- 1Institute for Systems Biology, Seattle, WA, United States
- 2Immunology Division, Garvan Institute of Medical Research, Sydney, NSW, Australia
- 3Faculty of Medicine, St. Vincent's Clinical School, University of New South Wales Sydney, Kensington, NSW, Australia
Lymphatic vessels collect interstitial fluid that has extravasated from blood vessels and return it to the circulatory system. Another important function of the lymphatic network is to facilitate immune cell migration and antigen transport from the periphery to draining lymph nodes. This migration plays a crucial role in immune surveillance, initiation of immune responses and tolerance. Here we discuss the significance and mechanisms of lymphatic migration of innate and adaptive immune cells in homeostasis, inflammation and cancer.
Introduction
The lymphatic system transports fluids from the periphery back into the circulatory system (1) using a series of open-ended capillaries known as lymphatic vessels (2). This network can be exploited by pathogens to facilitate rapid spread throughout the host (3). To prevent pathogen dissemination and enable a fast targeted immune response, the lymphatic system possesses filter-like structures termed lymph nodes (LNs) (3), where innate immune cells, such as macrophages, neutrophils and dendritic cells (DCs) trap and kill pathogens (3) and activate the adaptive immune response (4).
There are two routes by which immune cells can enter LNs: leukocytes can arrive from the bloodstream by crossing high endothelial venules (HEVs) (5). Alternatively, tissue-resident immune cells can enter afferent lymphatic vessels and migrate to draining LNs (dLNs) (5–8). Cells of the innate immune system including DCs, neutrophils, monocytes as well as adaptive immune leukocytes, such as T and B cells use lymphatic vessels to migrate from tissues into LNs (6–11). Lymphocytes exit LNs via efferent lymphatic vessels, and eventually return to the circulatory system by the thoracic duct (12), however, in this review we will focus on the mechanisms and consequences of immune cell migration via the afferent lymphatic system.
In vitro and ex vivo models including adhesion and transmigration assays and analysis of immune cell migration in explanted skin provided important mechanistic insight into leukocyte entry and migration within lymphatic vessels (13–17), while in vivo approaches allowed to examine this complex biological process in situ (Table 1). Historically, in vivo analysis of immune cell migration in afferent lymphatics involved direct transfer of immune cells into the skin, lymphatic cannulation, as well application of fluorescent sensitizers to the skin to label cells and induce inflammation (18). More recently photoconvertible transgenic mice have been utilized to track immune cell migration from the skin and tumors (6, 9, 19–23), while intravital imaging approaches, such as in vivo two-photon microscopy enabled direct visualization of immune cell migration in lymphatic vessels (6, 16, 24, 25).
Lymphatic Migration of Innate Immune Cells
Dendritic Cells
There are two distinct DC populations: plasmacytoid, which produce high amounts of type 1 interferon, and conventional DCs (cDCs) (26). Upon sensing inflammatory stimuli, cDCs enter lymphatic vessels and migrate to LNs (26, 27). They carry antigens (8) and pathogens including viruses (28, 29), spores (30) and bacteria (31–33) from the site of infection to LNs, while DC-mediated transport of innocuous antigens regulates tolerance (34). In dLNs DCs present antigen to CD4+ T cells, or cross-present to CD8+ T cells, thereby regulating adaptive immune responses (26). DC migration from the periphery has been discussed extensively in several recent reviews (27, 35). Here we provide a brief overview of the mechanisms of DC migration via lymphatic vessels (Figure 1).
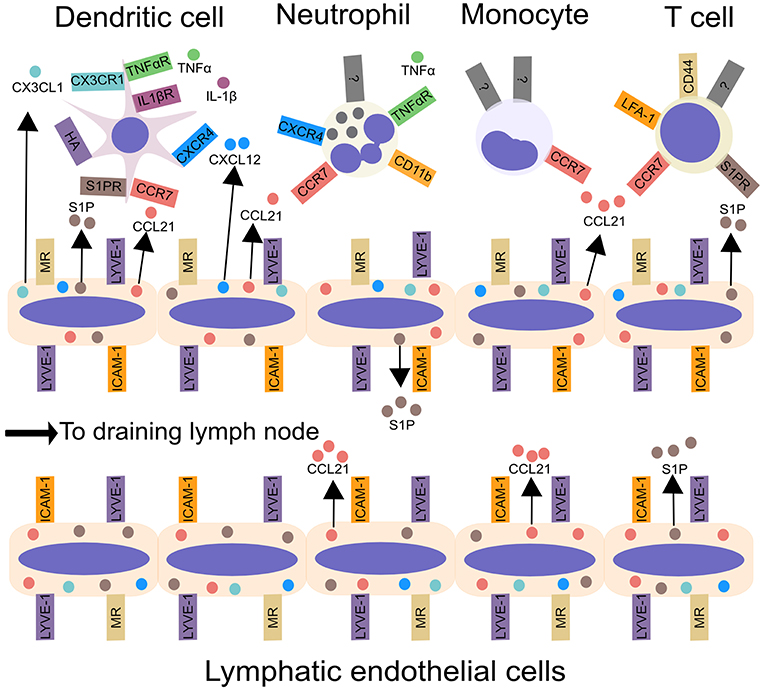
Figure 1. Leukocyte migration from peripheral tissues to draining lymph nodes via afferent lymphatic vessels. Inflammatory cytokines, including IL-1β and TNF-α, produced by tissue-resident myeloid cells, enhance DC and neutrophil migration from tissues to lymphatic vessels. Chemokines, such as CCL21, CX3CL1, and CXCL12, synthesized by LECs in the skin control leukocyte migration to lymphatic vessels and aid transmigration into the vessel lumen. In addition to chemokines, lymphatic endothelial cells produce the lipid S1P, which acts upon S1P receptors, to promote the migration of DCs and T cells into lymphatic vessels and aid trafficking to the draining lymph node. Integrins, such as ICAM-1, CD11b, and LFA-1 may promote leukocyte entry into lymphatic vessels and subsequent migration within lymphatics. Interactions between CD44 and MR promote T cell entry into lymphatic vessels. Lastly, LYVE-1 can bind to hyaluronic acid on DCs, and promote DC entry into lymphatic vessels. ICAM-1, Intercellular Adhesion Molecule 1; LFA-1, lymphocyte function-associated antigen 1; LYVE-1, Lymphatic vessel endothelial hyaluronan receptor 1; MR, macrophage mannose receptor; S1P, sphingosine-1-phosphate.
The most important regulator of DC migration is the chemokine receptor CCR7. Consistent with this, CCR7-deficient DCs show a 90 percent reduction in migration from the periphery in response to inflammatory stimuli (10, 36). An elegant intravital microscopy study demonstrated that CCR7 is required for the LPS-induced directed migration of DCs toward lymphatic vessels and subsequent transmigration (25). DCs also use CCR7 for trafficking to dLNs from the lamina propria (37), lung (34), and skin (23) under homeostatic conditions. Interestingly, CCR7-dependent DC migration decreases lymphatic permeability (38), indicating bi-directional communication between the lymphatic network and immune cells.
Lymphatic vessels express CCR7 ligand, CCL21 (25, 39–41), which is required for DC trafficking from skin to LNs under homeostatic (41) and inflammatory (42) conditions. Imaging studies have provided important insight into the role of CCL21 in DC migration. Firstly, the size of the CCL21 gradient, and distribution of lymphatic vessels, indicates that most skin DCs are able to sense CCL21 gradients (41). Secondly, DCs enter lymphatic vessels at sites of high CCL21 expression (25), suggesting that CCL21 directly regulates entry into lymphatics. Finally, intravital microscopy has revealed that CCL21 also enhances DC migration within lymphatic vessels (40). Collectively, these observations suggest that CCL21 regulates multiple steps in the lymphatic migration of DCs. In contrast, the other CCR7 ligand, CCL19, appears to be dispensable for DC lymphatic trafficking (42).
