- 1Department of Pathology, Microbiology and Immunology, Albert Einstein College of Medicine, New York, NY, United States
- 2Department of Microbiology, Immunology and Cancer Biology, Carter Immunology Center, University of Virginia School of Medicine, Charlottesville, VA, United States
Until a few years ago, lymphatic vessels and lymphatic endothelial cells (LEC) were viewed as part of a passive conduit for lymph and immune cells to reach lymph nodes (LN). However, recent work has shown that LEC are active immunological players whose interaction with dendritic cells and T cells is of important immunomodulatory relevance. While the immunological interaction between LEC and other immune cells has taken a center stage, molecular analysis of LEC antigen processing and presentation machinery is still lagging. Herein we review the current knowledge of LEC MHC I and MHC II antigen processing and presentation pathways, Including the role of LEC in antigen phagocytosis, classical, and non-classical MHC II presentation, proteasome processing and MHC I presentation, and cross-presentation. The ultimate goal is to provide an overview of the LEC antigen processing and presentation machinery that constitutes the molecular basis for their role in MHC I and MHC II-restricted immune responses.
MHC I and MHC II Antigen Processing Machinery
MHC I and MHC II Molecules
Under basal physiological conditions both human and murine lymphatic endothelial cells (LEC) express both MHC class I and MHC class II molecules (1). However, as previously reported for blood endothelial cells (BEC) (2) the level of MHC II expression differs according to the anatomical location from which the cells are isolated (1, 3). LEC from LN (LN-LEC) express a high number of MHC II molecules while LEC from diaphragm express a much lower number (1). The MHC II surface expression in LN-LEC is similar to what observed in BEC but less than fibroblastic reticular cells from LN (1). LEC MHC II molecules are both endogenously synthesized or acquired from hematopoietic cells, as determined by chimera experiments in MHC II−/− mice (1, 4, 5). At the transcription level, MHC II expression is regulated by CIITA, which is not a DNA binding factor but instead a transactivator that regulates quantitative aspects of MHC-II expression by binding the MHC-II enhanceosome (6). CIITA expression is under the control of 4 different promoters (I, II, III, IV) and, in non-professional APC, MHC II expression is mostly regulated by CIITA IV (6). This promoter is responsive to IFNγ and other pro-inflammatory cytokines, which induce MHC II expression/up-regulation in fibroblasts and BEC (6). Similarly, in LEC it has been shown that endogenous MHC II expression is controlled by CIITA IV (4, 5). However, it is interesting to notice that, in contrast to other non-professional APC where pro-inflammatory stimuli greatly up-regulate surface MHC II molecules, pro-inflammatory stimuli induce less robust MHC II up-regulation in LEC (3, 5, 7). In the future, it would be of interest to analyze why, even though LEC express the type IV INFγ-inducible CIITA, they do not strongly up-regulate MHCII during pro-inflammatory conditions (5).
The Proteasome and TAP
Every cell expresses the constitutive 26S proteasome (8). This large barrel-shaped protein complex is formed in part by the catalytic 20S core, which consists of two pairs of outer α rings organized in seven α (α1–α7) subunits and two pairs of inner β rings organized in seven β subunits (β1–β7). The outer α subunits function as docking domains that regulate access of substrates to the catalytic chamber. Three of the β subunits (β1, β2, and β5) have proteolytic activities, including caspase-like activity (β1), trypsin-like activity (β2), and chymotrypsin-like activity (β5) (9). In the 26S proteasome, this 20S core is capped at both ends by the 19S regulatory complex (9). Ubiquitinated proteins are recognized by the 19S regulatory elements, which transfer them to the 20S for proteolysis (10). Peptides will then be transported in the ER by the transporter associated with antigen processing (TAP) and trimmed by the ER aminopeptidase I (ERAPI). In the ER the MHC class I heavy chain and β2m will transiently associates with TAP to load the peptides into the binding groove (11).
Following IFNγ or TNFα stimulation, new proteasome subunits are incorporated to generate the immunoproteasome (4, 12). β1 is exchanged with the large multifunctional peptidase 2 (LMP2) (also known as iβ1 or psmb9). β2 is exchanged with the multicatalytic endopeptidase complex-like-1 (MECL-1) (also known as iβ2 or psmb10). β5 is exchanged with the large multifunctional peptidase 7 (LMP7) (also known as iβ5 or psmb8). The 19S regulatory complex is exchanged with the Proteasome Activator α (PA28α) and PA28β, known as 11S regulator (2). The proteolytic functions of the immunoproteasome are greatly enhanced compared to those of the constitutive proteasome, as the immunoproteasome is more efficient in degrading ubiquitinated proteins and viral proteins, and in generating peptides for MHC-I presentation (13).
Until a few years ago, the presence of the proteasome in LEC was only indirectly analyzed by determining that TAP deficient mice were much less efficient in presenting MHC-I restricted OVA-derived peptides (7). However, a recent paper reported proteasome transcripts in LEC and BEC from different anatomical locations [Table 1 and (14)]. All of these endothelial populations expressed comparable transcript levels for constitutive proteasome subunits and 19S regulatory subunits. However, LEC and BEC from LN expressed 5-8-fold higher levels of transcripts for psmb8, psmb9, and psmb10, and twice as much PA28α and β. This suggests that LN-localized LEC preferentially express immunoproteasomes. Similarly, LN-localized LEC and BEC express 2–6 fold higher levels of TAP1 and TAP2, and twice as much ERAP1 and tapasin. Although these cells were isolated from resting LN, this suggests that their MHC-I processing and presentation capability is elevated.
