- 1Laboratorio Biología Celular y Molecular, Instituto de Investigación en Ciencias Odontológicas, Facultad de Odontología, Universidad de Chile, Santiago, Chile
- 2Laboratorio de Microbiología Médica y Patogénesis, Facultad de Medicina, Universidad Andrés Bello, Concepción, Chile
- 3Departamento de Biología, Facultad de Química y Biología, Universidad de Santiago de Chile, Santiago, Chile
Neisseria gonorrhoeae is a significant health problem worldwide due to multi-drug resistance issues and absence of an effective vaccine. Patients infected with N. gonorrhoeae have not shown a better immune response in successive infections. This might be explained by the fact that N. gonorrhoeae possesses several mechanisms to evade the innate and adaptative immune responses at different levels. Macrophages are a key cellular component in the innate immune response against microorganisms. The current information suggests that gonococcus can hijack the host response by mechanisms that involve the control of macrophages activity. In this mini review, we intend to condense the recent knowledge on the macrophage–N. gonorrhoeae interactions with a focus on strategies developed by gonococcus to evade or to exploit immune response to establish a successful infection. Finally, we discuss the opportunities and challenges of therapeutics for controlling immune manipulation by N. gonorrhoeae.
Introduction
Neisseria gonorrhoeae or gonococcus, a gram-negative diplococcus, belongs to the genus Neisseria and is the etiological agent of the sexually transmitted bacterial infection (STI) gonorrhea. Nowadays, gonorrhea is the second most common bacterial STI and results in substantial morbidity and economic cost worldwide. The majority of infections are benign mucosal infections of the urogenital tract, pharynx, and rectum. Ascended infections such as endometritis, salpingitis, epididymitis, and pelvic inflammatory disease (PID) are more difficult to treat. Gonococcal PID and its related complications (infertility, ectopic pregnancy, and chronic pelvic pain) constitute the major morbidity and mortality associated with gonorrhea (1). The most recent annual incidence estimates, based on data collected in 2012, indicate 78 million new cases worldwide with a global incidence rate of 19 per 1,000 females and 24 per 1,000 males (2, 3). Another serious concern is the current identification of antimicrobial drug resistance of N. gonorrhoeae, which includes the most recent fluoroquinolones and extended-spectrum cephalosporin (4–6). Indeed, U.S. Centers for Disease Control (CDC) identified multidrug resistance as among the 3 most “urgent” hazard-level threats to the U.S. population (7).
Recent information shows that N. gonorrhoeae can prevent the development of a successful protective immune response; The causes of the weak immune response triggered by N. gonorrhoeae are varied and correspond to multiple mechanisms that include the immune privilege of the reproductive tissue colonized by bacteria (8, 9), as well as the own strategies developed by the bacteria, such as epitope mimicry, antigenic variations and phase variation. Moreover, gonococci seem to directly interfere with the cells involved in adaptive immune response, such as dendritic cells and B and T lymphocytes (10–13). Regarding innate immunity, phagocytic cells such as macrophages and neutrophils (PMN) represent the earliest line of defense against invading bacteria. However, PMN cannot clear infections by N. gonorrhoeae (14). Since gonococcus can survive into PMN and suppress the oxidative burst (14, 15), it has been hypothesized that bacteria actively recruit PMN to permit the spread to profounder tissues of the host and even to other hosts.
Moreover, N. gonorrhoeae also affects macrophages and their functionality, posing additional difficulties to its detection and elimination by the innate immune system (16).
Considering that macrophages are critical cells in the innate immune response to N. gonorrhoeae, and macrophage-driven innate immune response can be subverted by N. gonorrhoeae, promoting the persistence of gonorrhea, we will highlight our current knowledge about macrophage–N. gonorrhoeae interactions in this mini review with a focus on strategies developed by gonococcus to evade or to exploit immune response to establish a successful infection.
Macrophages and Antimicrobial Response
Macrophages are present in almost all tissues and have diverse functions ranged from clearance of microbes, dead, and senescent cells until reparative and regulatory functions. Tissue-resident macrophages can derive from yolk sac macrophages, fetal liver monocytes, or adult bone-marrow monocytes capable of entering in tissues during inflammation (17, 18). When bacteria cross the layer of epithelial cells, accessing submucosa, they have the first encounter with macrophages (19). Macrophages recognize microbial pathogen-associated molecular patterns (PAMPs) and endogenous danger-associated molecular patterns (DAMPs) by genome-encoded pattern recognition receptors (PRRs). It leads the activation of host defense pathways, which include internalization of the pathogen into phagosomes and fusion with lysosomes to form phagolysosomes, where the microbes are killed by reactive oxygen and nitrogen species and proteolytic enzymes, resulting in the clearance of the infection (20). Also, activation of macrophages includes secretion of pro-inflammatory cytokines and antigen-presentation (21). Currently, it has been demonstrated that many signals recognized by macrophages drive to classical activation (M1 macrophages) or alternative activation (M2 macrophages) (22). While M1 macrophages stimulate a robust anti-tumor and microbicide response, M2 macrophages are involved in tumor progression, tissue remodeling, response against parasites and they have regulatory functions (23).
Cellular Model for Examining Macrophages During Infection
Diverse cell models have been used to study the interaction between N. gonorrhoeae and macrophages; these include cell lines and primary macrophages. The most common murine line used is RAW 264.7. This is a tumor line induced by Abelson murine leukemia virus (24). Human macrophage cell lines mostly used include U937 and THP-1. THP-1 cells are derived from acute monocytic leukemia (25) and U-937 cells were obtained from a patient suffering from histiocytic lymphoma (26). We should consider the differences between these lines based on their diverse origin and maturation stage (27).
