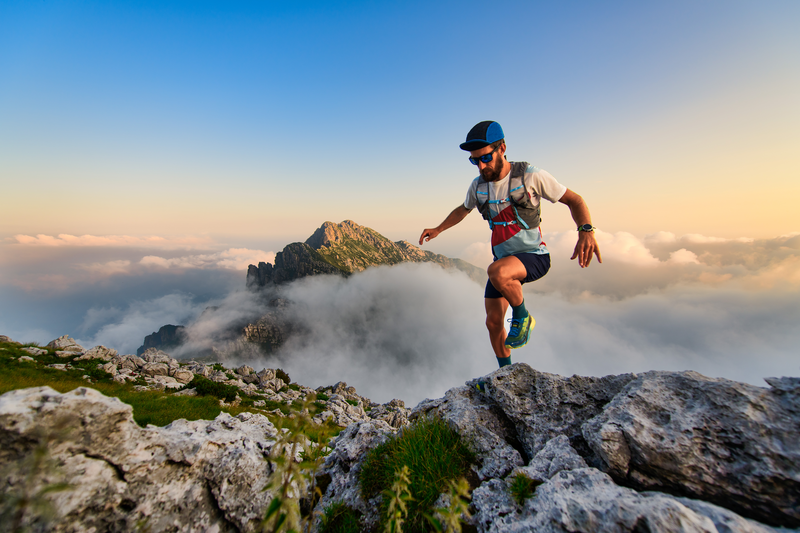
94% of researchers rate our articles as excellent or good
Learn more about the work of our research integrity team to safeguard the quality of each article we publish.
Find out more
REVIEW article
Front. Immunol. , 04 June 2019
Sec. Alloimmunity and Transplantation
Volume 10 - 2019 | https://doi.org/10.3389/fimmu.2019.01191
This article is part of the Research Topic Safety, Efficacy and Mechanisms of Action of Mesenchymal Stem Cell Therapies View all 22 articles
Expectations on mesenchymal stem cell (MSC) treatment are high, especially in the fields of sepsis, transplant medicine, and autoimmune diseases. Various pre-clinical studies have been conducted with encouraging results, although the mechanisms of action behind the observed immunomodulatory capacity of mesenchymal stem cells have not been fully understood. Previous studies have demonstrated that the immunomodulatory effect of MSCs is communicated via MSC-secreted cytokines and has been proven to rely on the local microenvironment as some of the observed effects depend on a pre-treatment of MSCs with inflammatory cytokines. Nonetheless, recent findings indicate that the cytokine-mediated effects are only one part of the equation as apoptotic, metabolically inactivated, or even fragmented MSCs have been shown to possess an immunomodulatory potential as well. Both cytokine-dependent and cytokine-independent mechanisms suggest a key role for regulatory T cells and monocytes in the overall pattern, but the principle as to why viable and non-viable MSCs have similar immunomodulatory capacities remains elusive. Here we review the current knowledge on cellular and molecular mechanisms involved in MSC-mediated immunomodulation and focus on the viability of MSCs, as there is still uncertainty concerning the tumorigenic potential of living MSCs.
Mesenchymal stem cell (MSC) therapy offers a promising treatment option for autoimmune diseases, sepsis, and in transplant surgery (1–7). However, the underlying cellular and molecular mechanisms of MSC-mediated immunomodulation have not been fully clarified. Studies have demonstrated various immunomodulatory changes following the administration of MSCs, although a clear picture is still missing and study results are often inconsistent. This might partially be explained by the fact that MSCs from different sources and under different culture conditions express different surface markers, show varying cytokine secretion profiles and differ in telomere-length and methylation patterns (8–15).
However, comparing the available data is complicated by a lack of standardization for the isolation, culture, and characterization of MSCs (16). MSCs can be harvested from various adult tissues such as bone marrow, adipose tissue, inner organs, and peripheral blood as well as from neonatal tissues (e.g., umbilical cord, placenta, amniotic fluid, amniotic membrane). In clinical studies, adipose tissue, and umbilical cord-derived MSCs have regularly been used due to their accessibility. The broad range of potential sources makes a comparison of study results challenging, as MSCs display varying features in vitro and in vivo depending on the tissue they originate from (17–19). In most study protocols MSCs were administered intravenously, yet in others they were delivered via an intraarterial, intraportal, intraperitoneal, or topical route or were administered directly into the damaged tissue (20–24).
Furthermore, freshly thawed MSCs seem to have an impaired immunomodulatory capacity compared to continuously cultured MSCs (25). The fact that MSCs act differently depending on the local microenvironment contributes even more to the complexity of understanding MSC-mediated immunomodulation (26–28). MSCs have a short half-life and cannot pass through the lung capillary network after IV administration, which appears to contradict the observed long-term immunomodulatory effects, particularly in transplant settings (29, 30).
Nevertheless, there are certain patterns and pathways that seem to be consistent and have been repeatedly demonstrated. MSC-mediated immunomodulation operates through a synergy of cell contact-dependent mechanisms and soluble factors (8, 31). MSCs reveal their immunomodulatory potential via functional changes of monocytes/macrophages, dendritic cells, T cells, B cells, and natural killer cells (6, 27, 32–36). In particular, anti-inflammatory monocytes/macrophages and regulatory T cells (Tregs) play a prominent role as they unfold their full immunomodulatory potential in a complex interaction catalyzed by MSCs (32, 37, 38). The interaction between MSCs, monocytes, and Tregs have often been attributed to MSC-secreted cytokines, although there is increasing evidence for mechanisms that rely on a direct cell-cell interaction, which—in the case of MSCs—does not necessarily require an intact cell metabolism (27, 31, 39, 40). Recent studies could demonstrate that apoptotic, metabolically inactivated, or even fragmented MSCs possess immunomodulatory capacities (21, 39, 41). As there are still ongoing concerns as to what extent living MSCs might contribute to tumorigenesis, the option to use dead cells or even cell fragments could be a promising alternative. This review summarizes the current knowledge on cellular and molecular interactions in MSC-derived immunomodulation by highlighting the different immune responses to living, apoptotic, and dead MSCs and provides an overview of the potential risks of MSC treatment in terms of tumor induction.
MSC were shown to promote the polarization of monocytes/macrophages toward an anti-inflammatory/immune-regulatory (type 2) phenotype and to directly inhibit the differentiation into the type 1 phenotype and dendritic cells (DCs) (10, 42–45). MSC-secreted Interleukin 1 Receptor Antagonist (IL1-RA) can promote the polarization of macrophages toward the type 2 phenotype (36). Anti-inflammatory monocytes secret high levels of IL-10 and have decreased levels of IL-12p70, TNF-a, and IL-17 expression—a process that is mediated by MSC-produced IL-6 and hepatocyte growth factor (HGF) (10, 40). A key role for the MSC-mediated, increased production of IL-10 has been demonstrated in a sepsis model in mice where IL-10 neutralization reversed the beneficial effect of bone marrow-derived MSCs on overall survival after induction of sepsis via cecal ligation and puncture (CLP) (6). Monocyte-derived IL-10 prevents monocyte differentiation into DCs and shifts monocytes toward an anti-inflammatory, IL-10-secreting subtype in terms of a positive-feedback loop (10). Apart from IL-10, MCS-primed monocytes express high levels of MHC class II, CD45R, and CD11b and seem to be able to suppress T-cell activity regardless of FoxP3+ Tregs (46). The supernatants of type 2 macrophages induce the formation of FoxP3+ Tregs from naïve CD4+ T cells, which emphasizes the role of soluble factors in MSC-mediated immunomodulation (47). The monocyte-induced Treg-formation is mediated by monocyte-produced CCL-18 and monocyte-released transforming growth factor beta 1 (TGF- β1) (45, 47). Macrophages bind and re-release TGF-β1 during their differentiation into type 2 macrophages and might thereby contribute to the MSC-induced formation of Tregs as MSCs have been shown to secrete TGF-β1 (45, 47). The neutralization of CCL-18 leads to a significant reduction in MSC-induced Treg formation (45). CCL-18 can turn memory CD4+ T cells into to CD4+CD25+Foxp3+ Tregs with an increased IL-10 and TGF- β1 production. CCL-18-pretreated Tregs inhibit CD4+CD25− effector T cell proliferation via the activation of G-protein-coupled receptors (48). Macrophage type 2-derived CCL-18 can differentiate DCs into tolerogenic DCs, which are in turn able to prime Tregs (45, 48, 49) (Figure 1A). Interestingly, high concentrations of CCL-18 producing antigen-presenting cells can be found in the lungs, where MSCs become caught in the capillary system after IV application (50–52) (Figure 1B).
