- 1Division of Hematology & Oncology, Department of Medicine, Medical College of Wisconsin, Milwaukee, WI, United States
- 2Biology Department, Viterbo University, La Crosse, WI, United States
Graft-vs.-host disease (GVHD) remains a major obstacle to the success of allogeneic hematopoietic stem cell transplantation (HSCT). GVHD occurs because donor T cells in the allograft recognize the genetically disparate host as foreign and attack the transplant recipient's tissues. While genetic incompatibility between donor and recipient is the primary determinant for the extent of alloimmune response, GVHD incidence and severity are also influenced by non-genetic factors. Recent advances in immunology establish that environmental factors, including dietary micronutrients, contribute significantly to modulating various immune responses and may influence the susceptibility to autoimmune and inflammatory diseases of experimental animals and humans. Emerging clinical and preclinical evidence indicates that certain micronutrients may participate in regulating GVHD risk after allogeneic HSCT. In this review, we summarize recent advances in our understanding with respect to the potential role of micronutrients in the pathogenesis of acute and chronic GVHD, focusing on vitamins A and D.
Micronutrients and Immunity
Micronutrients are compounds that are only needed in small amounts, yet are essential for the proper growth and development of the human body. These vitamins and minerals are indispensible for the production and function of various enzymes and hormones that are critical for maintaining optimal physical and mental function. An aberrant micronutrient status contributes to the increased susceptibility to various infectious, inflammatory, and metabolic conditions such as colitis, diabetes, cancer, obesity, and cardiovascular disease.
The importance of the micronutrients vitamins A and D in health has been recognized since the early twentieth century. More recent advances have led to the discovery of the critical role of these molecules in the immune system (1). Current highlights within this field include the finding that maternal vitamin A levels significantly influence the proper development of secondary lymphoid organs in offspring and determine the fitness of their immune system in later life (2). Lack of vitamin A-mediated signaling in utero substantially reduced the anti-pathogen immune response of newborn mice (2). Similarly, vitamin D also plays a role at the maternal-fetal interface, preventing inflammatory responses such as pre-eclampsia (3). These immunomodulatory effects may be long lasting as maternal vitamin D deficiency has been shown to contribute to a greater likelihood of atopic responses in the neonatal lung (4, 5). These findings reveal how nutritional status during fetal life can profoundly affect immune responses in adulthood, highlighting the importance of vitamins A and D in the development and maintenance of a competent, yet tightly regulated immune system.
Graft-VS.-Host Disease (GVHD) and Nutritional Factors
GVHD remains a major obstacle limiting the broader application of allogeneic hematopoietic stem cell transplantation (HSCT), an effective treatment for a number of malignant and non-malignant hematological disorders (6–8). GVHD is the consequence of a normal, yet exaggerated, immune reaction elicited by donor T cells when they encounter alloantigens expressed by the transplant recipient. Acute GVHD (aGVHD) pathophysiology is characterized by strong inflammatory components while chronic GVHD (cGVHD) displays more autoimmune manifestations (9–13). The pathogenesis of GVHD is a complex process involving a variety of host and donor immune cells (Figures 1, 2). The major determinant for the development and the severity of GVHD is the genetic disparity between the donor and recipient. However, some non-genetic factors such as the level of exposure to damage associated molecular patterns (DAMPs) and pathogen-associated molecular patterns (PAMPs) are also important components of GVHD pathophysiology, due to their ability to amplify inflammatory responses (14). In addition, other host factors may also influence the function of various immune cells and modulate the alloimmune response.
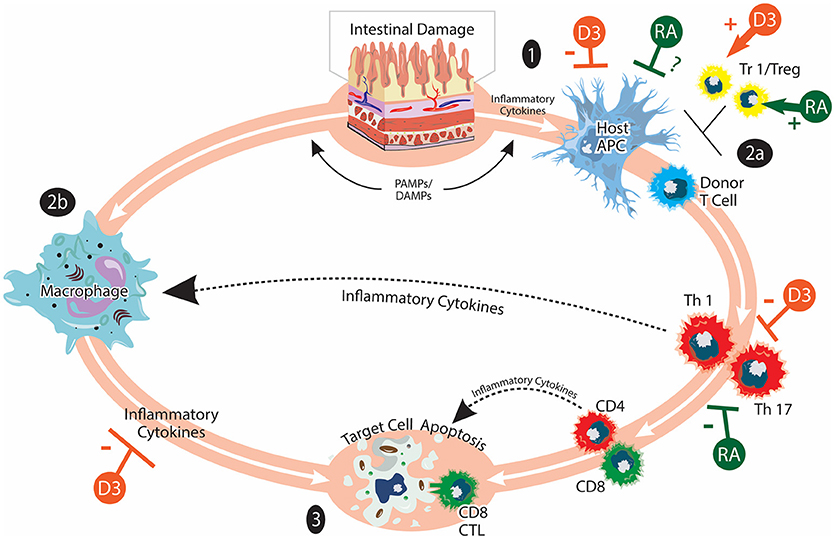
Figure 1. Acute GVHD and vitamins A and D. aGVHD pathogenesis involves: (1) Activation of host APCs due to release of inflammatory cytokines and PAMPs/DAMPs from tissue damaged by HSCT conditioning. Intestinal damage by conditioning serves to amplify inflammatory responses. (2) Activation of donor T cells when they encounter host APC. Donor T cells undergo differentiation, expansion, and acquisition of tissue homing specificity during this stage (2a). Inflammatory cytokines produced by donor T cells and bacterial LPS can further activate innate immune cells such as macrophages (2b). (3) Inflammatory mediators from donor T cells and innate immune cells lead to target cell apoptosis. Cytotoxic CD8 T cells can mediate direct cell killing. Tr1/Tregs play immunomodulatory roles in aGVHD pathogenesis. The effects of vitamin A/RA (shown in green) on aGVHD are complex and not completely understood. Vitamin A/RA promotes donor T-cell intestinal homing. Inhibiting donor T-cell RAR signaling suppresses the induction of gut-homing molecules and favors Treg cell differentiation. It has also been reported that RA inhibits donor T cell expansion and cytokine production. The potential effects of vitamin A/RA on host APCs are currently under investigation. The effects of vitamin D (shown in orange) on aGVHD may include suppressing the activation of host APCs, inhibiting the activation and cytokine production of donor T cells as well as promoting the induction of Tr1/Treg. The figure is adapted from Ferrara et al. (9).