Moreover, cell lines generally vary from primary macrophages, since repeated subculture typically results in some abnormalities such as the loss of genes expression (28); although this is not important for proliferation, it is critical for the immune function developed by macrophages. Because of these deficiencies of macrophage cell lines, it may be essential to complement such a model with culture of primary macrophages cells. Cell sources for primary macrophage models include mouse peritoneal macrophages (PM), bone marrow-derived macrophages (BMDM) and human monocyte-derived macrophage (MDM). Murine source of macrophages can be obtained from different strains of wild-type, transgenic and gene-targeted mice. Although PM can be easily harvested, the output is small and we should consider that sanitary and welfare conditions of the animal could affect the physiology of macrophages (29).
Conversely, BMDM do not present problems associated with the health status of the donor mice, because BMDM are obtained from bone marrow stem cells and are completely differentiated in vitro (29). In this line, human peripheral blood monocytes are the most commonly used precursors for generating macrophages in vitro. The MDM culture allows the monocytes differentiation and polarization toward different macrophage phenotypes using cytokines and/or bacterial products. Thus, M1 and M2 macrophages require GM-CSF or M-CSF in the presence of IFN-γ and/or LPS and IL-4 or IL-13, respectively (30, 31).
Mechanisms of Survival and Replication of N. gonorrhoeae During Macrophage Infection
Neisseria gonorrhoeae is able to prevent some defense mechanisms elicited by macrophages. Our laboratory and others have showed different mechanisms used by gonococcus to evade antimicrobial macrophage response. Below, we discuss some of the most relevant mechanisms observed in Macrophage–N. gonorrhoeae interactions.
Modulation of Phagocytosis by Macrophage
Neisseria gonorrhoeae can avoid phagocytosis mediated by opsonic antibodies. The membrane molecules such as lipooligosaccharide (LOS), Pili, Opa, and Porin (Por) are the main targets to generate antibodies against gonococci (32–34). By constantly varying these antigens, the bacteria elude antibody opsonization and successive IgG Fc-receptor-mediated phagocytosis (14, 35, 36). Additionally, LOS carbohydrates moiety mimic human surface antigens (37, 38) impairing the recognition by macrophages. Other membrane components such as pili reduce the association in vitro between N. gonorrhoeae and non-activated mouse PM macrophages, similar to streptococcal M protein which reduce the phagocytosis (39). However, the rate of phagocytosis of piliated gonococcus can be enhanced using immune anti-serum, indicating that this enhancement reflects primarily immunoglobulin G-mediated phagocytosis (opsonization through Fc receptor) rather than surface attachment (40, 41). In spite of this, later studies showed that pili (particularly pilin subunit PilE) acts as a factor that promotes non-opsonic ingestion of gonococci by human monocytes in absence of serum factors rather than as a protective factor (42).
Modulation of Intracellular Killing by Macrophage
It has been demonstrated that human monocytes and macrophages can kill intracellular gonococci (39) with diverse kinetics, ranged from a complete killing after 30 min of incubation in mouse peritoneal macrophages (43) to a more prolonged period of survival in RAW 264.7 cells and human MDM (44). When using membrane-impermeable antibiotics, such as gentamicin, our group and others have seen that the number of intracellular gonococcus inside macrophages increases over time (27, 45, 46). The persistence of N. gonorrhoeae inside macrophages is indicative of resistance mechanisms to innate host defenses. Thus, studies in monocytes showed that Opa-positive and piliated gonococci caused a differential oxidative response (42). Château et al. showed in differentiated U937 cells that internalized bacteria could escape from phagosome or endosome and retain viability (27).
Moreover, studies using MDM showed that purified PorB modifies phagosomal processing and reduces the delivery of the lysosomal enzyme cathepsin D, suggesting a delay in phagosome maturation and oxidative killing mechanisms (47), which is consistent with the absence of colocalization of N. gonorrhoeae with Lysosomal-associated membrane protein 1(LAMP-1) present in the lysosomal acidic compartment (27). In this way, two other proteins have been described, Ng-MIP (with homology to Macrophage infectivity potentiator), and Ng-OmpA (with homology to the outer membrane protein A). Ng-OmpA, unlike Ng-MIP, has been associated with the adhesion and internalization of N. gonorrhoeae in RAW 264.7 cells (44, 48). However, both proteins have shown that they protect bacteria from being killed by macrophages, probably through mechanisms related to the activity of peptidylprolyl cis/trans isomerase (PPIase) (44) and the inhibition of apoptosis of infected macrophages (48), as observed in other pathogens (49–51). Intracellular survival is also favored by the modulation of cellular iron metabolism by gonococcus. Infection of human monocytes, THP-1, MM6, and murine RAW 264.7 cell lines showed an upregulation of hepcidin, neutrophil gelatinase-associated lipocalin (NGAL) and Natural resistance-associated macrophage protein 1 (NRAMP1), suggesting an increase in cellular iron bioavailability (52). Similarly, infection of BMDM with N. gonorrhoeae was associated with increased availability of intracellular iron, associated with INF-β and cGAS/STING signaling pathway (53).
Modulation of Macrophage Cell Death
Apoptosis and autophagy constitute cell death modes for the elimination of infected cells. Failure in these mechanisms can favor the advance of disease (54, 55). Neisseria gonorrhoeae infection and its effects on apoptosis have been studied in PMN, and epithelial cells showed different results (56–59). A study using various sources of macrophages showed that gonococcus inhibit apoptosis in U937 and MDM cells in intrinsic and extrinsic pathways, but in THP-1 cells only extrinsic apoptotic pathway was affected (27). Concerning autophagy, Zughaier et al. showed that a phosphoethanolamine (PEA) modification of lipid A increases N. gonorrhoeae survival through evasion of autophagy affecting the TLR4-mediated induction of autophagic flux in RAW264 and THP-1 macrophages (60). Additionally, cell death can arise by pyroptosis. Studies using N. gonorrhoeae or isolated N. gonorrhoeae LOS showed cell death through NLRP3-mediated pyronecrosis in THP-1 cells (61) and cell death in human macrophages by activation of both canonical and non-canonical pyroptosis pathways (62).
