- 1Department of Immunology, Faculty of Medicine, Shahid Sadoughi University of Medical Sciences, Yazd, Iran
- 2Students' Scientific Research Center, Tehran University of Medical Sciences, Tehran, Iran
- 3Department of Community Nutrition, School of Nutritional Sciences and Dietetics, Tehran University of Medical Sciences, Tehran, Iran
- 4Department of Nutrition, School of Health, Shahid Sadoughi University of Medical Sciences, Yazd, Iran
- 5Department of Pharmaceutical Science, University of British Columbia, Vancouver, BC, Canada
- 6Department of Chemistry, Franklin & Marshall College, Lancaster, PA, United States
- 7Obesity and Eating Habits Research Center, Endocrinology and Metabolism Molecular-Cellular Sciences Institute, Tehran University of Medical Sciences, Tehran, Iran
- 8Department of Community Nutrition, Food Security Research Center, School of Nutrition and Food Science, Isfahan University of Medical Sciences, Isfahan, Iran
The increasing rate of autoimmune disorders and cancer in recent years has been a controversial issue in all aspects of prevention, diagnosis, prognosis and treatment. Among dietary factors, flavonoids have specific immunomodulatory effects that might be of importance to several cancers. Over different types of immune cells, T lymphocytes play a critical role in protecting the immune system as well as in the pathogenesis of specific autoimmune diseases. One of the important mediators of metabolism and immune system is mTOR, especially in T lymphocytes. In the current review, we assessed the effects of flavonoids on the immune system and then their impact on the mTOR pathway. Flavonoids can suppress mTOR activity and are consequently able to induce the T regulatory subset.
Introduction
Nutrition and metabolism play an important and undeniable role in public health. Although genes have specific importance in susceptibility to diseases, some environmental factors can affect a gene's ability to “switch on or off” (1). In fact, phenotypes are determined by a combination of genotypic and environmental factors (2). Diet is one of the environmental factors that could be considered in the prevention and treatment of several disorders (3), including some autoimmune diseases as MS (3, 4) and type I diabetes (4). Chemo-preventive effects of diet on cancer (5, 6) and autoimmune diseases have also been reported (7, 8).
The immune system plays a critical role in protecting the human body from infectious diseases and cancer. Its two main contributors include innate and acquired immunity responses. The most important feature of innate immunity is its lack of specific recognition. This arm of the immune system responds to all pathogens regardless to their nature (9). In contrast to innate immunity, acquired immunity recognizes pathogens specifically and responds to each pathogen according to its nature. Innate immunity is composed of immune and non-immune components, whilst acquired immunity has only immune elements. The major functions of the acquired immune system rely on immune cells, mainly B and T lymphocytes that recognize pathogens based on their antigenic receptors and respond in different ways. B cells produce antibodies to block pathogen activity and opsonize them for phagocytes. T cells are divided into two subsets: T cytotoxic cells (T CD8+) which kill cancer cells directly and T helper cells (T CD4+) that secrete cytokines and mediators that orchestrate other cells such as B lymphocytes and macrophages (9–11). Th cells secrete a wide range of cytokines, which can direct the type of antibodies produced by B cells and also are able to activate and polarize monocytes and macrophages. In light of this, Th cells play a central role in the immune system (12).
One of the research areas of immunometabolism is to study the effect of different metabolites on Th cell differentiation. Many researches have focused on the effects of glucose (13), oxygen (14), salt (3), fatty acids (1, 15–17), vitamins (18–20), and amino acids (21, 22) on mechanisms involved in Th cell differentiation. The importance of Th cell differentiation becomes clear when we consider uncontrolled Teff activation against self-antigen that triggers an immune response, through which damages self-tissue and interrupts some organ functions. One of the important dietary factors studied in terms of immunomodulatory effects are polyphenols and their effects on the level and composition of immunoglobulins, inflammation, immune cell population content and also their antioxidant effects on cancer cells have been investigated (23–25). Several studies have reported immunomodulating effects of polyphenols (26, 27). However, it remains unknown whether Th cells and changes in the ratio of inflammatory/regulatory cells can mediate such effects of polyphenols. Inflammatory and regulatory subsets of Th cells have different metabolic demands. In inflammatory subsets of Th cells, the mTOR pathway is activated. This pathway promotes glycolysis to supply their energy needs. This pathway is inactive in regulatory cells. If the mTOR pathway is suppressed in inflammatory subsets, they differentiate into the regulatory subset. Therefore, activation or suppression of the mTOR pathway determines Th cell differentiation into inflammatory and regulatory subsets (Figure 1). It is still unknown if flavonoids can suppress mTOR function and consequently induce Treg subsets. The current study summarizes the effects of flavonoids on the immune system and subsequently the role of specific pathways like PI3k/Akt/mTOR on immunomodulation and possible effects of polyphenols on this pathway.
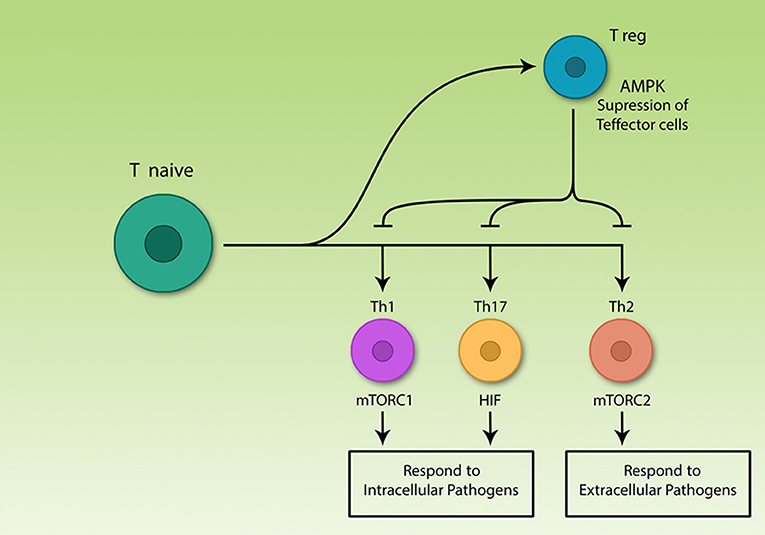
Figure 1. The central role of T lymphocytes in acquired immunity. Acquired immune cells, notably lymphocytes, recognize pathogens specifically and respond to them, according to their nature. T helper lymphocytes secrete various cytokines which are able to activate different immune cells including acquired and innate cells. The various Th subsets rely on different signaling pathways, but all effector subsets upregulate glycolysis. Among differentiated Th cells, T regulatory cells are the only non-glycolytic cells; effector subsets use different signaling molecules, however, all are glycolytic.
Th cell subsets are classified into Th1, Th2, Th17, and Treg. Th1, Th2, and Th17 are effector subsets and trigger immune response to different pathogens (13, 28, 29) while Treg cells restore homeostasis by suppression of Teff cell function after termination of immune response (30, 31). Precise function of the immune system is important for correct immune response, otherwise two pathological types of responses might occur. On the one hand, if the immune system fails to detect pathogens or cancer cells for any reason, the risk of developing disease increases (32). On the other hand, if the immune system cells by mistake identify self-antigens as foreign agents, the response of killer cells and/or antibody-producing cells interfering with cytokine levels, can result in serious damages to the body (33).
Currently, there is only sketchy understanding of the factors and mechanisms involved in autoimmunity. The loss of self-tolerance is one of the important causes of disease. Although the mechanism of loss of immune tolerance is not yet fully understood, some behaviors like smoking may interfere with tolerance and lead to autoimmunity disorders (34). For this reason, other environmental factors like diet also play a critical role in immune tolerance failure. In normal immune function, both central and peripheral tolerance mechanisms do not allow the immune system to respond against self-antigens (14). Treg cells are one of these tolerance mechanisms, since Treg cell suppress Teff cells and consequently is able to block unwanted prolonged immune response (35). Depending on the types of immune cells involved, symptoms, and treatment of this disease differ.
In addition to the possible role of diet in immune tolerance failure, this question arises whether the immune system and various metabolites can interact with each other. In fact, not only metabolites can affect the immune system, but also the function of the immune system affects metabolic tissues. This mutual interaction is referred as immunometabolism. Interestingly, malnutrition has a negative impact on immune function. But what is more, oversupply of metabolites is destructive as well (36).
As mentioned above, flavonoids generally have immunomodulatory effects on the immune system. To understand it better, at first we look at the effects of four common flavonoids on immune system. Here we get general information about the effects of flavonoids on different compartments of immune system; function of innate and acquired immune cells, antibody production, cytokine secretion, and nuclear transcription factor activity. The entire data suggests that flavonoids can suppress pro-inflammatory immune response.