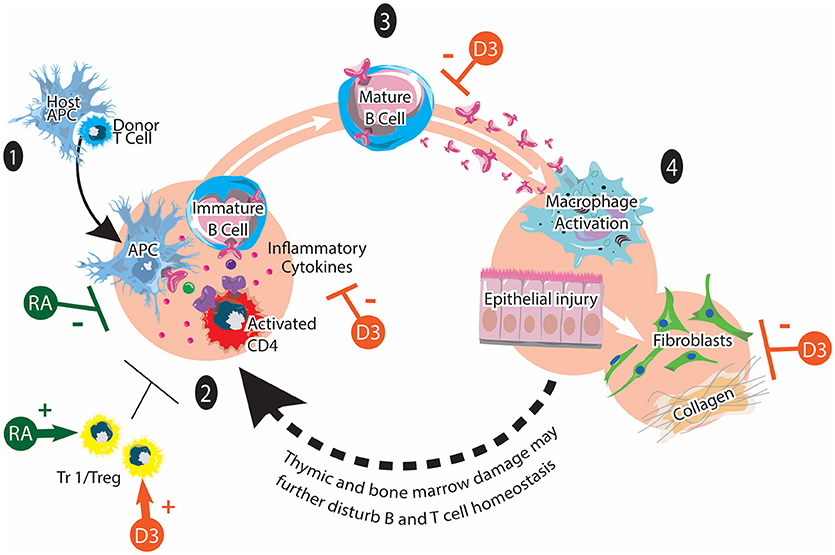
Figure 2. Chronic GVHD and vitamins A and D. cGVHD pathogenesis involves: (1) Early inflammation and tissue injury. An existing inflammation and danger signals activate innate immune cells and recruits donor T cells to the tissue. (2) Dysregulated immunity with loss of tolerance. Activated CD4 T cells stimulate the maturation of auto-reactive B cells. (3) Mature B cells produce various autoantibodies against host antigens. (4) Aberrant tissue repair and fibrosis via macrophages and fibroblasts. Tr1/Treg play immunomodulatory roles in chronic GVHD pathogenesis. It has been reported that synthetic retinoid (shown in green) reduces cGVHD by inhibiting Th1 and Th17 cells. It may also facilitate the generation of Tr1/Tregs. The potential effects of vitamin D on cGVHD (shown in orange) may include positive effects on Tr1/Treg function and polarization as well as inhibitory effects on proinflammatory T cell polarization, inflammatory cytokines, autoantibody secretion and collagen production. This figure is adapted from Cooke et al. (12).
Nutritional status is a significant variable among patients undergoing allogeneic HSCT. In fact, nutritional support appears to affect the development of GVHD, with adequate enteral nutrition being associated with reduced GVHD risk as compared to parenteral nutrition (15–18). These studies indicate that the interaction between certain oral nutrition and the gastrointestinal tract can modulate GVHD risk. Thus, patient nutritional status may be an independent and modifiable factor influencing GVHD severity (19). It is conceivable that an improved nutritional status may provide patients with an increased ability to tolerate treatment-associated toxicity and recover from GVHD-associated tissue damage. More importantly, certain micronutrients may also be actively involved in regulating the initiation, development, and resolution of inflammatory responses after HSCT. In this review, we briefly summarize the potential roles of vitamins A and D in GVHD pathogenesis.
Effects of Vitamin A on GVHD
Vitamin A is a multifunctional vitamin involved in a wide range of biological processes. Most biological effects of vitamin A are exerted by its major metabolite, retinoic acid (RA) (20). The conversion from vitamin A to RA requires two hydrolysis steps catalyzed first by alcohol dehydrogenases (ADHs), followed by aldehyde dehydrogenases (RALDHs). RALDHs are the rate-limiting enzymes for RA synthesis and are expressed in limited tissues (21). Heterodimers of retinoic acid receptors (RARs) and retinoid X receptors (RXRs) mediate RA signaling. These heterodimers bind to retinoic acid responsive elements (RARE) of target genes and regulate gene transcription. One of the most important physiological functions of vitamin A and RA is to regulate immune responses, and dysregulated retinoid signaling can lead to a weakened immunity against pathogens and/or the loss of immune homeostasis (20, 22).
RA has pleiotropic effects on cells of the innate and adaptive immune system (23–25). It can target T cells, B cells, antigen presenting cells (APCs), and innate lymphoid cells (ILCs) to regulate immune responses. RA induces the expression of gut-homing molecules CCR9 and α4β7 on various immune cells, augmenting cell migration to the intestines (26–28). At steady state, RA promotes the induction of tolerogenic dendritic cells (DCs). However, in the presence of inflammatory cytokines such as IL-15, RA promotes the induction of inflammatory DCs and intensifies pathogenic mucosal immune responses (29). RA has also been shown to influence the development of DC subsets in the spleen and intestines (30–33). RA also plays a central role in modulating intestinal CD4+ T cell responses and enhances the stability of natural regulatory T cells (Tregs) (34). Together with TGF-β, RA promotes the conversion of naïve T cells into induced-Tregs at the expense of Th17 cells (35, 36). Vitamin A deficiency is associated with impaired oral tolerance, suggesting an important role of RA in maintaining intestinal homeostasis (37, 38). On the other hand, the RA-RAR-α axis is important for CD4+ T cell activation and effector function under inflammatory conditions (39, 40). Finally, RA promotes the induction of ILC3 but suppresses the generation and cytokine production of ILC2 (41). These observations demonstrate the complex and sometimes paradoxical functions of RA within the immune system, as it can possess either pro-inflammatory or anti-inflammatory properties depending on the context.
In the context of GVHD, Koenecke and colleagues used a dietary approach to first examine how recipient vitamin A levels affect donor T cell trafficking after experimental HSCT (42). Vitamin A-deficient (VAD) recipient mice had a reduced percentage and absolute number of donor T cells in the intestine, which was attributable to diminished expression of gut-homing molecules α4β7 and CCR9. VAD recipients survived longer than control vitamin A-normal (VAN) mice due to gastrointestinal protection, though they developed more severe hepatic GVHD. These results indicated that vitamin A affects GVHD target organ tropism of donor T cells, with a particularly important role in controlling the migration of donor T cells to the intestine, a critical GVHD target organ (43). We and others then used a genetic approach to examine the role of RA signaling in GVHD pathogenesis. These studies consistently showed that genetic ablation of RAR-α on donor T cells significantly decreased the ability of these cells to cause lethal GVHD (44, 45). This was largely due to reduced expression of gut-homing molecules CCR9 and α4β7 on donor T cells with diminished intestinal migration. In contrast, administrating RA exogenously to recipient mice increased expression of gut-homing molecules on donor T cells and increased their gut-tropism, leading to a significantly increased overall mortality (44–46). In addition, inhibiting RAR-α reduced donor T cell differentiation toward a Th1 phenotype and favored the induction of Tregs (45), which also contribute to the decreased ability of these cells to cause GVHD. Importantly, genetic inhibition of RAR-α signaling on donor T cells does not compromise their ability to mediate the graft-vs.-leukemia effect.