Inflammatory mediators regulate DC lymphatic migration (26). The cytokines Interleukin-1β (IL-1β) and Tumor Necrosis Factor-α (TNF-α) promote inflammation-induced DC migration to LNs (43–45). Furthermore, the lipid prostaglandin E2 increased CCR7 expression on DCs, augmenting migration towards CCL19 and CCL21 in vitro (46). Additional regulators of CCR7-mediated migration include the cell surface molecules CD37, CD38, and CD47 which enhance DC movement toward CCR7 ligands and migration to dLNs (15, 47–50). In contrast, immunosuppressive molecules including IL-10 (51), TGF-β (52, 53) and the anti-inflammatory lipid Resolvin E1 (54) can inhibit DC trafficking.
In addition to CCR7, a number of other chemokine receptor/ligand pairs have been implicated in lymphatic DC migration. CXCR4/CXCL12 and CX3CR1/CX3CL1 enhance DC trafficking from inflamed skin (55, 56). The role of CCR8 is less clear with CCR8-deficient mice displaying reduced lymphatic migration of DCs following an injection of latex beads (57), but enhanced migration of DCs following FITC painting (58), suggesting that CCR8 plays a limited, or stimulus-specific, role in this process.
Intravital imaging and FITC-painting experiments have demonstrated that integrins, and integrin signaling are required for inflammation-induced DC migration to dLNs (14, 59, 60). However, DCs from mice lacking all integrins were able to migrate to LNs when injected into resting skin (61), indicating that integrins are important for DC migration in response to inflammation but dispensable for steady state DC egress. Accordingly, inflammation increases the expression of integrin ligands on lymphatic endothelial cells (LECs) (14). DC-expressed L1 cell adhesion molecule guides transendothelial migration of DCs thereby promoting trafficking to dLNs (17). A recent study demonstrated that interactions between LEC-expressed LYVE-1 and hyaluronan on the DC plasma membrane mediated DC adhesion and transmigration across LECs and subsequent migration to dLNs (13).
Sphingosine-1-phosphate (S1P), a lipid mediator of leukocyte egress from lymphoid organs (62), has been implicated in DC trafficking from the skin and lung (33, 63–65). However, in mice that lack S1P in lymphatic fluid, but not blood, the migration of adoptively transferred DCs to dLNs was comparable to that seen in wild-type mice (66). These results, and the fact that there are five S1P receptors (63), suggest that further experiments are required to uncover the precise role of S1P signaling in DC migration.
In contrast to cDCs, the lymphatic migration of pDCs is poorly understood. While one study reported that adoptively transferred pDCs migrated to dLNs from ovine skin (67), another showed that pDCs were not detected in the lymph of rats (68). However, pDCs transported harmless inhaled antigen from murine lungs to the mediastinal LN where they suppressed T cell activation, suggesting that pDC migration may play a role in preventing inflammation (69).
Neutrophils
Neutrophils are the first immune cells recruited to sites of inflammation, where they kill pathogens and release mediators that recruit other leukocytes (70, 71). Until recently neutrophils were thought to die at inflammatory foci. However, several groups, including ours, have shown that neutrophils can enter tissue lymphatic vessels and migrate to dLNs from the site of inflammation (6, 72–74).
Intravital imaging of inflamed mouse skin has enabled direct visualization of neutrophil migration within lymphatic vasculature (Figure 2A) (6, 72). However, in comparison to DCs, the significance and extent of neutrophil lymphatic migration are incompletely understood. Cannulation experiments have demonstrated that inflammation leads to a dramatic increase in neutrophils in ovine afferent lymph (8, 75, 76). Furthermore, neutrophils can transport antigens and microorganisms (Figure 2B) from the site of infection to LNs (6, 8, 77). Accordingly, inhibiting neutrophil lymphatic migration reduced early lymphocyte proliferation (6). Notably, a recent study did not detect substantial lymphatic migration of neutrophils in response to Staphylococcus aureus (S. aureus) (78). This likely highlights the fact that most neutrophils arrive in dLNs from the circulation via HEVs in response to bacteria already in dLNs, while a smaller population of neutrophils migrates directly from the site of inflammation to dLNs via afferent lymphatics. However, since neutrophils are the first innate immune cell subset to arrive in the LN from inflamed tissues, and often carry microbes, neutrophil lymphatic migration can exert considerable influence on the subsequent adaptive immune response (6, 77, 79).
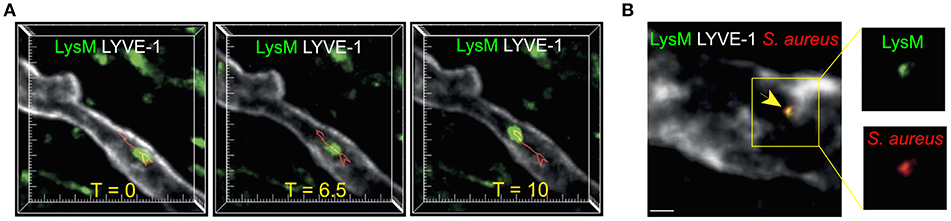
Figure 2. Neutrophil migration in skin lymphatic vessels. (A) Two-photon microscopy was used to examine the lymphatic migration of neutrophils in response to S. aureus. Images are maximum intensity projections of three-dimensional volumes acquired via two-photon microscopy. Lysozyme M+ GFP neutrophil (green) migrating inside a lymphatic vessel (LYVE-1, white) is show at three representative time points. Red track indicates neutrophil's path. Tick marks are 10 μm apart. (B) Two-photon image of a Lysozyme M reporter mouse skin with a Lysozyme M+ (green) neutrophil containing S. aureus (red) inside the LYVE-1+ lymphatic vessel (white). Scale bar is 10 μm. Figure was adapted from Hampton et al. (6).
Lymphatic migration of neutrophils could potentially be exploited by pathogens to enhance dissemination, since some microorganisms including the bacterium S. aureus can survive inside neutrophils (80). Consistent with this, injection of Leishmania major-containing neutrophils was sufficient to establish infection in mice, while depleting neutrophils reduced Leishmania burden when the pathogen was injected into the skin (81). Neutrophils also transported live Mycobacterium bovis bacille Calmette-Guérin from the skin to dLNs (77). In Toxoplasma gondii infection neutrophils removed the macrophages that line the subscapular sinus of the LN (82), however, it is not clear whether this favors pathogen control or spread.
CCR7 appears to be less important for neutrophil lymphatic migration than for that of DCs. Although it was required for neutrophil entry into lymphatic vessels in response to TNF-α and Complete Freund's Adjuvant (CFA) (72), and for CFA-driven migration from skin to LNs (83), neutrophil migration from the skin to dLNs in response to S. aureus was CCR7-independent (6). This suggests that the requirements for neutrophil trafficking vary depending on the stimulus and additional molecules may play key roles in guiding this migration.
The chemokine receptor CXCR4 regulates neutrophil migration from the bone marrow into the circulation (84) and may also play a role in their lymphatic migration. Inhibiting CXCR4 decreased neutrophil trafficking in response to immune complexes and S. aureus (6, 73). However, CXCR4 was not required for neutrophil entry into lymphatic vessels in response to CFA, as revealed by confocal imaging (72), again highlighting differences in neutrophil trafficking in response to distinct stimuli.
The cell surface receptor CD11b, which is involved in neutrophil recruitment from the vasculature into tissues (85), is emerging as a major regulator of neutrophil migration via lymphatics since neutrophil migration from inflammatory foci to LNs is substantially reduced when CD11b is inhibited (6, 73, 74). Intravital imaging demonstrated that blocking CD11b or its ligand ICAM-1 impaired neutrophil intraluminal crawling within lymphatic vasculature following CFA injection by reducing neutrophil speed and directionality (72). Likewise, inhibiting CD11b and ICAM-1 reduced neutrophil entry into lymphatic vessels and diminished egress from the skin in response to Mycobacterium bovis (74). Lymphocyte Function-associated Antigen (LFA-1), which binds to ICAMs and is involved in neutrophil entry into tissues from the circulation, was required for neutrophil migration via afferent lymphatics in response to immune complexes (73) but not S. aureus (6).