Flavonoids and Their Effects on Immune System
Flavonoids are considered as plant secondary metabolites that numerous pharmacological functions are attributed to them including antioxidant, anti-mutagenic, antibacterial, anti-angiogenic, anti-inflammatory, anti-allergic, enzyme modulation, and anti-cancer (37, 38). They are defined as phytochemicals which exist either as free aglycones or glycosidic conjugates (39). Flavonoids are polyphenolic with a wide range of structures (37). Based on this diversity, they are categorized mainly into flavones, flavanols, isoflavones, flavonols, flavanones, flavanonols, and chalcones (39). The diverse structures of flavonoids have resulted in many properties including anti-cancer and anti-inflammatory effects (37, 39, 40). Recently, it has been shown that flavonoids can affect immune system response and might have immune-modulator effects.
Quercetin
Quercetin is an abundant polyphenol in nature. It is an aglycone form of a number of other flavonoid glycosides such as rutin and quercitrin which can be found in variety of foods and plants, including apples, berries, Brassica vegetables, capers, grapes, onions, shallots, tea, and tomatoes, as well as different seeds, nuts, flowers, barks, and leaves (41, 42). Biosynthesis of Quercetin starts with phenylalanine in plants (41). It has been shown that Quercetin can affect lipid and glucose metabolisms by reducing oxidative stress and enhancing β-oxidation (43). In addition, some studies have examined the effects of Quercetin on the immune system. In an experimental study, dendritic cells (DCs) obtained from mouse bone marrow were treated by Quercetin. This flavonoid could effectively decrease the production of pro-inflammatory cytokines/chemokines and the expression levels of MHC class II and co-stimulatory molecules. These conditions inhibit the LPS-induced activation of DCs. Furthermore, endocytosis of DCs and the LPS-induced DC migration are decreased by Quercetin treatment (43). Quercetin also diminishes Ag-specific T cell activation by reducing the activity of LPS-stimulated DC's (44). In another experimental study, the effects of Quercetin-loaded micro-emulsion (QU-ME) were examined in a model of airway allergic inflammation. Mice received daily oral doses of QU-ME (3 or 10 mg/kg) over the course of 22 days. Compared with control group, QU-ME reduced inflammatory factors including IL-5 and IL-4. However, no change was observed in CCL11, IFN-gamma, and LTB levels. In addition, the nuclear transcription factor kappa B (NF-kappa B) activation, P-selectin expression and the mucus production in the lung were inhibited by oral treatment of QU-ME (41). In a study on peripheral blood mononuclear cells (PBMC) isolated from multiple sclerosis (MS) patients and from normal healthy subjects, Sternberg et al. showed that Quercetin decreased the proliferation of PBMC and modulated the level of IL-1beta and TNF-alpha released by PBMC in a dose-dependent manner. In this study, the modulation of TNF-alpha increased when Quercetin combined with human interferon-beta (IFN-beta) (45). In another mouse asthma model, Gupta et al. examined the potential of Quercetin to relieve asthma aggravation. This study revealed anti-asthmatic potential of Quercetin. Treatment with Quercetin significantly resulted in a reduction of specific immunoglobulin E (sIgE) production and anaphylaxis signs. Furthermore, Quercetin modulated the expression of Th2 cytokines including IL-4 and IL-5. These cytokines play a role in switching IgE class and suppressing the degranulation/secretion of different chemical mediators (PGD2, mMCPT-1 Cys-L, and TSLP) from activated mast cells (46). Other studies on the effects of Quercetin on the immune system showed inhibitory effects of Quercetin on cytotoxic lymphocyte function (47), IL-6 production in LPS-stimulated neutrophils (48), and anaphylactic contraction in guinea pig ileum smooth muscle (49). Moreover, it has been observed that Quercetin can regulate leukocyte biology with a stimulus-specific action and affects the balance of Th1/Th2 in a murine model of asthma (50, 51). Based on these findings, Quercetin has a potential role in modulating immune system responses.
Luteolin
Luteolin (3′,4′,5,7-tetrahydroxyflavone) and its glycosylated form luteolin-7-glucoside (L7G) belong to the flavone subclass of flavonoids and are among the most common flavonoids present in aromatic plants and other plant-based foods mostly consumed in the Mediterranean diet. Also, It is well distributed in many medicinal plants and some common fruits and vegetables including green leafy plants such as parsley, sweet peppers and celery (52–54). Although, glycosylated forms are the most common in nature it has been reported that Luteolin is absorbed in the aglycone form only. Apart from the antioxidant and anticarcinogenic properties, other features as anti-inflammatory and anti-allergic have also been reported for Luteolin (55–58). In an experimental study, treatment of asthmatic models of rats by Luteolin over 8 weeks resulted in a reduction in the total cell count, neutrophil count, eosinophil count and levels of IL-4 in comparison to a control group (59). In another mouse study, the effect of Luteolin on experimental autoimmune thyroiditis (EAT) showed that Luteolin treatment decreased lymphocytic infiltration and follicle destruction in thyroid glands. In addition, Luteolin inhibited the interferon-γ-induced increase in cyclooxygenase 2, and the secretion of the pro-inflammatory cytokine tumor necrosis factor-α (60). In an experimental study on human and murine auto-reactive T cells, Verbeek et al. reported that luteolin was a strong inhibitor for both murine and human T-cell responses. In this study, T-cell proliferation, and antigen-specific IFN-gamma production were significantly reduced in response to luteolin treatment. In addition, luteolin appears to be a strong inhibitor of mast cell histamine secretion (61). Moreover, anti-bacterial and anti-parasite properties of luteolin have been reported in recent studies (62, 63). The effects of Luteolin on the immune system and inflammation have also been assessed in vivo (64). Topical application of Reseda luteola extract, which is high in Luteolin, was as effective as hydrocortisone in decreasing inflammation following skin irradiation with Ultraviolet-B light (64). Overall, it seems that luteolin has beneficial effects on the modulation of immune responses. However, the mechanisms of this action might be variable and are not clearly known. Further studies are needed to shed light on these mechanisms.
Apigenin
Apigenin, or 40,5,7-trihydroxyflavone, is a common dietary flavonoid which is found in many fruits, vegetables, and herbs, such as orange, grapefruits, onion, wheat sprouts, parsley, celery, and chamomile tea (65, 66). Properties of Apigenin include anti-proliferative, anti-cancer antioxidant and anti-inflammatory activities (67). Apigenin exhibits anti-tumor effects by decelerating growth and inducing apoptosis through activation of pentose phosphate pathway-mediated NADPH generation in HepG2 human hepatoma cells, induction of apoptosis via the PI3K/AKT and ERK1/2 MAPK pathways, decreasing the viability, adhesion, and migration of cancer cells and modulating angiogenesis and metastasis (68). The effects of Apigenin on the immune system or modulation of immune responses have been assessed in recent studies. In an experimental study, Cardenas et al. reported Apigenin significantly modulated NF-κB activity in the lungs. This finding showed the ability of Apigenin to exert immune-regulatory activity in an organ-specific manner (69). In another study on models of rat colitis, administration of apigenin K, a soluble form of Apigenin, resulted in reduced inflammation as well as lower colonic damage scores and colonic weight/length ratio (68). In addition, administration of Apigenin K could normalize the expression of some colonic inflammatory markers [e.g., TNF-α, transforming growth factor-β, IL-6, intercellular adhesion molecule 1 or chemokine (C-C motif) ligand 2] (70). In another experimental study on asthma in mice, Li et al. reported that Apigenin administration (5 mg/kg or 10 mg/kg) inhibited OVA-induced increases in eosinophil count and also in Th17 cells. Therefore, Apigenin administration might effectively ameliorate the progression of asthma (71). Furthermore, it has been shown that Apigenin in combination with Quercetin and Luteolin has a protective effect on pancreatic beta-cells injured by cytokines during inflammation (72). The inhibitory effect of Apigenin on mast cell secretion has also been observed in recent studies (51). Apigenin combined with Luteolin are strong inhibitors for murine and human T-cell responses, in particular auto-reactive T cells (61). In sum, it seems that apigenin can be considered as a modulator of immune system.