In an effort to improve the translational potential of this research, we treated donor mice with BMS493, a pan-RAR antagonist. Recipients of BMS493-treated donor T cells showed improved overall survival after HSCT compared to recipients of vehicle-treated donor T cells, indicating that pharmacological inhibition of the retinoic acid pathway on donor T cells can reduce their alloreactivity and ability to cause GVHD (47). Interestingly, chronic vitamin A deficiency changed the composition of the donor T cell compartment with a reduction in the percentage of CD4+ T cells, resulting in reduced ability of transferred T cells from VAD mice to cause lethal GVHD (47). Thus, both host and donor vitamin A levels appear to affect the development of experimental GVHD (42, 47). While most preclinical studies suggest a detrimental effect of RA on GVHD, it has also been reported that RA treatment reduces aGVHD (48) and a synthetic retinoid ameliorates cGVHD (49). Differences in mouse GVHD models used, RA levels in situ, and local cytokine milieu could all potentially contribute to these differing observations.
Apart from above preclinical studies, emerging clinical data also demonstrate the involvement of vitamin A/RA in GVHD pathogenesis. A recent study found that lower levels of vitamin A are associated with increased intestinal GVHD in children receiving allogeneic HSCT (50). The incidence of grades 2–4 GVHD was also significantly higher in patients with lower vitamin A levels. These observations appear in contrast to a murine study in which vitamin A deficiency is associated with a reduced intestinal GVHD and improved overall survival (42). This discrepancy could be due to inherent differences between mouse model and human disease or increased severity of experimentally induced vitamin A deficiency compared to clinical deficiency/insufficiency. In addition, the study by Lounder et al. actually used serum vitamin A levels above or below a median value, instead of vitamin A deficiency or sufficiency, to separate patient groups. Finally, there was also evidence that low serum vitamin A levels are associated with more severe ocular GVHD in allogeneic HSCT patients (51). Thus, both preclinical and clinical data indicate a significant involvement of vitamin A and RA pathway in GVHD pathogenesis.
Effects of Vitamin D on GVHD
Vitamins D and A are similar in that they are the only two vitamins whose active metabolites have hormone-like properties. Indeed, the active metabolite of vitamin D, calcitriol, is a well-established secosteroid hormone with multiple roles throughout the human body (52). Though vitamin D may be acquired nutritionally, a large proportion of vitamin D is synthesized in the human body. This synthesis is initiated in the skin as UV-B rays cause the photolysis of 7-dehydrocholesterol, forming vitamin D3. In the liver, vitamin D3 is hydroxylated to 25-hydroxyvitamin D3 (25(OH)D3) by enzymes such as CYP2R1 and CYP27A1 (53, 54). 25-hydroxyvitamin D3 is the principal circulating metabolite of vitamin D and 25(OH)D3 concentration is typically used as an indicator of vitamin D status. This inactive 25(OH)D3 is hydroxylated once more in the kidney via the enzyme CYP27B1 to become the biologically active hormone 1,25-dihydroxyvitamin D3 (1,25(OH)2D3), also known as calcitriol.
Vitamin D utilizes similar signaling mechanisms to vitamin A. Calcitriol binds to the vitamin D receptor (VDR), which heterodimerizes with RXR (55, 56). VDR-RXR heterodimers that are bound to calcitriol act as transcriptional regulators by binding vitamin D response elements (VDREs) of target genes (57). The classical physiological roles of vitamin D (via calcitriol) are in calcium and phosphate homeostasis and bone metabolism, with other roles being referred to as “non-classical” functions. The discovery of vitamin D binding within immune cells in the early 1980s and eventual description of VDR expression in immune cells were key steps in the study of the non-classical effects of vitamin D on the immune system (58–60).
It has been shown in numerous studies that calcitriol inhibits maturation and inflammatory cytokine production of DCs (61–64). These changes in DC differentiation and function also result in a skew toward a more tolerogenic DC profile with the ability to drive Treg, T-regulatory cell type 1 (Tr1), and Th2 cell development (65). Importantly, calcitriol also appears to exhibit direct effects on CD4 T cell populations to modify immune function. In vitro treatment of T cells with calcitriol inhibits proliferation under several activating conditions (66, 67). Calcitriol has been shown to be effective as a treatment in numerous mouse models of diseases that are driven by Th1 and Th17 cells, suggesting a global immunomodulatory effect on these cell types (68–71). Calcitriol also appears to inhibit proliferation and pathogenicity of CD8 T cells since VDR-deficient CD8 T cells are hyperproliferative and proinflammatory (72, 73). Calcitriol inhibits production of IFN-γ and stimulates IL-4 secretion in invariant natural killer (iNKT) cells (70). Finally, calcitriol leads to decreased B cell proliferation and differentiation to plasma cells. However, it is unclear if these effects are due to direct effects on the B cell, or due to reduced interactions with CD4 T cells (74).
There is a significant history of studies on the effects of vitamin D and its analogs on allograft survival in several tissues (75, 76). However, preclinical studies in animal models of allogeneic HSCT are rather limited. To our knowledge, only one study of VDR agonism has been reported in animal models of GVHD. In this study, a vitamin D analog reduced aGVHD severity and immune cell infiltration in liver, skin, and spleen of rats (77). In vitro studies utilizing human monocyte-derived DCs recapitulated previously results showing that vitamin D led to the development of more immature tolerogenic DCs. Vitamin D-treated DCs activated allogeneic CD4 and CD8 T cells with a greater IL-10 to IFN-γ ratio, and these T cells were less proliferative in mixed lymphocyte reactions (MLRs) (78). In another study, alloreactive T cells were shown to express greater levels of VDR (79). Addition of calcitriol to the MLR led to a decrease in the percentage of proliferating T cells. This appeared to be due to direct action of calcitriol on the alloreactive T cells since the allogeneic DC were matured in the absence of calcitriol and irradiated prior to be used in the MLR (79).
