- 1Division of Hematology, Department of Internal Medicine, Comprehensive Cancer Center, The Ohio State University, Columbus, OH, United States
- 2Department of Veterinary Biosciences, College of Veterinary Medicine, The Ohio State University, Columbus, OH, United States
Acute graft-vs.-host disease (aGVHD) is a frequent complication of allogeneic hematopoietic stem cell transplantation (allo-HSCT), accounting for substantial morbidity and mortality associated with this treatment modality. The pathogenesis of aGVHD involves a complex cascade of humoral and cellular interactions in which donor T cells target HLA mismatched host tissues, causing tissue injury through secretion of pro-inflammatory cytokines and induction of direct cytotoxicity. Toll-like receptors (TLRs) are key components of the innate immune system that recognize endogenous danger-associated molecular patterns (DAMPs) and exogenous pathogen-associated molecular patterns (PAMPs). Patients receiving conditioning chemotherapy and/or whole-body irradiation prior to all-HSCT are prone to gastrointestinal damage and translocation of microbiota across compromised intestinal epithelium, resulting in release of PAMPs and DAMPs. These “danger signals” play critical roles in disease pathogenesis by both initiating and propagating aGVHD through dendritic cell maturation and alloreactive T cell responses. There are 10–15 TLRs identified in mammalian species, a subset of which recognize single-stranded RNA (ssRNA) and serve as a key component of viral immunity. Recently, ssRNAs other than those of viral origin have been investigated as potential ligands of TLRs. MicroRNAs (miRs) are short (19–24 nt) non-coding RNAs that play critical roles in a variety of diseases. While traditionally miRs post-translationally modulate gene expression, non-canonical functions such as regulating TLR stimulation by acting as TLR ligands have been described. Here, we review the role of TLRs in aGVHD pathogenesis, the function of miRs in TLR stimulation, and the recent literature describing miRs as TLR ligands in aGVHD.
Introduction
Acute graft-vs.-host disease (aGVHD) is a frequent complication of allogeneic hematopoietic stem cell transplants (allo-HSCTs), with 30–75% of allo-HSCT recipients developing aGVHD (1, 2). Furthermore, aGVHD accounts for ~10% of all non-relapse mortality in patients that receive allo-HSCT (3, 4), and those with severe aGVHD have a poor prognosis, with an overall 2 years survival of 20–30% (5–7). The morbidity and mortality associated with aGVHD pose a substantial barrier against the wider and safer application of HSCT as a curative modality.
While current prophylaxis and therapeutics function through systemic immunosuppression (1, 8–14), these treatments increase the risk of systemic infections and leukemia relapse (14–16). Therefore, aGVHD research efforts are being focused on not only the development of novel treatment strategies, but more so on the deeper understanding of aGVHD pathogenesis so that aGVHD may be prevented. The potent activation of Toll-like receptors (TLRs) on antigen presenting cells (APCs) following conditioning regimens is often considered a critical initiating event in the development of aGVHD (2, 17–20). Here, we review the current understanding of the classical role of TLRs and their ligands in aGVHD pathogenesis as well as the recent literature describing microRNAs (miRs) as novel ligands for TLRs both broadly and in the context of aGVHD.
Toll-Like Receptors (TLRs)
Toll-like receptors are a family of evolutionarily-conserved transmembrane pattern recognition receptors (PRRs) that are critical for innate immune responses and the cross-talk between innate and adaptive immune systems. The concept of PRRs is attributed to Dr. Charles Janeway, who, in 1989, proposed the existence of immune receptors on surveillance cells such as APCs. which allow the innate immune system to specifically recognize microbial infections and mount an appropriate immune response (21). The first member of the Toll family was identified in Drosophila flies in 1988, although at that time its function was only recognized as being critical for dorsoventral polarity during fly embryo development (22). The connection between Drosophila Toll and innate immunity was not recognized until later, when Drosophila Toll and human IL-1R were identified as having homologous cytoplasmic domains and the capability of inducing Rel family transcription factor activation (23). Furthermore, it was observed that Drosophila flies that carried non-functional Toll genes demonstrated significant defects in antifungal responses, although immune responses to bacterial organisms remained intact (24). In 1997, the first human Toll homolog, called hToll (now known as TLR4), was cloned and was shown to signal through the NF-κB signaling pathway, resulting in the production of inflammatory cytokines during the adaptive immune response (25). One year later in 1998, the connection between TLR4 and its ligand LPS was recognized as endotoxin-tolerant mouse strains were shown to have point mutations in the Tlr4 gene (26, 27). To date, there are 13 TLRs identified between mice and humans which allow the innate immune system to recognize not only bacteria but also viruses, fungi, and protozoa (28, 29).