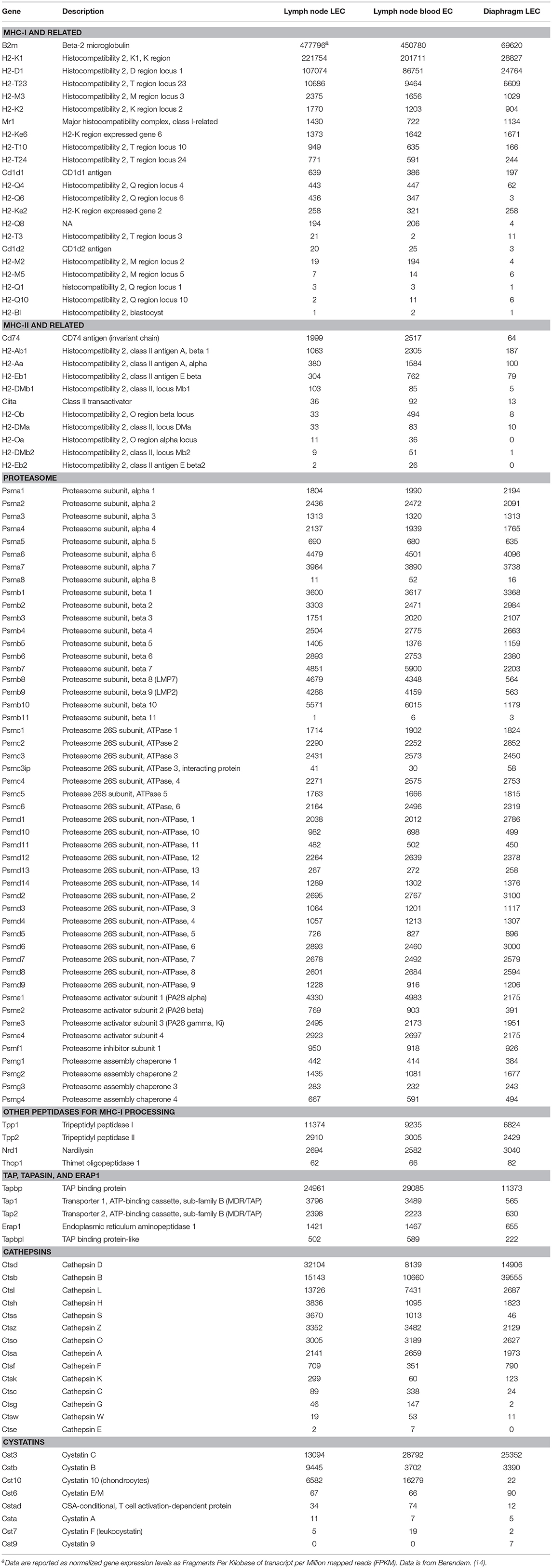
Table 1. Comparative transcriptome profiling of antigen processing and presentation pathway genes from mouse lymphatic and blood endothelial cells.
Other non-proteasomal proteases have been implicated in MHC-I presentation (15). These additional peptidases can trim the proteasome-generated N-extended precursors or even destroy epitopes, by trimming below the size needed for presentation. Among these, LEC from both LN and lymphatic vessels express significant and comparable transcript levels of tripeptidyl peptidases I and II and nardilysin, but negligible levels of thimet [Table 1 and (14)]. The functional implications of these additional LEC proteases, in generating the LEC MHC immunopeptidome, are currently unknown.
Endosomes and Lysosomes
Late endosomes (LE) and lysosomes (Lyso) are sub-cellular compartments, present in all cell types, specialized for the degradation of endogenous and exogenous materials for maintenance of cellular proteostasis and, in immune cells, for immunosurveillance (16). These organelles characteristically exhibit a low acidic pH, high concentrations of proteases, and expression of lysosome-associated membrane protein (Lamp) protein family members (16). In professional antigen presenting cells, LE and Lyso are also enriched in MHC class II proteins and molecules that regulate peptide loading (Invariant Chain, DM and DO) (17–21) and are referred as MHC class II compartments (MIIC) (22). Ultrastructurally these compartments can appear with different morphologies: multivesicular, multilamellar, or a combination of both (16).
Multivesicular bodies (MVB) are late endosomal compartments with a diameter of between 400 and 500 nm and a limiting membrane that encloses several internal vesicles with diameters of between 40 and 90 nm (16). MVB receive bio-synthetic cargo from the trans-Golgi, cytosolic cargo through autophagy, and exogenous proteins through phagocytosis. MVB are ubiquitously distributed and ultrastructural analysis has shown their presence in LEC (LS, unpublished observation) (23). However, it is currently unknown whether all/or a fraction of these compartments are MHC-II positive and whether there are differences in MHC-II expression in MVBs under steady state and inflammatory conditions. On the other hand the multilamellar bodies (MLB), which are lysosomal-like compartment formed by concentric lamellae and particularly enriched in MHC class II molecules (16) are more specifically expressed in professional APCs, such as DCs, B cells and macrophages, and they have not been found in LEC (LS unpublished observation).
Invariant Chain, DM, and DO
The MHC II molecules in association with their chaperone Invariant Chain, traffic from the trans-Golgi network to the plasma membrane before internalization to the endosomal MIIC. Sorting signals on the cytosolic tail of the Invariant chain are recognized by the clathrin-coated vesicle machinery for transport to LE/Lyso, where the Invariant chain will be processed by Cathepsins to generate class II-associated invariant chain peptides (CLIP), which occupy the MHC II binding groove and will be exchanged with peptides derived from endosomal processing (24). MHC II/Invariant Chain complexes are present at high levels in LEC and confocal microscopy, performed on primary LEC indicates that MHC II is correctly targeted both at the cell surface and in endosomal compartments (1).
