, chimpanzee and gorilla DNA, but not with Cercopithecinae (Macaque), Hylobates (Gibbon) and Pongids (orang-utan) DNAs, suggesting an absence of FCGR2C (Figure 3) in these species. For these three FCGR2 genes, and for all the tested primate species, gene specificity of the amplification products was confirmed by sequence conservation and organism specificity was controlled by sequence divergence observed at PCR product end.
Similarly, a PCR was designed to co-amplify FCGR3B together with FCGR3A. FCGR3B is characterized by a serine at codon position 203 (Ser203 generating the glycosyl-phosphatidylinositol anchor of FcγRIIIB) and a stop codon at position 216. The co-amplification product of FCGR3A and FCGR3B of human genomic DNA reveals a T (Phe) or a C (Ser) at the second position of codon 203 or a C (Arg) and T (stop) at the first position of codon 216. Both types of codons were observed at each position in gorilla and chimpanzee DNA, whereas only a Phe203 and an Arg216 or an alternative Pro216 codon were found in macaque, gibbon and orang-utan (Supplementary Figure 2). These results were confirmed with a different priming strategy. Two FCGR3B specific primers sets obtained by substitution of one of the aforementioned pair of primers by another one anchored on FCGR3B specific sequences failed to give amplification products in the macaque, gibbon and orang-utan DNA, but again, were effective in amplifying FCGR3B in humans, chimpanzees and gorillas (data not shown).
Comparative Sequence and Phylogenetic Analysis
To investigate the evolutionary relationships among FCGR2 genes, we constructed neighbor-joining trees using sequences obtained for FCGR2A, FCGR2B, and FCGR2C genes at various levels of the sequence. Using exon 3, the obtained patterns show two major groups of genes where murine Fcgr2b and Fcgr3 are orthologous to primate FCGR2B and FCGR2A, respectively (Supplementary Figure 3). This approach also revealed the paralogous relationship existing between FCGR2B and the 5′part of FCGR2C. Predicted amino acid sequences obtained with exon 4 (coding for EC2) shows a high degree of conservation in primates, notably for amino acids implicated in the interaction with the Fc portion of IgG (Supplementary Figure 4). Phylogenetic analysis of exons 4, 5, and 6 showed a deviation from the predicted evolutionary relationships among the FCGR2 genes in the different species and indicates gene conversion events (data not shown). Phylogenetic tree deduced from FCGR3A and FCGR3B sequences revealed that FCGR3A and FCGR3B are paralogous genes and that Fcgr4 is orthologous to primate FCGR3A (Supplementary Figure 3B). We also observed a high degree of conservation between primate FcγRIIIA for amino acids implicated in the interaction with the Fc portion of IgGs (Supplementary Figure 5).
Discussion
This study provides insights into the genomic structure and evolution of the FCGR cluster in mammals. We searched the genomes of non-human primates for low/medium affinity FCGR orthologs, amplified the corresponding genes using a long-PCR approach, characterized the products and deduced the evolutionary history of this locus between mice and humans. We conclude that a NAHR mechanism gave rise to a new block encompassing FCGR2C and FCGR3B in humans, chimpanzees and gorillas. A potential limitation of this study resides in the risk of decreased sensitivity when using a single pair of primers per gene. These long primers were designed using the screened contiguous genomes described in the methods section, to be highly gene-specific. However, they were designed in highlyconserved regions, as described in polymorphism studies performed in macaques (19). Moreover, these 2 genes were either both present or absent in the amplification products, supporting the hypothesis of a NAHR. The duplication gave rise to FCGR2C and two FCGR4-type genes that diverged from each other, FCGR3A keeping the original FCGR4 functionality and FCGR3B acquiring specific features. This duplication event took place in homininaes, and was rendered possible by the former evolution of mammal fcgr3 into primate FCGR2A. This latter evolution is presently shown to be the result of serial processes leading to the genesis of FCGR2A coding for the new activating receptor FcγRIIA.