Modulation of Macrophage Activation
Several membrane components from N. gonorrhoeae including LOS play a role during infection and modulation of antimicrobial response (63, 64). The immunomodulatory potential of LOS is based on lipid A backbone rather than carbohydrates structure. In this context, Patrone et al. showed that the variation in carbohydrates structure from LOS has no influence on production of IL-8, IL-12, and TNF-α cytokines (65). Indeed, THP-1 cells showed differences in their inflammatory response, TLR4/MD2 signaling or TNF-α production, toward LOS derived from a variety of Neisseria strains independent of the oligosaccharide truncation (66). Contrarily, the presence of single lauric acid residue in a determinate position of Lipid A and a hexa-acylated lipid A seems to be critical for the initiation of pro-inflammatory responses, since lpxLII and msbB mutants that lost these features showed a reduced ability to induce pro-inflammatory cytokines and stimulation of TLR-4-inflammatory signaling in U937, THP-1 and mouse primary macrophages (67, 68).
Similarly, modification of gonococcal lipid A with PEA also influences the production of CXCL3 and CXCL10 chemokines in infected macrophages (60). Besides, Knilans et al. showed that other molecules like peptidoglycan monomers released during N. gonorrhoeae cell wall remodeling by LtgA and LtgD lytic transglycosylases, also suppress TNF-α and IL-1β production in THP-1 cells by modulation TLR-2 and NOD2 signaling pathways (69). In this way, it is possible to hypothesize that Opa binding to CEACAM1 present on monocytes may suppress the activation of this cell, similarly to CD4 T cells (70, 71).
Modulation of Macrophage Phenotype
Macrophages are part of reproductive tissues together with epithelial and stromal cells both in humans and mice (72). Considering that reproductive organs, which are targets of N. gonorrhoeae infection, have a privileged immune status (73–75), one can speculate that macrophages could contribute to regulatory response. Findings of our laboratory have demonstrated that gonococcus affects murine RAW264 and its functionality. We showed a shift toward the production of IL-10 and TGF-β and inefficient up-regulation in molecules involved in antigen presentation such as MHC class II and CD86, and therefore a weak allogeneic T-cell stimulatory activity (45).
Similarly, differentiated macrophages from THP-1 and U937 cells challenged with N. gonorrhoeae showed induction of IL-10 and low levels of IL-12 (27). In this line, we have also demonstrated that MDM challenged with N. gonorrhoeae were differentiated toward an M2 profile, expressing the CD163 marker, inducing anti-inflammatory cytokines and inhibitory surface molecules with a low capacity to stimulate CD4 T cells proliferation (46). Regarding this, it is crucial to implement studies that characterize the phenotype of macrophages in a murine model or the genital tract of the patient.
Opportunities and Challenges of Therapeutics to Control Immune Manipulation
Due to multi-drug resistance problems related to gonorrhea, researchers and clinicians are dedicated to making preventive vaccines. However, the capacity to create an effective vaccine has not been possible yet due to the characteristics of the immune response against N. gonorrhoeae (76). Today, the development of gonorrhea vaccines has not been effective. Only one study has shown reduced rates of gonorrhea infection in patients immunized with outer membrane vesicle meningococcal B vaccine (MeNZB) with an effectiveness of 31% (77). However, these findings are the first evidence in humans lately. Thus, other types of treatments become necessary. Liu et al. assessed an intravaginal treatment using IL-12–loaded microspheres in a murine model of infection. Microencapsulated IL-12 transforms the infection in a “live vaccine” that triggers the production of local and systemic gonococcus-specific antibodies (78). This method was also evaluated together with a non-living gonococcal vaccine based on gonococcal outer membrane vesicles (OMV) showing an elicitation of long-term humoral protective immunity driven by a Th1 response, which was effective against antigenically diverse strains of N. gonorrhoeae (79). Besides, the blockade of TGF-β1 and IL-10 has been useful to reverse N. gonorrhoeae-mediated suppression of Th1 and Th2 responses and it facilitates the development of specific protective immunity (11, 80). Moreover, Youssef et al. hypothesized the use of curcumin and vitamin D to “rescue” the immunity, by counteracting the different ways used by N. gonorrhoeae to evade the immune response (76). Considering that gonococcus affects macrophages polarization toward M2 phenotype (42), a putative treatment which would reverse M2 to M1 polarization is likely to be beneficial, since M1 polarization is associated with control of acute infections by many intracellular bacteria (81). In this regard, Na et al. blocked M-CSF-induced M2 macrophages differentiation using a COX-2 inhibitor Etodolac driving pro-inflammatory activities in human and murine macrophages and proposing it as a therapy for induction of enhanced anti-tumor immunity (82). In this line, we think that the M2 phenotype promoted by gonococcus could be reverted using COX-2 inhibitor. We also showed no significant differences in IL-1β levels in MDM infected cells compared to non-infected MDM cells, suggesting that N. gonorrhoeae could trigger insufficient IL-1β levels to activate innate immune response (83). Then, we were able to promote the IL-1β processing and release using exogenous ATP. Interestingly, production of IL-1β did not correlate with the activation of inflammasome-mediated caspase-1, suggesting that ATP could be acting at the level of mechanisms related to vesicle trafficking or pore formation.
We also showed a significant up-regulation of co-inhibitory molecule Programmed Death Ligand 1 (PD-L1) and CD86 down-regulation in macrophages upon gonococcus infection (46). Considering that in the immunological synapse the outcome of the immune response relies at least on balance between positive (CD86) and negative (PD-L1) co-stimulation signals, we hypothesize that an increase in PD-L1 expression, particularly in macrophages, represents a strategy used by N. gonorrhoeae to inhibit previously activated T cells at the later stages of the immune response in peripheral tissues. It could be reversed by PD-L1 blockade, as it has been shown for the treatment of severe chronic infectious diseases such as LCMV, HIV, HCV, HBV, C. trachomatis, and T. crassiceps infection (84–87).