HLA-DM (H-2M in mice) is part of the endosomal antigen processing and presentation machinery and aids peptide loading onto MHC II molecules. HLA-DM (DM) was originally discovered following the analysis of B-cell lines that were inefficient at presenting peptides derived from the processing of phagocytosed proteins but easily presented peptides supplied exogenously (17–19, 25, 26). It was later determined that these cells were defective in the expression of either the HLA-DMA or HLA-DMB genes. Subsequent in vitro and in vivo experiments determined that the role of DM is to catalyze CLIP removal, stabilize empty MHC II molecules for peptide loading and skew the immunopeptidome repertoire toward high affinity peptides (17, 25, 26). Mice lacking H-2M expressed similar I-Ab MHC II cell surface levels as wild type mice. However, the I-Ab MHC II molecules were less compact/SDS-resistant and were predominantly associated with CLIP (27). In contrast, lack of DM led to decreased peptide capture by I-Ad molecules, but enhanced peptide loading by I-Ed MHC II molecules (28). Finally, lack of DM generated a substantial pool of empty or loosely occupied I-Ak MHC II conformers with increase peptide binding activity. Mass spectrometry profiles confirmed the presence of an MHC II-peptidome in absence of DM (28, 29). Additionally DM requirements are different for CLIP binding in different registers (30). These results demonstrate that DM has distinct roles depending on its specific class II partners.
Subsequently, an additional protein, DO, was discovered, whose role is to inhibit DM function (18, 31). Importantly, while DM expression is not greatly increased following pro-inflammatory stimuli (TLR activation) that induces dendritic cell maturation, DO is down-regulated (32). As such it was hypothesized that high DO expression in immature dendritic cells would inhibit DM activity and skew the MHC II peptidome toward a broader and less stably bound repertoire. Upon DC maturation, reduced DO expression would lead to high DM activity, shaping the peptide repertoire toward long-lived surface class II MHC complexes, thus promoting productive immune responses (18, 33, 34).
Transcript analysis has shown that Invariant chain, I-A alpha, and I-A beta are expressed significantly in LEC and BEC from LN, but not LEC from lymphatic vessels, but DM and DO expression is very low to negligible, albeit DM is up-regulated following inflammatory stimuli (1, 4) [Table 1 and (14)]. Because removal of CLIP from I-Ab molecules is strongly DM dependent, this could explain the inefficient processing and presentation of I-Ab restricted antigens by LEC (1). However, the haplotype variation data described above indicate that general conclusions about the ability of LEC to present MHC II restricted antigens should await analysis of other mouse haplotypes.
Cathepsins
Cathepsins are a large family of serine, cysteine or aspartyl proteases that are present in endo-lysosomal compartments, and may be secreted at steady state or during pathological conditions (35). Cathepsins are most active at acid pH, can still function at neutral pH but are inactive at alkaline pH (36). Although these enzymes are present in most cells, certain cathepsins are enriched in particular antigen presenting cells. For example, Cathepsin S is highly expressed in dendritic cells and B cells, Cathepsin F in macrophages, and Cathepsin L in thymocytes (36–41).
Transcriptome analysis indicated that LEC from vessels express relatively low levels of cathepsins L and F, and negligible levels of Cathepsin S, while the levels of Cathepsin S and L were significantly elevated in LEC from LN [Table 1 and (14)]. However, measured Cathepsin L activity was variable among LN LEC and not evident in LEC from diaphragm (1). The activity of Cathepsin L indicates that at least some LEC could potentially cleave the Invariant Chain and generate CLIP peptides (1). Additionally, LEC could not efficiently process HA (an influenza membrane protein) and the IE-α protein as determined by either CD4 T cell recognition of the MHC II presented HA epitope or FACS analysis using the Y-Ae Ab that recognize I-Ab molecules loaded with the IE-α epitope, either under basal conditions or upon IFNγ stimulation (1). Furthermore, new evidence indicates that LEC express high levels of Cystatin C, B and 10 [Table 1 and (14)], which function as natural inhibitors of cathepsins (42). Altogether, the data point to the possibility that CatL and S activity in LEC is diminished, which could affect the generation of LIP10 and CLIP, and might also diminish the processing of other endogenous antigens.
Exogenous Peptides Binding and Antigen Exchange
The MHC I and MHC II presented immunopeptidome not only derives from endosomally processed proteins but also from pre-processed peptides that can be directly acquired from the extracellular milieu. Recent proteomic analyses have indicated that processed peptides are present in every biological fluid, among which lymph and blood, have been best characterized (43–50). The Eisen and Raghavan groups demonstrated binding of extracellular peptides to MHC I molecules and their regulation of CD8 T cell function (51, 52). Our group, among others, characterized extracellular peptide binding to MHC II surface molecules (44, 53–57). We determined that peptides carried in lymph were present in the HLA-DR1 immunopeptidome of immature dendritic cells and some of these peptides were not generated by endosomal processing, pinpointing the physiological relevance of MHC II surface/early endosomes loading (44). As such, the peptides present in the lymph, which derive from the metabolic and catabolic process of different parenchymal organs could contribute to the LEC MHC II immunopeptidome, since it has already been shown that LEC can readily bind and present pre-processed peptides (1).