Fisetin
Fisetin (3, 3′, 4′, 7-tetrahydroxy flavone) is a type of flavonoid commonly found in plants like the smoke tree and numerous types of fruits and vegetables including strawberries, grapes, onions, and cucumbers (51, 73–75). Some properties of Fisetin include anti-cancer, anti-angiogenic, neuroprotective, neurotrophic, antioxidant, anti-inflammatory, anti-proliferative, and apoptotic effects (76). However, the powerful antioxidant property of Fisetin is due to the presence of phenolic hydroxyl group in the flavonoid structure (77). A few studies have examined the effects of Fisetin on the immune system. Song et al. assessed the immunosuppressive effects of Fisetin against T-cell activation in vitro and in vivo. Findings of this study showed that Fisetin significantly inhibited Th1 and Th2 cytokine production, cell cycle and the ratio of T CD4+/CD8+ cells in vitro. Furthermore, Fisetin suppressed mouse T lymphocytes through the suppression of nuclear factor kappa B activation and nuclear factor of activated T cells signaling in a dose-dependent manner. The in vivo finding showed that Fisetin also inhibited delayed-type hypersensitivity reactions in mice (76). One study on the effects of Fisetin on human mast cells (HMC-1) showed that Fisetin could down-regulate mast cell activation (73). In addition, two studies have reported that the anti-asthma properties of Fisetin are due to reduction of Th2 response as well as suppression of NF-κB (75, 78). In an experimental study using a mouse model of atopic dermatitis (AD), Kim et al. investigated the effects of Fisetin on AD-like clinical symptoms. They showed that Fisetin administration inhibited the infiltration of inflammatory cells including eosinophils, mast cells, and T CD4+ and T CD8+ cells. Furthermore, Fisetin was able to suppress the expression of cytokines and chemokines associated with dermal infiltrates in AD-like skin lesions. In a dose-dependent manner, Fisetin decreased the T CD4+ cell-induced production of interferon-gamma and interleukin-4, and in contrast, increased the anti-inflammatory cytokine such as interleukin-10 (79). Based on these findings, Fisetin is able to significantly affect immune system responses.
As mentioned, T CD4 + cells play a central role in orchestrating immune response. Moreover, while regulatory effects of flavonoids on T CD4+ have been observed, the exact mechanisms are under investigation. Here we elaborate why metabolism can play an important role in Th cells fate. What happens to metabolic machinery of Th cells when they get activated? Studies show that metabolic status of naive and activated Th cells is different, because of their different energetic demands.
Metabolism of Th cells
Resting and naive Th cells don't need great amount of energy. Hence, their metabolic status is generally at baseline. These cells use autophagy and catabolism of fatty acids to supply their housekeeping demands (80). When these cells are activated, they undergo rapid and excessive clonal expansion. Activated Th cells use anabolism to synthesize different types of essential macromolecules for proliferation, which is highly energetically costly. In fact, activated Th cells switch from catabolism to anabolism, a process known as metabolic reprogramming (80).
The hallmark of Th cell metabolic reprogramming is the use of glycolysis in the presence of sufficient oxygen (81). If following activation, Th cells are not able to induce metabolic reprogramming, they become anergic, and are not able to respond to pathogens (82). Therefore, the metabolism of these cells plays a critical role in Th cell activation. However, the main question is why activated Th cells use glycolysis instead of TCA for ATP production? Why do they prefer to use a low yield pathway (2 ATP) instead of high-yield cycle (32 ATP)? Although clonal expansion requires energy, it also relies on protein, DNA, and lipid synthesis for cell size augmentation, for which glycolysis provides the energetic drive. Otto Warburg in 1931 found that cancer cells grow in acidic conditions, as they use glycolysis and produce lactic acid (Figure 2).
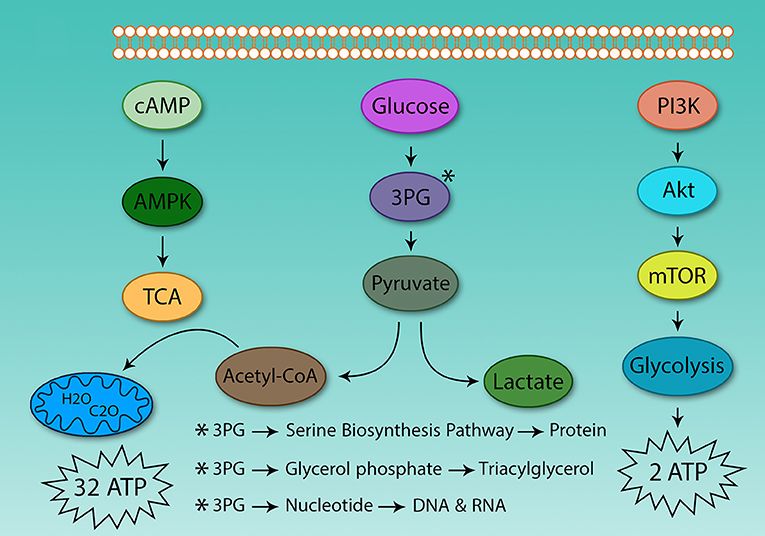
Figure 2. Differences between glycolysis and the tricarboxylic acid cycle. The energy obtained from glucose oxidation and glycolysis is very different, even in oxygen-activated Th cells which use glycolysis. The same situation also is observed in cancer cells. Because of this, the tumor environment is acidic. Activated Th and cancer cells use glycolysis to supply protein, DNA, and lipids to support proliferation.
Below, we discuss how metabolism and immune signals are linked together. Some immunological signals are integrated into metabolic pathways. One of the most important pathways which plays key role in Th cell differentiation is PI3K/Akt/mTOR pathway. The activation status of this pathway is affected by different immunologic signals. PI3K/Akt/mTOR pathway promotes glycolysis and it is necessary to be increased as it activates glycolysis pathway significantly and also increases the expression of a range of proteins including enzymes and transporters. The PI3K/Akt/mTOR pathway mediates up-regulation of glycolysis and prepares cells for proliferation.
mTOR; Mechanistic Target of Rapamycin
mTOR is a highly conserved molecule in mammalian cells (83), coded as a unique single gene but translated into two different proteins, mTOR complex 1 and 2 (mTORC1 and mTORC2) (82). These two complexes have different functions. Activation of mTORC1 results in enhancement of translation, cell size and lipogenesis in white fat tissue, whilst mTORC2 activation promotes glucose uptake in tissue, enhancement of glucose synthesis and reduction of gluconeogenesis in liver (83).
Levels of several metabolites [amino acids (83, 84) and glucose], growth factors, energy level (cytosolic AMP:ATP ratio), stress, and immunological signals [CD28, IL-2 (82)] regulate mTOR function (Figure 3) (83). At the same time, mTOR controls expression of several nutrition transporters (80). Different cytokines also regulate mTOR activity; IL-7 activates mTOR and inhibits autophagy, IL-4 promotes proliferation through mTOR activation and decrease apoptosis, IL-12 and IFN-γ also promote continuous mTOR activity (82).
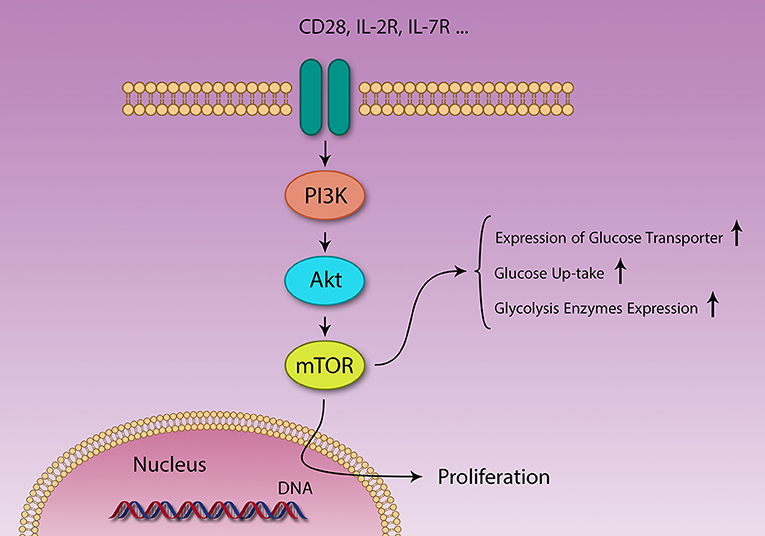
Figure 3. Different signaling pathways are activated by different stimuli. PI3K/Akt/mTOR and AMPK pathways act in contrasting fashion in metabolism and immunity. Several factors like IL-2 and CD28 signaling and growth factors activate the PI3K/Akt/mTOR pathway, resulting in survival, and proliferation of different cells. Naïve T cells use lipid β-oxidation to supply their low demands, but after activation, these cells generate a large amount of energy using glycolysis, through changing their metabolic machinery.
From the immunological point of view, two signals are needed for successful activation of Th cells. The first signal is TCR recognition of antigens and the second is additional signals produced by co-stimulator molecules. If the first signal is not accompanied by the second signal, Th cells will not be able to react (anergy). Anergic T cells are metabolically oxidative. They use oxidative phosphorylation to supply their energy demands (84) and it seems that inhibition of glycolysis is sufficient for induction of anergy. For example, 2-DG which blocks glycolysis, inhibits Th17 differentiation even under Th17-polarizing conditions (13, 84). Interestingly, mTOR inhibition using Rapamycin promotes the induction of anergy in Th cells, even in the presence of second signal (82, 85). This phenomenon is explained by the fact that mTOR is downstream of the second signaling pathway, hence its inhibition attenuates the up-stream signal.