The first reports for a potential role of vitamin D in human GVHD came through candidate gene studies analyzing known VDR polymorphisms. Interestingly, the results of these studies are quite variable. Some studies suggest roles for various polymorphisms in GVHD when present in the recipient only (80–82), some studies suggest a role for VDR genotype in both the donor and recipient (83), while another more recent study found no significant association between GVHD and VDR polymorphisms in the donor nor recipient (84). Taken together, these results suggest that in some instances the a allele of VDR may play a role in aGVHD risk when present in recipients of HSCT. However, not all studies have found such an association and the results may vary between different populations (84). One complicating factor of these studies was that the vitamin D status of the individuals studied was often unknown. Thus, any differences in VDR activity associated with disease could be obfuscated depending on whether an individual's vitamin D stores were sufficient to provide for VDR function.
Though genetic studies of VDR suggest a potential role for vitamin D signaling in GVHD, patient serum levels of vitamin D may provide a more direct method of investigation. Indeed, it appears that individuals undergoing HSCT are at particular risk for vitamin D deficiency/insufficiency (85–89). Several retrospective studies have thus investigated whether vitamin D status prior to HSCT corresponds with subsequent development of GVHD (90–92). These studies seem to suggest a relatively consistent association of cGVHD with lower vitamin D status, whereas the results for aGVHD are more variable. In a more recent study, however, levels of vitamin D pre-HSCT did not correlate to development of aGVHD nor cGVHD in a group of pediatric patients (93). Interestingly, the one-year survival rate did differ significantly, with 35% mortality in the deficient group vs. 0% in the insufficient and 7% in the sufficient groups, suggesting a beneficial effect of higher vitamin D levels on overall survival after allogeneic HSCT (93).
Given the potential association of vitamin D status and GVHD, the effect of supplementation was further investigated. Even though HSCT patients may be particularly at risk for vitamin D insufficiency/deficiency, supplementation can increase their vitamin D status (86, 90). Rosenblatt et al. reported observation of two patients with steroid refractory cGVHD who were treated with supplemental vitamin D for bone mineral abnormalities. Impressively, both had their symptoms wane to the point that they were removed from immunosuppression after vitamin D treatment (78). A marked improvement in cGVHD was also observed in a subsequent analysis of 12 adult HSCT patients who were given 1,000 IU/day vitamin D to treat osteopenia or osteoporosis (94).
To date, we are aware of only one published prospective study of vitamin D supplementation in HSCT patients (95). This investigation demonstrates that vitamin D may play a role in the prevention of cGVHD, as suggested previously (78, 90–92, 94). Intriguingly, there was no difference in aGVHD among the patient groups in this study. It is worth noting that in this study vitamin D supplementation began only 3 days prior to transplantation. Indeed, the authors show that significantly higher levels of 25(OH)D3 were not observed in the serum until day 7 in high-dose and day 21 in low-dose patients (95). This signifies that aGVHD may have been initiated in the absence of sufficiently elevated levels of vitamin D. Further investigation into the effect of earlier supplementation to raise serum vitamin D levels prior to HSCT to prevent aGVHD is of interest. Overall, the data surrounding vitamin D and the immune system as well as the initial studies on vitamin D and GVHD suggest that it is highly likely that vitamin D could exhibit positive effects in the prevention and/or treatment of GVHD (96).
Conclusions and Perspectives
In conclusion, we believe that small molecules like vitamins A and D could have the potential to influence the development of GVHD after allogeneic HSCT. These micronutrients may modulate crosstalk between the various immune cells involved in the pathogenesis of GVHD, thus influencing disease initiation, progression, and resolution (Figures 1, 2). Their levels may also have prognostic value, serving as independent risk factors for predicting the severity of organ-specific or systemic GVHD. Most importantly, nutritional intervention before and after allogeneic HSCT may be used as an adjuvant therapy to reduce GVHD risk and improve the outcome of allogeneic HSCT (95, 97, 98). We propose that GVHD research using animal models should consider dietary composition. More preclinical studies in this understudied research area will provide new insights into how nutritional factors contribute to GVHD pathogenesis. Finally, more prospective randomized controlled clinical trials are needed to fully reveal the potential of using micronutrients such as vitamins A and D as simple and inexpensive approaches with minimal side effects to mitigate clinical GVHD.
Author Contributions
All authors listed have made a substantial, direct and intellectual contribution to the work, and approved it for publication.
Funding
This work was supported by a grant from the National Institutes of Health, National Institute of Allergy and Infectious Diseases RO1 AI125334 (XC).
Conflict of Interest Statement
The authors declare that the research was conducted in the absence of any commercial or financial relationships that could be construed as a potential conflict of interest.
Acknowledgments
The authors thank Derek Fuchsberger for artistic design on the figures.
References
1. Mora JR, Iwata M, von Andrian UH. Vitamin effects on the immune system: vitamins A and D take centre stage. Nat Rev Immunol. (2008) 8:685–98. doi: 10.1038/nri2378