Inflammatory cytokines may enhance neutrophil entry into lymphatics. TNF-α promoted neutrophil entry and crawling within lymphatic vessels in mouse cremaster muscle (72). However, since inflammatory cytokines also control neutrophil recruitment to sites of inflammation and lifespan, identifying a distinct role for these molecules in neutrophil lymphatic migration requires further investigation.
Monocytes and Macrophages
Monocytes are circulating leukocytes that phagocytose and kill bacteria and fungi and regulate the activity of other immune cells via cytokine release (86–88). They can also differentiate into DC and macrophage subsets (89). Several studies have demonstrated that monocytes egress tissues via afferent lymphatic vessels and transport antigen to dLNs (7, 8, 90–92). Once there, monocytes may present and cross-present antigens since a subcutaneous injection of antigen-pulsed monocytes induced the proliferation of antigen-specific CD4+ and CD8+ T cells (91). The molecular mechanisms controlling monocyte migration via lymphatic vessels are yet to be identified. However, CCR7 may be important, since CCR7-deficient LPS-primed monocytes failed to migrate from the footpad to the popliteal LN (91). Accumulating evidence suggests that macrophages can also migrate from inflammatory lesions to dLNs (93–95) and that α1β1 integrin may limit macrophage egress via afferent lymphatics (93).
Lymphatic Migration of Adaptive Immune Cells
T Cells
T cells possess a rearranged T cell receptor which includes either αβ or γδ polypeptides (96). While αβ T cells are more abundant, γδ T cells are enriched in epithelial and mucosal tissues where they act as the first line of defense against pathogens. One of the main functions of CD8+ T cells is to kill infected cells (97), while CD4+ helper T cells secrete cytokines and regulate the function of other immune subsets (98). Photoconversion experiments (22), along with lymphatic cannulation (99), have demonstrated that effector, rather than naïve, T cells comprise the majority of lymph migrating T cells under homeostatic (22, 99) and inflammatory conditions (22, 100). This migration plays an important role in immune surveillance and in resolution of inflammation (18, 101–103).
Like DCs, T cells use CCR7 to migrate to LNs under homeostatic and inflammatory conditions. Following antigen challenge, T cells overexpressing CCR7 were preferentially lost from the lung and accumulated in the mediastinal LNs (104), while CCR7-deficient T cells failed to migrate from the footpad to the popliteal LN (11). However, the need for CCR7 in T cell migration might be context dependent, as T cells used CCR7 for trafficking from acute, but not chronically, inflamed skin (100). Tumor-infiltrating T cells could also emigrate to dLNs independently of CCR7 (9).
The lipid S1P, which promotes αβ T cell exit from LNs (62), can also mediate their migration via afferent lymphatics. Consequently, antagonizing S1P receptors led to T cell accumulation near skin lymphatic vessels and reduced migration to dLNs (100, 105). LEC-expressed macrophage mannose receptor (MR) and the cell surface molecule CD44, which interacts with MR, promoted T cell lymphatic migration by increasing T cell adhesion to lymphatic vessels (106, 107). Another LEC-expressed protein, CLEVER-1, was also demonstrated to be important for T cell migration via afferent lymphatics (108). Additionally, intravital imaging has shown that LFA-1 and its ligand ICAM-1, increased T cell velocity within afferent lymphatic vessels thereby promoting T cell migration to dLNs (16).
T cell egress from tissues to dLNs can either promote inflammatory responses or suppress them. For example, lymphatic migration of Regulatory T (Treg) cells may suppress allograft rejection (103). Likewise, CCR7-deficient Treg cells failed to migrate to the draining LN and accumulated in the skin, reducing skin inflammation during a delayed-type hypersensitivity reaction (101). Conversely, overexpression of CCR7 on antigen-specific Th1-cells enhanced their egress to dLNs and led to faster resolution of the inflammatory response in the skin (102).
Like αβ T cells, γδ T cells can migrate from the skin (23, 95, 109) and tumors (9) via lymphatic vessels to dLNs and comprise a large proportion of the cells in bovine lymph (110). Photoconversion experiments have demonstrated that murine γδ T cells can migrate from the skin to dLNs in the absence of CCR7 (23). Similarly, bovine γδ T cells can egress tissues independently of this receptor (111). The consequences of γδ T cell lymphatic migration are poorly understood, though it may enhance CD8+ T cell proliferation (23).
B Cells
Cannulation experiments in sheep (112) and photoconversion experiments in mice (95), suggest that B cells use afferent lymphatic vessels to migrate from tissues to dLNs. The mechanisms of this migration are not yet known, however, at least in chronic inflammation, B cell egress may be independent of S1P and requires CCR7 (100). Similarly to T cells, blocking CLEVER-1 reduced B cell migration to dLNs (108).
Lymphatic Migration of Immune Cells in Disease
The importance of immune cell migration via lymphatics in host defense is illustrated by the observations that mice lacking CCR7 are susceptible to microbial and viral infections (113–115). On the other hand, lymphatic migration of immune cells may also augment autoimmunity since preventing immune cell trafficking from the meninges to the cervical LNs reduced the severity of EAE (116). Furthermore, higher densities of lymphatic vessels in transplanted corneas (117) and kidneys (118) were associated with rejection, while preventing DC migration to the dLN by blocking Vascular Endothelial Growth Factor C (VEGF-C) improved corneal transplantation outcomes (119). Interestingly, in mice, obesity was associated with decreased lymphatic function and reduced immune cell migration to dLNs (120), suggesting that obesity may be linked to decreased immunity. CCR7 as well as its two ligands, CCL19 and CCL21, have been identified in mouse and human atherosclerotic lesions (121), consistent with accumulating evidence of a role for immune cell lymphatic migration in heart disease (122–125).
Lymphatic vasculature also plays a crucial role in tumor immunity by enabling transport of antigens from tumors to dLNs and egress of immune cells (9, 126). Lymphatic vessels can also serve as conduits for tumor cell spread (1). Their dual role in cancer is highlighted by the findings that many tumors overexpress VEGF-C, which promotes the growth and survival of LECs (127, 128) leading to increased LN metastasis (128–130). Furthermore, a recent study demonstrated that IFNγ-induced PD-L1 expression by LECs may dampen anti-tumor immunity by limiting cytotoxic CD8+ T cell accumulation in melanoma (131). On the other hand, overexpression of VEGF-C in a mouse melanoma model increased DC migration to dLNs (132). Consistent with an increase in lymphatic migration in cancer, enhanced trafficking of adoptively transferred B and T lymphocytes from footpads to dLNs was observed in melanoma-bearing mice (133).
Similarly to DC migration from the periphery, the DC-dependent transfer of antigen from B16F10 melanoma to the dLN required CCR7. This correlated with an increase in CD8+ T cell priming and a reduction in tumor growth (126). Overexpression of TGF-β1 in a model of squamous cell carcinoma reduced DC trafficking to dLNs, which led to an increase in LN metastasis (53). However, since CCR7 and the TGF-β1 receptor are not restricted to DCs (134), other immune cells may also contribute to these effects on tumor growth and metastasis. In contrast to DCs, photoconversion experiments demonstrated that tumor-infiltrating T cells do not require CCR7 to migrate to dLNs via afferent lymphatics (9).
Concluding Remarks
Lymphatic migration of immune cells presents opportunities for control of immune responses in infection and homeostasis. However, with the exception of DCs and T cells, the mechanisms controlling lymphatic migration of immune cells remain poorly understood. New tools, such as photoconversion and intravital imaging are poised to provide novel insight into the migration of previously overlooked immune subsets. A better understanding of the distinct mechanisms guiding lymphatic migration of specific immune subsets may suggest new approaches for treatment of cancer, autoimmunity and excessive inflammation.