Conclusion
Until now, much of the research associated with modulation of innate immune cell response has been focused on gonococcus-PMN interaction, and many advances in this field have been achieved. Nevertheless, the interaction of N. gonorrhoeae with the resident or recruited macrophages is less well understood. Our current knowledge of the N. gonorrhoeae interactions with macrophages shows the capacity of gonococcus to avoid the response to an infectious challenge (phagocytosis and effective killing) and the elicitation of acquired immune response (Figure 1). Although we are still investigating which are the molecules from N. gonorrhoeae that confer resistance to macrophage, we have advanced in many questions about modulation of macrophage response and its role in gonococcal pathogenesis. There are still questions associated with the signaling pathways and cellular mechanisms related to inflammatory response, as well as the effects in the T cell activation and differentiation. Accessibility to mouse and human macrophages and the capacity of genetic or pharmacological manipulation will facilitate the studies addressed to find potential targets to be exploited with new therapeutic approaches beyond antimicrobial drugs.
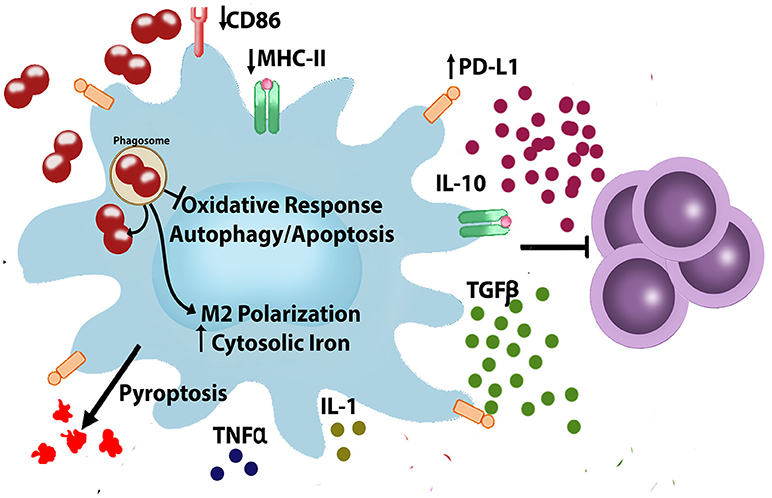
Figure 1. Neisseria gonorrhoeae evades and modulates macrophages. During infection, N. gonorrhoeae interact with immune cells such as macrophages. In macrophages N. gonorrhoeae is able to escape from phagosome (27, 42, 45–48), modulate cellular iron metabolism (52, 53), inhibit apoptosis and autophagy (27, 60), modulate production of inflammatory/anti-inflammatory cytokines (63–71, 83), and polarizes macrophages, resulting in macrophages that are less capable of T cell proliferation (45). CD86, Cluster of Differentiation 86; IL- 1, Interleukin 1; IL-10, Interleukin 10; MHC, Major Histocompatibility Complex; PD-L1, Programmed Death-Ligand 1; TGF-β, Transforming Growth Factor-beta; TNF-α, Tumor Necrosis Factor-alpha.
Author Contributions
AE conceived and designed the mini review and wrote the mini review. PR contributed to the writing and critically revised the paper. CA-C critically revised the paper. All authors read and approved the submitted version.
Funding
This work was supported by the grant from Fondo Nacional de Ciencia y Tecnología FONDECYT 1180666 and grant from Programa Apoyo a la Formación de Redes Internacionales PCI-CONICYT REDI170370.
Conflict of Interest Statement
The authors declare that the research was conducted in the absence of any commercial or financial relationships that could be construed as a potential conflict of interest.
Acknowledgments
Thanks to Mr. Juan Fernández, from the Language and Translation services of the Faculty for kindly proofreading and checking the spelling and grammar of this article.
References
1. Jerse AE, Deal CD. Vaccine research for gonococcal infections: where are we? Sex Transm Infect. (2013) 89(Suppl. 4):iv63–8. doi: 10.1136/sextrans-2013-051225
2. World Health Organization. Global Incidence and Prevalence of Selected Curable Sexually Transmitted Infections - 2008. (2012).
