- 1Clinic Unit of Infectious Diseases, Microbiology and Preventive Medicine, Institute of Biomedicine of Seville, IBiS, Virgen del Rocío University Hospital, Seville, Spain
- 2Laboratory of Immunovirology, Institute of Biomedicine of Seville, IBiS, Virgen del Rocío University Hospital/CSIC/University of Seville, Seville, Spain
- 3Immunology Laboratory, Vaccine Research Center, National Institute of Allergy and Infectious Diseases, National Institutes of Health, Bethesda, MD, United States
- 4Hospital San Cecilio, Internal Medicine, Granada, Spain
- 5Hospital Universitari Joan XXIII, IISPV, Universitat Rovira i Virgili, Tarragona, Spain
- 6Sección Inmunología, Laboratory InmunoBiología Molecular, Hospital General Universitario “Gregorio Marañón”, Madrid, Spain
- 7Instituto de Investigación Sanitaria del Gregorio Marañón, Madrid, Spain
- 8Servicio de Medicina Interna, Hospital Viamed, Santa Ángela de la Cruz, Seville, Spain
HIV-elite controllers are a minority group of HIV-infected patients with the ability to maintain undetectable HIV viremia for long time periods without antiretroviral treatment. A small group of HIV-controllers are also able to spontaneously clear the hepatitis C virus (HCV) whom we can refer to as “supercontrollers.” There are no studies that explore immune correlates looking for the mechanisms implicated in this extraordinary phenomenon. Herein, we have analyzed HCV- and HIV-specific T-cell responses, as well as T, dendritic and NK cell phenotypes. The higher HCV-specific CD4 T-cell polyfunctionality, together with a low activation and exhaustion T-cell phenotype was found in supercontrollers. In addition, the frequency of CD8 CD161high T-cells was related with HIV- and HCV-specific T-cells polyfunctionality. Interesting features regarding NK and plasmacytoid dendritic cells (pDCs) were found. The study of the supercontroller's immune response, subjects that spontaneously controls both chronic viral infections, could provide further insights into virus-specific responses needed to develop immunotherapeutic strategies in the setting of HIV cure or HCV vaccination.
Introduction
HIV-controllers are a rare group of HIV-infected patients who can maintain HIV viremia at low levels in the absence of antiretroviral treatment (1, 2). Although several efforts are being made to elucidate mechanisms responsible of this phenomenon, multiple factors seem to be involved (3, 4). Interestingly, the extraordinary capacity of these subjects allows them to show differential characteristic regarding hepatitis C virus (HCV) coinfection. We have previously communicated that Caucasian HIV-controllers exhibited lower HCV viral loads (VL) than non-controllers, and a different HCV genotype distribution (5). Furthermore, Sajadi et al. reported a high rate of spontaneous clearance of HCV among HIV-controllers (6). We referred as supercontrollers those patients that simultaneously, and spontaneously, control HIV and clear HCV. The existence of these patients suggests the idea of some common or additive mechanisms concur in the control of both viruses.
Virus-specific T-cell response has been extensively studied and a higher virus-specific T-cell response and polyfunctionality has been unequivocally related with HIV and HCV control (7, 8). Besides, innate immunity has been also related with natural viral control. Higher NK frequency and anti-viral activity has been found in HIV-controllers (9, 10) and a high differentiated NK phenotype (defined by CD57 and HLA-C-binding killer cell immunoglobulin-like receptor (KIR) expression) has been reported in HCV spontaneous clearers (11, 12). Also, myeloid dendritic cells (mDCs) and plasmacytoid dendritic cells (pDCs) frequency, phenotype and antigen-presenting capacity have been related with HIV control (13–15) and HCV clearance (16, 17). However, there are no studies focused on patients which spontaneously control both viruses. For this purpose, we have selected the extraordinary group of supercontrollers to perform deep immunophenotyping of T-cells, NK and dendritic cells and to analyze HCV- and HIV-specific T-cell responses in order to find shared mechanisms of control, and to know whether supercontrollers have immunological characteristics that enable them to control more than one virus infection.
Materials and Methods
Samples and Patients
Peripheral blood samples from 50 HIV and HCV co-infected patients were collected from three Spanish Hospitals: Virgen del Rocio University Hospital (Seville), San Cecilio University Hospital (Granada) and Joan XXIII University Hospital (Tarragona). Of those, eight were HCV spontaneous clearers and HIV-elite controllers (supercontrollers, SC), 14 non-spontaneously HCV clearers HIV-elite controllers (nSC), 13 HCV spontaneous clearers non-HIV-elite controllers (SnC), and 15 non-spontaneously HCV clearers non-HIV-elite controllers (nSnC). HIV-elite controllers were defined as HIV-infected patients with VL < 50 HIV-RNA copies/mL in absence of antiretroviral treatment for at least 1 year. Non-HIV-elite controllers were patients with confirmed VL > 2 × 103 HIV-RNA copies/mL in absence of antiretroviral treatment. HCV spontaneous clearers were defined as HCV infected patients (anti-HCV antibody positive) with < 10 HCV-RNA copies/ml in absence of anti-HCV treatment. Non-spontaneously HCV clearers or HCV chronic patients were those with confirmed detectable HCV VL, without anti-HCV treatment. This study was carried out in accordance with the recommendations of “Comité de Etica de la Investigación de Centro Hospital Universitario Virgen del Rocío de Sevilla” with written informed consent from all subjects. All subjects gave written informed consent in accordance with the Declaration of Helsinki. The protocol was approved by the Comité de Etica de la Investigación de Centro Hospital Universitario Virgen del Rocío de Sevilla (2012PI/240).
Laboratory Measurements
Absolute counts of CD4+ and CD8+ T-cells were determined in fresh whole blood by using an Epic XL-MCL flow cytometer (Beckman-Coulter, Brea, CA) according to the manufacturer's instructions. The plasma HIV-1 RNA concentration was measured by using quantitative PCR (Cobas Ampliprep/Cobas TaqMan HIV-1 test; Roche Molecular Systems, Basel, Switzerland) according to the manufacturer's protocol. The detection limit for this assay was 50 HIV-1 RNA copies/mL. Hepatitis C virus (HCV) RNA was determined using an available PCR procedure kit (Cobas Amplicor; Roche Diagnostics, Barcelona, Spain) with a detection limit of 10 HCV-RNA copies/ml. Plasma samples were tested for anti-HCV antibodies using HCV-ELISA (Siemens Healthcare Diagnosis, Deerfield, IL, USA). HCV genotype was determined using a reverse-hybridization assay (InnoLIPA HCV II; Innogenetics, Barcelona, Spain).
Cell Stimulation
Peripheral blood mononuclear cells (PBMCs) were thawed, washed and rested for 2h in DNase I (Roche Diagnostics, Indianapolis, IN)-containing R-10 complete medium. 1.5 × 106 PBMCs including 10 μg/mL of brefeldin A (Sigma Chemical Co, St. Louis MO), and 0.7 μg/mL of monensin (BD Biosciences), were stimulated in vitro for 6 h with 2 μg/ml of each peptide pool of HCV NS4A, HCV NS4B, HCV NS3, and HCV Core (BEI Resources Repository, Manassas, VA, USA). HCV peptides were based on HCV 1a H77 sequence. In addition, 1.5 × 106 PBMCs were stimulated with 2 μg/ml of an overlapped HIV (Gag)-specific peptide pool (NIH AIDS Reagent Program (https://www.aidsreagent.org/index.cfm)). 1.5 × 106 unstimulated cells and cells stimulated with staphylococcal enterotoxin B (SEB) as a positive control were included in each experiment. The stimulation was performed in the presence of titrated amounts of anti-CD107a-BV605 (clone H4A3; BD Biosciences, USA) monoclonal antibody as previously described (18). T-cell specific response was defined as the frequency of cells with detectable intracellular cytokine production, after background subtraction of the unstimulated condition, after stimulation with HCV NS3, NS4A, NS4B, and Core peptide and HIV Gag peptides. For this analysis 1 × 106 events were acquired and a median of 4.72 × 105 live T-cells were gated.