2. van de Pavert SA, Ferreira M, Domingues RG, Ribeiro H, Molenaar R, Moreira-Santos L, et al. Maternal retinoids control type 3 innate lymphoid cells and set the offspring immunity. Nature (2014) 508:123–7. doi: 10.1038/nature13158
3. Tamblyn JA, Hewison M, Wagner CL, Bulmer JN, Kilby MD. Immunological role of vitamin D at the maternal-fetal interface. J Endocrinol. (2015) 224:R107–21. doi: 10.1530/JOE-14-0642
4. Clark A, Mach N. Role of vitamin D in the hygiene hypothesis: the interplay between vitamin D, vitamin D receptors, gut microbiota, and immune response. Front. Immunol. (2016) 7:627. doi: 10.3389/fimmu.2016.00627
5. Macpherson AJ, de Aguero MG, Ganal-Vonarburg SC. How nutrition and the maternal microbiota shape the neonatal immune system. Nat Rev Immunol. (2017) 17:508–17. doi: 10.1038/nri.2017.58
7. Welniak LA, Blazar BR, Murphy WJ. Immunobiology of allogeneic hematopoietic stem cell transplantation. Annu Rev Immunol. (2007) 25:139–70. doi: 10.1146/annurev.immunol.25.022106.141606
8. Choi SW, Reddy P. Current and emerging strategies for the prevention of graft-versus-host disease. Nat Rev Clin Oncol. (2014) 11:536–47. doi: 10.1038/nrclinonc.2014.102
9. Ferrara JL, Levine JE, Reddy P, Holler E. Graft-versus-host disease. Lancet (2009) 373:1550–61. doi: 10.1016/S0140-6736(09)60237-3
10. Zeiser R, Blazar BR. Acute graft versus host disease – biologic process, prevention, and therapy. N Engl J Med. (2017) 377:2167–79. doi: 10.1056/NEJMra1609337
11. Zeiser R, Blazar BR. Pathophysiology of chronic graft versus host disease and therapeutic targets. N Engl J Med. (2017) 377:2565–79. doi: 10.1056/NEJMra1703472
12. Cooke KR, Luznik L, Sarantopoulos S, Hakim FT, Jagasia M, Fowler DH, et al. The biology of chronic graft-versus-host disease: a task force report from the national institutes of health consensus development project on criteria for clinical trials in chronic graft-versus-host disease. Biol Blood Marrow Transplant. (2017) 23:211–34. doi: 10.1016/j.bbmt.2016.09.023
13. MacDonald KP, Hill GR, Blazar BR. Chronic graft-versus-host disease: biological insights from preclinical and clinical studies. Blood (2017) 129:13–21. doi: 10.1182/blood-2016-06-686618
14. Toubai T, Mathewson ND, Magenau J, Reddy P. danger signals and graft-versus-host disease: current understanding and future perspectives. Front. Immunol. (2016) 7:539. doi: 10.3389/fimmu.2016.00539
15. Mattsson J, Westin S, Edlund S, Remberger M. Poor oral nutrition after allogeneic stem cell transplantation correlates significantly with severe graft-versus-host disease. Bone Marrow Transplant. (2006) 38:629–33. doi: 10.1038/sj.bmt.1705493
16. Svahn BM, Remberger M, Heijbel M, Martell E, Wikstrom M, Eriksson B, et al. Case-control comparison of at-home and hospital care for allogeneic hematopoietic stem-cell transplantation: the role of oral nutrition. Transplantation (2008) 85:1000–7. doi: 10.1097/TP.0b013e31816a3267
17. Seguy D, Duhamel A, Rejeb MB, Gomez E, Buhl ND, Bruno B, et al. Better outcome of patients undergoing enteral tube feeding after myeloablative conditioning for allogeneic stem cell transplantation. Transplantation (2012) 94:287–94. doi: 10.1097/TP.0b013e3182558f60
18. Beckerson J, Szydlo RM, Hickson M, Mactier CE, Innes AJ, Gabriel IH. Impact of route and adequacy of nutritional intake on outcomes of allogeneic haematopoietic cell transplantation for haematologic malignancies. Clin Nutr. (2018). doi: 10.1016/j.clnu.2018.03.008. [Epub ahead of print].
19. Kerby EH, Li Y, Getz KD, Smith EC, Smith LT, Bunin NJ, et al. Nutritional risk factors predict severe acute graft-versus-host disease and early mortality in pediatric allogeneic hematopoietic stem cell transplantation. Pediatr Blood Cancer (2018) 65:e26853. doi: 10.1002/pbc.26853
20. Stephensen CB. Vitamin A, infection, and immune function. Annu. Rev. Nutr. (2001) 21:167–92. doi: 10.1146/annurev.nutr.21.1.167
21. Kumar S, Sandell LL, Trainor PA, Koentgen F, Duester G. Alcohol and aldehyde dehydrogenases: retinoid metabolic effects in mouse knockout models. Biochim. Biophys. Acta (2012) 1821:198–205. doi: 10.1016/j.bbalip.2011.04.004
22. Larange A, Cheroutre H. Retinoic acid and retinoic acid receptors as pleiotropic modulators of the immune system. Annu Rev Immunol. (2016) 34:369–94. doi: 10.1146/annurev-immunol-041015-055427
23. Hall JA, Grainger JR, Spencer SP, Belkaid Y. The role of retinoic acid in tolerance and immunity. Immunity (2011) 35:13–22. doi: 10.1016/j.immuni.2011.07.002
24. Cassani B, Villablanca EJ, De Calisto J, Wang S, Mora JR. Vitamin A and immune regulation: role of retinoic acid in gut-associated dendritic cell education, immune protection and tolerance. Mol Aspects Med. (2012) 33:63–76. doi: 10.1016/j.mam.2011.11.001
25. Raverdeau M, Mills KH. Modulation of T cell and innate immune responses by retinoic acid. J. Immunol. (2014) 192:2953–8. doi: 10.4049/jimmunol.1303245
26. Iwata M, Hirakiyama A, Eshima Y, Kagechika H, Kato C, Song SY. Retinoic acid imprints gut-homing specificity on T cells. Immunity (2004) 21:527–38. doi: 10.1016/j.immuni.2004.08.011
27. Mora JR, Iwata M, Eksteen B, Song SY, Junt T, Senman B., et al. Generation of gut-homing IgA-secreting B cells by intestinal dendritic cells. Science (2006) 314:1157–60. doi: 10.1126/science.1132742
28. Kim MH, Taparowsky EJ, Kim CH. Retinoic acid differentially regulates the migration of innate lymphoid cell subsets to the gut. Immunity (2015) 43:107. doi: 10.1016/j.immuni.2015.06.009
29. DePaolo RW, Abadie V, Tang F, Fehlner-Peach H, Hall JA, Wang W, et al. Co-adjuvant effects of retinoic acid and IL-15 induce inflammatory immunity to dietary antigens. Nature (2011) 471:220–4. doi: 10.1038/nature09849
30. Beijer MR, Molenaar R, Goverse G, Mebius RE, Kraal G, den Haan JM. A crucial role for retinoic acid in the development of Notch-dependent murine splenic CD8- CD4- and CD4+ dendritic cells. Eur J Immunol. (2013) 43:1608–16. doi: 10.1002/eji.201343325