3. World Health Organization. Global Antimicrobial Resistance Surveillance System (GLASS) Report: Early Implementation 2016-2017. Geneva (2017). Available online at: http://www.who.int/glass/en/
4. Bolan GA, Sparling PF, Wasserheit JN. The emerging threat of untreatable gonococcal infection. N Engl J Med. (2012) 366:485–7. doi: 10.1056/NEJMp1112456
5. Fifer H, Natarajan U, Jones L, Alexander S, Hughes G, Golparian D, et al. Failure of dual antimicrobial therapy in treatment of Gonorrhea. N Engl J Med. (2016) 374:2504–6. doi: 10.1056/NEJMc1512757
6. Unemo M, Shafer WM. Antimicrobial resistance in Neisseria gonorrhoeae in the 21st century: past, evolution, and future. Clin Microbiol Rev. (2014) 27:587–613. doi: 10.1128/CMR.00010-1427/3/587
7. Wetzler LM, Feavers IM, Gray-Owen SD, Jerse AE, Rice PA, Deal CD. Summary and recommendations from the National Institute of Allergy and Infectious Diseases (NIAID) workshop “Gonorrhea Vaccines: the Way Forward”. Clin Vaccine Immunol. (2016) 23:656–63. doi: 10.1128/CVI.00230-16CVI.00230-16
8. Imarai M, Candia E, Rodriguez-Tirado C, Tognarelli J, Pardo M, Perez T, et al. Regulatory T cells are locally induced during intravaginal infection of mice with Neisseria gonorrhoeae. Infect Immun. (2008) 76:5456–65. doi: 10.1128/IAI.00552-08
9. Niederkorn JY. See no evil, hear no evil, do no evil: the lessons of immune privilege. Nat Immunol. (2006) 7:354–9. doi: 10.1038/ni1328
10. Arora S, Olszewski MA, Tsang TM, McDonald RA, Toews GB, Huffnagle GB. Effect of cytokine interplay on macrophage polarization during chronic pulmonary infection with Cryptococcus neoformans. Infect Immun. (2011) 79:1915–26. doi: 10.1128/IAI.01270-10
11. Liu Y, Islam EA, Jarvis GA, Gray-Owen SD, Russell MW. Neisseria gonorrhoeae selectively suppresses the development of Th1 and Th2 cells, and enhances Th17 cell responses, through TGF-beta-dependent mechanisms. Mucosal Immunol. (2012) 5:320–31. doi: 10.1038/Mi.2012.12
12. Plant LJ, Jonsson AB. Type IV pili of Neisseria gonorrhoeae influence the activation of human CD4+ T cells. Infect Immun. (2006) 74:442–8. doi: 10.1128/IAI.74.1.442-448.2006
13. Zhu W, Ventevogel MS, Knilans KJ, Anderson JE, Oldach LM, McKinnon KP, et al. Neisseria gonorrhoeae suppresses dendritic cell-induced, antigen-dependent CD4 T cell proliferation. PLoS ONE (2012) 7:e41260. doi: 10.1371/journal.pone.0041260
14. Criss AK, Seifert HS. A bacterial siren song: intimate interactions between Neisseria and neutrophils. Nat Rev Microbiol. (2012) 10:178–90. doi: 10.1038/nrmicro2713nrmicro2713
15. Palmer A, Criss AK. Gonococcal defenses against antimicrobial activities of neutrophils. Trends Microbiol. (2018) 26:1022–34. doi: 10.1016/j.tim.2018.07.003
16. Quillin SJ, Seifert HS. Neisseria gonorrhoeae host adaptation and pathogenesis. Nat Rev Microbiol. (2018) 16:226–40. doi: 10.1038/nrmicro.2017.169
17. Cole J, Aberdein J, Jubrail J, Dockrell DH. The role of macrophages in the innate immune response to Streptococcus pneumoniae and Staphylococcus aureus: mechanisms and contrasts. Adv Microb Physiol. (2014) 65:125–202. doi: 10.1016/bs.ampbs.2014.08.004
18. van de Laar L, Saelens W, De Prijck S, Martens L, Scott CL, Van Isterdael G, et al. Yolk Sac Macrophages, Fetal Liver, and Adult Monocytes can colonize an empty niche and develop into functional tissue-resident macrophages. Immunity (2016) 44:755–68. doi: 10.1016/j.immuni.2016.02.017
19. Rarick M, McPheeters C, Bright S, Navis A, Skefos J, Sebastiani P, et al. Evidence for cross-regulated cytokine response in human peripheral blood mononuclear cells exposed to whole gonococcal bacteria in vitro. Microb Pathog. (2006) 40:261–70. doi: 10.1016/j.micpath.2006.02.003
20. Vladimer GI, Marty-Roix R, Ghosh S, Weng D, Lien E. Inflammasomes and host defenses against bacterial infections. Curr Opin Microbiol. (2013) 16:23–31. doi: 10.1016/j.mib.2012.11.008
21. Gordon S, Taylor PR. Monocyte and macrophage heterogeneity. Nat Rev Immunol. (2005) 5:953–64. doi: 10.1038/nri1733
22. Biswas SK, Mantovani A. Macrophage plasticity and interaction with lymphocyte subsets: cancer as a paradigm. Nat Immunol. (2010) 11:889–96. doi: 10.1038/ni.1937ni.1937
23. Sica A, Mantovani A. Macrophage plasticity and polarization: in vivo veritas. J Clin Invest. (2012) 122:787–95. doi: 10.1172/JCI5964359643
24. Raschke WC, Baird S, Ralph P, Nakoinz I. Functional macrophage cell lines transformed by Abelson leukemia virus. Cell (1978) 15:261–7. doi: 10.1016/0092-8674(78)90101-0
25. Tsuchiya S, Yamabe M, Yamaguchi Y, Kobayashi Y, Konno T, Tada K. Establishment and characterization of a human acute monocytic leukemia cell line (THP-1). Int J Cancer (1980) 26:171–6. doi: 10.1002/ijc.2910260208
26. Yagnik DR, Hillyer P, Marshall D, Smythe CD, Krausz T, Haskard DO, et al. Noninflammatory phagocytosis of monosodium urate monohydrate crystals by mouse macrophages. implications for the control of joint inflammation in gout. Arthritis Rheum. (2000) 43:1779–89. doi: 10.1002/1529-0131(200008)43:8<1779::AID-ANR14>3.0.CO;2-2
27. Chateau A, Seifert HS. Neisseria gonorrhoeae survives within and modulates apoptosis and inflammatory cytokine production of human macrophages. Cell Microbiol. (2016) 18:546–60. doi: 10.1111/cmi.12529
28. Pan C, Kumar C, Bohl S, Klingmueller U, Mann M. Comparative proteomic phenotyping of cell lines and primary cells to assess preservation of cell type-specific functions. Mol Cell Proteomics (2009) 8:443–50. doi: 10.1074/mcp.RA118.000610
29. Marim FM, Silveira TN, Lima DS Jr, Zamboni DS. A method for generation of bone marrow-derived macrophages from cryopreserved mouse bone marrow cells. PLoS ONE (2010) 5:e15263. doi: 10.1371/journal.pone.0015263
30. Geissmann F, Manz MG, Jung S, Sieweke MH, Merad M, Ley K. Development of monocytes, macrophages, and dendritic cells. Science (2010) 327:656–61. doi: 10.1126/science.1178331
31. Martinez FO, Sica A, Mantovani A, Locati M. Macrophage activation and polarization. Front Biosci. (2008) 13:453–61. doi: 10.2741/2692