All reports suggest that mTOR is a mediator in T cells, between immunologic signals and metabolic demands. Further studies show that mTORC1 promotes Th1 and Th17 differentiation and mTORC2 induces Th2 differentiation. Suppression of both complexes results in Treg induction. The effect of Rapamycin as an antibiotic and immunosuppressive medicine, which blocks mTOR activation, sheds light on an incredible connection between metabolism and immune function. Earlier studies showed that using Rapamycin and knocking out mTORC1 have similar effects (85). However, the exact mechanism of mTOR in determining Th cell fate is not well understood. It seems that mTOR plays an important role in metabolic reprograming of activated Th cells (21).
In addition to mTOR, the protein kinase AMPK also plays a critical role in metabolism and differentiation of Th cells. Similar to mTOR, AMPK is highly conserved in eukaryotic cells as well (85). AMPK and mTOR play important roles in metabolism and immunity. Their function in immunity is also against together (15, 86). By mTOR activation, glucose metabolism is promoted, especially glycolysis, and mTOR suppression by Rapamycin results in the suppression of glycolysis and corresponding increase in fatty acid oxidation (15, 36, 85). Previous studies have shown that induction of AMPK activation has similar results to the suppression of mTOR (15, 36, 85), by activation of AMPK consequently fatty acid oxidation promotes and mTOR function is suppressed. Induction of fatty acid oxidation through mTOR suppression and/or AMPK activation in activated Th cells, as mentioned above, results in Treg differentiation (36, 85).
Rapamycin inhibits mTORC1 and mTORC2 function and induces Treg as well. Although the inhibitory effects of Rapamycin on mTORC2 was unclear for several years, Powell, and Delgoffe in their investigation in 2010 indicated that specific doses of Rapamycin might inhibit mTORC1 and mTORC2 in T-cells (82). They claimed that Rapamycin might also promote induction of Treg cells (87).
One of the questions that arises is the possibility of induction of Th subsets in vitro and in vivo through specific metabolites in precise doses by activation and suppression of mTOR. Some previous investigations have shown this effect (88, 89). These studies help us to understand how our diet influences immune system function. Furthermore, they provide explanations for autoimmune diseases and possible key points to treat them. For example, short chain fatty acids are able to induce intestinal Treg in mice. The imbalance of Th17/Treg is responsible for several diseases, like inflammatory bowel diseases (IBD) (16).
Is it possible that accumulation of metabolites in the cells and excessive dietary uptake also modulate mTOR activity? Does this modulation of mTOR activity result in changes in the fate of immune responses? Both answers are yes. Not only specific metabolites like glucose (36, 90), NaCl (3), fatty acids (1, 16, 17, 91), amino acids (21), all-trans retinoic acid (18, 92), cholesterol (93, 94) and vitamins (19) affect mTOR function, but also mTOR regulates expression of metabolite transporters (84). For example, after Th cell activation, since glucose is the main source of energy for these cells, mTOR activation results in up-regulation of glucose transporters. Additionally, the presence of cytosolic leucine and glutamine is essential for mTOR function (21).
The metabolic reprogramming in activated Th cells is the same as that of cancer cells (36, 82). This similarity may seem logical, as both cells must undergo rapid proliferation and this process is highly demanding for both energy and metabolic substrates. The difference between Th cells clonal expansion and cancer cells growth is that cancer cells proliferate uncontrollably. Some molecules like mTOR play a crucial role both in the metabolism of cancer cells and activated Th cells, which has been investigated earlier (Figure 4) (82).
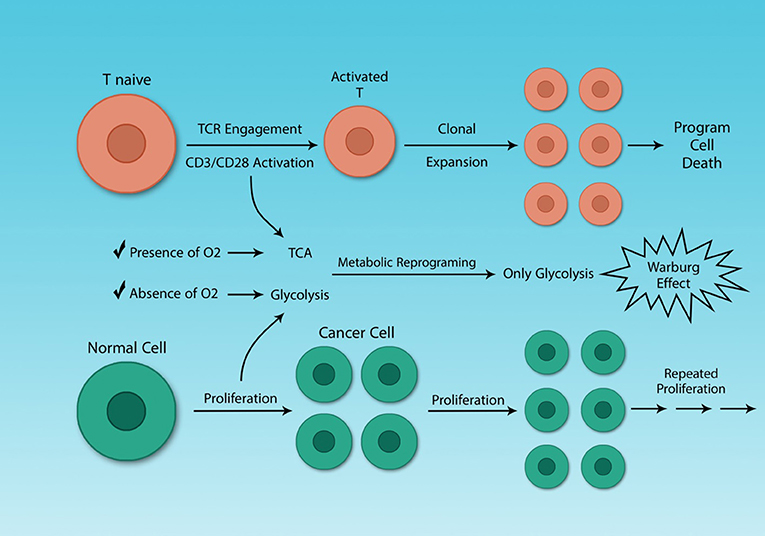
Figure 4. Cancer cells and activated Th cells use similar metabolic programs. Normal and cancer cells use distinct metabolic pathways, because of their different energy demands. Resting and activated Th cells also have different energy demands, because activated Th cells need to growth rapidly. Although cancer and activated Th cells are different, in general, they share a similar feature, their metabolism. The Warburg effect characterizes both cell types.
Effects of Flavonoids on PI3K/Akt/mTOR Axis Based on Studies in Cancer Cells
As mentioned, cancer cells and activated Th cells have similar metabolisms. Both use glycolysis to supply their demands. Although previous studies about the effects of flavonoids on the immune system might provide some new information for nutritionists, they are incompletely understood from molecular immunology point of view. In addition, findings from these studies are heterogeneous, for instance regarding the effect of flavonoids on some inflammatory cytokines like TNF-α (23, 95–97). Detailed data are available about the impacts of different flavonoids on cancer cell proliferation. Because of metabolic similarity between cancer cells and activated Th cells and lack of sufficient data about flavonoids effects on Th cells or the PI3K/Akt/mTOR axis, here we will focus on results from the cancer field.
Flavonoids also have specific effects on this axis in cancer cells (Table 1). Curcumin, a yellow-pigment substance and component of turmeric, significantly increases mTOR suppression and induces apoptosis in renal cancer cells (105). Curcumin is also able to arrest melanoma cells in G2/M and induce autophagy in these cells. In vitro investigations showed that Curcumin inhibits Akt, mTOR, and P70S6K activity. Moreover, Curcumin was shown to suppress tumors in BALB/c mice, though this effect was not significant (106). In breast and prostate cancer cell lines, Curcumin inhibits Akt and mTOR function even in the presence of EGF, a ligand of the EGF receptor. The Akt/PI3K/mTOR axis is one of the most important down-stream signaling pathways after EGFR activation (107). The suppression of the Akt/PI3K/mTOR axis, even in the presence of EGF, could be a promising finding in the field of cancer therapy research. Both ligand-dependent and independent activation of EGFR can cause resistance to current therapies, a major problem in cancer treatment (108). All results confirm that Curcumin induces apoptosis and inhibits tumor cell growth and it is also able to block metastasis (101).
Another attractive polyphenol, fisetin inhibits mTOR complexes, PI3K (25, 98), and Akt activity in prostate cancer cell lines (25, 98, 109). It also activates AMPK and PTEN in non-small lung cancer cells (25, 98). As mentioned before, AMPK and mTOR play contrary roles in metabolism. In cancer cells, AMPK activation and mTOR suppression result in both survival and proliferation failure (110).
Quercetin inhibits the Akt/PI3K/mTOR and Wnt/catenin pathways in lymphoma cells (111). mTOR inhibition and induction in apoptosis after Quercetin treatment in Burkitt's lymphoma cells has been observed (112). In a cervix cancer cell line, G2/M arrest was observed in the cell cycle. It also triggers release of cytochrome-C which is an indicator of apoptosis (113).
Other studies have shown that pomegranate polyphenols not only suppress Akt and mTOR expression and function, but also reduce IGF expression in colon cancer cells (100). In 2015 Zhang et al. showed that Aqueous Allspice Extract (AAE), which contains many different flavonoids, was able to activate autophagy signaling in breast cancer cells and induce cell death. AAE acts synergistically with Rapamycin and enhances autophagy and cell death. Akt and mTOR signaling are suppressed by AAE (114).
Another similar pathway that is active in tumor cells (102) as well as in Th cells (115) is aryl hydrocarbon receptor (AhR). It plays a central role in the differentiation of Th cells. If AhR is activated by dioxin or kynurenine, Treg cells differentiate in vivo and in vitro, whilst its other ligand, FICZ, induces the Th17 subset (115). In prostate tumor cells, AhR shows aberrant expression and its deletion or inhibition results in the inhibition of tumorgenesis and tumor growth. By suppression of AhR, G0/G1 cell cycle arrest occurs in prostate cancer cells. It can be concluded that AhR is necessary for induction of cell cycle arrest and apoptosis by Quercetin in prostate cell line (102). However, the exact mechanism of involvement of AhR in cancer cell apoptosis mediated by Quercetin is not well understood yet. Previous studies show that AhR can activate the Akt/PI3K/mTOR pathway, and AhR inhibition results in low PI3K activity and also restores sensitivity to apoptosis in the mouse hepatoma cell line (116).