The evolution from fcgr3 in non-primate mammals, to FCGR2A in primates appears to be the result of two genetic events comprising a NAHR between fcgr3 and fcgr2b, and the integration of a retroviral element creating a new exon coding for an ITAM-like motif, which is a unique characteristics of primate FCGR2A. As a consequence, primates possess a new FCGR2A gene encoding FcγRIIA, and their genomes display a new FCGR2A/FCGR4/FCGR2B locus, as compared to non-primate mammals. Several hypotheses could be raised to explain the simultaneous fcgr2b/fcgr3 NAHR and endogenous retrovirus integration. In mammals, and notably in mice, the homology between fcgr3 and fcgr2b is restricted to exons coding for extracellular domains. A first recombination event probably occurred between fcgr3 and fcgr2b genes, bringing the EC2-IC3 fcgr2b gene segment into fcgr3 and probably conferring an inhibitory function to FcγRIII since the FCGR2B IC3 exon encodes the ITIM motif. The subsequent retrovirus integration led to the disruption of the IC2-IC3 splicing site, suppressing IC3 transcription and hence the inhibitory function of the receptor. These observations also suggest that the retrovirus that disrupted the “inhibitory fcgr3 gene” (fcgr2b/3) brought the DX2YX2LX11YX2L ITAM motif coding sequence.
Therefore, the loss of ITIM and the acquisition of ITAM were probably contemporaneous. Such an fcgr2b/3 gene has never been found in living species but too few of them have been studied so far to be conclusive. We could however raise the hypothesis that the presence of two genes (fcgr2b and fcgr2b/3) encoding inhibitory FcγRs has rapidly been counter-selected unless one of them was rescued, which is now the case for fcgr2b/3, in the form of FCGR2A, in macaques and apes. Indeed, like a knock-in process, an endogenous retrovirus disrupted the ITIM encoding exon and brought an ITAM-like motif allowing the final transformation of fcgr2b/3 into FCGR2A, the first activating FcγR not depending on the accessory FcR-γ chain. Hundreds examples of molecular domestication have been described where coding sequences of functional genes derive entirely or partially from mobile element sequences but, to our knowledge, this is the first case where a new transduction motif originates from a transposon (20). This motif has been classified among the ITAMs despite the fact that it contains 11 (rhesus macaque) or 12 (pigtailed macaque, cynomolgus macaque apes and humans) amino acids instead of 7 between the two YXXL boxes. This atypical structure is responsible for the presence of three phosphorylation sites and is associated with specific functions (21–23). This once again illustrates the power of mobile elements in driving the evolution and diversification of immunity-related genes (24).
It has long been known that the cluster that encodes low-affinity FcγRs displays different genomic structures depending on the species (25). However, the initial scenario proposed to explain its evolution amongst mammals did not integrate subsequent findings such as the mechanism of FCGR2C genesis, the global structure of the human cluster and the structure of the murine cluster revealing the presence of Fcgr4 (2, 9, 25, 26). It is now clear that most mammals possess two activating and one inhibiting fcgr genes tighly associated with hspa6 in an ancestral cluster (fcgr3-hspa6-fcgr4-fcgr2b) (Figure 1). The fact that the murine cluster consists of only 3 fcgr genes appears as an exception rather than a rule, suggesting that hspa6 has been lost in the laboratory strains of mice. Monkeys also possess a four-gene cluster, differing from the ancestral cluster by the presence of FCGR2A rather than fcgr3. It has been shown that the human FCGR2/3 cluster is composed of two repetition blocks (∽80 kb each with > 95 % homology) which results from a segmental duplication, according with its current definition (27). FCGR2C, a well-known chimeric gene, links the two modules and appears as a signature of the segmental duplication event (9). This recombination event is the result of an unequal cross-over favored by the high degree of homology between FCGR2A and FCGR2B. Since FCGR2C can be found in gorillas and chimpanzees, contrarily to what had been previously published by Nimmerjahn et al. but not in orangutans, gibbons and macaques, the duplication event probably occurred in a homininae ancestor <9.2 million years ago (7, 28). Although the duplication gave rise to two FCGR4 copies, FCGR3B was identified in each species where FCGR2C was found. This suggests that the second copy of FCGR4 rapidly evolved into FCGR3B, i.e., into a gene coding for the only known GPIanchored FcγR.