Immunophenotyping and Intracellular Cytokine Staining
Stimulated PBMCs were washed and stained with LIVE/DEAD fixable aqua dead cell stain (Life Technologies, CA, USA). The cells were then surface stained with anti-CD14-BB630, anti-CD20-BB630 (clones MoP9 and 2H7B, respectively, BD Bioscience, custom made), anti-CXCR3-BV421 (clone 1C6/CXCR3), anti-TIGIT-BV785 (clone 1G9), anti-CXCR6-BUV395 (clone 13B1E5), anti-CD56-BUV563 (clone NCAM16.2), anti-CD4-BUV805 (clone SK3) (BD Biosciences, USA), anti-Lag3-BV650 (clone 11C3C63), anti-PD1-BV711 (clone EH12.2H7), anti-CD161 (clone HP-3G10), anti-HLA-DR (clone L243) (Biolegend, USA), anti-Tim3-PE (clone FAB2356P, R&D), anti-CD45RO-ECD (clone UCHL1), anti-CD27-PECy5 (1A4CD27) (Beckman Coulter, USA) for 20 min at room temperature. Cells were then permeabilized (BD Cytofix/Cytoperm buffer, BD Bioscience, USA) and stained intracellularly with anti-CD3-BUV496 (clone UCHT7), anti-IFNγ-FITC (clone B27), anti-tumor necrosis factor alpha (TNFα)-PECy7 (clone MIH1), anti-IL2-APC (clone 5344.111) (BD Biosciences, USA), and anti-Granzyme B-PECy5.5 (clone GB11) (Thermo Fisher, USA) for 30 min at 4°C, and then washed twice and fixed in PBS containing 4% paraformaldehyde (PFA). Cells were acquired on a 30-parameters A5 Symphony flow cytometer using FACS Diva Software (BD Bioscience, Bethesda, USA). Data were analyzed using the FlowJo software (Treestar, Ashland, OR).
Dendritic Cells Immunophenotyping
When samples were available, PBMCs were stained with zombie UV dye (Biolegend, USA) and surface stained with Lineage cocktail 3-FITC, anti-b7-BV605 (clone FIB504), anti-CD141-BV650 (clone 1A4), anti-CD103-BV711 (BerACT8), anti-CD83-BUV395 (clone HB15e), anti-CD16-BUV496 (clone 3G8), anti-CD56-BUV563 (NCAM16.2), anti-CD11c-BUV661 (clone B-ly6), anti-CD86-BUV737 (clone 2331), anti-CD4-BUV805 (clone SK3), anti-CCR7-Ax700 (clone 150502), anti-CCR5-APCCy7 (clone 2D7/CCR5), anti-CD40-PECy5 (clone 5C3), and anti-PDL1-PECy7 (clone MIH1) (BD Bioscience, USA), anti-CD123-BV421 (clone GH6), anti-CD1c-BV510 (clone LI61), anti-BDCA2-BV785 (clone 201A), anti-CCR2-APC (clone K036C2), and anti-CCR9-PE (clone L053E8) (Biolegend, USA), anti-CD2-PETexaRed (clone RPA-2.10), and anti-HLADR-PECy5.5 (clone TU36) (Thermo Fisher, USA) for 20 min at room temperature and then washed twice and fixed in PBS containing 4% PFA. Cells were acquired on a 30-parameters A5 Symphony flow cytometer using FACS Diva Software (BD Bioscience, Bethesda, USA); data were analyzed using the FlowJo software (Treestar, Ashland, OR).
Statistical Analysis
Differences between unpaired samples were analyzed by Mann–Whitney U-tests. Correlations between variables were assessed using the Spearman rank test. All differences with a P < 0.05 were considered statistically significant. Statistical analyses were performed by using Statistical Package for the Social Sciences software (SPSS 22.0; SPSS Inc., Chicago, IL). Graphs were generated with Prism, version 5.0 (GraphPad Software, Inc.) and R Statistical Software (Foundation for Statistical Computing, Vienna, Austria) (19). Polyfunctionality was defined as the percentage of lymphocytes producing multiple cytokines. Polyfunctionality pie charts were constructed using Pestle version 1.6.2 and Spice version 5.2 (provided by M. Roederer, NIH, Bethesda, MD) and was quantified with the polyfunctionality index algorithm (20) employing the 0.1.2 beta version of the FunkyCells Boolean Dataminer software provided by Martin Larson (INSERM U1135, Paris, France). Differences between unpaired distributions in pie charts were analyzed by Permutation test, Spice version 5.2. In this test a P < 0.05 was considered statistically significant.
Results
Patients' Characteristics
Patients' characteristics are summarized in Table 1. No differences were found in sex, time from HIV diagnosis and category of transmission among groups. HIV-controllers were slightly older than non-controllers (53 [52–54] years old of SC and 47 [42–53] years old of nSC vs. 41 [36–46] years old for SnC and 45 [40–49] years old for nSnC). As expected, HIV controller groups, SC (supercontrollers) and nSC (non-spontaneously HCV clearers HIV-elite controllers) had higher nadir and CD4 T-cells levels (438 [281–598] cells/μl of SC and 550 [329–698] cells/μl of nSC) than non-controller groups, SnC (HCV spontaneous clearers non-HIV-elite controllers, 114 [35–295] cells/μl) and nSnC (non-spontaneously HCV clearers non-HIV-elite controllers, 39 [11–169] cells/μl).
Improved Polyfunctionality of HCV- and HIV-Specific CD4 T-Cells in Supercontrollers
We analyzed the T-cell specific response defined as the frequency of cells (after background subtraction of the unstimulated condition) with detectable intracellular cytokine production after stimulation with HCV NS3, NS4A, NS4B, and Core peptide and HIV Gag peptides. CD4 HCV and HIV specific T-cell gating strategies are shown in Figure 1A. T-cells from SC exhibited the highest HCV-specific CD4 T-cell polyfunctionality, in terms of simultaneously production of IFNγ, IL2, and TNFα (Figure 1B, red portion of pie chart). Although the overall distribution of cytokine production was not statistically significant, when we analyzed the proportion of CD4 T-cells expressing IFNγ, IL2, and TNFα (3 functions) at the same time in response to HCV, the highest levels were shown in SC group (Figure 1C). Interestingly, this cell subset was higher in SC than SnC, the group of patients that spontaneously clear the HCV. When the HIV-specific CD4 T-cell response was analyzed, a higher polyfunctionality was present in HIV-controllers, independently of HCV clearance (SC and nSC groups) (Figure 1D, red portions). Also, the frequency of HIV specific CD4 T-cells producing the combination IFNγ+IL2+TNFα- was higher in the HIV-controller groups (SC and nSC) respect non-HIV- controller groups (SnC and nSnC) (Figure 1E). The CD4 and CD8 T-cell polyfunctionality index (pINDEX) in response to HCV positively correlated with the CD8 T-cell pINDEX in response to HIV (r = 0.364, p = 0.019; and r = 0.441, p = 0.004, respectively) (Figure 1F), reinforcing the idea of the better T-cell response to HCV corresponded with a better T-cell response to HIV. When CD4 T-cell cytotoxicity was analyzed, assessed by CD107a and Granzyme B production in any combination with other cytokine, no differences were observed in response to HCV and HIV stimulation (data not shown). Interestingly, controllers (SC and nSC) exhibited higher CD107a+GranzymeB-IFNg-IL2-TNFa- CD4 HIV-specific T-cells than non-controllers (Supplementary Figure 1). HCV- and HIV-specific CD8 T-cell response was also analyzed (Supplementary Figure 2). Gating strategies are summarized in Supplementary Figure 2A. No significant differences in HCV- and HIV-specific CD8 T-cell response among groups were observed (Supplementary Figure 2B). We observed differences in some cytokine combination, such as higher proportion of IFNγ+IL2-TNFα+ CD8 HCV-specific T-cells of SC compared with SnC and nSnC (p = 0.025 and 0.049, respectively) (Supplementary Figure 2C). Interestingly, we observed a trend to higher CD8 HIV-specific T-cells polyfunctionality (Supplementary Figure 2D) and higher proportion of IFNγ+IL2-TNFα+ and IFNγ+IL2+TNFα+ CD8 HIV-specific T-cells (Supplementary Figure 2E) in nSC group than SnC and nSnC.