Figure 1. (A) Proposed interaction of MSCs with host immune cells. (B) Proposed pathway of MSC-mediated anti-inflammation via phagocytosis of MSCs [in accordance with De Witte et al. (31) and Braza et al. (53)].
MSCs also suppress the migration and maturation of DCs (32). In the presence of MSCs, DCs are less capable to support antigen specific CD4+ T cell proliferation and to display an MHC class II-peptide complex (54). Mature type 1 DCs secrete significantly less TNF-α, if co-cultured with MSCs and anti-inflammatory mature type 2 DCs show an increased IL-10 secretion (55). Furthermore, Sca-1+CD117−Lin− bone marrow-derived MSCs have been shown to generate regulatory DCs with immune regulatory function from hematopoietic stem cells in mice (56).
Recently, a cytokine-independent pathway for the MSC-induced polarization of monocytes/macrophages has been revealed. In a mouse model of asthma, MSCs were phagocytosed by lung macrophages. The phagocytosis of MSCs caused monocytes to turn into a type 2 immunosuppressive phenotype (53). A previous study from 2012 observed a similar pattern in a p. aeruginosa peritonitis model in mice (57). The intravenous application of bone marrow-derived MSCs was followed by an increased phagocytic activity in blood monocytes compared with the PBS control group. Furthermore, an increase in alternately activated CD163+CD206+ monocytes/macrophages in the spleen of the MSC-treated mice could be observed (57). De Witte et al. (31) could show that phagocytosed MSCs are mainly found in non-classical Ly6Clow monocytes. The phagocytosis of MSCs caused CD14++CD16− classical monocytes to polarize toward a CD14++CD16+CD206+ immune regulatory intermediate subtype with anti-inflammatory properties and increased expression of IL-10 and programmed death ligand-1 (PD-L1). After phagocytosis of MSCs, these primed monocytes were able to induce CD4+CD25hi Treg formation in vitro to a significantly higher extent than un-primed monocytes (31). Likewise, an increase in anti-inflammatory Ly6Clow monocytes in the blood, heart, and spleen was observed after IV administration of MSCs in a Coxsackievirus B3-induced myocarditis model in mice (58).
The depletion of phagocytic cells demonstrates their indispensable role in MSC-mediated immunomodulation as the absence of monocytes/macrophages and dendritic cells abrogates the ability of MSCs to suppress T cell proliferation in vitro and their immunomodulatory effect in in vivo transplant models (59).
MSCs suppress T cell proliferation (CD4+ and CD8+ T cell subsets) in mixed lymphocyte reactions in a dose-dependent manner (39, 60, 61). In interaction with DCs, MSCs cause a shift from pro-inflammatory Th1 to anti-inflammatory Th2 cells including a change in the cytokine profile toward anti-inflammation (32, 62–64). Moreover, MSCs facilitate the formation of Tregs in vitro and in vivo (3, 32, 55). Tregs are essential for the immune homeostasis by preventing autoimmunity (65, 66). The induction of CD4+CD25+FoxP3+ Tregs is a mainstay in MSC-mediated immunomodulation and was shown to be essential for tolerance induction in a kidney allograft transplantation model (32). The MSC-induced upregulation of Tregs does not result from an expansion of pre-existing natural Tregs but via induction of Tregs from conventional T cells (67, 68). TGF-β1-neutralization studies have shown that the generation of Tregs is TGF-β1-mediated and that MSCs constitutively secrete TGF-β1. However, TGF-β1 seems not to be sufficient on its own, as the presence of monocytes was proven to be essential for the formation of Tregs (45). In co-treatment with MMF, MSCs have been shown to promote a direct conversion of IL-17A+ cells into IL-17AnegFoxp3+ Tregs (35). MSCs also constitutively secrete indoleamine 2,3-dioxygenase (IDO) and the secretion is increased upon stimulation by INF-γ. The consecutive tryptophan depletion leads to an inhibition of allogeneic T cell responses, stimulates the secretion of IL-4 in Th2 cells and decreases the IFN-γ production by Th1 cells (27, 32, 55, 69, 70). Gieseke et al. have shown that MSCs can directly inhibit the proliferation of alloreactive CD4+ and CD8+ T cells without the presence of other immune cells and that this process is partially mediated by MSC-derived galectin-1 (60). Via the secretion of PD-L1, MSCs can suppress T cell activation and induce an irreversible T cell hypo-responsiveness and apoptosis (71, 72) (Figure 1A).
MSCs directly interact with B cells and are able to reduce plasmablast formation as well as to promote the induction of regulatory B cells (Bregs) (73). Bregs have immunosuppressive properties through which they provide immunological tolerance (74). IL-10-producing Bregs were shown to convert effector CD4+ T cells into Foxp3+ Tregs (75). The stimulatory effect of MSCs on Breg formation and IL-10 production is not mediated via soluble factors but seems to be dependent on direct cell-cell contact or at least on a close proximity of the corresponding cells (27). However, it was shown that the stimulatory effect of MSCs on Breg formation and their suppressive effect on T cell proliferation requires an active cell metabolism (27, 41). Luz-Crawford et al. revealed a cytokine-triggered mechanism, by which MSC-secreted IL1-RA inhibits B cell differentiation (36). In the presence of T cells, MSCs also inhibit the proliferation of B cells, which could be due to T cell-secreted IFN-γ as IFN-γ pre-treated MSCs are able to inhibit B cell proliferation (27).
MSC are also strong inhibitors of natural killer cell (NK cell) proliferation. NK cells show an impaired cytotoxic activity and cytokine production after co-culture with MSCs. There is evidence that the inhibitory effect of MSCs on NK cells involves MSC-secreted prostaglandin E2 (PGE2), IDO, TGF-β1, IL-6 and nitric oxide (NO) (10, 33, 76) (Figure 1A).
The viability of MSCs does not appear to be a prerequisite for some of their immunomodulatory effects. Apoptotic adipose tissue-derived MSCs (A-ADMSCs) have been shown to reduce mortality in rats after sepsis induction via CLP (21) (Table 1). Mortality, circulating TNF-α level as well as circulating and splenic levels of T helper cells and cytotoxic T cells following CLP were significantly lower in the group treated with A-ADMSCs compared to CLP alone (21). A study group around Chen et al. provided similar results in a CLP-induced model of acute kidney injury in mice with a reduced splenic level of T helper cells and cytotoxic T cells as well as a lower circulating TNF-α level in the group treated with intravenous A-ADMSCs compared to the CLP control group (77). Interestingly, the study by Chang et al. could not prove any benefit and even a trend toward a reduced survival after administration of living MSCs (21). In keeping with the results of Chang et al. it was demonstrated that an IV A-ADMSC treatment is superior to a treatment with living MSCs in a CLP-induced sepsis model in rats (78). The parameters for sepsis-induced acute lung injury (ALI) and acute kidney injury were significantly lower in the group treated with apoptotic MSCs. Furthermore, A-ADMSC treatment was more effective in reducing inflammation, oxidative stress, and apoptosis as well as sepsis-induced histopathological alterations in the lungs and kidneys compared to living MSCs (78). Likewise, A-ADMSCs were shown to be superior to living MSCs for the treatment of an acute lung ischemia-reperfusion injury in rats if administered along with melatonin (79).