It is known that the presence of large duplicated segments subsequently favor additional NAHR events (29–31) resulting in gene number variations. A variable number of FCGR2C and FCGR3B was described and it seems that a co-variation number of these genes occurred in most cases (32–34). This co-variation of FCGR2C/FCGR3B copy number described in humans could be explained by the modular structure of the cluster which favors further recombination events through unequal crossing over between clusters, involving FCGR2C and upstream sequence on one chromatide, and FCGR2B-related sequences on the other (Supplementary Figure 6). Following this recombination model, human haplotypes characterized by FCGR3B duplication would also contain two FCGR2C and two HSPA7. By contrast, haplotypes characterized by an absence of FCGR3B would also be deficient in FCGR2C and HSPA7. As a matter of fact, a 10-fold higher identity region can be observed between modules b1 and b2 in the region expected to be place of secondary segmental duplication.
Altogether, it is clear that the low-affinity Fc receptor gene cluster has been submitted to serial genetic events which have been positively selected during primate evolution. In comparison with other mammals, homininaes -comprising humans- have acquired FcγRIIA, FcγRIIC, and FcγRIIIB. At least two of these receptors are fully functional (FcγRIIA and FcγRIIIB) and are involved in the mechanism of action of therapeutic antibodies. Finally, our results highlight genomic differences between humans and other mammals in terms of FcγR coding genes. Additional differences in terms of FcγR expression and function raise the issue of the relevance of animal models for pre-clinical studies of monoclonal antibodies.
Author Contributions
GB reanalyzed the data and provided critical revision and updating of the manuscript. JL performed the experiments, analyzed the results, and wrote the first draft of the manuscript. HW supervised the experiments and provided expert corrections to the manuscript.
Funding
This work was supported by a public grant overseen by the French National Research Agency as part of the Investissements d'Avenir program (reference: ANR-10LABX-53-01). JL was supported by a grant from Région Centre.
Conflict of Interest Statement
The authors declare that the research was conducted in the absence of any commercial or financial relationships that could be construed as a potential conflict of interest.
Acknowledgments
We thank Dr. Baptiste Mulot from ZooParc de Beauval (Saint Aignan sur Cher) and Pascal Guery from La vallée des singes (Romagne) for buccal/blood Gorilla and Orangutan samples. We also thank Marc Ohresser for his pioneering bioinformatic studies, and Marie-Noëlle Marson for performing the PCR analyses.
Supplementary Material
The Supplementary Material for this article can be found online at: https://www.frontiersin.org/articles/10.3389/fimmu.2019.01297/full#supplementary-material
Abbreviations
ADCC, antibody-dependent cell mediated cytotoxicity; EC, extracellular domain; ECACC, European collection of authenticated cell cultures; FcγR, Fc-gamma receptor; IC, intracellular domain; ITAM, immunoreceptor tyrosine-based activation motif; ITIM, immunoreceptor tyrosine-based inhibition motif; LTR, Long terminal repeat sequence; NAHR, non-allelic homologous recombination; NHP, non-human primate; PCR, polymerase chain reaction.
References
1. Mechetina LV, Najakshin AM, Alabyev BY, Chikaev NA, Taranin AV. Identification of CD16-2, a novel mouse receptor homologous to CD16/Fc gamma RIII. Immunogenetics. (2002) 54:463–8. doi: 10.1007/s00251-002-0486-0
2. Nimmerjahn F, Bruhns P, Horiuchi K, Ravetch JV. FcgammaRIV: a novel FcR with distinct IgG subclass specificity. Immunity. (2005) 23:41–51. doi: 10.1016/j.immuni.2005.05.010
3. Ravetch JV, Kinet JP. Fc receptors. Annu Rev Immunol. (1991) 9:457–92. doi: 10.1146/annurev.iy.09.040191.002325
4. Clynes RA, Towers TL, Presta LG, Ravetch JV. Inhibitory Fc receptors modulate in vivo cytotoxicity against tumor targets. Nat Med. (2000) 6:443–6. doi: 10.1038/74704
5. Takai T, Li M, Sylvestre D, Clynes R, Ravetch JV. FcR gamma chain deletion results in pleiotrophic effector cell defects. Cell. (1994) 76:519–29. doi: 10.1016/0092-8674(94)90115-5
6. Coggeshall KM. Inhibitory signaling by B cell Fc gamma RIIb. Curr Opin Immunol. (1998) 10:306–12. doi: 10.1016/S0952-7915(98)80169-6
7. Nimmerjahn F, Ravetch JV. Fcgamma receptors: old friends and new family members. Immunity. (2006) 24:19–28. doi: 10.1016/j.immuni.2005.11.010
8. Dai X, Jayapal M, Tay HK, Reghunathan R, Lin G, Too CT, et al. Differential signal transduction, membrane trafficking, and immune effector functions mediated by FcgammaRI versus FcgammaRIIa. Blood. (2009) 114:318–27. doi: 10.1182/blood-2008-10-184457
9. Warmerdam PA, Nabben NM, van de Graaf SA, van de Winkel JG, Capel PJ. The human low affinity immunoglobulin G Fc receptor IIC gene is a result of an unequal crossover event. J Biol Chem. (1993) 268:7346–9.