32. Brooks GF, Lammel CJ. Humoral immune response to gonococcal infections. Clin Microbiol Rev. (1989) 2(Suppl. ):S5–10.
33. Plummer FA, Chubb H, Simonsen JN, Bosire M, Slaney L, Nagelkerke NJ, et al. Antibodies to opacity proteins (Opa) correlate with a reduced risk of gonococcal salpingitis. J Clin Invest. (1994) 93:1748–55. doi: 10.1172/JCI117159
34. Zheng H. Analysis of the antigen-antibody specificity in the semen of patients with Neisseria gonorrhoeae. Chin Med Sci J. (1997) 12:47–9.
35. Shafer WM, Datta A, Kolli VS, Rahman MM, Balthazar JT, Martin LE, et al. Phase variable changes in genes lgtA and lgtC within the lgtABCDE operon of Neisseria gonorrhoeae can modulate gonococcal susceptibility to normal human serum. J Endotoxin Res. (2002) 8:47–58. doi: 10.1177/09680519020080010501
36. Virji M. Pathogenic neisseriae: surface modulation, pathogenesis and infection control. Nat Rev Microbiol. (2009) 7:274–86. doi: 10.1038/nrmicro2097nrmicro2097
37. Mandrell RE. Further antigenic similarities of Neisseria gonorrhoeae lipooligosaccharides and human glycosphingolipids. Infect Immun. (1992) 60:3017–20.
38. Mandrell RE, Griffiss JM, Macher BA. Lipooligosaccharides (LOS) of Neisseria gonorrhoeae and Neisseria meningitidis have components that are immunochemically similar to precursors of human blood group antigens. Carbohydrate sequence specificity of the mouse monoclonal antibodies that recognize crossreacting antigens on LOS and human erythrocytes. J Exp Med. (1988) 168:107–26. doi: 10.1084/jem.168.1.107
39. Blake M, Swanson J. Studies on Gonococcus infection. IX. In vitro decreased assocation of pilated gonococci with mouse peritoneal macrophages. Infect Immun. (1975) 11:1402–4.
40. Jones RB, Buchanan TM. Quantitative measurement of phagocytosis of Neisseria gonorrhoeae by mouse peritoneal macrophages. Infect Immun. (1978) 20:732–8.
41. Jones RB, Newland JC, Olsen DA, Buchanan TM. Immune-enhanced phagocytosis of Neisseria gonorrhoeae by macrophages: characterization of the major antigens to which opsonins are directed. J Gen Microbiol. (1980) 121:365–72. doi: 10.1099/00221287-121-2-365
42. Knepper B, Heuer I, Meyer TF, van Putten JP. Differential response of human monocytes to Neisseria gonorrhoeae variants expressing pili and opacity proteins. Infect Immun. (1997) 65:4122–9.
43. Cooper MD, Floyd SA. In vitro kinetics of phagocytosis and intracellular killing of gonococci by peritoneal macrophages from mice deficient in complement component 5. Infect Immun. (1982) 36:363–70.
44. Leuzzi R, Serino L, Scarselli M, Savino S, Fontana MR, Monaci E, et al. Ng-MIP, a surface-exposed lipoprotein of Neisseria gonorrhoeae, has a peptidyl-prolyl cis/trans isomerase (PPIase) activity and is involved in persistence in macrophages. Mol Microbiol. (2005) 58:669–81. doi: 10.1111/j.1365-2958.2005.04859.x
45. Escobar A, Candia E, Reyes-Cerpa S, Villegas-Valdes B, Neira T, Lopez M, et al. Neisseria gonorrhoeae induces a tolerogenic phenotype in macrophages to modulate host immunity. Mediators Inflamm. (2013) 2013:127017. doi: 10.1155/2013/127017
46. Ortiz MC, Lefimil C, Rodas PI, Vernal R, Lopez M, Acuna-Castillo C, et al. Neisseria gonorrhoeae modulates immunity by Polarizing human macrophages to a M2 profile. PLoS ONE (2015) 10:e0130713. doi: 10.1371/journal.pone.0130713
47. Mosleh IM, Huber LA, Steinlein P, Pasquali C, Gunther D, Meyer TF. Neisseria gonorrhoeae porin modulates phagosome maturation. J Biol Chem. (1998) 273:35332–8. doi: 10.1074/jbc.273.52.35332
48. Serino L, Nesta B, Leuzzi R, Fontana MR, Monaci E, Mocca BT, et al. Identification of a new OmpA-like protein in Neisseria gonorrhoeae involved in the binding to human epithelial cells and in vivo colonization. Mol Microbiol. (2007) 64:1391–403. doi: 10.1111/j.1365-2958.2007.05745.x
49. Lundemose AG, Kay JE, Pearce JH. Chlamydia trachomatis Mip-like protein has peptidyl-prolyl cis/trans isomerase activity that is inhibited by FK506 and rapamycin and is implicated in initiation of chlamydial infection. Mol Microbiol. (1993) 7:777–83. doi: 10.1111/j.1365-2958.1993.tb01168.x
50. Moro A, Ruiz-Cabello F, Fernandez-Cano A, Stock RP, Gonzalez A. Secretion by Trypanosoma cruzi of a peptidyl-prolyl cis-trans isomerase involved in cell infection. EMBO J. (1995) 14:2483–90. doi: 10.1002/j.1460-2075.1995.tb07245.x
51. Sukumaran SK, Selvaraj SK, Prasadarao NV. Inhibition of apoptosis by Escherichia coli K1 is accompanied by increased expression of BclXL and blockade of mitochondrial cytochrome c release in macrophages. Infect Immun. (2004) 72:6012–22. doi: 10.1128/IAI.72.10.6012-6022.2004
52. Zughaier SM, Kandler JL, Shafer WM. Neisseria gonorrhoeae modulates iron-limiting innate immune defenses in macrophages. PLoS ONE (2014) 9:e87688. doi: 10.1371/journal.pone.0087688