Because of similar metabolisms in active Th cells and cancer cells, described in detail above, it is expected that the polyphenols can suppress mTOR activity in Th cells. Hence, it can be concluded that polyphenols also induce Treg cells and these differentiated regulatory cells suppress unwanted immune response against self-antigens.
Conclusion
Before activation of naïve Th cells, they are catabolic. However, after activation and differentiation into effector subsets they become anabolic. If T effector cells are not able to change their metabolic status, they will be unable to respond to pathogens. The PI3k/Akt/mTOR pathway is up-regulated after Th activation, and its suppression results in anergy. By considering the important role of metabolism in the differentiation of Th cells, it seems reasonable that accumulation of specific metabolites may induce Th subsets. Indeed, flavonoids have been investigated for their effects on immune system. Flavonoids are able to modulate immune response, though the exact molecular mechanisms involved in these changes are not well understood. Flavonoids also have anti-proliferative effects on cancer cells through suppression of the PI3k/Akt/mTOR pathway in these cells. As cancer cells and activated Th cells use glycolysis and the PI3k/ Akt/mTOR pathway plays a crucial role in both cells, it can be concluded that flavonoids also suppress this pathway in Th cells. By suppression of the PI3k/AKT/mTOR pathway, T effector differentiation is reduced and T regulatory cells are induced.
Author Contributions
AH, OS, AN, and AE contributed to design, conception and manuscript drafting. AH and OS contributed to literature search and data extraction. SS and GB contributed to manuscript drafting. All authors approved the final version of manuscript.
Conflict of Interest Statement
The authors declare that the research was conducted in the absence of any commercial or financial relationships that could be construed as a potential conflict of interest.
Abbreviations
AD, Atopic Dermatitis; AhR, Aryl Hydrocarbon Receptor; AMPK, AMP-activated, protein kinase; EGF, Epidermal Growth Factor; FICZ, 5,11-Dihydroindolo 3,2 bcarbazole-6-carboxaldehyde; IFN, Interferon; IL, Interleukin; LPS, Lipopolysaccharide; mTORC1&2, Mechanistic Target of Rapamycin Complex 1&2; PTEN, Phosphatase and Tensin homolog; T CD4+ or Th, T helper; T CD8+ or Tc, T cytotoxic; Teff, T effector cell; Treg, T regulatory cell; TCA, Tricarboxylic Acid; TNF, Tumor Necrosis Factor; 2-DG, 2-deoxyglucose.
References
1. Fontenelle B, Gilbert K. n-Butyrate anergized effector CD4+ T cells independent of regulatory T cell generation or activity. Scand J Immunol. (2012) 76:457–63. doi: 10.1111/j.1365-3083.2012.02740.x
2. Narusyte J, Neiderhiser JM, D'onofrio BM, Reiss D, Spotts EL, Ganiban J, et al. Testing different types of genotype-environment correlation: an extended children-of-twins model. Dev Psychol. (2008) 44:1591. doi: 10.1037/a0013911
3. Kleinewietfeld M, Manzel A, Titze J, Kvakan H, Yosef N, Linker RA, et al. Sodium chloride drives autoimmune disease by the induction of pathogenic TH17 cells. Nature (2013) 496:518–22. doi: 10.1038/nature11868
4. White JH. Vitamin D metabolism and signaling in the immune system. Rev Endocr Metab Disords. (2012) 13:21–9. doi: 10.1007/s11154-011-9195-z
5. Berger H, Végran F, Chikh M, Gilardi F, Ladoire S, Bugaut H, et al. SOCS3 transactivation by PPARγ prevents IL-17–driven cancer growth. Cancer Res. (2013) 73:3578–90. doi: 10.1158/0008-5472.CAN-12-4018
6. Monk JM, Kim W, Callaway E, Turk HF, Foreman JE, Peters JM, et al. Immunomodulatory action of dietary fish oil and targeted deletion of intestinal epithelial cell PPARδ in inflammation-induced colon carcinogenesis. Am J Physiol Gastrointest Liver Physiol. (2012) 302:G153–67. doi: 10.1152/ajpgi.00315.2011
7. Kong W, Yen JH, Ganea D. Docosahexaenoic acid prevents dendritic cell maturation, inhibits antigen-specific Th1/Th17 differentiation and suppresses experimental autoimmune encephalomyelitis. Brain Behav Immun. (2011) 25:872–82. doi: 10.1016/j.bbi.2010.09.012
8. Jaudszus A, Gruen M, Watzl B, Ness C, Roth A, Lochner A, et al. Evaluation of suppressive and pro-resolving effects of EPA and DHA in human primary monocytes and T-helper cells. J Lipid Res. (2013) 54:923–35. doi: 10.1194/jlr.P031260
9. Baranowski M, Enns J, Blewett H, Yakandawala U, Zahradka P, Taylor CG. Dietary flaxseed oil reduces adipocyte size, adipose monocyte chemoattractant protein-1 levels and T-cell infiltration in obese, insulin-resistant rats. Cytokine (2012) 59:382–91. doi: 10.1016/j.cyto.2012.04.004
10. Araki K, Ellebedy AH, Ahmed R. TOR in the immune system. Curr Opin Cell Biol. (2011) 23:707–15. doi: 10.1016/j.ceb.2011.08.006
11. Odegaard JI, Chawla A. The immune system as a sensor of the metabolic state. Immunity (2013) 38:644–54. doi: 10.1016/j.immuni.2013.04.001
12. Zhu J, Yamane H, Paul WE. Differentiation of effector CD4 T cell populations. Annu Rev Immunol. (2009) 28:445–89. doi: 10.1146/annurev-immunol-030409-101212
13. Shi LZ, Wang R, Huang G, Vogel P, Neale G, Green DR, et al. HIF1α-dependent glycolytic pathway orchestrates a metabolic checkpoint for the differentiation of TH17 and Treg cells. J Exp Med. (2011) 208:1367–76. doi: 10.1084/jem.20110278
14. Dang EV, Barbi J, Yang H-Y, Jinasena D, Yu H, Zheng Y, et al. Control of T H 17/T reg balance by hypoxia-inducible factor 1. Cell (2011) 146:772–84. doi: 10.1016/j.cell.2011.07.033
15. Michalek RD, Gerriets VA, Jacobs SR, Macintyre AN, MacIver NJ, Mason EF, et al. Cutting edge: distinct glycolytic and lipid oxidative metabolic programs are essential for effector and regulatory CD4+ T cell subsets. J Immunol. (2011) 186:3299–303. doi: 10.4049/jimmunol.1003613
16. Arpaia N, Campbell C, Fan X, Dikiy S, van der Veeken J, Liu H, et al. Metabolites produced by commensal bacteria promote peripheral regulatory T-cell generation. Nature (2013) 504:451–5. doi: 10.1038/nature12726
17. Woodworth HL, McCaskey SJ, Duriancik DM, Clinthorne JF, Langohr IM, Gardner EM, et al. Dietary fish oil alters T lymphocyte cell populations and exacerbates disease in a mouse model of inflammatory colitis. Cancer Res. (2010) 70:7960–9. doi: 10.1158/0008-5472.CAN-10-1396
18. Benson MJ, Pino-Lagos K, Rosemblatt M, Noelle RJ. All-trans retinoic acid mediates enhanced T reg cell growth, differentiation, and gut homing in the face of high levels of co-stimulation. J Exp Med. (2007) 204:1765–74. doi: 10.1084/jem.20070719
19. Hart PH, Gorman S, Finlay-Jones JJ. Modulation of the immune system by UV radiation: more than just the effects of vitamin D? Nat Rev Immunol. (2011) 11:584–96. doi: 10.1038/nri3045
20. Raverdeau M, Mills KH. Modulation of T cell and innate immune responses by retinoic acid. J Immunol. (2014) 192:2953–8. doi: 10.4049/jimmunol.1303245
21. Sinclair LV, Rolf J, Emslie E, Shi Y-B, Taylor PM, Cantrell DA. Control of amino-acid transport by antigen receptors coordinates the metabolic reprogramming essential for T cell differentiation. Nat immunol. (2013) 14:500–8. doi: 10.1038/ni.2556
22. Coomes SM, Pelly VS, Wilson MS. Plasticity within the αβ+ CD4+ T-cell lineage: when, how and what for? Open Biol. (2013) 3:120157. doi: 10.1098/rsob.120157
23. Hämäläinen M, Nieminen R, Vuorela P, Heinonen M, Moilanen E. Anti-inflammatory effects of flavonoids: genistein, kaempferol, quercetin, and daidzein inhibit STAT-1 and NF-κB activations, whereas flavone, isorhamnetin, naringenin, and pelargonidin inhibit only NF-κB activation along with their inhibitory effect on iNOS expression and NO production in activated macrophages. Mediators Inflamm. (2007) 2007:45673. doi: 10.1155/2007/45673.