Author Contributions
All authors listed have made a substantial, direct and intellectual contribution to the work, and approved it for publication.
Funding
This work was funded by the National Health and Medical Research Council (NHMRC) project grant GNT1106043 (TC) and a Postdoctoral Fellowship from the Global Lyme Alliance (HH).
Conflict of Interest Statement
The authors declare that the research was conducted in the absence of any commercial or financial relationships that could be construed as a potential conflict of interest.
References
1. Alitalo K. The lymphatic vasculature in disease. Nat Med. (2011) 17:1371–80. doi: 10.1038/nm.2545
2. Cueni LN, Detmar M. New insights into the molecular control of the lymphatic vascular system and its role in disease. J Invest Dermatol. (2006) 126:2167–77. doi: 10.1038/sj.jid.5700464
3. Kastenmüller W, Torabi-Parizi P, Subramanian N, Lämmermann T, Germain RN. A spatially-organized multicellular innate immune response in lymph nodes limits systemic pathogen spread. Cell. (2012) 150:1235–48. doi: 10.1016/j.cell.2012.07.021
4. Von Andrian UH, Mempel TR. Homing and cellular traffic in lymph nodes. Nat Rev Immunol. (2003) 3:867–78. doi: 10.1038/nri1222
5. Miyasaka M, Tanaka T. Lymphocyte trafficking across high endothelial venules: dogmas and enigmas. Nat Rev Immunol. (2004) 4:360–70. doi: 10.1038/nri1354
6. Hampton HR, Bailey J, Tomura M, Brink R, Chtanova T. Microbe-dependent lymphatic migration of neutrophils modulates lymphocyte proliferation in lymph nodes. Nat Commun. (2015) 6:7139. doi: 10.1038/ncomms8139
7. Bonneau M, Epardaud M, Payot F, Niborski V, Thoulouze M-I, Bernex F, et al. Migratory monocytes and granulocytes are major lymphatic carriers of Salmonella from tissue to draining lymph node. J Leukoc Biol. (2006) 79:268–76. doi: 10.1189/jlb.0605288
8. Neeland MR, Elhay MJ, Nathanielsz J, Meeusen ENT, de Veer MJ. Incorporation of CpG into a liposomal vaccine formulation increases the maturation of antigen-loaded dendritic cells and monocytes to improve local and systemic immunity. J Immunol. (2014) 192:3666–75. doi: 10.4049/jimmunol.1303014
9. Torcellan T, Hampton HR, Bailey J, Tomura M, Brink R, Chtanova T. In vivo photolabeling of tumor-infiltrating cells reveals highly regulated egress of T-cell subsets from tumors. Proc Natl Acad Sci USA. (2017) 114:5677–82. doi: 10.1073/pnas.1618446114
10. Förster R, Schubel A, Breitfeld D, Kremmer E, Renner-Müller I, Wolf E, et al. CCR7 coordinates the primary immune response by establishing functional microenvironments in secondary lymphoid organs. Cell. (1999) 99:23–33. doi: 10.1016/S0092-8674(00)80059-8
11. Debes GF, Arnold CN, Young AJ, Krautwald S, Lipp M, Hay JB, et al. Chemokine receptor CCR7 required for T lymphocyte exit from peripheral tissues. Nat Immunol. (2005) 6:889–94. doi: 10.1038/ni1238
12. Haig DM, Hopkins J, Miller HR. Local immune responses in afferent and efferent lymph. Immunology. (1999) 96:155–63. doi: 10.1046/j.1365-2567.1999.00681.x
13. Johnson LA, Banerji S, Lawrance W, Gileadi U, Prota G, Holder KA, et al. Dendritic cells enter lymph vessels by hyaluronan-mediated docking to the endothelial receptor LYVE-1. Nat Immunol. (2017) 18:762–70. doi: 10.1038/ni.3750
14. Johnson LA, Clasper S, Holt AP, Lalor PF, Baban D, Jackson DG. An inflammation-induced mechanism for leukocyte transmigration across lymphatic vessel endothelium. J Exp Med. (2006) 203:2763–77. doi: 10.1084/jem.20051759
15. Van VQ, Lesage S, Bouguermouh S, Gautier P, Rubio M, Levesque M, et al. Expression of the self-marker CD47 on dendritic cells governs their trafficking to secondary lymphoid organs. EMBO J. (2006) 25:5560–8. doi: 10.1038/sj.emboj.7601415
16. Teijeira A, Hunter MC, Russo E, Proulx ST, Frei T, Debes GF, et al. T cell migration from inflamed skin to draining lymph nodes requires intralymphatic crawling supported by ICAM-1/LFA-1 interactions. Cell Rep. (2017) 18:857–65. doi: 10.1016/j.celrep.2016.12.078
17. Maddaluno L, Verbrugge SE, Martinoli C, Matteoli G, Chiavelli A, Zeng Y, et al. The adhesion molecule L1 regulates transendothelial migration and trafficking of dendritic cells. J Exp Med. (2009) 206:623–35. doi: 10.1084/jem.20081211
18. Hunter MC, Teijeira A, Halin C. T cell trafficking through lymphatic vessels. Front Immunol. (2016) 7:613. doi: 10.3389/fimmu.2016.00613
19. Bromley SK, Yan S, Tomura M, Kanagawa O, Luster AD. Recirculating memory T cells are a unique subset of CD4+ T cells with a distinct phenotype and migratory pattern. J Immunol. (2013) 190:970–6. doi: 10.4049/jimmunol.1202805
20. Tomura M, Yoshida N, Tanaka J, Karasawa S, Miwa Y, Miyawaki A, et al. Monitoring cellular movement in vivo with photoconvertible fluorescence protein “Kaede” transgenic mice. Proc Natl Acad Sci USA. (2008) 105:10871–6. doi: 10.1073/pnas.0802278105
21. Tomura M, Hata A, Matsuoka S, Shand FHW, Nakanishi Y, Ikebuchi R, et al. Tracking and quantification of dendritic cell migration and antigen trafficking between the skin and lymph nodes. Sci Rep. (2015) 4:6030. doi: 10.1038/srep06030
22. Tomura M, Honda T, Tanizaki H, Otsuka A, Egawa G, Tokura Y, et al. Activated regulatory T cells are the major T cell type emigrating from the skin during a cutaneous immune response in mice. J Clin Invest. (2010) 120:883–93. doi: 10.1172/JCI40926
23. Nakamizo S, Egawa G, Tomura M, Sakai S, Tsuchiya S, Kitoh A, et al. Dermal Vγ4 + γδ T cells possess a migratory potency to the draining lymph nodes and modulate CD8 + T-cell activity through TNF-α production. J Invest Dermatol. (2015) 135:1007–15. doi: 10.1038/jid.2014.516
24. Roediger B, Ng LG, Smith AL St. , Groth BF, Weninger W Visualizing dendritic cell migration within the skin. Histochem Cell Biol. (2008) 130:1131–46. doi: 10.1007/s00418-008-0531-7
25. Tal O, Lim HY, Gurevich I, Milo I, Shipony Z, Ng LG, et al. DC mobilization from the skin requires docking to immobilized CCL21 on lymphatic endothelium and intralymphatic crawling. J Exp Med. (2011) 208:2141–53. doi: 10.1084/jem.20102392
26. Alvarez D, Vollmann EH, von Andrian UH. Mechanisms and consequences of dendritic cell migration. Immunity. (2008) 29:325–42. doi: 10.1016/j.immuni.2008.08.006
27. Worbs T, Hammerschmidt SI, Förster R. Dendritic cell migration in health and disease. Nat Rev Immunol. (2017) 17:30–48. doi: 10.1038/nri.2016.116