53. Andrade WA, Agarwal S, Mo S, Shaffer SA, Dillard JP, Schmidt T, et al. Type I interferon induction by Neisseria gonorrhoeae: dual requirement of cyclic GMP-AMP synthase and toll-like receptor 4. Cell Rep. (2016) 15:2438–48. doi: 10.1016/j.celrep.2016.05.030
54. Lamkanfi M, Dixit VM. Manipulation of host cell death pathways during microbial infections. Cell Host Microbe (2010) 8:44–54. doi: 10.1016/j.chom.2010.06.007
55. Choy A, Roy CR. Autophagy and bacterial infection: an evolving arms race. Trends Microbiol. (2013) 21:451–6. doi: 10.1016/j.tim.2013.06.009
56. Binnicker MJ, Williams RD, Apicella MA. Infection of human urethral epithelium with Neisseria gonorrhoeae elicits an upregulation of host anti-apoptotic factors and protects cells from staurosporine-induced apoptosis. Cell Microbiol. (2003) 5:549–60. doi: 10.1046/j.1462-5822.2003.00300.x
57. Chen A, Seifert HS. Neisseria gonorrhoeae-mediated inhibition of apoptotic signalling in polymorphonuclear leukocytes. Infect Immun. (2011) 79:4447–58. doi: 10.1128/IAI.01267-10
58. Follows SA, Murlidharan J, Massari P, Wetzler LM, Genco CA. Neisseria gonorrhoeae infection protects human endocervical epithelial cells from apoptosis via expression of host antiapoptotic proteins. Infect Immun. (2009) 77:3602–10. doi: 10.1128/IAI.01366-08
59. Howie HL, Shiflett SL, So M. Extracellular signal-regulated kinase activation by Neisseria gonorrhoeae downregulates epithelial cell proapoptotic proteins Bad and Bim. Infect Immun. (2008) 76:2715–21. doi: 10.1128/IAI.00153-08
60. Zughaier SM, Kandler JL, Balthazar JT, Shafer WM. Phosphoethanolamine modification of Neisseria gonorrhoeae lipid A reduces autophagy flux in macrophages. PLoS ONE (2015) 10:e0144347. doi: 10.1371/journal.pone.0144347
61. Duncan JA, Gao X, Huang MT, O'Connor BP, Thomas CE, Willingham SB, et al. Neisseria gonorrhoeae activates the proteinase cathepsin B to mediate the signaling activities of the NLRP3 and ASC-containing inflammasome. J Immunol. (2009) 182:6460–9. doi: 10.4049/jimmunol.0802696182/10/6460
62. Ritter JL, Genco CA. Neisseria gonorrhoeae-induced inflammatory pyroptosis in human macrophages is dependent on intracellular gonococci and lipooligosaccharide. J Cell Death (2018) 11:1179066017750902. doi: 10.1177/1179066017750902
63. Merz AJ, So M. Interactions of pathogenic neisseriae with epithelial cell membranes. Annu Rev Cell Dev Biol. (2000) 16:423–57. doi: 10.1146/annurev.cellbio.16.1.42316/1/423
64. Takeuchi O, Akira S. Pattern recognition receptors and inflammation. Cell (2010) 140:805–20. doi: 10.1016/j.cell.2010.01.022
65. Patrone JB, Stein DC. Effect of gonococcal lipooligosaccharide variation on human monocytic cytokine profile. BMC Microbiol. (2007) 7:7. doi: 10.1186/1471-2180-7-7
66. Pridmore AC, Jarvis GA, John CM, Jack DL, Dower SK, Read RC. Activation of toll-like receptor 2 (TLR2) and TLR4/MD2 by Neisseria is independent of capsule and lipooligosaccharide (LOS) sialylation but varies widely among LOS from different strains. Infect Immun. (2003) 71:3901–8. doi: 10.1128/IAI.71.7.3901-3908.2003
67. Ellis CD, Lindner B, Anjam Khan CM, Zahringer U, Demarco de Hormaeche R. The Neisseria gonorrhoeae lpxLII gene encodes for a late-functioning lauroyl acyl transferase, and a null mutation within the gene has a significant effect on the induction of acute inflammatory responses. Mol Microbiol. (2001) 42:167–81. doi: 10.1046/j.1365-2958.2001.02619.x
68. Zhou X, Gao X, Broglie PM, Kebaier C, Anderson JE, Thom N, et al. Hexa-acylated lipid A is required for host inflammatory response to Neisseria gonorrhoeae in experimental gonorrhea. Infect Immun. (2014) 82:184–92. doi: 10.1128/IAI.00890-13
69. Knilans KJ, Hackett KT, Anderson JE, Weng C, Dillard JP, Duncan JA. Neisseria gonorrhoeae lytic transglycosylases LtgA and LtgD reduce host innate immune signaling through TLR2 and NOD2. ACS Infect Dis. (2017) 3:624–33. doi: 10.1021/acsinfecdis.6b00088
70. Lee HS, Boulton IC, Reddin K, Wong H, Halliwell D, Mandelboim O, et al. Neisserial outer membrane vesicles bind the coinhibitory receptor carcinoembryonic antigen-related cellular adhesion molecule 1 and suppress CD4+ T lymphocyte function. Infect Immun. (2007) 75:4449–55. doi: 10.1128/IAI.00222-07
71. Lee HS, Ostrowski MA, Gray-Owen SD. CEACAM1 dynamics during neisseria gonorrhoeae suppression of CD4+ T lymphocyte activation. J Immunol. (2008) 180:6827–35. doi: 10.4049/jimmunol.180.10.6827
72. Givan AL, White HD, Stern JE, Colby E, Gosselin EJ, Guyre PM, et al. Flow cytometric analysis of leukocytes in the human female reproductive tract: comparison of fallopian tube, uterus, cervix, and vagina. Am J Reprod Immunol. (1997) 38:350–9. doi: 10.1111/j.1600-0897.1997.tb00311.x
73. Chegini N, Gold LI, Williams RS. Localization of transforming growth factor beta isoforms TGF-beta 1, TGF-beta 2, and TGF-beta 3 in surgically induced endometriosis in the rat. Obstet Gynecol. (1994) 83:455–61.