However, there are several studies that demonstrated a significant positive effect of living MSCs in the attenuation of sepsis in different animal models (22, 81–83). Interestingly, a recent study demonstrated that recipient cytotoxic cells cause perforin-induced apoptosis in infused MSCs (80). The apoptosis of MSCs was the prerequisite for MSCs to unfold their immune-regulatory effect in a murine graft vs. host model. Hence, the cytotoxic activity against MSCs was demonstrated to be a crucial part in MSC-mediated immunomodulation. The recognition by cytotoxic cells in this model was shown to be MHC-independent and non–antigen-specific. Moreover, the use of apoptotic MSCs skipped the need for recipient cytotoxic cells. Apoptotic MSCs were immunosuppressive in a TH2-type inflammation model and induced the IDO production in recipient phagocytes (80) (Table 1). Another study provided evidence that the supernatants of macrophages that phagocytized apoptotic mesenchymal stem cells improve the survival of hypoxic cardiomyocytes (84). These findings are in keeping with the “dying stem cell hypothesis” published by Thum in 2005, which stated that the apoptosis of MSCs causes a modulation of the local immune response with a down-regulation of the innate and adaptive immunity (85).
Contrary findings were provided in an endotoxin-induced ALI model in mice. The intrapulmonary administration of apoptotic MSCs did not improve survival or decrease the severity of an endotoxin-induced ALI. Moreover, no decrease in TNF-α levels and no increase in IL-10 levels could be observed, neither in plasma nor in fluid from bronchoalveolar lavage (22). Compared with the above mentioned studies, the intrapulmonary application via trachea/bronchi was a unique characteristic of this study compared to the commonly used IV route and might explain the differing results.
In 2016, a heat-inactivation protocol for MSCs was introduced, in which human MSCs were heated for 30 min to 50°C (41). Heat-inactivation causes an irreversible cessation of the metabolic and proliferative activity of MSCs. HI-MSCs do not secrete cytokines but their cell integrity remains largely intact. Over the course of time, HI-MSCs are subject to physical disintegration rather than to apoptosis, as they do not overexpress heat shock proteins Hsp27 and Hsp70 and pro-apoptotic Bax (41). Therefore, in this review heat-inactivated MSCs (HI-MSCs) are referred to as being “dead.” In contrast to living MSCs, HI-MSCs do not inhibit T cell proliferation and do not induce Breg formation in mixed lymphocyte reactions. However, HI-MSCs are still able to attenuate the inflammatory response in mice following LPS-administration. After administration of HI-MSCs, serum levels of IFN-γ were significantly reduced whereas IL-10 serum levels were increased (41). MSCs and HI-MSCs show similar effects on monocyte function with significantly reduced production of TNF-α in response to lipopolysaccharide (41). Even MSC-derived membrane particles seem to possess immunomodulatory properties. Goncalves et al. used MSC-derived membrane particles with a size ranging between 63 and 700 nm (>95% smaller than 200 nm). These MSC-derived membrane particles were shown to be enzymatically active but did not suppress T cell proliferation in mixed lymphocyte reactions. The MSC membrane particles were taken up by monocytes and became bound to their plasma membranes thus inducing selective apoptosis of pro-inflammatory CD14+CD16+ monocytes (39) (Table 1).
There is an ongoing discussion, as to whether MSCs are able to migrate to the site of inflammation/tissue damage or to a transplanted organ. In that context, it is worthwhile to differentiate between endogenous MSCs and exogenously administered MSCs. The data concerning the migratory capacity of endogenous MSCs is controversial and there is no convincing evidence that endogenous MSCs find their way to the site of inflammation/tissue damage via the bloodstream (86). Nevertheless, endogenous MSCs seem to be able to migrate within the tissues and might thereby reach the damaged or inflamed areas (50). A recently published study revealed that exogenous MSCs, if transplanted directly into the tissue, could survive up to 4 months at the effector site with few of these transplanted MSCs beginning to develop a resident cell tissue phenotype (23).
However, in most clinical and pre-clinical models MSCs were administered intravenously. Interestingly, the majority of IV-administered MSCs does not pass the capillary system of the lungs, which contradicts the suggestion that MSCs exert their immunomodulatory effect by migrating to the sites of harm. Within 24 h after being trapped in the pulmonary system a major decrease in the number of viable MSCs can be observed (29, 30). The fate of these MSCs was further unveiled by demonstrating that MSCs become phagocytosed by blood-derived monocytes and neutrophils after their initial entrapment in the lungs and thereby become redistributed systemically with a major accumulation in the liver and the spleen (31, 87) (Figure 1B).
The change in the monocytes' profile toward an immuno-regulatory phenotype after phagocytosis of MSCs followed by their systemic redistribution might be the key mechanism to explain the contradictory findings with regards to the short half-life of MSCs and the long lasting immunomodulatory effects that have been observed (31, 35).
It remains unclear, whether living MSCs are subject to phagocytosis by host-innate immune cells or if MSCs have to undergo apoptosis in order to become phagocytosed. Galleu et al. have shown that infused living MSCs are subject to perforin-induced apoptosis through recipient cytotoxic cells (80). These findings emphasize the importance of apoptosis in MSC-mediated immunomodulation and might explain previous study results, in which apoptotic MSCs were shown to be superior compared to living MSCs (21, 78, 79). Nevertheless, apoptosis cannot explain by which means dead HI-MSCs—which are subject to physical disintegration rather than to apoptosis—unfold their immunomodulatory potential (27, 41).
Allogeneic MSCs are frequently used in clinical studies even though their immunogenic potential has not always been taken into account (88). In the past, MSCs were thought to be immune privileged. Several pre-clinical studies could show that both, autologous and allogenic MSCs suppress T cell proliferation in mixed lymphocyte reactions in a dose-dependent manner (61, 89). In animal models, donor-derived MSCs prolonged the survival of semi-allogeneic heart transplants in mice via the generation of Tregs and a shift in the Th1/Th2 balance toward Th2 (90, 91). Interestingly, the IV administration of MSCs is followed by a systemic inflammatory response with an increase in macrophages in lung tissue 2 h after infusion and increased serum levels of pro-inflammatory IL-6, CXCL1, and monocyte chemoattractant protein-1. The phase of acute inflammatory response seems to be followed by a phase with reduced immune reactivity, which might partially explain the increased allograft survival in animal models, in which MSCs were administered prior to transplantation (34, 59, 92).
However, donor-derived MSCs given prior to transplantation only prolonged the survival of allogenic heart grafts if a short course of mycophenolate mofetil (MMF) was administered from the day of transplantation onwards. The administration of donor-derived MSCs alone caused a prompt T cell infiltration of the grafts with consecutive graft rejection, which supports the assumption that allogeneic MSCs are immunogenic and sensitize the recipient (34, 59). It has been demonstrated that allogenic MSCs can activate T cells (89, 93). The additional treatment with MMF seems to eliminate these activated T cells with a consecutive reduction of T cell infiltration in the respective allografts (59).