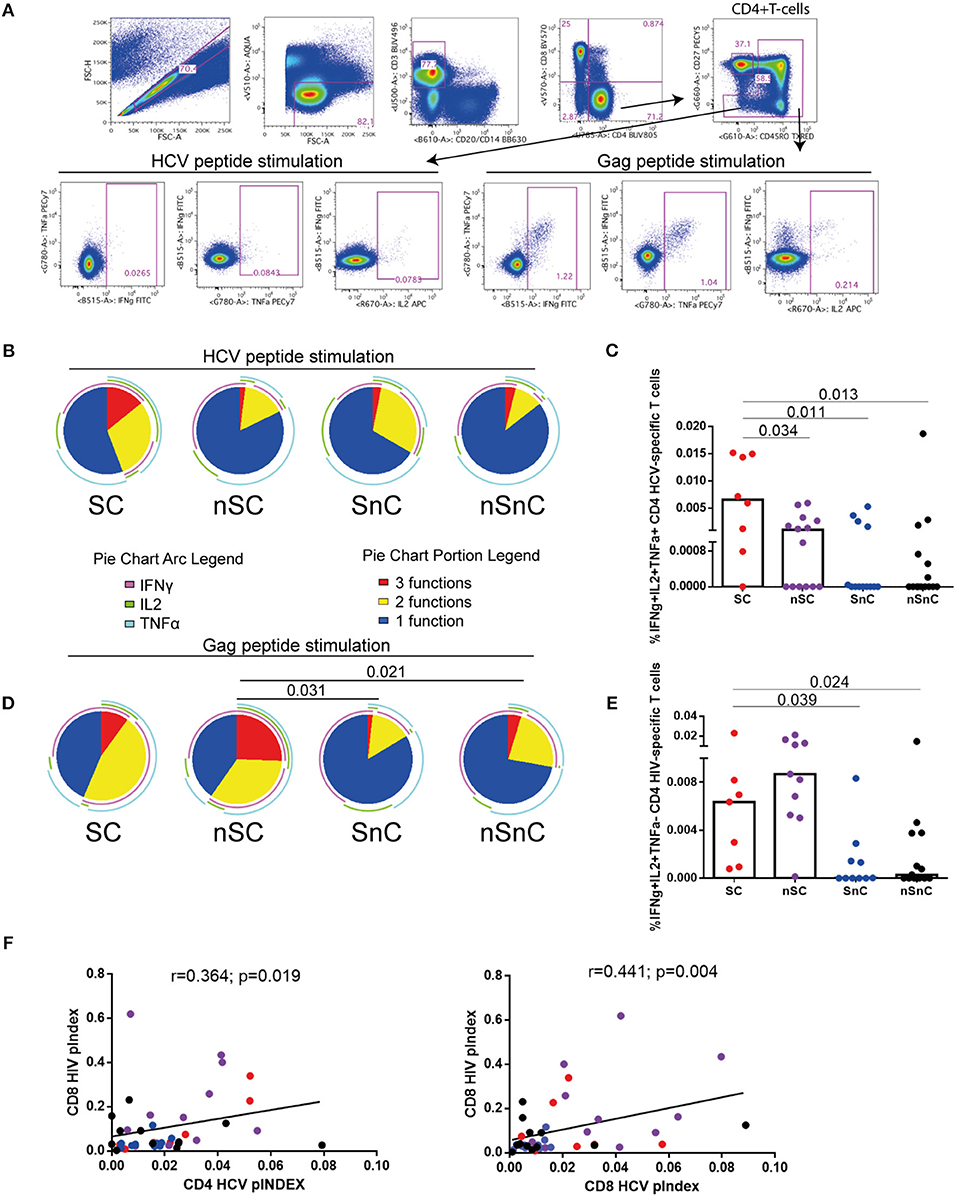
Figure 1. HCV- and HIV- CD4 specific T-cells. (A) gating stratregy. (B) HCV-specific CD4 T-cell polyfunctionality pie charts. Permutation test, following the Spice version 5.2 software was used to assess diferencies between pie charts. (C) Cytokines combinations from HCV-specific CD4 T-cell. Mann–Whitney U-tests was used to assess diferencies between groups. (D) HIV-specific CD4 T-cell polyfunctionality pie charts. Permutation test, following the Spice version 5.2 software was used to assess diferencies between pie charts. (E) Cytokines combinations from HIV-specific CD4 T-cell. Mann–Whitney U-tests was used to assess diferencies between groups. (F) Spearman correlations between CD4 and CD8 HCV-specific T-cells polyfunctionality index and CD8 HIV-specific T-cells polyfunctionality index. SC: supercontrollers, HCV spontaneous clearers and HIV-elite controllers; nSC: non-spontaneously HCV clearers HIV-elite controllers; SnC: HCV spontaneous clearers non-HIV-elite controllers; nSnC: non-spontaneously HCV clearers non-HIV-elite controllers.
Low Levels of T-Cell Exhaustion in HIV-Controllers Independently of HCV Clearance
To determine whether HCV-specific CD4 T-cell polyfunctionality was associated to lower T-cell exhaustion we quantified the expression of the exhaustion markers Lag3, PD1, TIGIT, and Tim3 in CD4 and CD8 memory T-cell subsets (gating strategy is shown in Supplementary Figure 3). The “multiple exhausted phenotype” (simultaneous expressions of three or more of the analyzed exhaustion markers) was represented by pie charts. SC exhibited low multiple exhausted phenotypes in both CD4 and CD8 T-cells, in all memory subpopulations. These levels were similar to the other HIV-controller group, nSC (Figures 2A,B). This trend was observed when specific combinations of exhaustion markers were analyzed in each group (Figure 2C). Regarding CD8 T-cell subsets similar patterns to CD4 T-cell subsets were shown when exhaustion marker combinations were analyzed (Figure 2D).
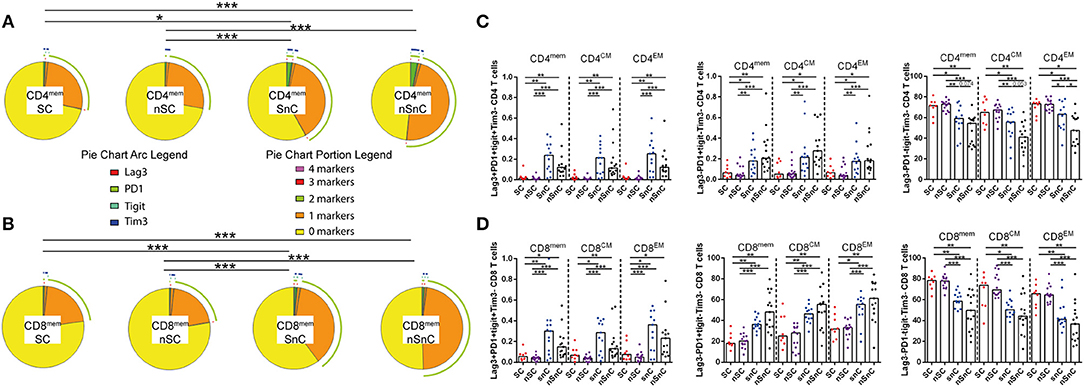
Figure 2. T-cell exhaustion. (A) multiple exhaustion phenotype from CD4 memory T-cells pie charts. Permutation test, following the Spice version 5.2 software was used to assess diferencies between pie charts. (B) multiple exhaustion phenotype from CD8 memory T-cells pie charts. Permutation test, following the Spice version 5.2 software was used to assess diferencies between pie charts. (C) Combinations of exhaustion markers within CD4 memory subsets. Mann–Whitney U-tests was used to assess diferencies between groups. (D) Combinations of exhaustion markers within CD8 memory subsets. Mann–Whitney U-tests was used to assess diferencies between groups. mem, total memory; CM, central memory; EM, effector memory. SC, supercontrollers, HCV spontaneous clearers and HIV-elite controllers; nSC, non-spontaneously HCV clearers HIV-elite controllers; SnC, HCV spontaneous clearers non-HIV-elite controllers; nSnC, non-spontaneously HCV clearers non-HIV-elite controllers. Statistical values are shown as: *p = 0.05–0.01; **p = 0.01–0.001; ***p < 0.001.
T-Cell Activation and CD161high CD8 T-Cell Correlated With HCV and HIV T-Cell Response
T-cell activation was assessed by HLA-DR expression both in CD4 and CD8 T-cell subpopulations. SC presented lower CD4 HLA-DR+ T-cell levels (Figure 3A) and CD8 HLA-DR T-cells (Figure 3B) in every subpopulation studied and, similarly to T-cell exhaustion, at the same levels than the other HIV controller group (nSC). Interestingly, this activated phenotype of CD8 and CD4 central and effector memory T-cells showed a common pattern of negative correlations with CD4 and CD8 HCV-and HIV-specific T-cell responses (Figure 3C). In addition, the chemokine receptor CXCR3, involved in T-cell trafficking to inflamed tissue (21) was also analyzed in CD4 and CD8 T-cells. We did not found differences in CXCR3 expression in any memory subset, neither in CD4 nor CD8 T-cell (data not shown). Unexpectedly, we observed lower levels of CXCR3+ naïve CD4 and CD8 T-cells in HIV-controllers (SC and nSC) compared with non-HIV controllers (SnC and nSnC) (Figures 4A,B).