31. Klebanoff CA, Spencer SP, Torabi-Parizi P, Grainger JR, Roychoudhuri R, Ji Y, et al. Retinoic acid controls the homeostasis of pre-cDC-derived splenic and intestinal dendritic cells. J. Exp. Med. (2013) 210:1961–76. doi: 10.1084/jem.20122508
32. Saurer L, McCullough KC, Summerfield A. In vitro induction of mucosa-type dendritic cells by all-trans retinoic acid. J Immunol. (2007) 179:3504–14. doi: 10.4049/jimmunol.179.6.3504
33. Zeng R, Oderup C, Yuan R, Lee M, Habtezion A, Hadeiba H, et al. Retinoic acid regulates the development of a gut-homing precursor for intestinal dendritic cells. Mucosal Immunol. (2013) 6:847–56. doi: 10.1038/mi.2012.123
34. Zhou X, Kong N, Wang J, Fan H, Zou H, Horwitz D, et al. Cutting edge: all-trans retinoic acid sustains the stability and function of natural regulatory T cells in an inflammatory milieu. J Immunol. (2010) 185:2675–79. doi: 10.4049/jimmunol.1000598
35. Mucida D, Park Y, Kim G, Turovskaya O, Scott I, Kronenberg M, et al. Reciprocal TH17 and regulatory T cell differentiation mediated by retinoic acid. Science (2007) 317:256–60. doi: 10.1126/science.1145697
36. Benson MJ, Pino-Lagos K, Rosemblatt M, Noelle RJ. All-trans retinoic acid mediates enhanced Treg cell growth, differentiation, and gut homing in the face of high levels of co-stimulation. J Exp Med. (2007) 204:1765–74. doi: 10.1084/jem.20070719
37. Nakamoto A, Shuto E, Tsutsumi R, Nakamoto M, Nii Y, Sakai T. Vitamin A deficiency impairs induction of oral tolerance in mice. J Nutr Sci Vitaminol. (2015) 61:147–53. doi: 10.3177/jnsv.61.147
38. Turfkruyer M, Rekima A, Macchiaverni P, Le Bourhis L, Muncan V, van den Brink GR, et al. Oral tolerance is inefficient in neonatal mice due to a physiological vitamin A deficiency. Mucosal Immunol. (2016) 9:479–91. doi: 10.1038/mi.2015.114
39. Hall JA, Cannons JL, Grainger JR, Dos Santos LM, Hand TW, Naik S, et al. Essential role for retinoic acid in the promotion of CD4(+) T cell effector responses via retinoic acid receptor alpha. Immunity (2011) 34:435–47. doi: 10.1016/j.immuni.2011.03.003
40. Pino-Lagos K, Guo Y, Brown C, Alexander MP, Elgueta R, Bennett KA, et al. A retinoic acid-dependent checkpoint in the development of CD4+ T cell-mediated immunity. J. Exp. Med. (2011) 208:1767–75. doi: 10.1084/jem.20102358
41. Spencer SP, Wilhelm C, Yang Q, Hall JA, Bouladoux N, Boyd A, et al. Adaptation of innate lymphoid cells to a micronutrient deficiency promotes type 2 barrier immunity. Science (2014) 343:432–7. doi: 10.1126/science.1247606
42. Koenecke C, Prinz I, Bubke A, Schreder A, Lee CW, Pabst O, et al. Shift of graft versus host disease organ tropism by dietary vitamin A. PLoS ONE (2012) 7:e38252. doi: 10.1371/journal.pone.0038252
43. Hill GR, Ferrara JLM. The primacy of the gastrointestinal tract as a target organ of acute graft-versus-host disease: rationale for the use of cytokine shields in allogeneic bone marrow transplantation. Blood (2000) 95:2754–9.
44. Chen X, Dodge J, Komorowski R, Drobyski WR. A critical role for the retinoic acid signaling pathway in the pathophysiology of gastrointestinal graft-versus-host disease. Blood (2013) 121:3970–80. doi: 10.1182/blood-2012-08-445130
45. Aoyama K, Saha A, Tolar J, Riddle MJ, Veenstra RG, Taylor PA, et al. Inhibiting retinoic acid signaling ameliorates graft-versus-host disease by modifying T-cell differentiation and intestinal migration. Blood (2013) 122:2125–34. doi: 10.1182/blood-2012-11-470252
46. Wang D, Yu Y, Haarberg K, Fu J, Kaosaard K, Nagaraj S, et al. Dynamic change and impact of myeloid-derived suppressor cells in allogeneic bone marrow transplantation in mice. Biol Blood Marrow Transplant. (2013) 19:692–702. doi: 10.1016/j.bbmt.2013.01.008
47. Dodge J, Stephans A, Lai J, Drobyski WR, Chen X. Effects of donor vitamin A deficiency and pharmacologic modulation of donor T cell retinoic acid pathway on the severity of experimental graft-versus-host disease. Biol Blood Marrow Transplant. (2016) 22:2141–28. doi: 10.1016/j.bbmt.2016.09.001
48. Yang H, Gu J, Zhu Q, Lu H, Wang K, Ni X, et al. Protection of acute GVHD by all-trans retinoic acid through suppression of T cell expansion and induction of regulatory T cells through IL-2 signaling. Int Immunopharmacol. (2015) 28:911–6. doi: 10.1016/j.intimp.2015.03.042
49. Nishimori H, Maeda Y, Teshima T, Sugiyama H, Kobayashi K, Yamasuji Y, et al. Synthetic retinoid Am80 ameliorates chronic graft-versus-host disease by down-regulating Th1 and Th17. Blood (2012) 119:285–95. doi: 10.1182/blood-2011-01-332478
50. Lounder DT, Khandelwal P, Dandoy CE, Jodele S, Grimley MS, Wallace G, et al. Lower levels of vitamin A are associated with increased gastrointestinal graft-versus-host disease in children. Blood (2017) 129:2801–07. doi: 10.1182/blood-2017-02-765826
51. Tong J, Hu R, Zhao Y, Xu Y, Zhao X, Jin X. Serum vitamin A levels may affect the severity of ocular graft-versus-host disease. Front Med. (2017) 4:67. doi: 10.3389/fmed.2017.00067
52. Holick MF, Chen TC. Vitamin D deficiency: a worldwide problem with health consequences. Am J Clin Nutr. (2008) 87:1080S−6S. doi: 10.1093/ajcn/87.4.1080S
53. Cheng JB, Levine MA, Bell NH, Mangelsdorf DJ, Russell DW. Genetic evidence that the human CYP2R1 enzyme is a key vitamin D 25-hydroxylase. Proc Natl Acad Sci USA. (2004) 101:7711–5. doi: 10.1073/pnas.0402490101
54. Sawada N, Sakaki T, Ohta M, Inouye K. Metabolism of vitamin D by human CYP27A1. Biochem Biophys Res Commun. (2000) 273:977–84. doi: 10.1006/bbrc.2000.3050
55. Haussler MR, Norman AW. Chromosomal receptor for a vitamin D metabolite. Proc Natl Acad Sci USA. (1969) 62:155–62. doi: 10.1073/pnas.62.1.155
56. Jin CH, Kerner SA, Hong MH, Pike JW. Transcriptional activation and dimerization functions in the human vitamin D receptor. Mol Endocrinol. (1996) 10:945–57.