Further evidence that MSCs are not immune privileged has been provided in a study, in which syngeneic, erythropoietin-releasing MSCs persisted for more than 200 days, whereas allogeneic MSCs were rapidly rejected (94). Zangi et al. provided similar findings with luciferase-labeled MSCs. The survival of allogeneic MSCs was significantly shorter compared to syngeneic MSCs. Moreover, allogeneic MSCs seem to induce an immune memory represented by an increase in T cells with a memory phenotype (95).
It can be assumed that MSCs are not immune privileged, but rather that allogenic MSCs have a lower immunogenic potential as other allogeneic cell types (88). As the cytotoxic cell-induced apoptosis of MSCs was described to be essential for the MSC-mediated immunomodulation and because this effect was shown to be MHC-independent and non–antigen-specific, the allogenic component might be of secondary importance (80).
Previous studies have suggested that MSCs could favor tumor growth in vivo (96, 97). It has been reported that implanted MSCs cause an earlier onset of syngeneic tumors and allow B16 melanoma cells to form tumors in allogenic mice (98). Furthermore, TNFα-activated MSCs can facilitate tumor growth and promote cancer metastasis via CXCR2+ neutrophil recruitment (99).
The mechanism behind MSC-induced tumor growth involves the formation of carcinoma associated fibroblasts (CAFs). Human bone marrow-derived MSCs (BM-MSCs) were shown to adopt a CAF-like phenotype with similar functional properties after prolonged exposure to tumor-conditioned medium (100, 101). CAFs and CAF-like MSCs produce growth factors, cytokines, and chemokines, and thereby provide the microvascularization and the stromal network for tumor progression (102). In a mouse model of inflammation-induced gastric cancer, at least 20% of CAFs derived from the bone marrow and developed from MSCs (103).
Furthermore, MSCs seem to have a distinct tropism for tumors as BM-MSCs were shown to accumulate in brain tumors after intracarotid injection, whereas fibroblasts and U87 glioma cells did not (20). This tropism was further elucidated in a study from 2013 that unveiled an active recruitment of MSCs to prostate cancer via prostate cancer-secreted CXCL-16. CXCL-16 binds to CXCR6 expressed by MSCs. The CXCL16/CXCR6 signaling induces the conversion of MSCs into CAFs (104). Ren et al. could show that under inflammatory conditions CAF-like MSCs stimulate tumor growth via the recruitment of monocytes and macrophages. The essential role monocytes/macrophages in MSC-mediated immunomodulation was demonstrated once more as their depletion abrogated the promotion of tumor growth by lymphoma isolated-MSCs (105).
Whilst MSCs can exhibit immunosuppressive or immune-enhancing properties depending on the presence or absence of certain inflammatory or anti-inflammatory stimuli, the tumorigenic potential of living MSCs poses a risk that cannot be neglected (26, 28, 106). As dead MSCs have no active cell metabolism, it can be assumed that they do not differentiate into CAF-like cells with the corresponding secretion of growth factors, cytokines, and chemokines. However, a clear discrimination between CAFs, CAF-like cells, and MSCs is still missing in the current literature. Furthermore, dead MSCs may still provoke changes in the tumor microenvironment due to their immunomodulatory properties. Therefore, the question whether dead or fragmented MSCs are beneficial in terms of a reduced cancer risk cannot be answered, yet.
It remains a challenge to connect the dots between the various MSC-mediated immunomodulatory effects, especially as MSCs are very heterogenic and subject to significant changes upon inflammatory or anti-inflammatory stimuli. Viable MSCs might provoke more complex immunomodulatory mechanisms due to their intact secretome. However, since the discussion about a universal donor for MSC therapy has not been finally answered, the possibility to use dead MSCs should also be considered. HI-MSCs or fragmented MSCs are most likely not subject to changes in their immunomodulatory characteristics upon different environmental stimuli. Hence, their immunomodulatory effects might be more predictable, which would allow a better comparison of future study results.
AW drafted and wrote the manuscript. MD drafted and critically revised the manuscript. Both authors have approved the manuscript for publication.
The authors declare that the research was conducted in the absence of any commercial or financial relationships that could be construed as a potential conflict of interest.
The authors want to thank Prof. Schlitt and his team in the surgical department at the University Hospital Regensburg for their support.
1. Le Blanc K, Frassoni F, Ball L, Locatelli F, Roelofs H, Lewis I, et al. Mesenchymal stem cells for treatment of steroid-resistant, severe, acute graft-versus-host disease: a phase II study. Lancet. (2008) 371:1579–86. doi: 10.1016/S0140-6736(08)60690-X
2. Forbes GM, Sturm MJ, Leong RW, Sparrow MP, Segarajasingam D, Cummins AG, et al. A phase 2 study of allogeneic mesenchymal stromal cells for luminal Crohn's disease refractory to biologic therapy. Clin Gastroenterol Hepatol. (2014) 12:64–71. doi: 10.1016/j.cgh.2013.06.021
3. Gonzalez MA, Gonzalez-Rey E, Rico L, Buscher D, Delgado M. Adipose-derived mesenchymal stem cells alleviate experimental colitis by inhibiting inflammatory and autoimmune responses. Gastroenterology. (2009) 136:978–89. doi: 10.1053/j.gastro.2008.11.041
4. Reinders ME, de Fijter JW, Roelofs H, Bajema IM, de Vries DK, Schaapherder AF, et al. Autologous bone marrow-derived mesenchymal stromal cells for the treatment of allograft rejection after renal transplantation: results of a phase I study. Stem Cells Transl Med. (2013) 2:107–11. doi: 10.5966/sctm.2012-0114
5. Bartholomew A, Sturgeon C, Siatskas M, Ferrer K, McIntosh K, Patil S, et al. Mesenchymal stem cells suppress lymphocyte proliferation in vitro and prolong skin graft survival in vivo. Exp Hematol. (2002) 30:42–8. doi: 10.1016/S0301-472X(01)00769-X
6. Nemeth K, Leelahavanichkul A, Yuen PS, Mayer B, Parmelee A, Doi K, et al. Bone marrow stromal cells attenuate sepsis via prostaglandin E(2)-dependent reprogramming of host macrophages to increase their interleukin-10 production. Nat Med. (2009) 15:42–9. doi: 10.1038/nm.1905
7. Hu J, Yu X, Wang Z, Wang F, Wang L, Gao H, et al. Long term effects of the implantation of Wharton's jelly-derived mesenchymal stem cells from the umbilical cord for newly-onset type 1 diabetes mellitus. Endocr J. (2013) 60:347–57. doi: 10.1507/endocrj.EJ12-0343
8. Wu Y, Hoogduijn MJ, Baan CC, Korevaar SS, de Kuiper R, Yan L, et al. Adipose Tissue-derived mesenchymal stem cells have a heterogenic cytokine secretion profile. Stem Cells Int. (2017) 2017:4960831. doi: 10.1155/2017/4960831
9. Nasef A, Mathieu N, Chapel A, Frick J, Francois S, Mazurier C, et al. Immunosuppressive effects of mesenchymal stem cells: involvement of HLA-G. Transplantation. (2007) 84:231–7. doi: 10.1097/01.tp.0000267918.07906.08