74. Grant KS, Wira CR. Effect of mouse uterine stromal cells on epithelial cell transepithelial resistance (TER) and TNFalpha and TGFbeta release in culture. Biol Reprod. (2003) 69:1091–8. doi: 10.1095/biolreprod.103.015495
75. Wada K, Nomura S, Morii E, Kitamura Y, Nishizawa Y, Miyake A, et al. Changes in levels of mRNAs of transforming growth factor (TGF)-beta1, -beta2, -beta3, TGF-beta type II receptor and sulfated glycoprotein-2 during apoptosis of mouse uterine epithelium. J Steroid Biochem Mol Biol. (1996) 59:367–75.
76. Youssef DA, Peiris AN, Kelley JL, Grant WB. The possible roles of vitamin D and curcumin in treating gonorrhea. Med Hypotheses (2013) 81:131–5. doi: 10.1016/j.mehy.2013.04.013
77. Petousis-Harris H, Paynter J, Morgan J, Saxton P, McArdle B, Goodyear-Smith F, et al. Effectiveness of a group B outer membrane vesicle meningococcal vaccine against gonorrhoea in New Zealand: a retrospective case-control study. Lancet (2017) 390:1603–10. doi: 10.1016/S0140-6736(17)31449-6
78. Liu Y, Egilmez NK, Russell MW. Enhancement of adaptive immunity to Neisseria gonorrhoeae by local intravaginal administration of microencapsulated interleukin 12. J Infect Dis. (2013) 208:1821–9. doi: 10.1093/infdis/jit354
79. Liu Y, Hammer LA, Liu W, Hobbs MM, Zielke RA, Sikora AE, et al. Experimental vaccine induces Th1-driven immune responses and resistance to Neisseria gonorrhoeae infection in a murine model. Mucosal Immunol. (2017) 10:1594–608. doi: 10.1038/mi.2017.11mi201711
80. Liu Y, Liu W, Russell MW. Suppression of host adaptive immune responses by Neisseria gonorrhoeae: role of interleukin 10 and type 1 regulatory T cells. Mucosal Immunol. (2014) 7:165–76. doi: 10.1038/mi.2013.36mi201336
81. Mege JL, Mehraj V, Capo C. Macrophage polarization and bacterial infections. Curr Opin Infect Dis. (2011) 24:230–4. doi: 10.1097/QCO.0b013e328344b73e
82. Na YR, Yoon YN, Son DI, Seok SH. Cyclooxygenase-2 inhibition blocks M2 macrophage differentiation and suppresses metastasis in murine breast cancer model. PLoS ONE (2013) 8:e63451. doi: 10.1371/journal.pone.0063451
83. Garcia K, Escobar G, Mendoza P, Beltran C, Perez C, Arancibia S, et al. ATP Induces IL-1beta secretion in Neisseria gonorrhoeae-infected human macrophages by a mechanism not related to the NLRP3/ASC/Caspase-1 axis. Mediators Inflamm. (2016) 2016:1258504. doi: 10.1155/2016/1258504
84. Fankhauser SC, Starnbach MN. PD-L1 limits the mucosal CD8+ T cell response to Chlamydia trachomatis. J Immunol. (2014) 192:1079–90. doi: 10.4049/jimmunol.1301657
85. Liu J, Zhang E, Ma Z, Wu W, Kosinska A, Zhang X, et al. Enhancing virus-specific immunity in vivo by combining therapeutic vaccination and PD-L1 blockade in chronic hepadnaviral infection. PLoS Pathog. (2014) 10:e1003856. doi: 10.1371/journal.ppat.1003856
86. Peligero C, Argilaguet J, Guerri-Fernandez R, Torres B, Ligero C, Colomer P, et al. PD-L1 blockade differentially impacts regulatory T cells from HIV-infected individuals depending on plasma viremia. PLoS Pathog. (2015) 11:e1005270. doi: 10.1371/journal.ppat.1005270
Keywords: innate immunity, macrophages, Neisseria gonorrhoeae, host response, immunomodulation
Citation: Escobar A, Rodas PI and Acuña-Castillo C (2018) Macrophage–Neisseria gonorrhoeae Interactions: A Better Understanding of Pathogen Mechanisms of Immunomodulation. Front. Immunol. 9:3044. doi: 10.3389/fimmu.2018.03044
Received: 30 August 2018; Accepted: 10 December 2018;
Published: 21 December 2018.
Edited by:
Michael W. Russell, University at Buffalo, United StatesReviewed by:
Gary Jarvis, University of California, San Francisco, United StatesFrédéric J. Veyrier, Institut National de la Recherche Scientifique (INRS), Canada
Copyright © 2018 Escobar, Rodas and Acuña-Castillo. This is an open-access article distributed under the terms of the Creative Commons Attribution License (CC BY). The use, distribution or reproduction in other forums is permitted, provided the original author(s) and the copyright owner(s) are credited and that the original publication in this journal is cited, in accordance with accepted academic practice. No use, distribution or reproduction is permitted which does not comply with these terms.
*Correspondence: Alejandro Escobar, janodvm@gmail.com