Phagocytosis and Autophagy
Only very recently LEC have been analyzed for their ability to capture exogenous and endogenous antigens through phagocytosis. In vivo experiments using fluorescently labeled OVA indicated that within 90 min the subcutaneously injected protein was identified in LYVE-1+ cells, present in LN sub-capsular sinuses (7). Additionally, genes encoding several scavenger receptors, known to be involved in receptor-mediated endocytosis, are upregulated in LEC from lymph node (14). LEC efficiency in processing phagocytosed proteins through the MHC II pathway in steady state condition is low (1); nevertheless LEC can transfer Ags to dendritic cells, which are known to be present in close proximity with LEC in the lymphatic capillary and collectors, to induce CD4 T-cell anergy (1, 58). In addition, LEC efficiently present MHC-I peptides, and it has been reported that phagocytosis in early endosomes can route exogenous antigens (both self and non self) for cross-presentation on MHC class I in a proteasome and TAP-1-dependent manner (1, 3, 7, 58–61). It is interesting to consider that the acquisition of cross-presented material is mediated by these scavenger receptors. A second mechanism that can transfer endogenous proteins in the endosomes is autophagy. Although autophagy has been extensively characterized in BEC (62), there are no reports on the role of autophagy in antigen processing and presentation in LEC.
LEC and Pathogen Immunity
A growing body of evidences indicates that LEC are involved in immune response to pathogens. It has been recently reported that in extrapulmonary tuberculosis, the lymphatic system is the most common site of infection and LEC function as a niche for Mycobacterium tuberculosis (59). Indeed M. tuberculosis can replicate in the LEC cytosol and within autophagosomes suggesting that LEC are a previously unrecognized site for infection persistence. Similarly, Hantaviruses have been shown to have a tropism for lymphatic vessels and LEC infection with either Andes virus and Hantaan virus induces LEC hyperpermeabilization and pulmonary edema (63). The edema can be inhibited by αvβ3 integrin as well as VEGFR3 antibodies (63). A LEC role in HIV infection was also reported in promoting infection and latency formation in resting CD4+ T cells (64, 65). Recently an interesting role of LEC in antigen persistence, after resolution of the infection, has been shown (66). After viral challenge and vaccination, the antigen was captured by LEC under proliferative conditions and stored for extended periods of time. This “antigen archiving” mechanism positively influenced the degree of protective immunity provided by circulating memory CD8+ T-cells (66, 67).
Costimulatory and Co-Inhibitory Molecules
Effective activation of T-cells requires the display of MHC-I and MHC-II-peptide complexes as well as an antigen-independent signals provided by co-stimulatory molecules, among which CD40, CD80 (B7.1), and CD86 (B7.2) have been extensively analyzed in their requirements for naïve and memory T-cells activation (68, 69). LN LEC were shown to express very low levels of CD40 and negligible levels of CD80 and CD86 (3, 60). More recent transcriptome analysis has validated these observations, and extended them to include additional costimulatory molecules [Table 1 and (14)]. Importantly these costimulatory molecules did not up-regulate following stimulation with an MHC-I cognate ligand as well as inflammatory signals (TLRs binders or IFNγ) (3, 60).
In contrast, LEC in LN, but not in peripheral tissue lymphatics, express multiple inhibitory receptors that engage counter-receptors on activated T-cells to dampen the immune response (69). These include PD-L1 (CD274) and PD-L2 (CD273), which are present on resting LEC and greatly up-regulated by inflammatory stimuli (1, 70). Interestingly, the ligand for LAG-3, another inhibitory receptor on T-cells, is MHC-II, and induction of CD8 T-cell tolerance by LEC depends on engagement of LAG-3 as well as PD-1 (1, 60). Consequently, it has been suggested that in the absence of functional Ag presentation, the expression of MHC-II molecules on LEC is concerned with inducing Lag-3 mediated tolerance. While the low expression of costimulatory molecules would suggest that LEC would be unable to activate T-cells, they stimulate profound proliferation of CD8 T-cells in vivo and in vitro, and after peptide pulsing and CD4 T-cell proliferation in vitro (58, 60). However, the expression of the co-inhibitory molecules leads to deletional tolerance of CD8 T-cells due to a failure to sustain upregulation of the IL-2 receptor. Thus, LEC represent an important mechanism for mediation of systemic peripheral tolerance (58, 60, 61).
Exosomes and Other Vesicles
Most cells in the human body release vesicles of different sizes and content which can be classified as apoptotic bodies, micro and macrovesicles and exosomes (71). Exosomes are small (30–120 mm) vesicles generated from the multivesicular late endosomes upon fusion with the plasma membrane and release in the extracellular milieu. Exosomes from different sources have been shown to transport a protein cargo as well as mRNAs and microRNAs. Their physiological and pathological relevance has been established in several immune and cancer-related models (72). Although very little is known about LEC-released exosomes, recently it has been shown that LEC release a vesicular fraction, which includes exosomes, following an inflammatory signal (73). The LEC-derived exosomes are reportedly enriched with a motility-promoting protein signature, which act as a cue for the dendritic cells migratory response (73). In particular LEC released vesicles accumulate in the perivascular stroma of small lymphatic vessels, mostly in the presence of inflammatory cytokines and promote directional migration of CX3CR1-expressing cells (73).
Concluding Remarks
LEC cells are anatomically placed between parenchymal organs and draining lymph nodes, functioning as a conduit for the lymphatic fluid and are known to control DC and T cell migration in and out of the lymph node (74, 75). During the last few years their functionality in antigen processing and presentation and T cell immune responses has emerged. Under steady-state conditions LEC can present self-antigens to induce T cell tolerance either through expression of peripheral tissue antigens (76) or acquisition of extracellular antigens through phagocytosis or by acquisition of pre-loaded MHC II molecules from DC. Under inflammatory conditions LEC also play an immunosuppressive role by decreasing DC maturation (77) and by up-regulating surface PDL1 (76).