10. Deng Y, Zhang Y, Ye L, Zhang T, Cheng J, Chen G, et al. Umbilical cord-derived mesenchymal stem cells instruct monocytes towards an IL10-producing phenotype by secreting IL6 and HGF. Sci Rep. (2016) 6:37566. doi: 10.1038/srep37566
11. De Witte SFH, Peters FS, Merino A, Korevaar SS, Van Meurs JBJ, O'Flynn L, et al. Epigenetic changes in umbilical cord mesenchymal stromal cells upon stimulation and culture expansion. Cytotherapy. (2018) 20:919–29. doi: 10.1016/j.jcyt.2018.05.005
12. Bonab MM, Alimoghaddam K, Talebian F, Ghaffari SH, Ghavamzadeh A, Nikbin B. Aging of mesenchymal stem cell in vitro. BMC Cell Biol. (2006) 7:14. doi: 10.1186/1471-2121-7-14
13. Schellenberg A, Lin Q, Schuler H, Koch CM, Joussen S, Denecke B, et al. Replicative senescence of mesenchymal stem cells causes DNA-methylation changes which correlate with repressive histone marks. Aging. (2011) 3:873–88. doi: 10.18632/aging.100391
14. Baxter MA, Wynn RF, Jowitt SN, Wraith JE, Fairbairn LJ, Bellantuono I. Study of telomere length reveals rapid aging of human marrow stromal cells following in vitro expansion. Stem Cells. (2004) 22:675–82. doi: 10.1634/stemcells.22-5-675
15. de Witte SFH, Lambert EE, Merino A, Strini T, Douben HJCW, O'Flynn L, et al. Aging of bone marrow- and umbilical cord-derived mesenchymal stromal cells during expansion. Cytotherapy. (2017) 19:798–807. doi: 10.1016/j.jcyt.2017.03.071
16. Yin JQ, Zhu J, Ankrum JA. Manufacturing of primed mesenchymal stromal cells for therapy. Nat Biomed Eng. (2019) 28:90–104. doi: 10.1038/s41551-018-0325-8
17. Elahi KC, Klein G, Avci-Adali M, Sievert KD, MacNeil S, Aicher WK. Human Mesenchymal stromal cells from different sources diverge in their expression of cell surface proteins and display distinct differentiation patterns. Stem Cells Int. (2016) 2016:5646384. doi: 10.1155/2016/5646384
18. Hass R, Kasper C, Bohm S, Jacobs R. Different populations and sources of human mesenchymal stem cells (MSC): a comparison of adult and neonatal tissue-derived MSC. Cell Commun Signal. (2011) 9:12. doi: 10.1186/1478-811X-9-12
19. da Silva Meirelles L, Chagastelles PC, Nardi NB. Mesenchymal stem cells reside in virtually all post-natal organs and tissues. J Cell Sci. (2006) 119(Pt 11):2204–13. doi: 10.1242/jcs.02932
20. Nakamizo A, Marini F, Amano T, Khan A, Studeny M, Gumin J, et al. Human bone marrow-derived mesenchymal stem cells in the treatment of gliomas. Cancer Res. (2005) 65:3307–18. doi: 10.1158/0008-5472.CAN-04-1874
21. Chang CL, Leu S, Sung HC, Zhen YY, Cho CL, Chen A, et al. Impact of apoptotic adipose-derived mesenchymal stem cells on attenuating organ damage and reducing mortality in rat sepsis syndrome induced by cecal puncture and ligation. J Transl Med. (2012) 10:244. doi: 10.1186/1479-5876-10-244
22. Gupta N, Su X, Popov B, Lee JW, Serikov V, Matthay MA. Intrapulmonary delivery of bone marrow-derived mesenchymal stem cells improves survival and attenuates endotoxin-induced acute lung injury in mice. J Immunol. (2007) 179:1855–63. doi: 10.4049/jimmunol.179.3.1855
23. Munoz MF, Arguelles S, Guzman-Chozas M, Guillen-Sanz R, Franco JM, Pintor-Toro JA, et al. Cell tracking, survival, and differentiation capacity of adipose-derived stem cells after engraftment in rat tissue. J Cell Physiol. (2018) 233:6317–28. doi: 10.1002/jcp.26439
24. Avritscher R, Abdelsalam ME, Javadi S, Ensor J, Wallace MJ, Alt E, et al. Percutaneous intraportal application of adipose tissue-derived mesenchymal stem cells using a balloon occlusion catheter in a porcine model of liver fibrosis. J Vasc Interv Radiol. (2013) 24:1871–8. doi: 10.1016/j.jvir.2013.08.022
25. Moll G, Geissler S, Catar R, Ignatowicz L, Hoogduijn MJ, Strunk D, et al. Cryopreserved or fresh mesenchymal stromal cells: only a matter of taste or key to unleash the full clinical potential of MSC therapy? Adv Exp Med Biol. (2016) 951:77–98. doi: 10.1007/978-3-319-45457-3_7
26. Wang Y, Chen X, Cao W, Shi Y. Plasticity of mesenchymal stem cells in immunomodulation: pathological and therapeutic implications. Nat Immunol. (2014) 15:1009–16. doi: 10.1038/ni.3002
27. Luk F, Carreras-Planella L, Korevaar SS, de Witte SFH, Borras FE, Betjes MGH, et al. Inflammatory conditions dictate the effect of mesenchymal stem or stromal cells on B cell function. Front Immunol. (2017) 8:1042. doi: 10.3389/fimmu.2017.01042
28. de Witte SFH, Merino AM, Franquesa M, Strini T, van Zoggel JAA, Korevaar SS, et al. Cytokine treatment optimises the immunotherapeutic effects of umbilical cord-derived MSC for treatment of inflammatory liver disease. Stem Cell Res Ther. (2017) 8:140. doi: 10.1186/s13287-017-0590-6
29. Eggenhofer E, Benseler V, Kroemer A, Popp FC, Geissler EK, Schlitt HJ, et al. Mesenchymal stem cells are short-lived and do not migrate beyond the lungs after intravenous infusion. Front Immunol. (2012) 3:297. doi: 10.3389/fimmu.2012.00297
30. Fischer UM, Harting MT, Jimenez F, Monzon-Posadas WO, Xue H, Savitz SI, et al. Pulmonary passage is a major obstacle for intravenous stem cell delivery: the pulmonary first-pass effect. Stem Cells Dev. (2009) 18:683–91. doi: 10.1089/scd.2008.0253
31. de Witte SFH, Luk F, Sierra Parraga JM, Gargesha M, Merino A, Korevaar SS, et al. Immunomodulation by therapeutic mesenchymal stromal cells (MSC) is triggered through phagocytosis of msc by monocytic cells. Stem Cells. (2018) 36:602–15. doi: 10.1002/stem.2779
32. Ge W, Jiang J, Arp J, Liu W, Garcia B, Wang H. Regulatory T-cell generation and kidney allograft tolerance induced by mesenchymal stem cells associated with indoleamine 2,3-dioxygenase expression. Transplantation. (2010) 90:1312–20. doi: 10.1097/TP.0b013e3181fed001
33. Spaggiari GM, Capobianco A, Abdelrazik H, Becchetti F, Mingari MC, Moretta L. Mesenchymal stem cells inhibit natural killer-cell proliferation, cytotoxicity, and cytokine production: role of indoleamine 2,3-dioxygenase and prostaglandin E2. Blood. (2008) 111:1327–33. doi: 10.1182/blood-2007-02-074997
34. Popp FC, Eggenhofer E, Renner P, Slowik P, Lang SA, Kaspar H, et al. Mesenchymal stem cells can induce long-term acceptance of solid organ allografts in synergy with low-dose mycophenolate. Transpl Immunol. (2008) 20:55–60. doi: 10.1016/j.trim.2008.08.004
35. Obermajer N, Popp FC, Soeder Y, Haarer J, Geissler EK, Schlitt HJ, et al. Conversion of Th17 into IL-17A(neg) regulatory T cells: a novel mechanism in prolonged allograft survival promoted by mesenchymal stem cell-supported minimized immunosuppressive therapy. J Immunol. (2014) 193:4988–99. doi: 10.4049/jimmunol.1401776
36. Luz-Crawford P, Djouad F, Toupet K, Bony C, Franquesa M, Hoogduijn MJ, et al. Mesenchymal stem cell-derived interleukin 1 receptor antagonist promotes macrophage polarization and inhibits B cell differentiation. Stem Cells. (2016) 34:483–92. doi: 10.1002/stem.2254
37. Eggenhofer E, Popp FC, Mendicino M, Silber P, Van't Hof W, Renner P, et al. Heart grafts tolerized through third-party multipotent adult progenitor cells can be retransplanted to secondary hosts with no immunosuppression. Stem Cells Transl Med. (2013) 2:595–606. doi: 10.5966/sctm.2012-0166