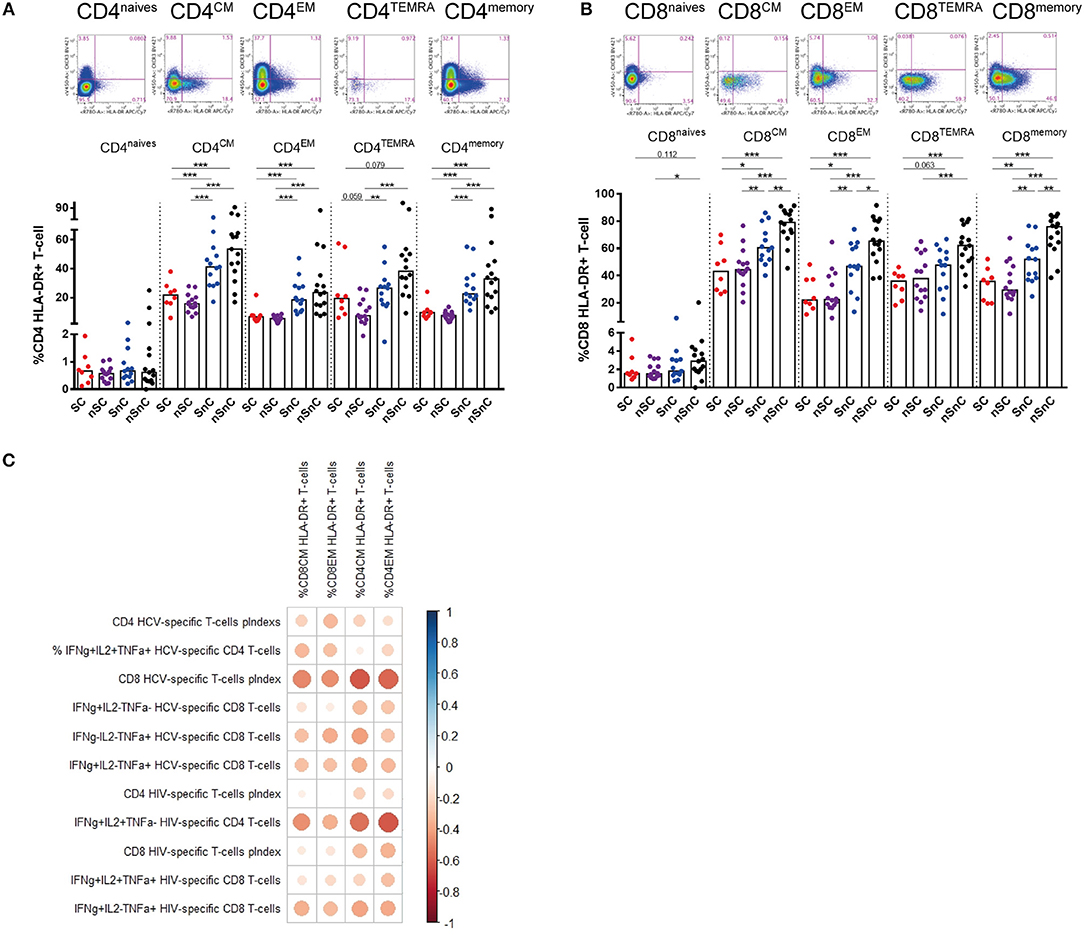
Figure 3. T-cell activation. (A) Frequency of CD4 T-cell subsets expressing HLA-DR. Mann–Whitney U-tests was used to assess diferencies between groups. (B) Frequency of CD8 T-cell subsets expressing HLA-DR. Mann–Whitney U-tests was used to assess diferencies between groups. (C) Pearson correlation between CD4 and CD8 expressing HLA-DR and HCV- and HIV-specific T-cell responses. mem, memory total; CM, central memory; EM, effector memory; TEMRA, terminally differenciated. SC, supercontrollers, HCV spontaneous clearers and HIV-elite controllers; nSC, non-spontaneously HCV clearers HIV-elite controllers; SnC, HCV spontaneous clearers non-HIV-elite controllers; nSnC, non-spontaneously HCV clearers non-HIV-elite controllers. Statistical values are shown as: *p = 0.05–0.01; **p = 0.01–0.001; ***p < 0.001. The ball size corresponds with the magnitude of Pearson's r-value.
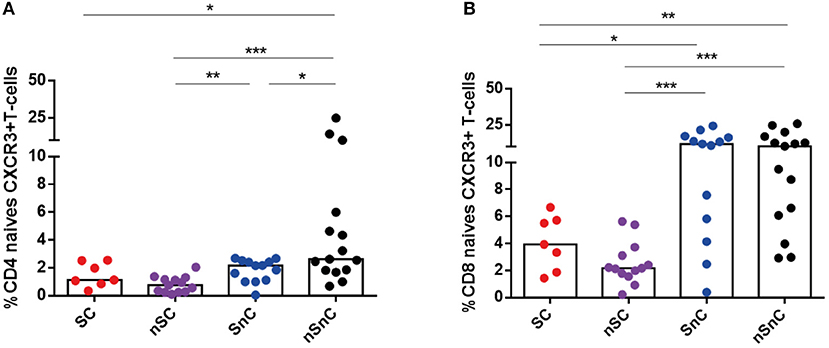
Figure 4. CXCR3 expression in naive CD4 and CD8 T-cells. (A) Frequency of naive CD4 CXCR3+ T-cells. (B) Frequency of naive CD8 CXCR3+ T-cells. One outlyer SC and one nSC were excluded. SC, supercontrollers, HCV spontaneous clearers and HIV-elite controllers; nSC, non-spontaneously HCV clearers HIV-elite controllers; SnC, HCV spontaneous clearers non-HIV-elite controllers; nSnC, non-spontaneously HCV clearers non-HIV-elite controllers. Mann–Whitney U-tests was used to assess diferencies between groups. Statistical values are shown as: *p = 0.05–0.01; **p = 0.01–0.001; ***p < 0.001.
The expression of the C-type lectin CD161 was also analyzed in CD4 and CD8 T-cells. No significant differences in CD161 expression of CD4 T-cells were found among groups (data not shown). Higher frequency of CD161high CD8 T-cell was presented in HIV-controllers, SC and nSC, compared with non-HIV controllers, SnC and nSnC (Figure 5A). Notably, we observed higher frequency of CD161high CD8 effector memory T-cells in SnC than nSnC. This peculiar CD8 T-cell phenotype from central and effector memory subsets strongly correlated with HCV-and HIV-specific CD4 and CD8 T-cell responses (Figure 5B).
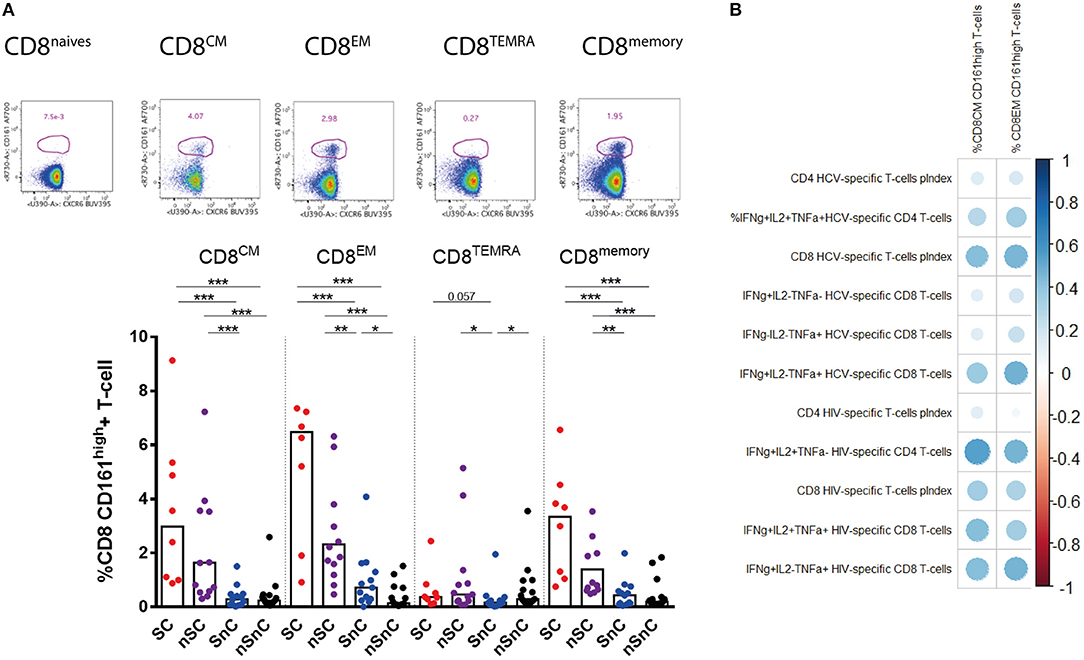
Figure 5. CD161high CD8 T-cells. (A) Frecuency of CD161high among CD8 T-cells subsets. Mann–Whitney U-tests was used to assess diferencies between groups. (B) Pearson correlation between CD161high CD8 T-cells and HCV- and HIV-specific T-cell responses. SC, supercontrollers, HCV spontaneous clearers and HIV-elite controllers; nSC, non-spontaneously HCV clearers HIV-elite controllers; SnC, HCV spontaneous clearers non-HIV-elite controllers; nSnC, non-spontaneously HCV clearers non-HIV-elite controllers. Statistical values are shown as: *p = 0.05–0.01; **p = 0.01–0.001; ***p < 0.001. The ball size correspond with the magnitude of Pearson's r-value.