However, the advances in understanding the cross-talk between LEC and T cells has not been paralleled by a detailed mechanistic analysis of their antigen processing and presentation machinery. Characterization of LEC immunoproteasomes, endosomal processing compartments, and antigen acquisition from the lymphatic fluid still needs to be investigated. Nevertheless, the work to date points to an emerging picture of the role played by LEC in maintenance of self-tolerance.
Author Contributions
LS and VE wrote the review. SB contributed the primary data presented in the table.
Funding
Support was provided by United States Public Health Services Research Grant R21 AI109250 (VE) and RO1 AG045223 (LS).
Conflict of Interest Statement
The authors declare that the research was conducted in the absence of any commercial or financial relationships that could be construed as a potential conflict of interest.
Abbreviations
BEC, blood endothelial cells; LEC, lymphatic endothelial cells; LN, lymph node; LN-BEC, lymph node-associated blood endothelial cells; LN-LEC, lymph node-associated lymphatic endothelial cells; DC, dendritic cells; LE, late endosomes; Lyso, lysosomes; MIIC, MHC class II compartments; MVB, multivesicular bodies; MLB, multilamellar bodies; DM, HLA-DM; Ii, invariant chain; DO, HLA-DO.
References
1. Rouhani SJ, Eccles JD, Riccardi P, Peske JD, Tewalt EF, Cohen JN, et al. Roles of lymphatic endothelial cells expressing peripheral tissue antigens in CD4 T-cell tolerance induction. Nat Commun. (2015) 6:6771. doi: 10.1038/ncomms7771
2. Pober JS, Merola J, Liu R, Manes TD. Antigen presentation by vascular cells. Front Immunol. (2017) 8:1907. doi: 10.3389/fimmu.2017.01907
3. Tewalt EF, Cohen JN, Rouhani SJ, Engelhard VH. Lymphatic endothelial cells - key players in regulation of tolerance and immunity. Front Immunol. (2012) 3:305. doi: 10.3389/fimmu.2012.00305
4. Dubrot J, Duraes FV, Potin L, Capotosti F, Brighouse D, Suter T, et al. Lymph node stromal cells acquire peptide-MHCII complexes from dendritic cells and induce antigen-specific CD4(+) T cell tolerance. J Exp Med. (2014) 211:1153–66. doi: 10.1084/jem.20132000
5. Dubrot J, Duraes FV, Harle G, Schlaeppi A, Brighouse D, Madelon N, et al. Absence of MHC-II expression by lymph node stromal cells results in autoimmunity. Life Sci Alliance. (2018) 1:e201800164. doi: 10.26508/lsa.201800164
6. LeibundGut-Landmann S, Waldburger JM, Krawczyk M, Otten LA, Suter T, Fontana A, et al. Mini-review: specificity and expression of CIITA, the master regulator of MHC class II genes. Eur J Immunol. (2004) 34:1513–25. doi: 10.1002/eji.200424964
7. Hirosue S, Vokali E, Raghavan VR, Rincon-Restrepo M, Lund AW, Corthesy-Henrioud P, et al. Steady-state antigen scavenging, cross-presentation, and CD8+ T cell priming:a new role for lymphatic endothelial cells. J Immunol. (2014) 192:5002–11. doi: 10.4049/jimmunol.1302492
8. Bard JAM, Goodall EA, Greene ER, Jonsson E, Dong KC, Martin, A. Structure and function of the 26S proteasome. Annu Rev Biochem. (2018) 87:697–724. doi: 10.1146/annurev-biochem-062917-011931
9. Rousseau A, Bertolotti A. Regulation of proteasome assembly and activity in health and disease. Nat Rev Mol Cell Biol. (2018) 19:697–712. doi: 10.1038/s41580-018-0040-z
10. Yu H, Matouschek A. Recognition of Client Proteins by the Proteasome. Annu Rev Biophys. (2017) 46:149–73. doi: 10.1146/annurev-biophys-070816-033719
11. Hansen TH, Bouvier M. MHC class I antigen presentation:learning from viral evasion strategies. Nat Rev Immunol. (2009) 9:503–13. doi: 10.1038/nri2575
12. Ferrington DA, Gregerson DS. Immunoproteasomes:structure, function, and antigen presentation. Prog Mol Biol Transl Sci. (2012) 109:75–112. doi: 10.1016/B978-0-12-397863-9.00003-1
13. Kimura H, Caturegli P, Takahashi M, Suzuki, K. New insights into the function of the immunoproteasome in immune and nonimmune cells. J Immunol Res. (2015) 2015:541984. doi: 10.1155/2015/541984
14. Berendam SJ, Koeppel AF, Godfrey NR, Rouhani SJ, Woods AN, Rodriguez AB, et al. Comparative transcriptomic analysis identify a range of immunologically related functional elaborations of lymph node associated lymphatic and blood endothelial cells. Front Immunol. (2019). doi: 10.3389/fimmu.2019.00816
15. Kloetzel PM, Ossendorp, F. Proteasome and peptidase function in MHC-class-I-mediated antigen presentation. Curr Opin Immunol. (2004) 16:76–81. doi: 10.1016/j.coi.2003.11.004
16. Stern LJ, Potolicchio I, Santambrogio, L. MHC class II compartment subtypes:structure and function. Curr Opin Immunol. (2006) 18:64–9. doi: 10.1016/j.coi.2005.11.005