38. Riquelme P, Haarer J, Kammler A, Walter L, Tomiuk S, Ahrens N, et al. TIGIT(+) iTregs elicited by human regulatory macrophages control T cell immunity. Nat Commun. (2018) 9:2858. doi: 10.1038/s41467-018-05167-8
39. Goncalves FDC, Luk F, Korevaar SS, Bouzid R, Paz AH, Lopez-Iglesias C, et al. Membrane particles generated from mesenchymal stromal cells modulate immune responses by selective targeting of pro-inflammatory monocytes. Sci Rep. (2017) 7:12100. doi: 10.1038/s41598-017-12121-z
40. Melief SM, Geutskens SB, Fibbe WE, Roelofs H. Multipotent stromal cells skew monocytes towards an anti-inflammatory interleukin-10-producing phenotype by production of interleukin-6. Haematologica. (2013) 98:888–95. doi: 10.3324/haematol.2012.078055
41. Luk F, de Witte SF, Korevaar SS, Roemeling-van Rhijn M, Franquesa M, Strini T, et al. Inactivated mesenchymal stem cells maintain immunomodulatory capacity. Stem Cells Dev. (2016) 25:1342–54. doi: 10.1089/scd.2016.0068
42. Zheng G, Huang R, Qiu G, Ge M, Wang J, Shu Q, et al. Mesenchymal stromal cell-derived extracellular vesicles: regenerative and immunomodulatory effects and potential applications in sepsis. Cell Tissue Res. (2018) 374:1–15. doi: 10.1007/s00441-018-2871-5
43. Ramasamy R, Fazekasova H, Lam EW, Soeiro I, Lombardi G, Dazzi F. Mesenchymal stem cells inhibit dendritic cell differentiation and function by preventing entry into the cell cycle. Transplantation. (2007) 83:71–6. doi: 10.1097/01.tp.0000244572.24780.54
44. Jiang XX, Zhang Y, Liu B, Zhang SX, Wu Y, Yu XD, et al. Human mesenchymal stem cells inhibit differentiation and function of monocyte-derived dendritic cells. Blood. (2005) 105:4120–6. doi: 10.1182/blood-2004-02-0586
45. Melief SM, Schrama E, Brugman MH, Tiemessen MM, Hoogduijn MJ, Fibbe WE, et al. Multipotent stromal cells induce human regulatory T cells through a novel pathway involving skewing of monocytes toward anti-inflammatory macrophages. Stem Cells. (2013) 31:1980–91. doi: 10.1002/stem.1432
46. Ko JH, Lee HJ, Jeong HJ, Kim MK, Wee WR, Yoon SO, et al. Mesenchymal stem/stromal cells precondition lung monocytes/macrophages to produce tolerance against allo- and autoimmunity in the eye. Proc Natl Acad Sci USA. (2016) 113:158–63. doi: 10.1073/pnas.1522905113
47. Schmidt A, Zhang XM, Joshi RN, Iqbal S, Wahlund C, Gabrielsson S, et al. Human macrophages induce CD4(+)Foxp3(+) regulatory T cells via binding and re-release of TGF-β. Immunol Cell Biol. (2016) 94:747–62. doi: 10.1038/icb.2016.34
48. Chang Y, de Nadai P, Azzaoui I, Morales O, Delhem N, Vorng H, et al. The chemokine CCL18 generates adaptive regulatory T cells from memory CD4+ T cells of healthy but not allergic subjects. FASEB J. (2010) 24:5063–72. doi: 10.1096/fj.10-162560
49. Azzaoui I, Yahia SA, Chang Y, Vorng H, Morales O, Fan Y, et al. CCL18 differentiates dendritic cells in tolerogenic cells able to prime regulatory T cells in healthy subjects. Blood. (2011) 118:3549–58. doi: 10.1182/blood-2011-02-338780
50. Eggenhofer E, Luk F, Dahlke MH, Hoogduijn MJ. The life and fate of mesenchymal stem cells. Front Immunol. (2014) 5:148. doi: 10.3389/fimmu.2014.00148
51. Kodelja V, Muller C, Politz O, Hakij N, Orfanos CE, Goerdt S. Alternative macrophage activation-associated CC-chemokine-1, a novel structural homologue of macrophage inflammatory protein-1 alpha with a Th2-associated expression pattern. J Immunol. (1998) 160:1411–8.
52. Vulcano M, Struyf S, Scapini P, Cassatella M, Bernasconi S, Bonecchi R, et al. Unique regulation of CCL18 production by maturing dendritic cells. J Immunol. (2003) 170:3843–9. doi: 10.4049/jimmunol.170.7.3843
53. Braza F, Dirou S, Forest V, Sauzeau V, Hassoun D, Chesne J, et al. Mesenchymal stem cells induce suppressive macrophages through phagocytosis in a mouse model of asthma. Stem Cells. (2016) 34:1836–45. doi: 10.1002/stem.2344
54. English K, Barry FP, Mahon BP. Murine mesenchymal stem cells suppress dendritic cell migration, maturation and antigen presentation. Immunol Lett. (2008) 115:50–8. doi: 10.1016/j.imlet.2007.10.002
55. Aggarwal S, Pittenger MF. Human mesenchymal stem cells modulate allogeneic immune cell responses. Blood. (2005) 105:1815–22. doi: 10.1182/blood-2004-04-1559
56. Liu X, Ren S, Ge C, Cheng K, Zenke M, Keating A, et al. Sca-1+Lin-CD117- mesenchymal stem/stromal cells induce the generation of novel IRF8-controlled regulatory dendritic cells through Notch-RBP-J signaling. J Immunol. (2015) 194:4298–308. doi: 10.4049/jimmunol.1402641
57. Krasnodembskaya A, Samarani G, Song Y, Zhuo H, Su X, Lee JW, et al. Human mesenchymal stem cells reduce mortality and bacteremia in gram-negative sepsis in mice in part by enhancing the phagocytic activity of blood monocytes. Am J Physiol Lung Cell Mol Physiol. (2012) 302:L1003–13. doi: 10.1152/ajplung.00180.2011
58. Miteva K, Pappritz K, El-Shafeey M, Dong F, Ringe J, Tschope C, et al. Mesenchymal stromal cells modulate monocytes trafficking in coxsackievirus b3-induced myocarditis. Stem Cells Transl Med. (2017) 6:1249–61. doi: 10.1002/sctm.16-0353
59. Eggenhofer E, Steinmann JF, Renner P, Slowik P, Piso P, Geissler EK, et al. Mesenchymal stem cells together with mycophenolate mofetil inhibit antigen presenting cell and T cell infiltration into allogeneic heart grafts. Transpl Immunol. (2011) 24:157–63. doi: 10.1016/j.trim.2010.12.002
60. Gieseke F, Bohringer J, Bussolari R, Dominici M, Handgretinger R, Muller I. Human multipotent mesenchymal stromal cells use galectin-1 to inhibit immune effector cells. Blood. (2010) 116:3770–9. doi: 10.1182/blood-2010-02-270777
61. Duffy MM, Ritter T, Ceredig R, Griffin MD. Mesenchymal stem cell effects on T-cell effector pathways. Stem Cell Res Ther. (2011) 2:34. doi: 10.1186/scrt75
62. Wang Q, Sun B, Wang D, Ji Y, Kong Q, Wang G, et al. Murine bone marrow mesenchymal stem cells cause mature dendritic cells to promote T-cell tolerance. Scand J Immunol. (2008) 68:607–15. doi: 10.1111/j.1365-3083.2008.02180.x
63. Bai L, Lennon DP, Eaton V, Maier K, Caplan AI, Miller SD, et al. Human bone marrow-derived mesenchymal stem cells induce Th2-polarized immune response and promote endogenous repair in animal models of multiple sclerosis. Glia. (2009) 57:1192–203. doi: 10.1002/glia.20841
64. Fiorina P, Jurewicz M, Augello A, Vergani A, Dada S, La Rosa S, et al. Immunomodulatory function of bone marrow-derived mesenchymal stem cells in experimental autoimmune type 1 diabetes. J Immunol. (2009) 183:993–1004. doi: 10.4049/jimmunol.0900803
65. Marson A, Kretschmer K, Frampton GM, Jacobsen ES, Polansky JK, MacIsaac KD, et al. Foxp3 occupancy and regulation of key target genes during T-cell stimulation. Nature. (2007) 445:931–5. doi: 10.1038/nature05478
66. Sakaguchi S, Sakaguchi N, Asano M, Itoh M, Toda M. Immunologic self-tolerance maintained by activated T cells expressing IL-2 receptor alpha-chains (CD25). Breakdown of a single mechanism of self-tolerance causes various autoimmune diseases. J Immunol. (1995) 155:1151–64.