28. Ho AWS, Prabhu N, Betts RJ, Ge MQ, Dai X, Hutchinson PE, et al. Lung CD103+ dendritic cells efficiently transport influenza virus to the lymph node and load viral antigen onto MHC class I for presentation to CD8 T cells. J Immunol. (2011) 187:6011–21. doi: 10.4049/jimmunol.1100987
29. Lukens MV, Kruijsen D, Coenjaerts FEJ, Kimpen JLL, Bleek GM van. Respiratory syncytial virus-induced activation and migration of respiratory dendritic cells and subsequent antigen presentation in the lung-draining lymph node. J Virol. (2009) 83:7235–43. doi: 10.1128/JVI.00452-09
30. Cleret A, Quesnel-Hellmann A, Vallon-Eberhard A, Verrier B, Jung S, Vidal D, et al. Lung dendritic cells rapidly mediate anthrax spore entry through the pulmonary route. J Immunol. (2007) 178:7994–8001. doi: 10.4049/jimmunol.178.12.7994
31. Bravo-Blas A, Utriainen L, Clay SL, Kästele V, Cerovic V, Cunningham AF, et al. Salmonella enterica serovar typhimurium travels to mesenteric lymph nodes both with host cells and autonomously. J Immunol. (2018) 202:260–7. doi: 10.4049/jimmunol.1701254
32. Voedisch S, Koenecke C, David S, Herbrand H, Förster R, Rhen M, et al. Mesenteric lymph nodes confine dendritic cell-mediated dissemination of Salmonella enterica serovar typhimurium and limit systemic disease in mice. Infect Immun. (2009) 77:3170–80. doi: 10.1128/IAI.00272-09
33. St. John AL, Ang WXG, Huang M-N, Kunder CA, Chan EW, Gunn MD, et al. S1P-Dependent Trafficking of Intracellular Yersinia pestis through Lymph Nodes Establishes Buboes and Systemic Infection. Immunity. (2014) 41:440–50. doi: 10.1016/j.immuni.2014.07.013
34. Hintzen G, Ohl L, del Rio M-L, Rodriguez-Barbosa J-I, Pabst O, Kocks JR, et al. Induction of tolerance to innocuous inhaled antigen relies on a CCR7-dependent dendritic cell-mediated antigen transport to the bronchial lymph node. J Immunol. (2006) 177:7346–54. doi: 10.4049/jimmunol.177.10.7346
35. Permanyer M, Bošnjak B, Förster R. Dendritic cells, T cells and lymphatics: dialogues in migration and beyond. Curr Opin Immunol. (2018) 53:173–9. doi: 10.1016/j.coi.2018.05.004
36. Clatworthy MR, Aronin CEP, Mathews RJ, Morgan NY, Smith KGC, Germain RN. Immune complexes stimulate CCR7-dependent dendritic cell migration to lymph nodes. Nat Med. (2014) 20:1458–63. doi: 10.1038/nm.3709
37. Jang MH, Sougawa N, Tanaka T, Hirata T, Hiroi T, Tohya K, et al. CCR7 is critically important for migration of dendritic cells in intestinal lamina propria to mesenteric lymph nodes. J Immunol. (2006) 176:803–10. doi: 10.4049/jimmunol.176.2.803
38. Ivanov S, Scallan JP, Kim K-W, Werth K, Johnson MW, Saunders BT, et al. CCR7 and IRF4-dependent dendritic cells regulate lymphatic collecting vessel permeability. J Clin Invest. (2016) 126:1581–91. doi: 10.1172/JCI84518
39. Russo E, Nitschké M, Halin C. Dendritic cell interactions with lymphatic endothelium. Lymphat Res Biol. (2013) 11:172–82. doi: 10.1089/lrb.2013.0008
40. Russo E, Teijeira A, Vaahtomeri K, Willrodt A-H, Bloch JS, Nitschké M, et al. Intralymphatic CCL21 promotes tissue egress of dendritic cells through afferent lymphatic vessels. Cell Rep. (2016) 14:1723–34. doi: 10.1016/j.celrep.2016.01.048
41. Weber M, Hauschild R, Schwarz J, Moussion C, de Vries I, Legler DF, et al. Interstitial dendritic cell guidance by haptotactic chemokine gradients. Science. (2013) 339:328–32. doi: 10.1126/science.1228456
42. Britschgi MR, Favre S, Luther SA. CCL21 is sufficient to mediate DC migration, maturation and function in the absence of CCL19. Eur J Immunol. (2010) 40:1266–71. doi: 10.1002/eji.200939921
43. Antonopoulos C, Cumberbatch M, Dearman RJ, Daniel RJ, Kimber I, Groves RW. Functional caspase-1 is required for Langerhans cell migration and optimal contact sensitization in mice. J Immunol. (2001) 166:3672–7. doi: 10.4049/jimmunol.166.6.3672
44. Pang IK, Ichinohe T, Iwasaki A. IL-1R signaling in dendritic cells replaces pattern-recognition receptors in promoting CD8+ T cell responses to influenza A virus. Nat Immunol. (2013) 14:246–53. doi: 10.1038/ni.2514
45. Wang B, Fujisawa H, Zhuang L, Kondo S, Shivji GM, Kim CS, et al. Depressed Langerhans cell migration and reduced contact hypersensitivity response in mice lacking TNF receptor p75. J Immunol. (1997) 159:6148–55.
46. Scandella E, Men Y, Gillessen S, Förster R, Groettrup M. Prostaglandin E2 is a key factor for CCR7 surface expression and migration of monocyte-derived dendritic cells. Blood. (2002) 100:1354–61. doi: 10.1182/blood-2001-11-0017
47. Partida-Sánchez S, Goodrich S, Kusser K, Oppenheimer N, Randall TD, Lund FE. Regulation of dendritic cell trafficking by the ADP-ribosyl cyclase CD38: impact on the development of humoral immunity. Immunity. (2004) 20:279–91. doi: 10.1016/S1074-7613(04)00048-2
48. Jones EL, Wee JL, Demaria MC, Blakeley J, Ho PK, Vega-Ramos J, et al. Dendritic cell migration and antigen presentation are coordinated by the opposing functions of the tetraspanins CD82 and CD37. J Immunol. (2016) 196:978–87. doi: 10.4049/jimmunol.1500357
49. Gartlan KH, Wee JL, Demaria MC, Nastovska R, Chang TM, Jones EL, et al. Tetraspanin CD37 contributes to the initiation of cellular immunity by promoting dendritic cell migration. Eur J Immunol. (2013) 43:1208–19. doi: 10.1002/eji.201242730
50. Hagnerud S, Manna PP, Cella M, Stenberg A, Frazier WA, Colonna M, et al. Deficit of CD47 results in a defect of marginal zone dendritic cells, blunted immune response to particulate antigen and impairment of skin dendritic cell migration. J Immunol. (2006) 176:5772–8. doi: 10.4049/jimmunol.176.10.5772
51. Cumberbatch M, Clelland K, Dearman RJ, Kimber I. Impact of cutaneous IL-10 on resident epidermal Langerhans' cells and the development of polarized immune responses. J Immunol. (2005) 175:43–50. doi: 10.4049/jimmunol.175.1.43
52. Kel JM, Girard-Madoux MJH, Reizis B, Clausen BE. TGF-β is required to maintain the pool of immature Langerhans cells in the epidermis. J Immunol. (2010) 185:3248–55. doi: 10.4049/jimmunol.1000981
53. Imai K, Minamiya Y, Koyota S, Ito M, Saito H, Sato Y, et al. Inhibition of dendritic cell migration by transforming growth factor-β1 increases tumor-draining lymph node metastasis. J Exp Clin Cancer Res. (2012) 31:3. doi: 10.1186/1756-9966-31-3
54. Sawada Y, Honda T, Hanakawa S, Nakamizo S, Murata T, Ueharaguchi-Tanada Y, et al. Resolvin E1 inhibits dendritic cell migration in the skin and attenuates contact hypersensitivity responses. J Exp Med. (2015) 212:1921–30. doi: 10.1084/jem.20150381