17. Doebele RC, Busch R, Scott HM, Pashine A, Mellins ED. Determination of the HLA-DM interaction site on HLA-DR molecules. Immunity. (2000) 13:517–27. doi: 10.1016/S1074-7613(00)00051-0
18. Mellins ED, Stern LJ. HLA-DM and HLA-DO, key regulators of MHC-II processing and presentation. Curr Opin Immunol. (2014) 26:115–22. doi: 10.1016/j.coi.2013.11.005
19. Denzin LK, Cresswell, P. HLA-DM induces CLIP dissociation from MHC class II alpha beta dimers and facilitates peptide loading. Cell. (1995) 82:155–65. doi: 10.1016/0092-8674(95)90061-6
20. van Ham SM, Tjin EP, Lillemeier BF, Gruneberg U, van Meijgaarden KE, Pastoors L, et al. HLA-DO is a negative modulator of HLA-DM-mediated MHC class II peptide loading. Curr Biol. (1997) 7:950–7. doi: 10.1016/S0960-9822(06)00414-3
21. Roche PA, Furuta, K. The ins and outs of MHC class II-mediated antigen processing and presentation. Nat Rev Immunol. (2015) 15:203–16. doi: 10.1038/nri3818
22. Tulp A, Verwoerd D, Dobberstein B, Ploegh HL, Pieters, J. Isolation and characterization of the intracellular MHC class II compartment. Nature. (1994) 369:120–6. doi: 10.1038/369120a0
23. Zolla V, Nizamutdinova IT, Scharf B, Clement CC, Maejima D, Akl T, et al. Aging-related anatomical and biochemical changes in lymphatic collectors impair lymph transport, fluid homeostasis, and pathogen clearance. Aging Cell. (2015) 14:582–94. doi: 10.1111/acel.12330
24. Cresswell P, Roche PA. Invariant chain-MHC class II complexes:always odd and never invariant. Immunol Cell Biol. (2014) 92:471–2. doi: 10.1038/icb.2014.36
25. Morris P, Shaman J, Attaya M, Amaya M, Goodman S, Bergman C, et al. An essential role for HLA-DM in antigen presentation by class II major histocompatibility molecules. Nature. (1994) 368:551–4. doi: 10.1038/368551a0
26. Sloan VS, Cameron P, Porter G, Gammon M, Amaya M, Mellins E, et al. Mediation by HLA-DM of dissociation of peptides from HLA-DR. Nature. (1995) 375:802–6. doi: 10.1038/375802a0
27. Martin WD, Hicks GG, Mendiratta SK, Leva HI, Ruley HE, Van Kaer L. H2-M mutant mice are defective in the peptide loading of class II molecules, antigen presentation, and T cell repertoire selection. Cell. (1996) 84:543–50. doi: 10.1016/S0092-8674(00)81030-2
28. Koonce CH, Wutz G, Robertson EJ, Vogt AB, Kropshofer H, Bikoff EK. DM loss in k haplotype mice reveals isotype-specific chaperone requirements. J Immunol. (2003) 170:3751–61. doi: 10.4049/jimmunol.170.7.3751
29. Bikoff EK, Wutz G, Kenty GA, Koonce CH, Robertson EJ. Relaxed DM requirements during class II peptide loading and CD4+ T cell maturation in BALB/c mice. J Immunol. (2001) 166:5087–98. doi: 10.4049/jimmunol.166.8.5087
30. Belmares MP, Busch R, Mellins ED, McConnell HM. Formation of two peptide/MHC II isomers is catalyzed differentially by HLA-DM. Biochemistry. (2003) 42:838–47. doi: 10.1021/bi020466p
31. Guce AI, Mortimer SE, Yoon T, Painter CA, Jiang W, Mellins ED, et al. HLA-DO acts as a substrate mimic to inhibit HLA-DM by a competitive mechanism. Nat Struct Mol Biol. (2013) 20:90–8. doi: 10.1038/nsmb.2460
32. Fallas JL, Tobin HM, Lou O, Guo D, Sant'Angelo DB, Denzin LK. Ectopic expression of HLA-DO in mouse dendritic cells diminishes MHC class II antigen presentation. J Immunol. (2004) 173:1549–60. doi: 10.4049/jimmunol.173.3.1549
33. Yi W, Seth NP, Martillotti T, Wucherpfennig KW, Sant'Angelo DB, Denzin LK. Targeted regulation of self-peptide presentation prevents type I diabetes in mice without disrupting general immunocompetence. J Clin Invest. (2010) 120:1324–36. doi: 10.1172/JCI40220
34. Santambrogio L, DiLorenzo TP. Autoimmunity. The benefit of self-control. Immunol Cell Biol. (2010) 88:513–4. doi: 10.1038/icb.2010.59
35. Kramer L, Turk D, Turk, B. The future of cysteine cathepsins in disease management. Trends Pharmacol Sci. (2017) 38:873–98. doi: 10.1016/j.tips.2017.06.003
36. Turk V, Stoka V, Vasiljeva O, Renko M, Sun T, Turk B, et al. Cysteine cathepsins:from structure, function and regulation to new frontiers. Biochim Biophys Acta. (2012) 1824:68–88. doi: 10.1016/j.bbapap.2011.10.002
37. Riese RJ, Chapman HA. Cathepsins and compartmentalization in antigen presentation. Curr Opin Immunol. (2000) 12:107–13. doi: 10.1016/S0952-7915(99)00058-8
38. Mason RW, Johnson DA, Barrett AJ, Chapman HA. Elastinolytic activity of human cathepsin L. Biochem J. (1986) 233:925–7. doi: 10.1042/bj2330925
39. Reilly JJ, Chen P, Sailor LZ, Mason RW, Chapman HA. Uptake of extracellular enzyme by a novel pathway is a major determinant of cathepsin L levels in human macrophages. J Clin Invest. (1990) 86:176–83. doi: 10.1172/JCI114682