67. Khosravi M, Karimi MH, Hossein Aghdaie M, Kalani M, Naserian S, Bidmeshkipour A. Mesenchymal stem cells can induce regulatory T cells via modulating miR-126a but not miR-10a. Gene. (2017) 627:327–36. doi: 10.1016/j.gene.2017.06.012
68. Engela AU, Hoogduijn MJ, Boer K, Litjens NHR, Betjes MGH, Weimar W, et al. Human adipose-tissue derived mesenchymal stem cells induce functional de-novo regulatory T cells with methylated FOXP3 gene DNA. Clin Exp Immunol. (2013) 173:343–54. doi: 10.1111/cei.12120
69. Meisel R, Zibert A, Laryea M, Gobel U, Daubener W, Dilloo D. Human bone marrow stromal cells inhibit allogeneic T-cell responses by indoleamine 2,3-dioxygenase-mediated tryptophan degradation. Blood. (2004) 103:4619–21. doi: 10.1182/blood-2003-11-3909
70. Cutler AJ, Limbani V, Girdlestone J, Navarrete CV. Umbilical cord-derived mesenchymal stromal cells modulate monocyte function to suppress T cell proliferation. J Immunol. (2010) 185:6617–23. doi: 10.4049/jimmunol.1002239
71. Davies LC, Heldring N, Kadri N, Le Blanc K. Mesenchymal stromal cell secretion of programmed death-1 ligands regulates T cell mediated immunosuppression. Stem Cells. (2017) 35:766–76. doi: 10.1002/stem.2509
72. Beyth S, Borovsky Z, Mevorach D, Liebergall M, Gazit Z, Aslan H, et al. Human mesenchymal stem cells alter antigen-presenting cell maturation and induce T-cell unresponsiveness. Blood. (2005) 105:2214–9. doi: 10.1182/blood-2004-07-2921
73. Franquesa M, Mensah FK, Huizinga R, Strini T, Boon L, Lombardo E, et al. Human adipose tissue-derived mesenchymal stem cells abrogate plasmablast formation and induce regulatory B cells independently of T helper cells. Stem Cells. (2015) 33:880–91. doi: 10.1002/stem.1881
74. Rosser EC, Mauri C. Regulatory B cells: origin, phenotype, and function. Immunity. (2015) 42:607–12. doi: 10.1016/j.immuni.2015.04.005
75. Carter NA, Vasconcellos R, Rosser EC, Tulone C, Munoz-Suano A, Kamanaka M, et al. Mice lacking endogenous IL-10-producing regulatory B cells develop exacerbated disease and present with an increased frequency of Th1/Th17 but a decrease in regulatory T cells. J Immunol. (2011) 186:5569–79. doi: 10.4049/jimmunol.1100284
76. Sato K, Ozaki K, Oh I, Meguro A, Hatanaka K, Nagai T, et al. Nitric oxide plays a critical role in suppression of T-cell proliferation by mesenchymal stem cells. Blood. (2007) 109:228–34. doi: 10.1182/blood-2006-02-002246
77. Chen HH, Lin KC, Wallace CG, Chen YT, Yang CC, Leu S, et al. Additional benefit of combined therapy with melatonin and apoptotic adipose-derived mesenchymal stem cell against sepsis-induced kidney injury. J Pineal Res. (2014) 57:16–32. doi: 10.1111/jpi.12140
78. Sung PH, Chang CL, Tsai TH, Chang LT, Leu S, Chen YL, et al. Apoptotic adipose-derived mesenchymal stem cell therapy protects against lung and kidney injury in sepsis syndrome caused by cecal ligation puncture in rats. Stem Cell Res Ther. (2013) 4:155. doi: 10.1186/scrt385
79. Yip HK, Chang YC, Wallace CG, Chang LT, Tsai TH, Chen YL, et al. Melatonin treatment improves adipose-derived mesenchymal stem cell therapy for acute lung ischemia-reperfusion injury. J Pineal Res. (2013) 54:207–21. doi: 10.1111/jpi.12020
80. Galleu A, Riffo-Vasquez Y, Trento C, Lomas C, Dolcetti L, Cheung TS, et al. Apoptosis in mesenchymal stromal cells induces in vivo recipient-mediated immunomodulation. Sci Transl Med. (2017) 9:eaam7828. doi: 10.1126/scitranslmed.aam7828
81. Sun CK, Yen CH, Lin YC, Tsai TH, Chang LT, Kao YH, et al. Autologous transplantation of adipose-derived mesenchymal stem cells markedly reduced acute ischemia-reperfusion lung injury in a rodent model. J Transl Med. (2011) 9:118. doi: 10.1186/1479-5876-9-118
82. Gonzalez-Rey E, Anderson P, Gonzalez MA, Rico L, Buscher D, Delgado M. Human adult stem cells derived from adipose tissue protect against experimental colitis and sepsis. Gut. (2009) 58:929–39. doi: 10.1136/gut.2008.168534
83. Johnson CL, Soeder Y, Dahlke MH. Concise review: mesenchymal stromal cell-based approaches for the treatment of acute respiratory distress and sepsis syndromes. Stem Cells Transl Med. (2017) 6:1141–51. doi: 10.1002/sctm.16-0415
84. Lu W, Fu C, Song L, Yao Y, Zhang X, Chen Z, et al. Exposure to supernatants of macrophages that phagocytized dead mesenchymal stem cells improves hypoxic cardiomyocytes survival. Int J Cardiol. (2013) 165:333–40. doi: 10.1016/j.ijcard.2012.03.088
85. Thum T, Bauersachs J, Poole-Wilson PA, Volk HD, Anker SD. The dying stem cell hypothesis: immune modulation as a novel mechanism for progenitor cell therapy in cardiac muscle. J Am Coll Cardiol. (2005) 46:1799–802. doi: 10.1016/j.jacc.2005.07.053
86. Hoogduijn MJ, Verstegen MM, Engela AU, Korevaar SS, Roemeling-van Rhijn M, Merino A, et al. No evidence for circulating mesenchymal stem cells in patients with organ injury. Stem Cells Dev. (2014) 23:2328–35. doi: 10.1089/scd.2014.0269
87. Kidd S, Spaeth E, Dembinski JL, Dietrich M, Watson K, Klopp A, et al. Direct evidence of mesenchymal stem cell tropism for tumor and wounding microenvironments using in vivo bioluminescent imaging. Stem Cells. (2009) 27:2614–23. doi: 10.1002/stem.187
88. Ankrum JA, Ong JF, Karp JM. Mesenchymal stem cells: immune evasive, not immune privileged. Nat Biotechnol. (2014) 32:252–60. doi: 10.1038/nbt.2816
89. Le Blanc K, Tammik L, Sundberg B, Haynesworth SE, Ringden O. Mesenchymal stem cells inhibit and stimulate mixed lymphocyte cultures and mitogenic responses independently of the major histocompatibility complex. Scand J Immunol. (2003) 57:11–20. doi: 10.1046/j.1365-3083.2003.01176.x