Innate Immune Cells Involvement in HIV Control and HCV Spontaneous Clearance
We then ought to analyze the association of innate immunity in the supercontroller phenotype. The gating strategies for different dendritic cell subsets are summarized in Figure 6A. As expected, higher frequency of pDCs were found in HIV-controllers (SC and nSC) compared with non-controllers (SnC and nSnC), independently of HCV spontaneous clearance (Figure 6B). No differences in mDCs were found among groups (data not shown). Interestingly, there was a trend to show higher frequency of pDCs expressing the lymph node homing marker CCR7 in SC (Figure 6C). Furthermore, positive correlations were found between frequency of pDCs and HCV-specific CD4 T-cell pINDEX (r = 0.802, p < 0.001) (Figure 6D) and frequency of IFNγ+IL2+TNFα+ CD4 HCV-specific T-cells (the combination shown in Figure 1C) (r = 0.571, p = 0.013) (Figure 6E). Also, positive correlations were found between pDCs levels and HCV-specific CD8 T-cell pINDEX (r = 0.585, p = 0.011) (Figure 6F) and frequency of IFNγ+IL2-TNFα+ HIV-specific CD8 T-cells (r = 0.515, p = 0.029) (Figure 6G).
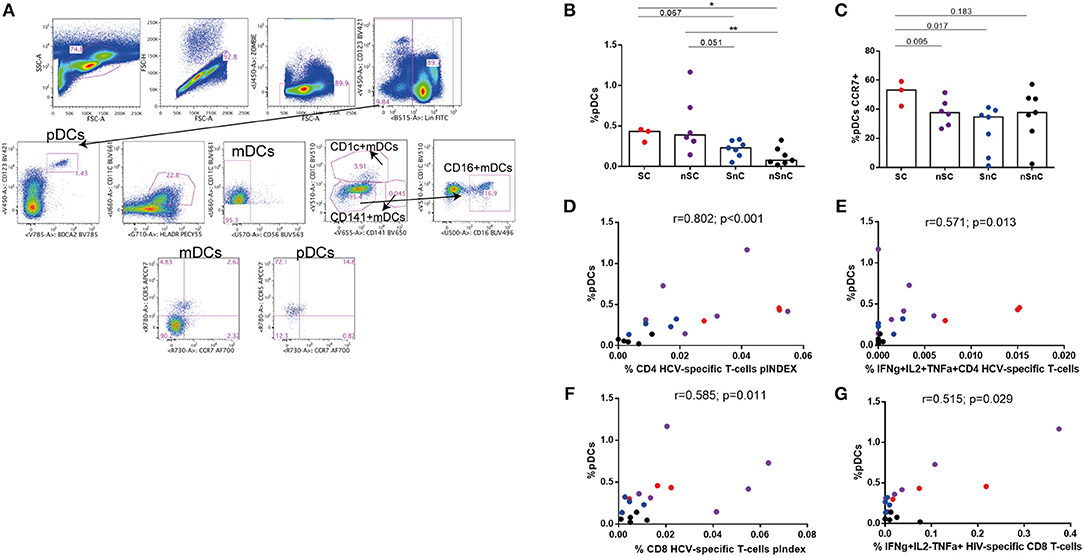
Figure 6. Dendritic cells. (A) Gating strategy. (B) Plasmacytoid dendritic cells (pDCs) frequency. Mann–Whitney U-tests was used to assess diferencies between groups. (C) CCR7 expresing pDCs. Mann–Whitney U-tests was used to assess diferencies between groups. (D-G) Spearman correlations between pDCs frequency and HCV- and HIV-specific T-cell responses. SC, supercontrollers, HCV spontaneous clearers and HIV-elite controllers; nSC, non-spontaneously HCV clearers HIV-elite controllers; SnC, HCV spontaneous clearers non-HIV-elite controllers; nSnC, non-spontaneously HCV clearers non-HIV-elite controllers. Statistical values are shown as: *p = 0.05–0.01; **p = 0.01–0.001; ***p < 0.001.
Regarding NK cells, we also observed higher levels of NK CD56dim cells among HIV-controller group, SC and nSC (Figure 7A). No differences of NK CD56high cells were found among groups (Figure 7B). In line with the results for CD4 and CD8 T-cell activation, HIV controller groups, SC and nSC, presented lower levels of NK CD56dim cells expressing HLA-DR (Figure 7C), likewise some exhaustion markers such as TIGIT (Figure 7D) and Lag3 (Figure 7E), and CXCR6 (Figure 7F). Gating strategies of NK cells are shown in Supplementary Figure 4.
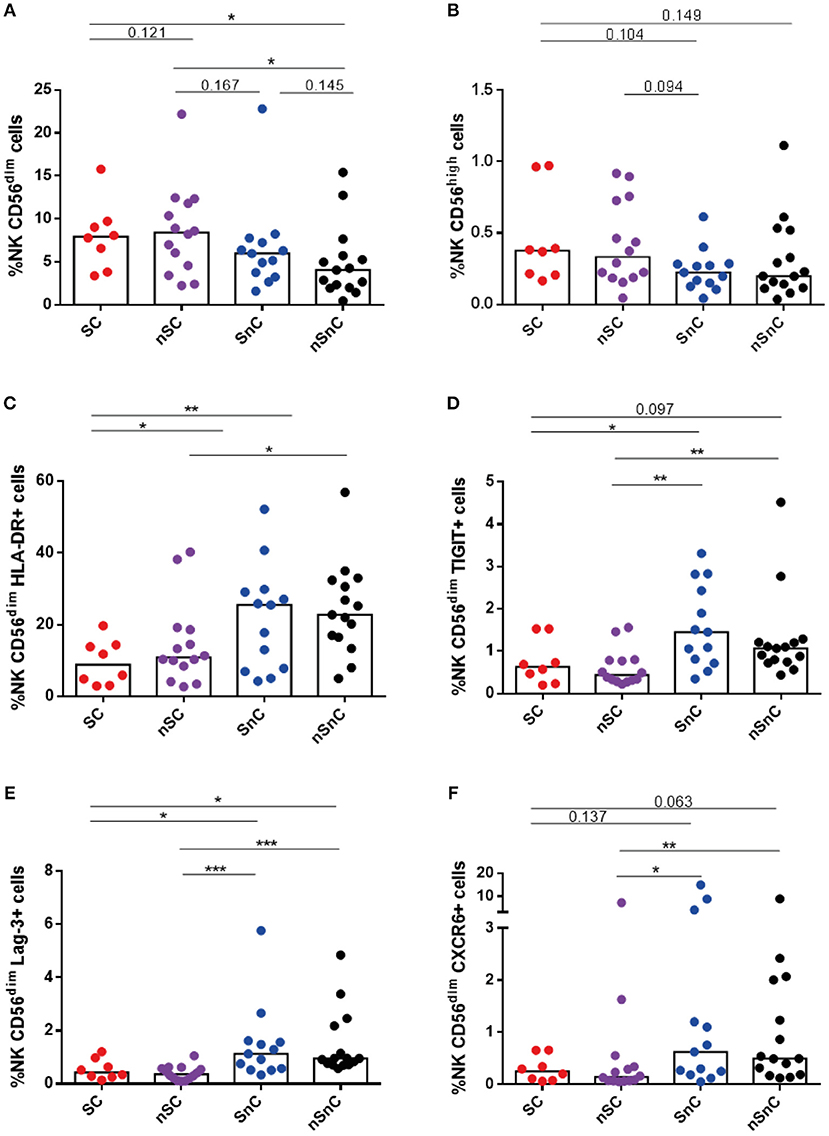
Figure 7. NK cels. (A) NK CD56dim cells frequency and (B) NK CD56high cells frequency. (C–E) NK CD56dim cells expressing HLA-DR, Lag-3 and TIGIT. (F) NK CD56dim cells expressing CXCR6. SC, supercontrollers, HCV spontaneous clearers and HIV-elite controllers; nSC, non-spontaneously HCV clearers HIV-elite controllers; SnC, HCV spontaneous clearers non-HIV-elite controllers; nSnC, non-spontaneously HCV clearers non-HIV-elite controllers. Mann–Whitney U-tests was used to assess diferencies between groups. Statistical values are shown as: *p = 0.05–0.01; **p = 0.01–0.001; ***p < 0.001.
Discussion
HIV-elite controllers that spontaneously clear HCV (supercontrollers) are extremely difficult to investigate, as they are very uncommon. Considering that about 1% of patients are able to control HIV (22), and 10–20% of infected patients spontaneously clear the HCV (23), the proportion of patients that control both viruses is very low (we estimates < 0.2%). Herein, we have described for the first time the immunological features of these abovementioned supercontrollers, consisting in high CD4 HCV-specific T-cell polyfunctionality together with low exhausted and activated phenotype of T- and innate immunity cells.