55. Kabashima K, Shiraishi N, Sugita K, Mori T, Onoue A, Kobayashi M, et al. CXCL12-CXCR4 engagement is required for migration of cutaneous dendritic cells. Am J Pathol. (2007) 171:1249–57. doi: 10.2353/ajpath.2007.070225
56. Johnson LA, Jackson DG. The chemokine CX3CL1 promotes trafficking of dendritic cells through inflamed lymphatics. J Cell Sci. (2013) 126:5259–70. doi: 10.1242/jcs.135343
57. Qu C, Edwards EW, Tacke F, Angeli V, Llodrá J, Sanchez-Schmitz G, et al. Role of CCR8 and other chemokine pathways in the migration of monocyte-derived dendritic cells to lymph nodes. J Exp Med. (2004) 200:1231–41. doi: 10.1084/jem.20032152
58. Yabe R, Shimizu K, Shimizu S, Azechi S, Choi B-I, Sudo K, et al. CCR8 regulates contact hypersensitivity by restricting cutaneous dendritic cell migration to the draining lymph nodes. Int Immunol. (2015) 27:169–81. doi: 10.1093/intimm/dxu098
59. Nitschke M, Aebischer D, Abadier M, Haener S, Lucic M, Vigl B, et al. Differential requirement for ROCK in dendritic cell migration within lymphatic capillaries in steady-state and inflammation. Blood. (2012) 120:2249–58. doi: 10.1182/blood-2012-03-417923
60. Xu H, Guan H, Zu G, Bullard D, Hanson J, Slater M, et al. The role of ICAM-1 molecule in the migration of Langerhans cells in the skin and regional lymph node. Eur J Immunol. (2001) 31:3085–93. doi: 10.1002/1521-4141(2001010)31:10<3085::AID-IMMU3085>3.0.CO;2-B
61. Lämmermann T, Bader BL, Monkley SJ, Worbs T, Wedlich-Söldner R, Hirsch K, et al. Rapid leukocyte migration by integrin-independent flowing and squeezing. Nature. (2008) 453:51–5. doi: 10.1038/nature06887
62. Cyster JG, Schwab SR. Sphingosine-1-phosphate and lymphocyte egress from lymphoid organs. Annu Rev Immunol. (2012) 30:69–94. doi: 10.1146/annurev-immunol-020711-075011
63. Czeloth N, Bernhardt G, Hofmann F, Genth H, Förster R. Sphingosine-1-phosphate mediates migration of mature dendritic cells. J Immunol. (2005) 175:2960–7. doi: 10.4049/jimmunol.175.5.2960
64. Idzko M, Hammad H, van Nimwegen M, Kool M, Müller T, Soullié T, et al. Local application of FTY720 to the lung abrogates experimental asthma by altering dendritic cell function. J Clin Invest. (2006) 116:2935–44. doi: 10.1172/JCI28295
65. Rathinasamy A, Czeloth N, Pabst O, Forster R, Bernhardt G. The origin and maturity of dendritic cells determine the pattern of sphingosine 1-phosphate receptors expressed and required for efficient migration. J Immunol. (2010) 185:4072–81. doi: 10.4049/jimmunol.1000568
66. Pham THM, Baluk P, Xu Y, Grigorova I, Bankovich AJ, Pappu R, et al. Lymphatic endothelial cell sphingosine kinase activity is required for lymphocyte egress and lymphatic patterning. J Exp Med. (2010) 207:17–27. doi: 10.1084/jem.20091619
67. Pascale F, Pascale F, Contreras V, Bonneau M, Courbet A, Chilmonczyk S, et al. Plasmacytoid dendritic cells migrate in afferent skin lymph. J Immunol. (2008) 180:5963–72. doi: 10.4049/jimmunol.180.9.5963
68. Yrlid U, Cerovic V, Milling S, Jenkins CD, Zhang J, Crocker PR, et al. Plasmacytoid dendritic cells do not migrate in intestinal or hepatic lymph. J Immunol. (2006) 177:6115–21. doi: 10.4049/jimmunol.177.9.6115
69. de Heer HJ, Hammad H, Soullié T, Hijdra D, Vos N, Willart MAM, et al. Essential role of lung plasmacytoid dendritic cells in preventing asthmatic reactions to harmless inhaled antigen. J Exp Med. (2004) 200:89–98. doi: 10.1084/jem.20040035
70. Amulic B, Cazalet C, Hayes GL, Metzler KD, Zychlinsky A. Neutrophil function: from mechanisms to disease. Annu Rev Immunol. (2012) 30:459–89. doi: 10.1146/annurev-immunol-020711-074942
71. Tecchio C, Micheletti A, Cassatella MA. Neutrophil-derived cytokines: facts beyond expression. Front Immunol. (2014) 5:508. doi: 10.3389/fimmu.2014.00508
72. Arokiasamy S, Zakian C, Dilliway J, Wang W, Nourshargh S, Voisin M-B. Endogenous TNFα orchestrates the trafficking of neutrophils into and within lymphatic vessels during acute inflammation. Sci Rep. (2017) 7:44189. doi: 10.1038/srep44189
73. Gorlino CV, Ranocchia RP, Harman MF, García IA, Crespo MI, Morón G, et al. Neutrophils exhibit differential requirements for homing molecules in their lymphatic and blood trafficking into draining lymph nodes. J Immunol. (2014) 193:1966–74. doi: 10.4049/jimmunol.1301791
74. Rigby DA, Ferguson DJP, Johnson LA, Jackson DG. Neutrophils rapidly transit inflamed lymphatic vessel endothelium via integrin-dependent proteolysis and lipoxin-induced junctional retraction. J Leukoc Biol. (2015) 98:897–912. doi: 10.1189/jlb.1HI0415-149R
75. Cahill RN, Frost H, Trnka Z. The effects of antigen on the migration of recirculating lymphocytes through single lymph nodes. J Exp Med. (1976) 143:870–88. doi: 10.1084/jem.143.4.870
76. Neeland MR, Shi W, Collignon C, Taubenheim N, Meeusen ENT, Didierlaurent AM, et al. The lymphatic immune response induced by the adjuvant AS01: a comparison of intramuscular and subcutaneous immunization routes. J Immunol. (2016) 197:2704–14. doi: 10.4049/jimmunol.1600817
77. Abadie V, Badell E, Douillard P, Ensergueix D, Leenen PJM, Tanguy M, et al. Neutrophils rapidly migrate via lymphatics after Mycobacterium bovis BCG intradermal vaccination and shuttle live bacilli to the draining lymph nodes. Blood. (2005) 106:1843–50. doi: 10.1182/blood-2005-03-1281
78. Bogoslowski A, Butcher EC, Kubes P. Neutrophils recruited through high endothelial venules of the lymph nodes via PNAd intercept disseminating Staphylococcus aureus. Proc Natl Acad Sci USA. (2018) 115:2449–54. doi: 10.1073/pnas.1715756115
79. Yang C-W, Strong BSI, Miller MJ, Unanue ER. Neutrophils influence the level of antigen presentation during the immune response to protein antigens in adjuvants. J Immunol. (2010) 185:2927–34. doi: 10.4049/jimmunol.1001289
80. Gresham HD, Lowrance JH, Caver TE, Wilson BS, Cheung AL, Lindberg FP. Survival of Staphylococcus aureus inside neutrophils contributes to infection. J Immunol. (2000) 164:3713–22. doi: 10.4049/jimmunol.164.7.3713
81. Peters NC, Egen JG, Secundino N, Debrabant A, Kimblin N, Kamhawi S, et al. In vivo imaging reveals an essential role for neutrophils in leishmaniasis transmitted by sand flies. Science. (2008) 321:970–4. doi: 10.1126/science.1159194
82. Chtanova T, Schaeffer M, Han S-J, van Dooren GG, Nollmann M, Herzmark P, et al. Dynamics of neutrophil migration in lymph nodes during infection. Immunity. (2008) 29:487–96. doi: 10.1016/j.immuni.2008.07.012