10. Ravetch JV, Perussia B. Alternative membrane forms of Fc gamma RIII(CD16) on human natural killer cells and neutrophils. Cell type-specific expression of two genes that differ in single nucleotide substitutions. J Exp Med. (1989) 170:481–97. doi: 10.1084/jem.170.2.481
11. Rogers KA, Scinicariello F, Attanasio R. IgG Fc receptor III homologues in nonhuman primate species: genetic characterization and ligand interactions. J Immunol. (2006) 177:3848–56. doi: 10.4049/jimmunol.177.6.3848
12. Hogarth PM, Anania JC, Wines BD. The FcγR of humans and non-human primates and their interaction with IgG: implications for induction of inflammation, resistance to infection and the use of therapeutic monoclonal antibodies. Curr Top Microbiol Immunol. (2014) 382:321–52. doi: 10.1007/978-3-319-07911-0_15
13. Kacskovics I. Fc receptors in livestock species. Vet Immunol Immunopathol. (2004) 102:351–62. doi: 10.1016/j.vetimm.2004.06.008
14. Trist HM, Tan PS, Wines BD, Ramsland PA, Orlowski E, Stubbs J, et al. Polymorphisms and interspecies differences of the activating and inhibitory FcγRII of Macaca nemestrina influence the binding of human IgG subclasses. J Immunol. (2014) 192:792–803. doi: 10.4049/jimmunol.1301554
15. Altschul SF, Gish W, Miller W, Myers EW, Lipman DJ. Basic local alignment search tool. J Mol Biol. (1990) 215:403–10. doi: 10.1016/S0022-2836(05)80360-2
16. Kohany O, Gentles AJ, Hankus L, Jurka J. Annotation, submission and screening of repetitive elements in Repbase: RepbaseSubmitter and censor. BMC Bioinformatics. (2006) 7:474. doi: 10.1186/1471-2105-7-474
17. Thompson JD, Higgins DG, Gibson TJ. CLUSTAL W: improving the sensitivity of progressive multiple sequence alignment through sequence weighting, positionspecific gap penalties and weight matrix choice. Nucleic Acids Res. (1994) 22:4673–80. doi: 10.1093/nar/22.22.4673
18. Lassmann T, Sonnhammer EL. Kalign – an accurate and fast multiple sequence alignment algorithm. BMC Bioinformatics. (2005) 6:298. doi: 10.1186/1471-2105-6-298
19. Nguyen DC, Scinicariello F, Attanasio R. Characterization and allelic polymorphisms of rhesus macaque (Macaca mulatta) IgG Fc receptor genes. Immunogenetics. (2011) 63:351–62. doi: 10.1007/s00251-011-0514-z
20. Lorenc A, Makałowski W. Transposable elements and vertebrate protein diversity. Genetica. (2003) 118:183–91. doi: 10.1023/A:1024105726123
21. Mitchell MA, Huang MM, Chien P, Indik ZK, Pan XQ, Schreiber AD. Substitutions and deletions in the cytoplasmic domain of the phagocytic receptor Fc gamma RIIA: effect on receptor tyrosine phosphorylation and phagocytosis. Blood. (1994) 84:1753–9.