40. Shi GP, Bryant RA, Riese R, Verhelst S, Driessen C, Li Z, et al. Role for cathepsin F in invariant chain processing and major histocompatibility complex class II peptide loading by macrophages. J Exp Med. (2000) 191:1177–86. doi: 10.1084/jem.191.7.1177
41. Shi GP, Villadangos JA, Dranoff G, Small C, Gu L, Haley KJ, et al. Cathepsin S required for normal MHC class II peptide loading and germinal center development. Immunity. (1999) 10:197–206. doi: 10.1016/S1074-7613(00)80020-5
42. Kopitar-Jerala N. The role of cystatins in cells of the immune system. FEBS Lett. (2006) 580:6295–301. doi: 10.1016/j.febslet.2006.10.055
43. Clement CC, Aphkhazava D, Nieves E, Callaway M, Olszewski W, Rotzschke O, et al. Protein expression profiles of human lymph and plasma mapped by 2D-DIGE and 1D SDS-PAGE coupled with nanoLC-ESI-MS/MS bottom-up proteomics. J Proteom. (2013) 78:172–87. doi: 10.1016/j.jprot.2012.11.013
44. Clement CC, Becerra A, Yin L, Zolla V, Huang L, Merlin S, et al. The dendritic cell major histocompatibility complex II (MHC II) peptidome derives from a variety of processing pathways and includes peptides with a broad spectrum of HLA-DM sensitivity. J Biol Chem. (2016) 291:5576–95. doi: 10.1074/jbc.M115.655738
45. Clement CC, Cannizzo ES, Nastke MD, Sahu R, Olszewski W, Miller NE, et al. An expanded self-antigen peptidome is carried by the human lymph as compared to the plasma. PLoS ONE. (2010) 5:e9863. doi: 10.1371/journal.pone.0009863
46. Clement CC, Rotzschke O, Santambrogio, L. The lymph as a pool of self-antigens. Trends Immunol. (2011) 32:6–11. doi: 10.1016/j.it.2010.10.004
47. Clement CC, Santambrogio, L. The lymph self-antigen repertoire. Front Immunol. (2013) 4:424. doi: 10.3389/fimmu.2013.00424
48. Petricoin EF, Belluco C, Araujo RP, Liotta LA. The blood peptidome:a higher dimension of information content for cancer biomarker discovery. Nat Rev Cancer. (2006) 6:961–7. doi: 10.1038/nrc2011
49. Smith CR, Batruch I, Bauca JM, Kosanam H, Ridley J, Bernardini MQ, et al. Deciphering the peptidome of urine from ovarian cancer patients and healthy controls. Clin Proteom. (2014) 11:23. doi: 10.1186/1559-0275-11-23
50. Ling XB, Lau K, Deshpande C, Park JL, Milojevic D, Macaubas C, et al. Urine peptidomic and targeted plasma protein analyses in the diagnosis and monitoring of systemic juvenile idiopathic arthritis. Clin Proteom. (2010) 6:175–93. doi: 10.1007/s12014-010-9058-8
51. Eisen HN, Hou XH, Shen C, Wang K, Tanguturi VK, Smith C, et al. Promiscuous binding of extracellular peptides to cell surface class I MHC protein. Proc Natl Acad Sci USA. (2012) 109:4580–5. doi: 10.1073/pnas.1201586109
52. Geng J, Altman JD, Krishnakumar S, Raghavan, M. Empty conformers of HLA-B preferentially bind CD8 and regulate CD8(+) T cell function. Elife. (2018) 7:e36341. doi: 10.7554/eLife.36341
53. Potolicchio I, Chitta S, Xu X, Fonseca D, Crisi G, Horejsi V, et al. Conformational variation of surface class II MHC proteins during myeloid dendritic cell differentiation accompanies structural changes in lysosomal MIIC. J Immunol. (2005) 175:4935–47. doi: 10.4049/jimmunol.175.8.4935
54. Bosch B, Berger AC, Khandelwal S, Heipertz EL, Scharf B, Santambrogio L, et al. Disruption of multivesicular body vesicles does not affect major histocompatibility complex (MHC) class II-peptide complex formation and antigen presentation by dendritic cells. J Biol Chem. (2013) 288:24286–92. doi: 10.1074/jbc.M113.461996
55. Chou CL, Mirshahidi S, Su KW, Kim A, Narayan K, Khoruzhenko S, et al. Short peptide sequences mimic HLA-DM functions. Mol Immunol. (2008) 45:1935–43. doi: 10.1016/j.molimm.2007.10.033
56. Marin-Esteban V, Falk K, Rotzschke O. “Chemical analogues” of HLA-DM can induce a peptide-receptive state in HLA-DR molecules. J Biol Chem. (2004) 279:50684–90. doi: 10.1074/jbc.M407598200
57. Rupp B, Gunther S, Makhmoor T, Schlundt A, Dickhaut K, Gupta S, et al. Characterization of structural features controlling the receptiveness of empty class II MHC molecules. PLoS ONE. (2011) 6:e18662. doi: 10.1371/journal.pone.0018662
58. Rouhani SJ, Eccles JD, Tewalt EF, Engelhard VH. Regulation of T-cell tolerance by lymphatic endothelial cells. J Clin Cell Immunol. (2014) 5:242. doi: 10.4172/2155-9899.1000242
59. Lerner TR, de Souza Carvalho-Wodarz C, Repnik U, Russell MR, Borel S, Diedrich CR, et al. Lymphatic endothelial cells are a replicative niche for Mycobacterium tuberculosis. J Clin Invest. (2016) 126:1093–108. doi: 10.1172/JCI83379
60. Tewalt EF, Cohen JN, Rouhani SJ, Guidi CJ, Qiao H, Fahl SP, et al. Lymphatic endothelial cells induce tolerance via PD-L1 and lack of costimulation leading to high-level PD-1 expression on CD8 T cells. Blood. (2012) 120:4772–82. doi: 10.1182/blood-2012-04-427013