90. Casiraghi F, Azzollini N, Cassis P, Imberti B, Morigi M, Cugini D, et al. Pretransplant infusion of mesenchymal stem cells prolongs the survival of a semiallogeneic heart transplant through the generation of regulatory T cells. J Immunol. (2008) 181:3933–46. doi: 10.4049/jimmunol.181.6.3933
91. Zhou HP, Yi DH, Yu SQ, Sun GC, Cui Q, Zhu HL, et al. Administration of donor-derived mesenchymal stem cells can prolong the survival of rat cardiac allograft. Transplant Proc. (2006) 38:3046–51. doi: 10.1016/j.transproceed.2006.10.002
92. Hoogduijn MJ, Roemeling-van Rhijn M, Engela AU, Korevaar SS, Mensah FK, Franquesa M, et al. Mesenchymal stem cells induce an inflammatory response after intravenous infusion. Stem Cells Dev. (2013) 22:2825–35. doi: 10.1089/scd.2013.0193
93. Klyushnenkova E, Mosca JD, Zernetkina V, Majumdar MK, Beggs KJ, Simonetti DW, et al. T cell responses to allogeneic human mesenchymal stem cells: immunogenicity, tolerance, and suppression. J Biomed Sci. (2005) 12:47–57. doi: 10.1007/s11373-004-8183-7
94. Eliopoulos N, Stagg J, Lejeune L, Pommey S, Galipeau J. Allogeneic marrow stromal cells are immune rejected by MHC class I- and class II-mismatched recipient mice. Blood. (2005) 106:4057–65. doi: 10.1182/blood-2005-03-1004
95. Zangi L, Margalit R, Reich-Zeliger S, Bachar-Lustig E, Beilhack A, Negrin R, et al. Direct imaging of immune rejection and memory induction by allogeneic mesenchymal stromal cells. Stem Cells. (2009) 27:2865–74. doi: 10.1002/stem.217
96. Djouad F, Plence P, Bony C, Tropel P, Apparailly F, Sany J, et al. Immunosuppressive effect of mesenchymal stem cells favors tumor growth in allogeneic animals. Blood. (2003) 102:3837–44. doi: 10.1182/blood-2003-04-1193
97. Zhu W, Xu W, Jiang R, Qian H, Chen M, Hu J, et al. Mesenchymal stem cells derived from bone marrow favor tumor cell growth in vivo. Exp Mol Pathol. (2006) 80:267–74. doi: 10.1016/j.yexmp.2005.07.004
98. Djouad F, Bony C, Apparailly F, Louis-Plence P, Jorgensen C, Noel D. Earlier onset of syngeneic tumors in the presence of mesenchymal stem cells. Transplantation. (2006) 82:1060–6. doi: 10.1097/01.tp.0000236098.13804.0b
99. Yu PF, Huang Y, Han YY, Lin LY, Sun WH, Rabson AB, et al. TNFα-activated mesenchymal stromal cells promote breast cancer metastasis by recruiting CXCR2(+) neutrophils. Oncogene. (2017) 36:482–90. doi: 10.1038/onc.2016.217
100. Mishra PJ, Mishra PJ, Humeniuk R, Medina DJ, Alexe G, Mesirov JP, et al. Carcinoma-associated fibroblast-like differentiation of human mesenchymal stem cells. Cancer Res. (2008) 68:4331–9. doi: 10.1158/0008-5472.CAN-08-0943
101. Spaeth EL, Dembinski JL, Sasser AK, Watson K, Klopp A, Hall B, et al. Mesenchymal stem cell transition to tumor-associated fibroblasts contributes to fibrovascular network expansion and tumor progression. PLoS ONE. (2009) 4:e4992. doi: 10.1371/journal.pone.0004992
102. Shi Y, Du L, Lin L, Wang Y. Tumour-associated mesenchymal stem/stromal cells: emerging therapeutic targets. Nat Rev Drug Discov. (2017) 16:35–52. doi: 10.1038/nrd.2016.193
103. Quante M, Tu SP, Tomita H, Gonda T, Wang SS, Takashi S, et al. Bone marrow-derived myofibroblasts contribute to the mesenchymal stem cell niche and promote tumor growth. Cancer Cell. (2011) 19:257–72. doi: 10.1016/j.ccr.2011.01.020
104. Jung Y, Kim JK, Shiozawa Y, Wang J, Mishra A, Joseph J, et al. Recruitment of mesenchymal stem cells into prostate tumours promotes metastasis. Nat Commun. (2013) 4:1795. doi: 10.1038/ncomms2766
105. Ren G, Zhao X, Wang Y, Zhang X, Chen X, Xu C, et al. CCR2-dependent recruitment of macrophages by tumor-educated mesenchymal stromal cells promotes tumor development and is mimicked by TNFα. Cell Stem Cell. (2012) 11:812–24. doi: 10.1016/j.stem.2012.08.013
Keywords: mesenchymal stem cell (MSC), immunomodulation, immunogenicity, tumor induction, apoptosis, HI-MSC, monocytes, regulatory T cells
Citation: Weiss ARR and Dahlke MH (2019) Immunomodulation by Mesenchymal Stem Cells (MSCs): Mechanisms of Action of Living, Apoptotic, and Dead MSCs. Front. Immunol. 10:1191. doi: 10.3389/fimmu.2019.01191
Received: 06 March 2019; Accepted: 10 May 2019;
Published: 04 June 2019.
Edited by:
James A. Ankrum, The University of Iowa, United StatesReviewed by:
Bruce Alan Bunnell, Tulane University School of Medicine, United StatesCopyright © 2019 Weiss and Dahlke. This is an open-access article distributed under the terms of the Creative Commons Attribution License (CC BY). The use, distribution or reproduction in other forums is permitted, provided the original author(s) and the copyright owner(s) are credited and that the original publication in this journal is cited, in accordance with accepted academic practice. No use, distribution or reproduction is permitted which does not comply with these terms.
*Correspondence: Andreas Robert Rudolf Weiss, YW5kaS13ZWlzc0BvdXRsb29rLmNvbQ==
Disclaimer: All claims expressed in this article are solely those of the authors and do not necessarily represent those of their affiliated organizations, or those of the publisher, the editors and the reviewers. Any product that may be evaluated in this article or claim that may be made by its manufacturer is not guaranteed or endorsed by the publisher.
Research integrity at Frontiers
Learn more about the work of our research integrity team to safeguard the quality of each article we publish.