Virus specific CD4 T-cells have an important role in controlling viral infections, not only by helping the CD8 T-cell cytotoxic activity and protective antibodies production by B cells, but also exercising antiviral functions (24, 25). We have found higher HCV-specific CD4 T-cell polyfunctionality in subjects that control HIV and clear HCV at the same time. That was in line with the only study of HCV T-cell response within HIV-controllers, in which the authors found a strong Th1 response against HCV (26). This study was performed with chronic HCV patients, not with HCV clearers, but an inverse correlation between Th1 frequency specific for HCV core protein and HCV VL was found (26). That emphasized the importance of CD4 T-cells in HCV control within HIV-controllers.
Interestingly, a higher, broadly and long lasting HCV-specific CD4 T-cell response has been associated with spontaneous clearance of HCV compared with chronic HCV patients (27–32). Also remarkably, these studies found broad CD4 T-cell responses to HCV non-structural protein NS3 and NS4 in HCV clearers, peptides that we have included in our study in the peptide pool for stimulations. Furthermore, Shulze et al. demonstrated that peptide recognized by CD4 T-cells from HCV spontaneous clearers were highly promiscuous, which can be restricted by multiple MHC-II molecules (32). Curiously, same phenomenon seems to occur with Gag peptide restriction in HIV-controllers, which same peptide can be restricted by up to five HLA-DR allomorphs to one T-cell receptor (33). Actually, several studies have associated some HLA-DRB1 alleles (34–36) and HLA-DQB1 (34, 37, 38) with HCV spontaneous clearance. Unfortunately, because of samples unavailability after flow cytometry experiments we were unable to test HLA-II typing. However, due to the small sample size this important immune correlate of virus control may be confirmed in larger cohorts of supercontrollers.
On the other hand, CD4 T-cell response were also related with HIV spontaneous control (39). It is known that HIV-controllers exhibit more vigorous, proliferative and polyfunctional CD4 HIV-specific T-cell responses than non-controller patients, even after the antiretroviral treatment onset (40–44). Furthermore, Potter et al. showed that CD4 T-cells from HIV-elite controllers were enriched of central memory, which expressed higher levels of CD127. This fact could contribute to a long-term antiviral memory activity in these patients (44), probably including HIV- and HCV-specific response. In our experiments, the CD4 HIV-specific response was similar in both HIV controller groups.
We did not observe significant differences in polyfunctionality of HCV-specific CD8 T-cell response among groups. It has been demonstrated that HCV-specific CD8 T-cell in chronic patients is difficult to find in peripheral blood, due to liver accumulation of these cells (45). Of note, although both SC and SnC spontaneously cleared the HCV, a higher IFNγ and TNFα HCV-specific CD8 T-cell production were found in patients that control HIV and clear HCV. However, we highlight the importance of the CD4 HCV-specific polyfunctionality found in SC. First, because a HCV-specific CD8 T-cell activity is known not to be enough to mount a protective response, as it has been demonstrate in the setting of HCV vaccines (46), probably because of the high scape mutation rate in this virus (47, 48). And second, because the importance of developing adequate CD4 T-cell response over a CD8 T-cell has been demonstrated in an elegant experiment with the HCV chimpanzee model in which Grakoui et al. depleted CD4 T-cells from animals that previously cleared the HCV, and then they reinfected them with HCV thus, demonstrating that despite of having memory HCV-specific CD8 T-cells, it was not enough to control the virus scape mutations onset (47).
T-cell exhaustion and activation are hallmarks of the HIV infection (49, 50). Interestingly, SC exhibited low levels of both measurements. Interestingly, CD4+ T-cell expressing PD1, Lag-3 and TIGIT has been strongly correlated with HIV reservoir size and HIV persistence (50). Maybe, this extraordinary group of controllers is enriched in patients with in addition to HCV spontaneous eradication, have minimal amount of integrated HIV. Unfortunately, we could not measure HIV reservoir because of lack of samples. Further analyses in this regard should be done. In this sense, Banga et al. argue that CD4 T-cell expressing CXCR3 represent the major blood compartment with HIV proviral population (51). No differences in CXCR3 expression in CD4 and CD8 T-cell memory subsets were found, but we observed lower frequency of naïve CD4 and CD8 T-cell expressing CXCR3 in HIV-controllers. This molecule is involved in T-cell homing to inflamed peripheral tissue after maturation (21). We did not expect to find CXCR3 expression in naive T-cells but although the expression were low (< 5% in HIV-controller groups), we observed differences with the non-controllers groups. We do not have a plausible explanation for this fact; maybe this is putative marker of lower inflammation/activation levels in HIV-controllers.
Interestingly, we found higher frequency of CD161high CD8 T-cells in HIV-controllers, with a trend to high levels in SC. The significance of CD161 expression in CD8 T-cell is controversial (52). It has been suggested that CD161high CD8 T-cell population represents a memory stem cell subset (53), whereas other authors argue that these cells are IL-17 producing CD8 T-cells (54, 55). Our results suggest that this CD8 T-cell subset could be involved somehow with the antiviral response (56), as they positively correlate with HIV- and HCV-specific T-cell responses.
Innate immunity also has a major role on viral control in both HIV and HCV (57, 58). We have previously communicated that pDCs are related with HIV spontaneous control (13, 14), and other authors showed higher responsiveness of pDCs from HCV spontaneous clearers (16). In this work we found higher pDCs frequency in HIV-controllers compared with non-controllers and interestingly, a trend to higher CCR7 expression of pDCs in SC. CCR7 is a homing molecule that enable pDC migrate to lymph nodes (59) and prime CD4 and CD8 T-cells (60). This is in agreement with the correlation found between pDCs and HIV- and HCV-specific T-cell levels. On the other hand, NK cells also play an important role in HIV control (9, 10) and HCV clearance (11, 12). Similarly to T-cells, HIV-controllers showed lower activation and exhaustion of CD56dim NK cells compare with non-controllers, the high cytotoxic NK subset (61).
In conclusion, CD4 T-cell response seems to be the most differential feature in SC. Furthermore, this extraordinary group of subjects exhibited low levels of T-cell exhaustion and activation. Other T-cell subsets, as CD161high were interestingly related with HIV and HCV-specific T-cell responses, as well as pDCs. Further analyses with a large cohort of SC must be done to deeply analyze HIV- and HCV-specific T-cell phenotype and the correlates with the control of both viruses in relation with other players of adaptive and innate immunity. The study of these patients could serve as a model for the development of therapeutic strategies aimed to enhance antiviral responses.
Author Contributions
BD-M designed and performed experiments, analyzed and interpreted the data, designed the figures, and wrote the manuscript. SF-M and LT-D participated in the methodology and data analysis. JH-Q, MG, FV, and ML collaborated with the patient's characterization and samples collection. ML, MM-F, and RK supervised all the experimental procedures. ER-M and ML conceived and designed the study. ER-M interpreted the data and wrote the manuscript. All the authors critically reviewed, edited and approved the final manuscript.
Funding
This work was supported by the Instituto de Salud Carlos III: research projects PI12/02283 and PI16/00684 and research contracts CPII014/00025 to ER-M, and FI14/00431 to LT-D; and also by Red Temática de Investigación Cooperativa en SIDA (RD12/0017/0029, RD16/0025/0020, RD16/0025/0019 and RD16/0025/0006), which is included in the Acción Estratégica en Salud, Plan Nacional de Investigación Científica, Desarrollo e Innovación Tecnológica 2008–2011, Instituto de Salud Carlos III, Fondos FEDER. This study was also supported by Gilead Fellowships, Gilead Science Inc. grant GLD17/00299. ER-M was supported by Consejería de Salud y Bienestar Social of Junta de Andalucía through the Nicolás Monardes program (C-0032/17). BD-M received a grant from The Spanish Ministry of Education (FPU13/02451). Peptides Array from Hepatitis C Virus, H77 NS4A, NS4B, NS3, and Core were obtained through the NIH Biodefense and Emerging Infections Research Resources Repository, NIAID, NIH.
This study would not have been possible without the collaboration of all of the patients, medical and nursery staff, and data managers who have taken part in the project. We particular acknowledge the cytometry core from VRC, NIH, Bethesda, which help in the cytometry panels development.
Conflict of Interest Statement
The authors declare that the research was conducted in the absence of any commercial or financial relationships that could be construed as a potential conflict of interest.