83. Beauvillain C, Cunin P, Doni A, Scotet M, Jaillon S, Loiry M-L, et al. CCR7 is involved in the migration of neutrophils to lymph nodes. Blood. (2011) 117:1196–204. doi: 10.1182/blood-2009-11-254490
84. Eash KJ, Greenbaum AM, Gopalan PK, Link DC. CXCR2 and CXCR4 antagonistically regulate neutrophil trafficking from murine bone marrow. J Clin Invest. (2010) 120:2423–31. doi: 10.1172/JCI41649
85. Dinauer MC. Primary immune deficiencies with defects in neutrophil function. Hematology. (2016) 2016:43–50. doi: 10.1182/asheducation-2016.1.43
86. Van de Weert–van Leeuwen PB, Van Meegen MA, Speirs JJ, Pals DJ, Rooijakkers SHM, Van der Ent CK, et al. Optimal complement-mediated phagocytosis of Pseudomonas aeruginosa by monocytes is cystic fibrosis transmembrane conductance regulator–dependent. Am J Respir Cell Mol Biol. (2013) 49:463–70. doi: 10.1165/rcmb.2012-0502OC
87. Smeekens SP, van de Veerdonk FL, Joosten LAB, Jacobs L, Jansen T, Williams DL, et al. The classical CD14++ CD16− monocytes, but not the patrolling CD14+ CD16+ monocytes, promote Th17 responses to Candida albicans. Eur J Immunol. (2011) 41:2915–24. doi: 10.1002/eji.201141418
88. Mohanty S, Joshi SR, Ueda I, Wilson J, Blevins TP, Siconolfi B, et al. Prolonged proinflammatory cytokine production in monocytes modulated by interleukin 10 after influenza vaccination in older adults. J Infect Dis. (2015) 211:1174–84. doi: 10.1093/infdis/jiu573
89. Ginhoux F, Jung S. Monocytes and macrophages: developmental pathways and tissue homeostasis. Nat Rev Immunol. (2014) 14:392–404. doi: 10.1038/nri3671
90. Calabro S, Tortoli M, Baudner BC, Pacitto A, Cortese M, O'Hagan DT, et al. Vaccine adjuvants alum and MF59 induce rapid recruitment of neutrophils and monocytes that participate in antigen transport to draining lymph nodes. Vaccine. (2011) 29:1812–23. doi: 10.1016/j.vaccine.2010.12.090
91. Leirião P, del Fresno C, Ardavín C. Monocytes as effector cells: activated Ly-6C(high) mouse monocytes migrate to the lymph nodes through the lymph and cross-present antigens to CD8+ T cells. Eur J Immunol. (2012) 42:2042–51. doi: 10.1002/eji.201142166
92. Jakubzick C, Gautier EL, Gibbings SL, Sojka DK, Schlitzer A, Johnson TE, et al. Minimal differentiation of classical monocytes as they survey steady-state tissues and transport antigen to lymph nodes. Immunity. (2013) 39:599–610. doi: 10.1016/j.immuni.2013.08.007
93. Becker HM, Rullo J, Chen M, Ghazarian M, Bak S, Xiao H, et al. α1β1 integrin-mediated adhesion inhibits macrophage exit from a peripheral inflammatory lesion. J Immunol. (2013) 190:4305–14. doi: 10.4049/jimmunol.1202097
94. Bellingan GJ, Caldwell H, Howie SE, Dransfield I, Haslett C. In vivo fate of the inflammatory macrophage during the resolution of inflammation: inflammatory macrophages do not die locally, but emigrate to the draining lymph nodes. J Immunol. (1996) 157:2577–85.
95. Hayes AJ, Rane S, Scales HE, Meehan GR, Benson RA, Maroof A, et al. Spatiotemporal modeling of the key migratory events during the initiation of adaptive immunity. Front Immunol. (2019) 10:598. doi: 10.3389/fimmu.2019.00598
96. Gascoigne NRJ, Rybakin V, Acuto O, Brzostek J. TCR signal strength and T cell development. Annu Rev Cell Dev Biol. (2016) 32:327–48. doi: 10.1146/annurev-cellbio-111315-125324
97. Wong P, Pamer EG. CD8 T cell responses to infectious pathogens. Annu Rev Immunol. (2003) 21:29–70. doi: 10.1146/annurev.immunol.21.120601.141114
98. Zhu J, Yamane H, Paul WE. Differentiation of effector CD4 T cell populations. Annu Rev Immunol. (2010) 28:445–89. doi: 10.1146/annurev-immunol-030409-101212
99. Mackay CR, Marston WL, Dudler L. Naive and memory T cells show distinct pathways of lymphocyte recirculation. J Exp Med. (1990) 171:801–17. doi: 10.1084/jem.171.3.801
100. Brown MN, Fintushel SR, Lee MH, Jennrich S, Geherin SA, Hay JB, et al. Chemoattractant receptors and lymphocyte egress from extralymphoid tissue: changing requirements during the course of inflammation. J Immunol. (2010) 185:4873–82. doi: 10.4049/jimmunol.1000676
101. Menning A, Höpken UE, Siegmund K, Lipp M, Hamann A, Huehn J. Distinctive role of CCR7 in migration and functional activity of naive- and effector/memory-like Treg subsets. Eur J Immunol. (2007) 37:1575–83. doi: 10.1002/eji.200737201
102. Gómez D, Diehl MC, Crosby EJ, Weinkopff T, Debes GF. Effector T cell egress via afferent lymph modulates local tissue inflammation. J Immunol. (2015) 195:3531–6. doi: 10.4049/jimmunol.1500626
103. Zhang N, Schröppel B, Lal G, Jakubzick C, Mao X, Chen D, et al. Regulatory T cells sequentially migrate from inflamed tissues to draining lymph nodes to suppress the alloimmune response. Immunity. (2009) 30:458–69. doi: 10.1016/j.immuni.2008.12.022
104. Bromley SK, Thomas SY, Luster AD. Chemokine receptor CCR7 guides T cell exit from peripheral tissues and entry into afferent lymphatics. Nat Immunol. (2005) 6:895–901. doi: 10.1038/ni1240
105. Ledgerwood LG, Lal G, Zhang N, Garin A, Esses SJ, Ginhoux F, et al. The sphingosine 1-phosphate receptor 1 causes tissue retention by inhibiting the entry of peripheral tissue T lymphocytes into afferent lymphatics. Nat Immunol. (2008) 9:42–53. doi: 10.1038/ni1534
106. Marttila-Ichihara F, Turja R, Miiluniemi M, Karikoski M, Maksimow M, Niemela J, et al. Macrophage mannose receptor on lymphatics controls cell trafficking. Blood. (2008) 112:64–72. doi: 10.1182/blood-2007-10-118984
107. Salmi M, Karikoski M, Elima K, Rantakari P, Jalkanen S. CD44 binds to macrophage mannose receptor on lymphatic endothelium and supports lymphocyte migration via afferent lymphatics. Circ Res. (2013) 112:1577–82. doi: 10.1161/CIRCRESAHA.111.300476
108. Karikoski M, Irjala H, Maksimow M, Miiluniemi M, Granfors K, Hernesniemi S, et al. Clever-1/Stabilin-1 regulates lymphocyte migration within lymphatics and leukocyte entrance to sites of inflammation. Eur J Immunol. (2009) 39:3477–87. doi: 10.1002/eji.200939896
109. Gray EE, Ramírez-Valle F, Xu Y, Wu S, Wu Z, Karjalainen KE, et al. Deficiency in IL-17-committed Vγ4+ γδ T cells in a spontaneous Sox13-mutant CD45.1+ congenic mouse substrain provides protection from dermatitis. Nat Immunol. (2013) 14:584–92. doi: 10.1038/ni.2585
110. Van Rhijn I, Rutten VPMG, Charleston B, Smits M, van Eden W, Koets AP. Massive, sustained γδ T cell migration from the bovine skin in vivo. J Leukoc Biol. (2007) 81:968–73. doi: 10.1189/jlb.0506331