24. Cady RJ, Durham PL. Cocoa-enriched diets enhance expression of phosphatases and decrease expression of inflammatory molecules in trigeminal ganglion neurons. Brain Res. (2010) 1323:18–32. doi: 10.1016/j.brainres.2010.01.081
25. Khan N, Afaq F, Khusro FH, Adhami VM, Suh Y, Mukhtar H. Dual inhibition of PI3K/AKT and mTOR signaling in human non-small cell lung cancer cells by a dietary flavonoid fisetin. Int J Cancer (2012) 130:1695. doi: 10.1002/ijc.26178
26. Abril-Gil M, Massot-Cladera M, Perez-Cano FJ, Castellote C, Franch A, Castell M. A diet enriched with cocoa prevents IgE synthesis in a rat allergy model. Pharmacol Res. (2012) 65:603–8. doi: 10.1016/j.phrs.2012.02.001
27. Akiyama H, Sato Y, Watanabe T, Nagaoka MH, Yoshioka Y, Shoji T, et al. Dietary unripe apple polyphenol inhibits the development of food allergies in murine models. FEBS Lett. (2005) 579:4485–91. doi: 10.1016/j.febslet.2005.07.019
28. Ikejiri A, Nagai S, Goda N, Kurebayashi Y, Osada-Oka M, Takubo K, et al. Dynamic regulation of Th17 differentiation by oxygen concentrations. Int Immunol. (2012) 24:137–46. doi: 10.1093/intimm/dxr111
29. Kim JS, Sklarz T, Banks LB, Gohil M, Waickman AT, Skuli N, et al. Natural and inducible TH17 cells are regulated differently by Akt and mTOR pathways. Nat Immunol. (2013) 14:611–8. doi: 10.1038/ni.2607
30. Tse K, Tse H, Sidney J, Sette A, Ley K. T cells in atherosclerosis. Int Immunol. (2013) 25:615–22. doi: 10.1093/intimm/dxt043
31. O'Shea JJ, Paul WE. Mechanisms underlying lineage commitment and plasticity of helper CD4+ T cells. Science (2010) 327:1098–102. doi: 10.1126/science.1178334.
32. Riley JL, June CH, Blazar BR. Human T regulatory cell therapy: take a billion or so and call me in the morning. Immunity (2009) 30:656–65. doi: 10.1016/j.immuni.2009.04.006
33. Kim YC, Bhairavabhotla R, Yoon J, Golding A, Thornton AM, Tran DQ, et al. Oligodeoxynucleotides stabilize Helios-expressing Foxp3+ human T regulatory cells during in vitro expansion. Blood (2012) 119:2810–8. doi: 10.1182/blood-2011-09-377895
34. Takvorian S, Merola J, Costenbader K. Cigarette smoking, alcohol consumption and risk of systemic lupus erythematosus. Lupus (2014) 23:537–44. doi: 10.1177/0961203313501400
35. Andersson J, Tran DQ, Pesu M, Davidson TS, Ramsey H, O'Shea JJ, et al. CD4+ FoxP3+ regulatory T cells confer infectious tolerance in a TGF-beta-dependent manner. J Exp Med. (2008) 205:1975–81. doi: 10.1084/jem.20080308
36. Gerriets VA, Rathmell JC. Metabolic pathways in T cell fate and function. Trends Immunol. (2012) 33:168–73. doi: 10.1016/j.it.2012.01.010
37. Ravishankar D, Rajora AK, Greco F, Osborn HM. Flavonoids as prospective compounds for anti-cancer therapy. Int J Biochem Cell Biol. (2013) 45:2821–31. doi: 10.1016/j.biocel.2013.10.004
38. Hodek P, Trefil P, Stiborová M. Flavonoids-potent and versatile biologically active compounds interacting with cytochromes P450. Chem Biol Interact. (2002) 139:1–21. doi: 10.1016/S0009-2797(01)00285-X
39. Middleton E, Kandaswami C, Theoharides TC. The effects of plant flavonoids on mammalian cells: implications for inflammation, heart disease, and cancer. Pharmacol Rev. (2000) 52:673–751.
40. Cardenas C, Quesada AR, Medina MA. Anti-angiogenic and anti-inflammatory properties of kahweol, a coffee diterpene. PLoS ONE (2011) 6:e23407. doi: 10.1371/journal.pone.0023407
41. Rogerio AP, Dora CL, Andrade EL, Chaves JS, Silva LF, Lemos-Senna E, et al. Anti-inflammatory effect of quercetin-loaded microemulsion in the airways allergic inflammatory model in mice. Pharmacol Res. (2010) 61:288–97. doi: 10.1016/j.phrs.2009.10.005
42. Patil BS, Jayaprakasha G, Chidambara Murthy K, Vikram A. Bioactive compounds: historical perspectives, opportunities, and challenges. J Agric Food Chem. (2009) 57:8142–60. doi: 10.1021/jf9000132
43. Sun X, Yamasaki M, Katsube T, Shiwaku K. Effects of quercetin derivatives from mulberry leaves: improved gene expression related hepatic lipid and glucose metabolism in short-term high-fat fed mice. Nut Res Practice (2015) 9:137–43. doi: 10.4162/nrp.2015.9.2.137
44. Huang R-Y, Yu Y-L, Cheng W-C, OuYang C-N, Fu E, Chu C-L. Immunosuppressive effect of quercetin on dendritic cell activation and function. J Immunol. (2010) 184:6815–21. doi: 10.4049/jimmunol.0903991
45. Sternberg Z, Chadha K, Lieberman A, Hojnacki D, Drake A, Zamboni P, et al. Quercetin and interferon-β modulate immune response (s) in peripheral blood mononuclear cells isolated from multiple sclerosis patients. J Neuroimmunol. (2008) 205:142–7. doi: 10.1016/j.jneuroim.2008.09.008
46. Gupta K, Kumar S, Gupta RK, Sharma A, Verma AK, Stalin K, et al. Reversion of asthmatic complications and mast cell signalling pathways in BALB/c mice model using quercetin nanocrystals. J Biomed Nanotechnol. (2016) 12:717–31. doi: 10.1166/jbn.2016.2197
47. Schwartz A, Sutton SL, Middleton E. Quercetin inhibition of the induction and function of cytotoxic T lymphocytes. Immunopharmacology (1982) 4:125–38. doi: 10.1016/0162-3109(82)90015-7
48. Liu J, Li X, Yue Y, Li J, He T, He Y. The inhibitory effect of quercetin on IL-6 production by LPS-stimulated neutrophils. Cell Mol Immunol. (2005) 2:455–60.
49. Fanning M, Macander P, Drzewiecki G, Middleton Jr E. Quercetin inhibits anaphylactic contraction of guinea pig ileum smooth muscle. Int Arch Allergy Immunol. (1983) 71:371–3. doi: 10.1159/000233423
50. Park HJ, Lee C-M, Jung ID, Lee JS, Jeong YI, Chang JH, et al. Quercetin regulates Th1/Th2 balance in a murine model of asthma. Int Immunopharmacol. (2009) 9:261–7. doi: 10.1016/j.intimp.2008.10.021
51. Chirumbolo S. The role of quercetin, flavonols and flavones in modulating inflammatory cell function. Inflamm Allergy Drug Targets (2010) 9:263–85. doi: 10.2174/187152810793358741
52. Arts IC, Hollman PC. Polyphenols and disease risk in epidemiologic studies. Am J Clin Nutr. (2005) 81:317S−25S. doi: 10.1093/ajcn/81.1.317S
53. Maron DJ. Flavonoids for reduction of atherosclerotic risk. Curr Atheroscler Rep. (2004) 6:73–8. doi: 10.1007/s11883-004-0119-1
54. Mennen LI, Sapinho D, de Bree A, Arnault N, Bertrais S, Galan P, et al. Consumption of foods rich in flavonoids is related to a decreased cardiovascular risk in apparently healthy French women. J Nutr. (2004) 134:923–6. doi: 10.1093/jn/134.4.923
55. Nazari QA, Kume T, Takada-Takatori Y, Izumi Y, Akaike A. Protective effect of luteolin on an oxidative-stress model induced by microinjection of sodium nitroprusside in mice. J Pharmacol Sci. (2013) 122:109–17. doi: 10.1254/jphs.13019FP
56. Kanazawa K, Uehara M, Yanagitani H, Hashimoto T. Bioavailable flavonoids to suppress the formation of 8-OHdG in HepG2 cells. Arch Biochem Biophys. (2006) 455:197–203. doi: 10.1016/j.abb.2006.09.003
57. Si H, Wyeth RP, Liu D. The flavonoid luteolin induces nitric oxide production and arterial relaxation. Eur J Nutr. (2014) 53:269–75. doi: 10.1007/s00394-013-0525-7
58. Jang S, Dilger RN, Johnson RW. Luteolin inhibits microglia and alters hippocampal-dependent spatial working memory in aged mice. J Nutr. (2010) 140:1892–8. doi: 10.3945/jn.110.123273
59. Zeng W, Wu C, Dai Y. Regulatory effects of luteolin on airway inflammation in asthmatic rats. Zhonghua yi xue za zhi (2014) 94:2535–9.