Supplementary Material
The Supplementary Material for this article can be found online at: https://www.frontiersin.org/articles/10.3389/fimmu.2018.02897/full#supplementary-material
References
1. Madec Y, Boufassa F, Rouzioux C, Delfraissy J-F, Meyer L. Undetectable viremia without antiretroviral therapy in patients with HIV seroconversion: an uncommon phenomenon? Clin Infect Dis. (2005) 40:1350–4. doi: 10.1086/429318
2. Lambotte O, Boufassa F, Madec Y, Nguyen A, Meyer L, Rouzioux C, et al. HIV Controllers : a homogeneous group of hiv-1 – infected patients with spontaneous control of viral replication. Clin Infect Dis. (2005) 41:1053–6. doi: 10.1086/433188
3. Jacques Thèze. HIV controllers: a multifactorial phenotype of spontaneous viral suppression. (2012) 141:15–30. doi: 10.1016/j.clim.2011.07.007.HIV
4. Deeks SG, Walker BD. Human immunodeficiency virus controllers: mechanisms of durable virus control in the absence of antiretroviral therapy. Immunity (2007) 27:406–16. doi: 10.1016/j.immuni.2007.08.010
5. Ruiz-Mateos E, Machmach K, Romero-Sánchez MC, Ferrando-Martínez S, Viciana P, Del Val M, et al. Hepatitis C virus replication in Caucasian HIV controllers. J Viral Hepat. (2011) 18:350–357. doi: 10.1111/j.1365-2893.2010.01431.x
6. Sajadi MM, Shakeri N, Talwani R, Redfield RR. Hepatitis C Infection in HIV-1 Natural Viral Suppressors. AIDS (2010) 24:1689–95. doi: 10.1097/QAD.0b013e32833a2a32
7. Holz L, Rehermann B. T cell responses in hepatitis C virus infection: Historical overview and goals for future research. Antiviral Res. (2015) 114:96–105. doi: 10.1016/j.antiviral.2014.11.009
8. Walker B, McMichael A. The T-cell response to HIV. Cold Spring Harb Perspect Med. (2012) 2:a007054. doi: 10.1101/cshperspect.a007054
9. Tomescu C, Duh F-M, Hoh R, Viviani A, Harvill K, Martin MP, et al. Impact of Protective KIR/HLA genotypes on nk cell and t cell function in hiv-1 infected controllers costin. AIDS (2012) 26:1713–23. doi: 10.1109/TMI.2012.2196707.Separate
10. Taborda NA, González SM, Alvarez CM, Correa LA, Montoya CJ, Rugeles MT. et al. Higher frequency of NK and CD4+ T-cells in mucosa and potent cytotoxic response in HIV controllers. PLoS ONE (2015) 10:1–15. doi: 10.1371/journal.pone.0136292
11. de Groen RA, Groothuismink ZMA, van Oord G, Kootstra NA, Janssen HLA, Prins M, et al. NK cells in self-limited HCV infection exhibit a more extensively differentiated, but not memory-like, repertoire. J Viral Hepat. (2017) 24:917–26. doi: 10.1111/jvh.12716
12. Alter G, Jost S, Rihn S, Reyor LL, Nolan BE, Ghebremichael M, et al. Reduced frequencies of NKp30+NKp46+, CD161+, and NKG2D+ NK cells in acute HCV infection may predict viral clearance. J Hepatol. (2011) 55:278–88. doi: 10.1016/j.jhep.2010.11.030
13. Barblu L, Machmach K, Gras C, Delfraissy J-F, Boufassa F, Leal M, et al. Plasmacytoid dendritic cells (pDCs) from HIV controllers produce interferon-α and differentiate into functional killer pDCs under HIV activation. J Infect Dis. (2012) 206:790–801. doi: 10.1093/infdis/jis384
14. Machmach K, Leal M, Gras C, Viciana P, Genebat M, Franco E, et al. Plasmacytoid dendritic cells reduce HIV production in elite controllers. J Virol. (2012) 86:4245–52. doi: 10.1128/JVI.07114-11
15. Huang J, Burke PS, Cung TDH, Pereyra F, Toth I, Walker BD, et al. Leukocyte immunoglobulin-like receptors maintain unique antigen-presenting properties of circulating myeloid dendritic cells in HIV-1-infected elite controllers. J Virol. (2010) 84:9463–71. doi: 10.1128/JVI.01009-10
16. Pelletier S, Said E, Ancuta P, Bruneau J, Shoukry NH, Sciences H, et al. Sustained hyperresponsiveness of dendritic cells is associated with spontaneous resolution of acute hepatitis C. J Virol. (2013) 87:6769–81. doi: 10.1128/JVI.02445-12
17. Auffermann-Gretzinger S, Keeffe EB, Levy S. Impaired dendritic cell maturation in patients with chronic, but not resolved, hepatitis C virus infection. Blood (2001) 97:3171–6. doi: 10.1182/blood.V97.10.3171
18. Ferrando-martínez S, Casazza JP, Leal M, Machmach K, Muñoz-fernández MÁ. Differential gag-specific polyfunctional T cell maturation patterns in HIV-1 elite controllers. J Virol. (2012) 86:3667–3674. doi: 10.1128/JVI.07034-11
19. RCT. R: A Language and Environment for Statistical Computing. Vienna: R Foundation for Statistical Computing. Available online at: https://www.R-project.org/
20. Larsen M, Sauce D, Arnaud L, Fastenackels S, Appay V, Gorochov G. Evaluating cellular polyfunctionality with a novel polyfunctionality index. PLoS ONE (2012) 7:1–10. doi: 10.1371/journal.pone.0042403
21. Groom JR, Luster AD. CXCR3 in T cell function. Exp Cell Res. (2011) 317:620–31. doi: 10.1016/j.yexcr.2010.12.017
22. Madec Y, Boufassa F, Porter K, Prins M, Sabin C, d'Arminio Monforte A, et al. Natural history of HIV-control since seroconversion. AIDS (2013) 27:2451–60. doi: 10.1097/01.aids.0000431945.72365.01
23. Axley P, Ahmed Z, Ravi S, Singal AK. Hepatitis C virus and hepatocellular carcinoma: a narrative review. J Clin Transl Hepatol. (2018) 6:1–6. doi: 10.14218/JCTH.2017.00067
24. Sant AJ, McMichael A. Revealing the role of CD4(+) T cells in viral immunity. J Exp Med. (2012) 209:1391–5. doi: 10.1084/jem.20121517
25. Swain SL, Mckinstry KK, Strutt TM. Expanding roles for CD4 + T cells in immunity to viruses. Nat Rev Immunol. (2012) 12:136–48. doi: 10.1038/nri3152
26. Alatrakchi N, Di Martino V, Thibault V, Autran B. Strong CD4 Th1 responses to HIV and hepatitis C virus in HIV-infected long-term non-progressors co-infected with hepatitis C virus. AIDS (2002) 16:713–7. doi: 10.1097/00002030-200203290-00006
27. Diepolder HM, Zachoval R, Hoffmann RM, Jung M-C, Pape GR, Wierenga EA, et al. Possible mechanism involving T-lymphocyte response to non-structural protein 3 in viral clearance in acute hepatitis C virus infection. Lancet (1995) 346:1006–7. doi: 10.1016/S0140-6736(95)91691-1
28. Diepolder H, Gerlach J, Zachoval R, Hoffmann R, Jung M, Wierenga E, et al. Immunodominant CD4+ T-cell epitope within nonstructural protein 3 in acute hepatitis C virus infection. J Virol. (1997) 71:6011–9.
29. Day CL, Lauer GM, Robbins GK, McGovern B, Wurcel AG, Gandhi RT, et al. Broad specificity of virus-specific CD4+ T-helper-cell responses in resolved hepatitis C virus infection. J Virol. (2002) 76:12584–95. doi: 10.1128/JVI.76.24.12584-12595.2002
30. Gerlach JT, Diepolder HM, Jung M, Gruener NH, Schraut WW, Zachoval R, et al. Recurrence of hepatitis C virus after loss of virus-specific CD4+ T-cell response in acute hepatitis C. Gastroenterology (1999) 117:933–41. doi: 10.1016/S0016-5085(99)70353-7
31. Thimme R, Oldach D, Chang K-M, Steiger C, Ray SC, Chisari FV. Determinants of Viral Clearance and Persistence during Acute Hepatitis C Virus Infection. J Exp Med. (2001) 194:1395–406. doi: 10.1084/jem.194.10.1395
32. Shulze zur Wiesch J, Lauer GM, Day CL, Kim AY, Ouchi K, Duncan JE, et al. Broad Repertoire of the CD4+Th cell response in spontaneously controlled hepatitis c virus infection includes dominant and highly promiscuous epitopes. J Immunol. (2005) 175:3603–13. doi: 10.4049/jimmunol.175.6.3603
33. Galperin M, Farenc C, Mukhopadhyay M, Jayasinghe D, Decroos A, Benati D, et al. CD4+ T cell-mediated HLA class II cross-restriction in HIV controllers. Sci Immunol. (2018) 3:eaat0687. doi: 10.1126/sciimmunol.aat0687
34. Samimi-Rad K, Sadeghi F, Amirzargar A, Eshraghian MR, Alavian S-M, Rahimnia R. Association of HLA class II alleles with hepatitis C virus clearance and persistence in thalassemia patients from Iran. J Med Virol. (2015) 87:1565–72. doi: 10.1002/jmv.24211
35. Thursz M, Yallop R, Goldin R, Trepo C, Thomas HC. Influence of MHC class II genotype on outcome of infection with hepatitis C virus. Lancet (1999) 354:2119–24. doi: 10.1016/S0140-6736(99)91443-5
36. McKiernan SM, Hagan R, Curry M, McDonald GSA, Kelly A, Nolan N, et al. Distinct MHC class I and II alleles are associated with hepatitis C viral clearance, originating from a single source. Hepatology (2004) 40:108–14. doi: 10.1002/hep.20261
37. Wang JH, Zheng X, Ke X, Dorak MT, Shen J, Boodram B, et al. Ethnic and geographical differences in HLA associations with the outcome of hepatitis C virus infection. Virol J. (2009) 6:46. doi: 10.1186/1743-422X-6-46
38. Thio CL, Thomas DL, Goedert JJ, Vlahov D, Nelson KE, Hilgartner MW, et al. Racial differences in HLA class ii associations with hepatitis c virus outcomes. J Infect Dis. (2001) 184:16–21. doi: 10.1086/321005
39. Porichis F, Kaufmann DE. HIV-specific CD4 T cells and immune control of viral replication. Curr Opin HIV AIDS (2011) 6:174–80. doi: 10.1097/COH.0b013e3283454058
40. Rosenberg ES, Billingsley JM, Caliendo AM, Boswell SL, Sax PE, Kalams SA, et al. Vigorous HIV-1-specific CD4+ T cell responses associated with control of viremia. Science (1997) 278:1447–50.