111. Vrieling M, Santema W, Van Rhijn I, Rutten V, Koets A. γδ T cell homing to skin and migration to skin-draining lymph nodes is CCR7 independent. J Immunol. (2012) 188:578–84. doi: 10.4049/jimmunol.1101972
112. Mackay CR, Kimpton WG, Brandon MR, Cahill RN. Lymphocyte subsets show marked differences in their distribution between blood and the afferent and efferent lymph of peripheral lymph nodes. J Exp Med. (1988) 167:1755–65. doi: 10.1084/jem.167.6.1755
113. Noor S, Habashy AS, Nance JP, Clark RT, Nemati K, Carson MJ, et al. CCR7-dependent immunity during acute Toxoplasma gondii infection. Infect Immun. (2010) 78:2257–63. doi: 10.1128/IAI.01314-09
114. Bardina SV, Brown JA, Michlmayr D, Hoffman KW, Sum J, Pletnev AG, et al. Chemokine receptor CCR7 restricts fatal west nile virus encephalitis. J Virol. (2017) 91:e02409–16 doi: 10.1128/JVI.02409-16
115. Olmos S, Stukes S, Ernst JD. Ectopic activation of Mycobacterium tuberculosis-specific CD4+ T cells in lungs of CCR7−/− mice. J Immunol. (2010) 184:895–901. doi: 10.4049/jimmunol.0901230
116. Louveau A, Herz J, Alme MN, Salvador AF, Dong MQ, Viar KE, et al. CNS lymphatic drainage and neuroinflammation are regulated by meningeal lymphatic vasculature. Nat Neurosci. (2018) 21:1380–91. doi: 10.1038/s41593-018-0227-9
117. Dietrich T, Bock F, Yuen D, Hos D, Bachmann BO, Zahn G, et al. Cutting edge: lymphatic vessels, not blood vessels, primarily mediate immune rejections after transplantation. J Immunol. (2010) 184:535–9. doi: 10.4049/jimmunol.0903180
118. Kerjaschki D, Regele HM, Moosberger I, Nagy-Bojarski K, Watschinger B, Soleiman A, et al. Lymphatic neoangiogenesis in human kidney transplants is associated with immunologically active lymphocytic infiltrates. J Am Soc Nephrol. (2004) 15:603–12. doi: 10.1097/01.ASN.0000113316.52371.2E
119. Chen L, Hamrah P, Cursiefen C, Zhang Q, Pytowski B, Streilein JW, et al. Vascular endothelial growth factor receptor-3 mediates induction of corneal alloimmunity. Nat Med. (2004) 10:813–5. doi: 10.1038/nm1078
120. Weitman ES, Aschen SZ, Farias-Eisner G, Albano N, Cuzzone DA, Ghanta S, et al. Obesity impairs lymphatic fluid transport and dendritic cell migration to lymph nodes. PLoS ONE. (2013) 8:e70703. doi: 10.1371/journal.pone.0070703
121. Damas JK, Smith C, Øie E, Fevang B, Halvorsen B, Wæhre T, et al. Enhanced expression of the homeostatic chemokines CCL19 and CCL21 in clinical and experimental atherosclerosis. Arterioscler Thromb Vasc Biol. (2007) 27:614–20. doi: 10.1161/01.ATV.0000255581.38523.7c
122. Luchtefeld M, Grothusen C, Gagalick A, Jagavelu K, Schuett H, Tietge UJF, et al. Chemokine receptor 7 knockout attenuates atherosclerotic plaque development. Circulation. (2010) 122:1621–8. doi: 10.1161/CIRCULATIONAHA.110.956730
123. Wan W, Lionakis MS, Liu Q, Roffê E, Murphy PM. Genetic deletion of chemokine receptor Ccr7 exacerbates atherogenesis in ApoE-deficient mice. Cardiovasc Res. (2013) 97:580–8. doi: 10.1093/cvr/cvs349
124. Feig JE, Shang Y, Rotllan N, Vengrenyuk Y, Wu C, Shamir R, et al. Statins promote the regression of atherosclerosis via activation of the CCR7-dependent emigration pathway in macrophages. PLoS ONE. (2011) 6:e28534. doi: 10.1371/journal.pone.0028534
125. Trogan E, Feig JE, Dogan S, Rothblat GH, Angeli V, Tacke F, et al. Gene expression changes in foam cells and the role of chemokine receptor CCR7 during atherosclerosis regression in ApoE-deficient mice. Proc Natl Acad Sci USA. (2006) 103:3781–6. doi: 10.1073/pnas.0511043103
126. Roberts EW, Broz ML, Binnewies M, Headley MB, Nelson AE, Wolf DM, et al. Critical role for CD103+/CD141+ dendritic cells bearing CCR7 for tumor antigen trafficking and priming of T cell immunity in melanoma. Cancer Cell. (2016) 30:324–36. doi: 10.1016/j.ccell.2016.06.003
127. Su J-L, Yen C-J, Chen P-S, Chuang S-E, Hong C-C, Kuo I-H, et al. The role of the VEGF-C/VEGFR-3 axis in cancer progression. Br J Cancer. (2007) 96:541–5. doi: 10.1038/sj.bjc.6603487
128. Tammela T, Alitalo K. Lymphangiogenesis: molecular mechanisms and future promise. Cell. (2010) 140:460–76. doi: 10.1016/j.cell.2010.01.045
129. Skobe M, Hawighorst T, Jackson DG, Prevo R, Janes L, Velasco P, et al. Induction of tumor lymphangiogenesis by VEGF-C promotes breast cancer metastasis. Nat Med. (2001) 7:192–8. doi: 10.1038/84643
130. Yanai Y, Furuhata T, Kimura Y, Yamaguchi K, Yasoshima T, Mitaka T, et al. Vascular endothelial growth factor C promotes human gastric carcinoma lymph node metastasis in mice. J Exp Clin Cancer Res. (2001) 20:419–28.
131. Lane RS, Femel J, Breazeale AP, Loo CP, Thibault G, Kaempf A, et al. IFNγ-activated dermal lymphatic vessels inhibit cytotoxic T cells in melanoma and inflamed skin. J Exp Med. (2018) 215:3057–74. doi: 10.1084/jem.20180654
132. Lund AW, Duraes FV, Hirosue S, Raghavan VR, Nembrini C, Thomas SN, et al. VEGF-C promotes immune tolerance in B16 melanomas and cross-presentation of tumor antigen by lymph node lymphatics. Cell Rep. (2012) 1:191–9. doi: 10.1016/j.celrep.2012.01.005
133. Habenicht LM, Albershardt TC, Iritani BM, Ruddell A. Distinct mechanisms of B and T lymphocyte accumulation generate tumor-draining lymph node hypertrophy. Oncoimmunology. (2016) 5:e1204505. doi: 10.1080/2162402X.2016.1204505
Keywords: lymphatic, migration, neutrophils, T cells, dendritic cells
Citation: Hampton HR and Chtanova T (2019) Lymphatic Migration of Immune Cells. Front. Immunol. 10:1168. doi: 10.3389/fimmu.2019.01168
Received: 31 January 2019; Accepted: 08 May 2019;
Published: 28 May 2019.
Edited by:
Amanda W. Lund, Oregon Health & Science University, United StatesReviewed by:
Alanna Ruddell, University of Washington, United StatesMaria M. Steele, Oregon Health & Science University, United States
Copyright © 2019 Hampton and Chtanova. This is an open-access article distributed under the terms of the Creative Commons Attribution License (CC BY). The use, distribution or reproduction in other forums is permitted, provided the original author(s) and the copyright owner(s) are credited and that the original publication in this journal is cited, in accordance with accepted academic practice. No use, distribution or reproduction is permitted which does not comply with these terms.
*Correspondence: Tatyana Chtanova, dC5jaHRhbm92YUBnYXJ2YW4ub3JnLmF1