57. Haussler MR, Whitfield GK, Kaneko I, Haussler CA, Hsieh D, Hsieh J-C, et al. Molecular mechanisms of vitamin D action. Calcif Tissue Int. (2013) 92:77–98. doi: 10.1007/s00223-012-9619-0
58. Abe E, Miyaura C, Sakagami H, Takeda M, Konno K, Yamazaki T, et al. Differentiation of mouse myeloid leukemia cells induced by 1 alpha,25-dihydroxyvitamin D3. Proc Natl Acad Sci USA. (1981) 78:4990–4. doi: 10.1073/pnas.78.8.4990
59. Bhalla AK, Amento EP, Clemens TL, Holick MF, Krane SM. Specific high-affinity receptors for 1,25-dihydroxyvitamin D3 in human peripheral blood mononuclear cells: presence in monocytes and induction in T lymphocytes following activation. J Clin Endocrinol Metab. (1983) 57:1308–10. doi: 10.1210/jcem-57-6-1308
60. Provvedini DM, Tsoukas CD, Deftos LJ, Manolagas SC. 1,25-dihydroxyvitamin D3 receptors in human leukocytes. Science (1983) 221:1181–3. doi: 10.1126/science.6310748
61. Penna G, Adorini L. 1 Alpha,25-dihydroxyvitamin D3 inhibits differentiation, maturation, activation, and survival of dendritic cells leading to impaired alloreactive T cell activation. J Immunol. (2000) 164:2405–11. doi: 10.4049/jimmunol.164.5.2405
62. Piemonti L, Monti P, Sironi M, Fraticelli P, Leone BE, Dal Cin E, et al. Vitamin D3 affects differentiation, maturation, and function of human monocyte-derived dendritic cells. J Immunol. (2000) 164:4443–51. doi: 10.4049/jimmunol.164.9.4443
63. Adorini L, Penna G, Giarratana N, Roncari A, Amuchastegui S, Daniel KC, et al. Dendritic cells as key targets for immunomodulation by Vitamin D receptor ligands. J Steroid Biochem Mol Biol. (2004) 89–90:437–41. doi: 10.1016/j.jsbmb.2004.03.013
64. Adorini L, Penna G. Dendritic cell tolerogenicity: a key mechanism in immunomodulation by vitamin D receptor agonists. Hum Immunol. (2009) 70:345–52. doi: 10.1016/j.humimm.2009.01.016
65. Penna G, Amuchastegui S, Giarratana N, Daniel KC, Vulcano M, Sozzani S, et al. 1,25-Dihydroxyvitamin D3 selectively modulates tolerogenic properties in myeloid but not plasmacytoid dendritic cells. J Immunol. (2007) 178:145–53. doi: 10.4049/jimmunol.178.1.145
66. Mahon BD, Wittke A, Weaver V, Cantorna MT. The targets of vitamin D depend on the differentiation and activation status of CD4 positive T cells. J Cell Biochem. (2003) 89:922–32. doi: 10.1002/jcb.10580
67. Correale J, Ysrraelit MC, Gaitán MI. Immunomodulatory effects of Vitamin D in multiple sclerosis. Brain (2009) 132:1146–60. doi: 10.1093/brain/awp033
68. Tang J, Zhou R, Luger D, Zhu W, Silver PB, Grajewski RS, et al. Calcitriol suppresses antiretinal autoimmunity through inhibitory effects on the Th17 effector response. J Immunol. (2009) 182:4624–32. doi: 10.4049/jimmunol.0801543
69. Mayne CG, Spanier JA, Relland LM, Williams CB, Hayes CE. 1,25-Dihydroxyvitamin D3 acts directly on the T lymphocyte vitamin D receptor to inhibit experimental autoimmune encephalomyelitis. Eur J Immunol. (2011) 41:822–32. doi: 10.1002/eji.201040632
70. Cantorna MT, Snyder L, Lin Y-D, Yang L. Vitamin D and 1,25(OH)2D Regulation of T cells. Nutrients (2015) 7:3011–21. doi: 10.3390/nu7043011
71. Hayes CE, Hubler SL, Moore JR, Barta LE, Praska CE, Nashold FE. Vitamin D Actions on CD4+ T Cells in Autoimmune Disease. Front Immunol. (2015) 6:100. doi: 10.3389/fimmu.2015.00100
72. Rigby WF, Stacy T, Fanger MW. Inhibition of T lymphocyte mitogenesis by 1,25-dihydroxyvitamin D3 (calcitriol). J Clin Invest. (1984) 74:1451–5. doi: 10.1172/JCI111557
73. Chen J, Bruce D, Cantorna MT. Vitamin D receptor expression controls proliferation of naïve CD8+ T cells and development of CD8 mediated gastrointestinal inflammation. BMC Immunol. (2014) 15:6. doi: 10.1186/1471-2172-15-6
74. Chen S, Sims GP, Chen XX, Gu YY, Chen S, Lipsky PE. Modulatory effects of 1,25-dihydroxyvitamin D3 on human B cell differentiation. J Immunol. (2007) 179:1634–47. doi: 10.4049/jimmunol.179.3.1634
75. Kallio E, Häyry P, Pakkala S. MC1288, a vitamin D analogue, reduces short- and long-term renal allograft rejection in the rat. Transplant Proc. (1996) 28:311.