22. Powell MS, Barnes NC, Bradford TM, Musgrave IF, Wines BD, Cambier JC, et al. Alteration of the Fc gamma RIIa dimer interface affects receptor signaling but not ligand binding. J Immunol. (2006) 176:7489–94. doi: 10.4049/jimmunol.176.12.7489
23. Van den Herik-Oudijk IE, Ter Bekke MW, Tempelman MJ, Capel PJ, Van de Winkel JG. Functional differences between two Fc receptor ITAM signaling motifs. Blood. (1995) 86:3302–7.
24. Deragon JM, Capy P. Impact of transposable elements on the human genome. Ann Med. (2000) 32:264–73. doi: 10.3109/07853890009011771
25. Qiu WQ, de Bruin D, Brownstein BH, Pearse R, Ravetch JV. Organization of the human and mouse low-affinity Fc gamma R genes: duplication and recombination. Science. (1990) 248:732–5. doi: 10.1126/science.2139735
26. Su K, Wu J, Edberg JC, McKenzie SE, Kimberly RP. Genomic organization of classical human low-affinity Fcgamma receptor genes. Genes Immun. (2002) 3(suppl. 1):S5156. doi: 10.1038/sj.gene.6363879
27. Feuk L, Carson AR, Scherer SW. Structural variation in the human genome. Nat Rev Genet. (2006) 7:85–97. doi: 10.1038/nrg1767
28. Steiper ME, Wolfe ND, Karesh WB, Kilbourn AM, Bosi EJ, Ruvolo M. The phylogenetic and evolutionary history of a novel alpha-globin-type gene in orangutans (Pongo pygmaeus). Infect Genet Evol. (2006) 6:277–86. doi: 10.1016/j.meegid.2005.08.001
29. Inoue K, Lupski JR. Molecular mechanisms for genomic disorders. Annu Rev Genomics Hum Genet. (2002) 3:199–242. doi: 10.1146/annurev.genom.3.032802.120023
30. Lupski JR. Genomic disorders: structural features of the genome can lead to DNA rearrangements and human disease traits. Trends Genet. (1998) 14:417–22. doi: 10.1016/S0168-9525(98)01555-8
31. Sharp AJ, Cheng Z, Eichler EE. Structural variation of the human genome. Annu Rev Genomics Hum Genet. (2006) 7:407–42. doi: 10.1146/annurev.genom.7.080505.115618
32. Aitman TJ, Dong R, Vyse TJ, Norsworthy PJ, Johnson MD, Smith J, et al. Copy number polymorphism in Fcgr3 predisposes to glomerulonephritis in rats and humans. Nature. (2006) 439:851–5. doi: 10.1038/nature04489
33. Fromont P, Bettaieb A, Skouri H, Floch C, Poulet E, Duedari N, et al. Frequency of the polymorphonuclear neutrophil Fc gamma receptor III deficiency in the French population and its involvement in the development of neonatal alloimmune neutropenia. Blood. (1992) 79:2131–4.
Keywords: FcgR, genetics, mammals, evolution, recombination, evolution, retroelement
Citation: Lejeune J, Brachet G and Watier H (2019) Evolutionary Story of the Low/Medium-Affinity IgG Fc Receptor Gene Cluster. Front. Immunol. 10:1297. doi: 10.3389/fimmu.2019.01297
Received: 18 December 2018; Accepted: 21 May 2019;
Published: 06 June 2019.
Edited by:
Falko Steinbach, University of Surrey, United KingdomReviewed by:
John Anthony Hammond, Pirbright Institute (BBSRC), United KingdomJeffrey V. Ravetch, The Rockefeller University, United States
Copyright © 2019 Lejeune, Brachet and Watier. This is an open-access article distributed under the terms of the Creative Commons Attribution License (CC BY). The use, distribution or reproduction in other forums is permitted, provided the original author(s) and the copyright owner(s) are credited and that the original publication in this journal is cited, in accordance with accepted academic practice. No use, distribution or reproduction is permitted which does not comply with these terms.
*Correspondence: Hervé Watier, watier@med.univ-tours.fr
†These authors have contributed equally to this work