41. Dyer WB, Zaunders JJ, Yuan FF, Wang B, Learmont JC, Geczy AF, et al. Mechanisms of HIV non-progression; robust and sustained CD4+ T-cell proliferative responses to p24 antigen correlate with control of viraemia and lack of disease progression after long-term transfusion-acquired HIV-1 infection. Retrovirology (2008) 5:112. doi: 10.1186/1742-4690-5-112
42. Harari A, Petitpierre S, Vallelian F, Pantaleo G. Skewed representation of functionally distinct populations of virus-specific CD4 T cells in HIV-1-infected subjects with progressive disease: changes after antiretroviral therapy. Blood (2004) 103:966–72. doi: 10.1182/blood-2003-04-1203
43. Pereyra F, Addo MM, Kaufmann DE, Liu Y, Miura T, Rathod A, et al. Genetic and immunologic heterogeneity among persons who control HIV infection in the absence of therapy. J Infect Dis. (2008) 197:563–71. doi: 10.1086/526786
44. Potter SJ, Lacabaratz C, Lambotte O, Perez-Patrigeon S, Vingert B, Sinet M, et al. Preserved central memory and activated effector memory CD4+ T-cell subsets in human immunodeficiency virus controllers: an ANRS EP36 study. J Virol. (2007) 81:13904–15. doi: 10.1128/JVI.01401-07
45. Neumann-Haefelin C, Timm J, Spangenberg HC, Wischniowski N, Nazarova N, Kersting N, et al. Virological and immunological determinants of intrahepatic virus-specific CD8+ T-cell failure in chronic hepatitis C virus infection. Hepatology (2008) 47:1824–36. doi: 10.1002/hep.22242
46. Wieland D, Thimme R. Vaccine-induced hepatitis C virus-specific CD8+ T cells do not always help. Hepatology (2016) 63:1411–4. doi: 10.1002/hep.28388
47. Timm J, Walker CM. Mutational escape of CD8+ T cell epitopes: implications for prevention and therapy of persistent hepatitis virus infections. Med Microbiol Immunol. (2015) 3:29–38. doi: 10.1007/s00430-014-0372-z
48. Bowen DG, Walker CM. Mutational escape from CD8+ T cell immunity: HCV evolution, from chimpanzees to man. J Exp Med. (2005) 201:1709–14. doi: 10.1084/jem.20050808
49. Khaitan A, Unutmaz D. Revisiting immune exhaustion during HIV infection. Curr HIV/AIDS Rep. (2011) 8:4–11. doi: 10.1007/s11904-010-0066-0
50. Jiménez VC, Wit FWNM, Joerink M, Maurer I, Harskamp AM, Schouten J, et al. T-Cell Activation independently associates with immune senescence in HIV-infected recipients of long-term antiretroviral treatment. J Infect Dis. (2016) 214:216–25. doi: 10.1093/infdis/jiw146
51. Banga R, Procopio FA, Ruggiero A, Noto A, Ohmiti K, Cavassini M, et al. Blood CXCR3+ CD4 T cells are enriched in inducible replication competent HIV in aviremic antiretroviral therapy-treated individuals. Front Immunol. (2018) 9:144. doi: 10.3389/fimmu.2018.00144
52. Fergusson JR, Fleming VM, Klenerman P, Obar JJ, Hogquist K, William S. CD161-expressing human T cells. Front Immunol. (2011) 2:36. doi: 10.3389/fimmu.2011.00036
53. Turtle CJ, Swanson HM, Fujii N, Estey EH, Riddell SR. A distinct subset of self-renewing human memory CD8+ T cells survives cytotoxic chemotherapy. Immunity (2009) 31:834–44. doi: 10.1016/j.immuni.2009.09.015
54. Dusseaux M, Martin E, Serriari N, Péguillet I, Premel V, Louis D, et al. Human MAIT cells are xenobiotic-resistant, tissue-targeted, CD161hi IL-17-secreting T cells. Blood (2011) 117:1250–9. doi: 10.1182/blood-2010-08-303339
55. Billerbeck E, Kang Y-H, Walker L, Lockstone H, Grafmueller S, Fleming V, et al. Analysis of CD161 expression on human CD8+ T cells defines a distinct functional subset with tissue-homing properties. Proc Natl Acad Sci USA. (2010) 107:3006–11. doi: 10.1073/pnas.0914839107
56. Beudeker BJB, van Oord GW, Arends JE, Schulze Zur Wiesch J, van der Heide MS, de Knegt RJ, et al. Mucosal-associated invariant T-cell frequency and function in blood and liver of HCV mono- and HCV/HIV co-infected patients with advanced fibrosis. Liver Int. (2018) 38:458–68. doi: 10.1111/liv.13544
57. Heim MH, Thimme R. Innate and adaptive immune responses in HCV infections. J Hepatol. (2014) 61:S14–25. doi: 10.1016/j.jhep.2014.06.035
58. Altfeld M, Gale M Jr. Innate immunity against HIV-1 infection. Nat Immunol. (2015) 16:554–62. doi: 10.1038/ni.3157
59. Lehmann C, Lafferty M, Garzino-demo A, Jung N, Hartmann P, Wolf JS, et al. Plasmacytoid dendritic cells accumulate and secrete interferon alpha in lymph nodes of HIV-1 patients. PLoS ONE (2010) 5:e11110. doi: 10.1371/journal.pone.0011110
60. Fonteneau J-F, Gilliet M, Larsson M, Dasilva I, Münz C, Liu Y-J, et al. Activation of influenza virus-specific CD4+ and CD8+ T cells: a new role for plasmacytoid dendritic cells in adaptive immunity. Blood (2003) 101:3520–6. doi: 10.1182/blood-2002-10-3063
Keywords: HIV, controllers, HCV, spontaneous clearance, T-cells, pDCs, NK, supercontrollers
Citation: Dominguez-Molina B, Ferrando-Martinez S, Tarancon-Diez L, Hernandez-Quero J, Genebat M, Vidal F, Muñoz-Fernandez MA, Leal M, Koup R and Ruiz-Mateos E (2018) Immune Correlates of Natural HIV Elite Control and Simultaneous HCV Clearance—Supercontrollers. Front. Immunol. 9:2897. doi: 10.3389/fimmu.2018.02897
Received: 15 August 2018; Accepted: 26 November 2018;
Published: 10 December 2018.
Edited by:
Guido Poli, Vita-Salute San Raffaele University, ItalyReviewed by:
Giulia Carla Marchetti, University of Milan, ItalySarah Rowland-Jones, University of Oxford, United Kingdom
Copyright © 2018 Dominguez-Molina, Ferrando-Martinez, Tarancon-Diez, Hernandez-Quero, Genebat, Vidal, Muñoz-Fernandez, Leal, Koup and Ruiz-Mateos. This is an open-access article distributed under the terms of the Creative Commons Attribution License (CC BY). The use, distribution or reproduction in other forums is permitted, provided the original author(s) and the copyright owner(s) are credited and that the original publication in this journal is cited, in accordance with accepted academic practice. No use, distribution or reproduction is permitted which does not comply with these terms.
*Correspondence: Ezequiel Ruiz-Mateos, ZXplcXVpZWwucnVpem1hdGVvc0BnbWFpbC5jb20=
†Present Address: Sara Ferrando-Martinez, Microbial Sciences, MedImmune LLC, Gaithersburg, MD, United States