60. Xia N, Chen G, Liu M, Ye X, Pan Y, Ge J, et al. Anti-inflammatory effects of luteolin on experimental autoimmune thyroiditis in mice. Exp Ther Med. (2016) 12:4049–54. doi: 10.3892/etm.2016.3854
61. Verbeek R, Plomp AC, van Tol EA, van Noort JM. The flavones luteolin and apigenin inhibit in vitro antigen-specific proliferation and interferon-gamma production by murine and human autoimmune T cells. Biochem Pharmacol. (2004) 68:621–9. doi: 10.1016/j.bcp.2004.05.012
62. Jiang H, Hu J, Zhan W, Liu X. Screening for fractions of oxytropis falcata bunge with antibacterial activity. Nat Prod Res. (2009) 23:953–9. doi: 10.1080/14786410902906934
63. Marín C, Boutaleb-Charki S, Díaz JsG, Huertas O, Rosales MaJ, Pérez-Cordon G, et al. Antileishmaniasis activity of flavonoids from Consolida oliveriana. J Nat Prod. (2009) 72:1069–74. doi: 10.1021/np8008122
64. Casetti F, Jung W, Wölfle U, Reuter J, Neumann K, Gilb B, et al. Topical application of solubilized Reseda luteola extract reduces ultraviolet B-induced inflammation in vivo. J Photochem Photobiol B Biol. (2009) 96:260–5. doi: 10.1016/j.jphotobiol.2009.07.003
65. Lefort ÉC, Blay J. Apigenin and its impact on gastrointestinal cancers. Mol Nutr Food Res. (2013) 57:126–44. doi: 10.1002/mnfr.201200424
66. Shukla S, Gupta S. Apigenin: a promising molecule for cancer prevention. Pharm Res. (2010) 27:962–78. doi: 10.1007/s11095-010-0089-7
67. Liu H-J, Fan Y-L, Liao H-H, Liu Y, Chen S, Ma Z-G, et al. Apigenin alleviates STZ-induced diabetic cardiomyopathy. Mol Cell Biochem. (2017) 428:9–21. doi: 10.1007/s11010-016-2913-9
68. Wang J, Li T, Zang L, Pan X, Wang S, Wu Y, et al. Apigenin inhibits human SW620 cell growth by targeting polyamine catabolism. Evid Based Complement Alternat Med. (2017) 2017:3684581. doi: 10.1155/2017/3684581
69. Cardenas H, Arango D, Nicholas C, Duarte S, Nuovo GJ, He W, et al. Dietary apigenin exerts immune-regulatory activity in vivo by reducing NF-κB activity, halting leukocyte infiltration and restoring normal metabolic function. Int J Mol Sci. (2016) 17:323. doi: 10.3390/ijms17030323
70. Mascaraque C, González R, Suárez MD, Zarzuelo A, de Medina FS, Martínez-Augustin O. Intestinal anti-inflammatory activity of apigenin K in two rat colitis models induced by trinitrobenzenesulfonic acid and dextran sulphate sodium. Br J Nutr. (2015) 113:618–26. doi: 10.1017/S0007114514004292
71. Li J, Zhang B. Apigenin protects ovalbumin-induced asthma through the regulation of Th17 cells. Fitoterapia (2013) 91:298–304. doi: 10.1016/j.fitote.2013.09.009
72. Kim E-K, Kwon K-B, Song M-Y, Han M-J, Lee J-H, Lee Y-R, et al. Flavonoids protect against cytokine-induced pancreatic β-cell damage through suppression of nuclear factor κB activation. Pancreas (2007) 35:e1–9. doi: 10.1097/mpa.0b013e31811ed0d2
73. Park H-H, Lee S, Oh J-M, Lee M-S, Yoon K-H, Park BH, et al. Anti-inflammatory activity of fisetin in human mast cells (HMC-1). Pharm Res. (2007) 55:31–7. doi: 10.1016/j.phrs.2006.10.002
74. Suh Y, Afaq F, Johnson JJ, Mukhtar H. A plant flavonoid fisetin induces apoptosis in colon cancer cells by inhibition of COX2 and Wnt/EGFR/NF-κB-signaling pathways. Carcinogenesis (2009) 30:300–7. doi: 10.1093/carcin/bgn269
75. Goh FY, Upton N, Guan S, Cheng C, Shanmugam MK, Sethi G, et al. Fisetin, a bioactive flavonol, attenuates allergic airway inflammation through negative regulation of NF-κB. Eur J Pharmacol. (2012) 679:109–16. doi: 10.1016/j.ejphar.2012.01.002
76. Song B, Guan S, Lu J, Chen Z, Huang G, Li G, et al. Suppressive effects of fisetin on mice T lymphocytes in vitro and in vivo. J Surg Res. (2013) 185:399–409. doi: 10.1016/j.jss.2013.05.093
77. Sun Q, Zhang W, Zhong W, Sun X, Zhou Z. Dietary fisetin supplementation protects against alcohol-induced liver injury in mice. alcoholism: Clin Exp Res. (2016) 40:2076–84. doi: 10.1111/acer.13172
78. Wu M-Y, Hung S-K, Fu S-L. Immunosuppressive effects of fisetin in ovalbumin-induced asthma through inhibition of NF-κB activity. J Agric Food Chem. (2011) 59:10496–504. doi: 10.1021/jf202756f
79. Kim G-D, Lee SE, Park YS, Shin D-H, Park GG, Park C-S. Immunosuppressive effects of fisetin against dinitrofluorobenzene-induced atopic dermatitis-like symptoms in NC/Nga mice. Food Chem Toxicol. (2014) 66:341–9. doi: 10.1016/j.fct.2014.01.057
80. Li D, Tsun A, Li B, Chen C, Nie J, Piccioni M, et al. T Cell Metabolism in Autoimmune Diseases. London: INTECH Open Access Publisher (2012).
81. Waickman AT, Powell JD. mTOR, metabolism, and the regulation of T-cell differentiation and function. Immunol Rev. (2012) 249:43–58. doi: 10.1111/j.1600-065X.2012.01152.x
82. Powell JD, Delgoffe GM. The mammalian target of rapamycin: linking T cell differentiation, function, and metabolism. Immunity (2010) 33:301–11. doi: 10.1016/j.immuni.2010.09.002
83. Pierdominici M, Vacirca D, Delunardo F, Ortona E. mTOR signaling and metabolic regulation of T cells: new potential therapeutic targets in autoimmune diseases. Curr Pharmaceut Design (2011) 17:3888–97. doi: 10.2174/138161211798357809
84. Rathmell JC. Metabolism and autophagy in the immune system: immunometabolism comes of age. Immunol Rev. (2012) 249:5–13. doi: 10.1111/j.1600-065X.2012.01158.x
85. O'Neill LA, Hardie DG. Metabolism of inflammation limited by AMPK and pseudo-starvation. Nature (2013) 493:346–55. doi: 10.1038/nature11862.