76. Hullett DA, Cantorna MT, Redaelli C, Humpal-Winter J, Hayes CE, Sollinger HW, et al. Prolongation of allograft survival by 1,25-dihydroxyvitamin D3. Transplantation (1998) 66:824–8. doi: 10.1097/00007890-199810150-00002
77. Pakkala I, Taskinen E, Pakkala S, Räisänen-Sokolowski A. MC1288, a vitamin D analog, prevents acute graft-versus-host disease in rat bone marrow transplantation. Bone Marrow Transplant. (2001) 27:863–7. doi: 10.1038/sj.bmt.1702873
78. Rosenblatt J, Bissonnette A, Ahmad R, Wu Z, Vasir B, Stevenson K, et al. Immunomodulatory effects of vitamin D: implications for GVHD. Bone Marrow Transplant. (2010) 45:1463–8. doi: 10.1038/bmt.2009.366
79. Joseph RW, Bayraktar UD, Kim TK, St John LS, Popat U, Khalili J, et al. Vitamin D receptor upregulation in alloreactive human T cells. Hum Immunol. (2012) 73:693–8. doi: 10.1016/j.humimm.2012.04.019
80. Middleton PG, Cullup H, Dickinson AM, Norden J, Jackson GH, Taylor PRA, et al. Vitamin D receptor gene polymorphism associates with graft-versus-host disease and survival in HLA-matched sibling allogeneic bone marrow transplantation. Bone Marrow Transplant. (2002) 30:223–8. doi: 10.1038/sj.bmt.1703629
81. Rocha V, Porcher R, Fernandes JF, Filion A, Bittencourt HWS Jr, Vilela G, et al. Association of drug metabolism gene polymorphisms with toxicities, graft-versus-host disease and survival after HLA-identical sibling hematopoietic stem cell transplantation for patients with leukemia. Leukemia (2009) 23:545–56. doi: 10.1038/leu.2008.323
82. Cho HJ, Shin D-Y, Kim JH, Bae J-Y, Lee K-H, See CJ, et al. Impact of vitamin D receptor gene polymorphisms on clinical outcomes of HLA-matched sibling hematopoietic stem cell transplantation. Clin Transplant. (2012) 26:476–83. doi: 10.1111/j.1399-0012.2011.01523.x
83. Bogunia-Kubik K, Middleton P, Norden J, Dickinson A, Lange A. Association of vitamin D receptor polymorphisms with the outcome of allogeneic haematopoietic stem cell transplantation. Int J Immunogenet. (2008) 35:207–13. doi: 10.1111/j.1744-313X.2008.00758.x
84. Kamel AM, El-Fishawi S, Rasekh EO, Radwan ER, Zeidan A, El-Said A, et al. Variability of contribution of vitamin D receptor gene polymorphisms to outcome of HLA-matched sibling allogeneic bone marrow transplantation. Leuk Lymphoma (2018) 4:1–10. doi: 10.1080/10428194.2018.1459608
85. Kreutz M, Eissner G, Hahn J, Andreesen R, Drobnik W, Holler E. Variations in 1 alpha,25-dihydroxyvitamin D3 and 25-hydroxyvitamin D3 serum levels during allogeneic bone marrow transplantation. Bone Marrow Transplant. (2004) 33:871–3. doi: 10.1038/sj.bmt.1704448
86. Robien K, Strayer LG, Majhail N, Lazovich D, Baker KS, Smith AR, et al. Vitamin D status among long-term survivors of hematopoietic cell transplantation. Bone Marrow Transplant. (2011) 46:1472–79. doi: 10.1038/bmt.2010.326
87. Urbain P, Ihorst G, Biesalski H-K, Bertz H. Course of serum 25-hydroxyvitamin D status and its influencing factors in adults undergoing allogeneic hematopoietic cell transplantation. Ann Hematol. (2012) 91:759–66. doi: 10.1007/s00277-011-1365-2
88. Myers KC, Howell JC, Wallace G, Dandoy C, El-Bietar J, Lane A, et al. Poor growth, thyroid dysfunction and vitamin D deficiency remain prevalent despite reduced intensity chemotherapy for hematopoietic stem cell transplantation in children and young adults. Bone Marrow Transplant. (2016) 51:980–4. doi: 10.1038/bmt.2016.39
89. Wallace G, Jodele S, Myers KC, Dandoy CE, El-Bietar J, Nelson A, et al. Vitamin D deficiency in pediatric hematopoietic stem cell transplantation patients despite both standard and aggressive supplementation. Biol Blood Marrow Transplant. (2016) 22:1271–4. doi: 10.1016/j.bbmt.2016.03.026
90. Glotzbecker B, Ho VT, Aldridge J, Kim HT, Horowitz G, Ritz J, et al. Low levels of 25-hydroxyvitamin D before allogeneic hematopoietic SCT correlate with the development of chronic GVHD. Bone Marrow Transplant. (2013) 48:593–7. doi: 10.1038/bmt.2012.177
91. von Bahr L, Blennow O, Alm J, Björklund A, Malmberg K-J, Mougiakakos D, et al. Increased incidence of chronic GvHD and CMV disease in patients with vitamin D deficiency before allogeneic stem cell transplantation. Bone Marrow Transplant. (2015) 50:1217–23. doi: 10.1038/bmt.2015.123
92. Hansson MEA, Norlin A-C, Omazic B, Wikström A-C, Bergman P, Winiarski J, et al. Vitamin D levels affect outcome in pediatric hematopoietic stem cell transplantation. Biol Blood Marrow Transplant. (2014) 20:1537–43. doi: 10.1016/j.bbmt.2014.05.030
93. Beebe K, Magee K, McNulty A, Stahlecker J, Salzberg D, Miller H, et al. Vitamin D deficiency and outcomes in pediatric hematopoietic stem cell transplantation. Pediatr Blood Cancer (2018) 65:1–6. doi: 10.1002/pbc.26817
94. Silva F, Pérez-Simón JA, Caballero-Velazquez T, Sánchez-Guijo FM, Villanueva-Gomez F, Vazquez L, et al. Effect of vitamin D treatment in chronic GVHD. Bone Marrow Transplant. (2011) 46:1395–7. doi: 10.1038/bmt.2010.317
95. Caballero-Velázquez T, Montero I, Sánchez-Guijo F, Parody R, Saldaña R, Valcarcel D, et al. Immunomodulatory effect of vitamin D after allogeneic stem cell transplantation: results of a prospective multicenter clinical trial. Clin Cancer Res. (2016) 22:5673–81. doi: 10.1158/1078-0432.CCR-16-0238
96. Benrashid M, Moyers K, Mohty M, Savani BN. Vitamin D deficiency, autoimmunity, and graft-versus-host-disease risk: implication for preventive therapy. Exp Hematol. (2012) 40:263–7. doi: 10.1016/j.exphem.2012.01.006
97. Carpenter PA. Vitamin A to reduce gut leak and GVHD? Blood (2017) 129:2715–7. doi: 10.1182/blood-2017-03-773226
Keywords: vitamin A, vitamin D, retinoic acid, vitamin D receptor, graft-vs.-host disease, allogeneic hematopoietic stem cell transplantation
Citation: Chen X and Mayne CG (2018) The Role of Micronutrients in Graft-VS.-Host Disease: Immunomodulatory Effects of Vitamins A and D. Front. Immunol. 9:2853. doi: 10.3389/fimmu.2018.02853
Received: 01 October 2018; Accepted: 20 November 2018;
Published: 06 December 2018.
Edited by:
Xue-Zhong Yu, Medical University of South Carolina, United StatesReviewed by:
Michael Uhlin, Karolinska Institutet (KI), SwedenLuiza Guilherme, University of São Paulo, Brazil
Copyright © 2018 Chen and Mayne. This is an open-access article distributed under the terms of the Creative Commons Attribution License (CC BY). The use, distribution or reproduction in other forums is permitted, provided the original author(s) and the copyright owner(s) are credited and that the original publication in this journal is cited, in accordance with accepted academic practice. No use, distribution or reproduction is permitted which does not comply with these terms.
*Correspondence: Xiao Chen, xchen@mcw.edu