61. Cohen JN, Guidi CJ, Tewalt EF, Qiao H, Rouhani SJ, Ruddell A, et al. Lymph node-resident lymphatic endothelial cells mediate peripheral tolerance via Aire-independent direct antigen presentation. J Exp Med. (2010) 207:681–8. doi: 10.1084/jem.20092465
62. Gatica D, Chiong M, Lavandero S, Klionsky DJ. Molecular mechanisms of autophagy in the cardiovascular system. Circ Res. (2015) 116:456–67. doi: 10.1161/CIRCRESAHA.114.303788
63. Mackow ER, Gorbunova EE, Dalrymple NA, Gavrilovskaya IN. Role of vascular and lymphatic endothelial cells in hantavirus pulmonary syndrome suggests targeted therapeutic approaches. Lymphat Res Biol. (2013) 11:128–35. doi: 10.1089/lrb.2013.0006
64. Schilthuis M, Verkaik S, Walhof M, Philipose A, Harlow O, Kamp D, et al. Lymphatic endothelial cells promote productive and latent HIV infection in resting CD4+ T cells. Virol J. (2018) 15:152. doi: 10.1186/s12985-018-1068-6
65. Zhang X, Jiang S, Yu J, Kuzontkoski PM, Groopman JE. Cocaine enhances HIV-1 gp120-induced lymphatic endothelial dysfunction in the lung. Physiol Rep. (2015) 3:e12482. doi: 10.14814/phy2.12482
66. Tamburini BA, Burchill MA, Kedl RM. Antigen capture and archiving by lymphatic endothelial cells following vaccination or viral infection. Nat Commun. (2014) 5:3989. doi: 10.1038/ncomms4989
67. Kedl RM, Lindsay RS, Finlon JM, Lucas ED, Friedman RS, Tamburini BAJ. Migratory dendritic cells acquire and present lymphatic endothelial cell-archived antigens during lymph node contraction. Nat Commun. (2017) 8:2034. doi: 10.1038/s41467-017-02247-z
68. Schildberg FA, Klein SR, Freeman GJ, Sharpe AH. Coinhibitory pathways in the B7-CD28 ligand-receptor family. Immunity. (2016) 44:955–72. doi: 10.1016/j.immuni.2016.05.002
69. Zang X, Allison JP. The B7 family and cancer therapy:costimulation and coinhibition. Clin Cancer Res. (2007) 13:5271–9. doi: 10.1158/1078-0432.CCR-07-1030
70. Thomas SN, Rutkowski JM, Pasquier M, Kuan EL, Alitalo K, Randolph GJ, et al. Impaired humoral immunity and tolerance in K14-VEGFR-3-Ig mice that lack dermal lymphatic drainage. J Immunol. (2012) 189:2181–90. doi: 10.4049/jimmunol.1103545
71. Zhang H, Freitas D, Kim HS, Fabijanic K, Li Z, Chen H, et al. Identification of distinct nanoparticles and subsets of extracellular vesicles by asymmetric flow field-flow fractionation. Nat Cell Biol. (2018) 20:332–43. doi: 10.1038/s41556-018-0040-4
72. Meldolesi J. Exosomes and ectosomes in intercellular communication. Curr Biol. (2018) 28:R435–44. doi: 10.1016/j.cub.2018.01.059
73. Brown M, Johnson LA, Leone DA, Majek P, Vaahtomeri K, Senfter D, et al. Lymphatic exosomes promote dendritic cell migration along guidance cues. J Cell Biol. (2018) 217:2205–21. doi: 10.1083/jcb.201612051
74. Yeo KP, Angeli V. Bidirectional crosstalk between lymphatic endothelial cell and T cell and its implications in tumor immunity. Front Immunol. (2017) 8:83. doi: 10.3389/fimmu.2017.00083
75. Russo E, Teijeira A, Vaahtomeri K, Willrodt AH, Bloch JS, Nitschke M, et al. Intralymphatic CCL21 promotes tissue egress of dendritic cells through afferent lymphatic vessels. Cell Rep. (2016) 14:1723–34. doi: 10.1016/j.celrep.2016.01.048
76. Fletcher AL, Acton SE, Knoblich, K. Lymph node fibroblastic reticular cells in health and disease. Nat Rev Immunol. (2015) 15:350–61. doi: 10.1038/nri3846
Keywords: lymphatic endothelial cells, MHC class I, MHC class II, antigen processing and presentation, lymph
Citation: Santambrogio L, Berendam SJ and Engelhard VH (2019) The Antigen Processing and Presentation Machinery in Lymphatic Endothelial Cells. Front. Immunol. 10:1033. doi: 10.3389/fimmu.2019.01033
Received: 26 February 2019; Accepted: 23 April 2019;
Published: 07 May 2019.
Edited by:
Amanda W. Lund, Oregon Health & Science University, United StatesReviewed by:
Stephanie Hugues, Université de Genève, SwitzerlandRoss M. Kedl, University of Colorado Denver, United States
Copyright © 2019 Santambrogio, Berendam and Engelhard. This is an open-access article distributed under the terms of the Creative Commons Attribution License (CC BY). The use, distribution or reproduction in other forums is permitted, provided the original author(s) and the copyright owner(s) are credited and that the original publication in this journal is cited, in accordance with accepted academic practice. No use, distribution or reproduction is permitted which does not comply with these terms.
*Correspondence: Laura Santambrogio, bGF1cmEuc2FudGFtYnJvZ2lvQGVpbnN0ZWluLnl1LmVkdQ==