86. Wang R, Green DR. Metabolic checkpoints in activated T cells. Nat Immunol. (2012) 13:907–15. doi: 10.1038/ni.2386
87. Delgoffe GM, Pollizzi KN, Waickman AT, Heikamp E, Meyers DJ, Horton MR, et al. The kinase mTOR regulates the differentiation of helper T cells through the selective activation of signaling by mTORC1 and mTORC2. Nat Immunol. (2011) 12:295–303. doi: 10.1038/ni.2005
88. Scottà C, Esposito M, Fazekasova H, Fanelli G, Edozie FC, Ali N, et al. Differential effects of rapamycin and retinoic acid on expansion, stability and suppressive qualities of human CD4+ CD25+ FOXP3+ T regulatory cell subpopulations. Haematologica (2013) 98:1291–9. doi: 10.3324/haematol.2012.074088
89. Park J, Kim M, Kang SG, Jannasch AH, Cooper B, Patterson J, et al. Short-chain fatty acids induce both effector and regulatory T cells by suppression of histone deacetylases and regulation of the mTOR–S6K pathway. Mucosal Immunol. (2015) 8:80–93. doi: 10.1038/mi.2014.44
90. Kumar P, Natarajan K, Shanmugam N. High glucose driven expression of pro-inflammatory cytokine and chemokine genes in lymphocytes: molecular mechanisms of IL-17 family gene expression. Cell Signal (2014) 26:528–39. doi: 10.1016/j.cellsig.2013.11.031
91. Monk JM, Jia Q, Callaway E, Weeks B, Alaniz RC, McMurray DN, et al. Th17 cell accumulation is decreased during chronic experimental colitis by (n-3) PUFA in Fat-1 mice. J Nutr. (2012) 142:117–24. doi: 10.3945/jn.111.147058
92. Haribhai D, Lin W, Edwards B, Ziegelbauer J, Salzman NH, Carlson MR, et al. A central role for induced regulatory T cells in tolerance induction in experimental colitis. J Immunol. (2009) 182:3461–8. doi: 10.4049/jimmunol.0802535
93. Surls J, Nazarov-Stoica C, Kehl M, Olsen C, Casares S, Brumeanu T-D. Increased membrane cholesterol in lymphocytes diverts T-cells toward an inflammatory response. PLoS ONE (2012) 7:e38733. doi: 10.1371/journal.pone.0038733
94. Kullenberg D, Taylor LA, Schneider M, Massing U. Health effects of dietary phospholipids. Lipids Health Dis. (2012) 11:3. doi: 10.1186/1476-511X-11-3
95. Vazquez-Agell M, Urpi-Sarda M, Sacanella E, Camino-Lopez S, Chiva-Blanch G, Llorente-Cortes V, et al. Cocoa consumption reduces NF-kappaB activation in peripheral blood mononuclear cells in humans. Nutr Metab Cardiovasc Dis. (2013) 23:257–63. doi: 10.1016/j.numecd.2011.03.015
96. Mao TK, van de Water J, Keen CL, Schmitz HH, Gershwin ME. Modulation of TNF-alpha secretion in peripheral blood mononuclear cells by cocoa flavanols and procyanidins. Develop Immunol. (2002) 9:135–41. doi: 10.1080/1044667031000137601
97. Ramos-Romero S, Perez-Cano FJ, Perez-Berezo T, Castellote C, Franch A, Castell M. Effect of a cocoa flavonoid-enriched diet on experimental autoimmune arthritis. Br J Nutr. (2012) 107:523–32. doi: 10.1017/S000711451100328X
98. Khan N, Afaq F, Syed DN, Mukhtar H. Fisetin, a novel dietary flavonoid, causes apoptosis and cell cycle arrest in human prostate cancer LNCaP cells. Carcinogenesis (2008) 29:1049–56. doi: 10.1093/carcin/bgn078
99. Wee LH, Morad NA, Aan GJ, Makpol S, Ngah WZW, Yusof YAM. Mechanism of chemoprevention against colon cancer cells using combined Gelam honey and Ginger extract via mTOR and Wnt/β-catenin pathways. Asian Pac J Cancer Prev. (2015) 16:6549–56. doi: 10.7314/APJCP.2015.16.15.6549
100. Banerjee N, Kim H, Talcott S, Mertens-Talcott S. Pomegranate polyphenolics suppressed azoxymethane-induced colorectal aberrant crypt foci and inflammation: possible role of miR-126/VCAM-1 and miR-126/PI3K/AKT/mTOR. Carcinogenesis (2013) 34:2814–22. doi: 10.1093/carcin/bgt295
101. Johnson SM, Gulhati P, Arrieta I, Wang X, Uchida T, Gao T, et al. Curcumin inhibits proliferation of colorectal carcinoma by modulating Akt/mTOR signaling. Anticancer Res. (2009) 29:3185–90.
102. Ramakrishna E, Maurya R, Konwar R, Chattopadhyay N. Quercetin-6-C-b-D-glucopyranoside, natural analog of quercetin exhibits anti-prostate cancer activity by inhibiting Akt-mTOR pathway via aryl hydrocarbon receptor. Biochimie (2015) 119:68–79. doi: 10.1016/j.biochi.2015.10.012
103. Guo Z, Hu X, Xing Z, Xing R, Lv R, Cheng X, et al. Baicalein inhibits prostate cancer cell growth and metastasis via the caveolin-1/AKT/mTOR pathway. Mol Cell Biochem. (2015) 406:111–9. doi: 10.1007/s11010-015-2429-8
104. Bai X, Ma Y, Zhang G. Butein suppresses cervical cancer growth through the PI3K/AKT/mTOR pathway. Oncol Rep. (2015) 33:3085–92. doi: 10.3892/or.2015.3922
105. Seo BR, Min K-J, Cho IJ, Kim SC, Kwon TK. Curcumin significantly enhances dual PI3K/Akt and mTOR inhibitor NVP-BEZ235-induced apoptosis in human renal carcinoma Caki cells through down-regulation of p53-dependent Bcl-2 expression and inhibition of Mcl-1 protein stability. PLoS ONE (2014) 9:e95588. doi: 10.1371/journal.pone.0095588
106. Zhao G, Han X, Zheng S, Li Z, Sha Y, Ni J, et al. Curcumin induces autophagy, inhibits proliferation and invasion by downregulating AKT/mTOR signaling pathway in human melanoma cells. Oncol Rep. (2016) 35:1065–74. doi: 10.3892/or.2015.4413
107. Alanazi IO, Khan Z. Understanding EGFR signaling in breast cancer and breast cancer stem cells: overexpression and therapeutic implications. Asian Pac J Cancer Prev. (2016) 17:445–53.
108. Tabernero J. Overcoming Resistance to Anti-EGFR Therapy in Colorectal Cancer. Chicago: American Society of Clinical Oncology (2015).
109. Suh Y, Afaq F, Khan N, Johnson JJ, Khusro FH, Mukhtar H. Fisetin induces autophagic cell death through suppression of mTOR signaling pathway in prostate cancer cells. Carcinogenesis (2010) 31:1424–33. doi: 10.1093/carcin/bgq115
110. Kim GT, Lee SH, Kim YM. Quercetin regulates sestrin 2-AMPK-mTOR signaling pathway and induces apoptosis via increased intracellular ROS in HCT116 Colon Cancer Cells. J Cancer Prev. (2013) 18:264–70. doi: 10.15430/JCP.2013.18.3.264
111. Granato M, Rizzello C, Montani MS, Cuomo L, Vitillo M, Santarelli R, et al. Quercetin induces apoptosis and autophagy in primary effusion lymphoma cells by inhibiting PI3K/AKT/mTOR and STAT3 signaling pathways. J Nutr Biochem. (2017) 41:124–36. doi: 10.1016/j.jnutbio.2016.12.011
112. Granato M, Rizzello C, Romeo MA, Yadav S, Santarelli R, D'Orazi G, et al. Concomitant reduction of c-Myc expression and PI3K/AKT/mTOR signaling by quercetin induces a strong cytotoxic effect against Burkitt's lymphoma. Int J Biochem Cell Biol. (2016) 79:393–400. doi: 10.1016/j.biocel.2016.09.006.
113. Bishayee K, Ghosh S, Mukherjee A, Sadhukhan R, Mondal J, Khuda-Bukhsh AR. Quercetin induces cytochrome-c release and ROS accumulation to promote apoptosis and arrest the cell cycle in G2/M, in cervical carcinoma: signal cascade and drug-DNA interaction. Cell Prolif. (2013) 46:153–63. doi: 10.1111/cpr.12017
114. Zhang L, Shamaladevi N, Jayaprakasha GK, Patil BS, Lokeshwar BL. Polyphenol-rich extract of Pimenta dioica berries (Allspice) kills breast cancer cells by autophagy and delays growth of triple negative breast cancer in athymic mice. Oncotarget (2015) 6:16379–95. doi: 10.18632/oncotarget.3834
115. Mezrich JD, Fechner JH, Zhang X, Johnson BP, Burlingham WJ, Bradfield CA. An interaction between kynurenine and the aryl hydrocarbon receptor can generate regulatory T cells. J Immunol. (2010) 185:3190–8. doi: 10.4049/jimmunol.0903670
Keywords: flavonoids, mTOR, autoimmunity, metabolism, T regulatory cells
Citation: Hosseinzade A, Sadeghi O, Naghdipour Biregani A, Soukhtehzari S, Brandt GS and Esmaillzadeh A (2019) Immunomodulatory Effects of Flavonoids: Possible Induction of T CD4+ Regulatory Cells Through Suppression of mTOR Pathway Signaling Activity. Front. Immunol. 10:51. doi: 10.3389/fimmu.2019.00051
Received: 22 August 2018; Accepted: 09 January 2019;
Published: 31 January 2019.
Edited by:
Jia Sun, Jiangnan University, ChinaReviewed by:
Jue Hou, Virginia Mason Medical Center, United StatesJeffrey Knauf, Memorial Sloan Kettering Cancer Center, United States
Copyright © 2019 Hosseinzade, Sadeghi, Naghdipour Biregani, Soukhtehzari, Brandt and Esmaillzadeh. This is an open-access article distributed under the terms of the Creative Commons Attribution License (CC BY). The use, distribution or reproduction in other forums is permitted, provided the original author(s) and the copyright owner(s) are credited and that the original publication in this journal is cited, in accordance with accepted academic practice. No use, distribution or reproduction is permitted which does not comply with these terms.
*Correspondence: Ahmad Esmaillzadeh, YS1lc21haWxsemFkZWhAc2luYS50dW1zLmFjLmly