TLRs in aGVHD
aGVHD is a complex, multistep disease in which immunocompetent donor T cells destroy MHC-mismatched host tissues by secreting inflammatory cytokines and/or direct cytotoxicity (30, 31). However, pathogenesis of aGVHD is a self-perpetuating cycle that often begins even before the graft is transplanted into the patient. Whole body irradiation and/or chemotherapy frequently used as conditioning regimens are very efficient in reducing leukemia burden and clearing any immune or hematopoietic cells prior to transplantation to prevent graft rejection (32). The cytotoxic effects, however, are not specific to only leukocytes or other hematopoietic cells within the body. Instead, the GI tract is one of the most sensitive organs to radiation and chemotherapy induced acute damage (33, 34–36). Following conditioning therapy, there is extensive tissue damage in the GI as well as compromise to the GI epithelium. This allows translocation of GI flora across the mucosal barrier (37, 38) resulting in the release of inflammatory cytokines (39), danger associated molecular patterns (DAMPs), and pathogen associated molecular patterns (PAMPs). These molecules are then recognized by PRRs on APCs, allowing for their activation (40–42).
DAMPs, also called alarmins, are host-derived “danger” signals produced by the body to allow the immune system to recognize times of extreme cellular stress (43). Typically, the release of DAMPs from damaged tissues occurs when the cells undergoes necrosis (as opposed to apoptosis) since the process of necrosis leads to cell swelling and lysis. DAMPs can arise from two sources in the body: intracellular or extracellular (44). Intracellular DAMPs are released from necrotic cells and include shock proteins (45) and purine metabolites such as ATP (46). On the other hand, extracellular DAMPs arise from breakdown products of the extracellular matrix surrounding stressed cells. Examples of extracellular DAMPs include biglycan, heparin sulfate, and hyaluronan (47). PAMPs, in contrast, are molecules found in/on infectious agents that allow the immune system to recognize exogenous organisms. In aGVHD, PAMPs generally arise from translocated GI flora from the lumen of the intestines to tissues or blood. Examples of common PAMPs critical for aGVHD pathogenesis include lipopolysaccharide (LPS), flagellin, peptidoglycans, and microbial CpG-DNA (17, 48–50). Donor and recipient (host) APCs recognize DAMPs and PAMPs through PRRs, the most well-described of which are TLRs (19, 51). For example, microbial PAMPs such as LPS, flagellin, and CpG-motifs, which may be found in or on translocated GI bacteria, are recognized by TLRs 4, 5, and 9, respectively (48, 50). The consequences of TLR activation in aGVHD are upregulation of adhesion molecules, human leukocyte antigen molecules, and pro-inflammatory cytokine production such as IL-1β, IL-6, IL-12, TNFα, and IFNγ. The downstream effects of TLR-induced APC stimulation are the potent donor T cell activation, expansion, differentiation, and trafficking in aGVHD (52).
As TLRs are important for the initiation of aGVHD, researchers have studied the roles of single nucleotide polymorphisms (SNPs) in TLRs of both donor and recipients and their impacts on aGVHD development. The most well-studied TLR polymorphisms in aGVHD are Asp299Gly and Thr399Ile in TLR4. These TLR4 SNPs were first described in allo-HSCT donors and recipients by Lorenz et al. (53). In this report, the authors demonstrated that the presence of polymorphisms in either the donor or recipient are associated with a lower incidence of aGVHD, although statistical significance was not achieved. Elmaagacli et al. documented slightly conflicting results, with the presence of Thr399Ile SNP in TLR4 in either donor alone or both recipient and donor being associated with more severe aGVHD using univariant analysis; however, statistical significance was lost when using multivariant analysis (54). SNPs in TLR9 have also been shown to impact aGVHD susceptibility, as patients receiving allo-HSCT from donors with either one of two SNPs in TLR9 demonstrated more frequent grade II-IV aGVHD when compared to those receiving allo-HSCT from donors with wildtype TLR9 (55). Sivula et al. demonstrated that many different TLR SNPs found in allo-HSCT donors and/or recipients are associated with aGVHD occurrence when evaluated independently from one another, including one SNP in TLR1, one SNP in TLR4, three SNPs in TLR5, one SNP in TLR6, and one SNP in TLR10, based on multivariant analyses (56). Interestingly, the authors also demonstrate that one SNP in TLR4 found in allo-HSCT recipients was protective from aGVHD.
Yet another focus of research regarding TLRs and aGVHD is how modulation of TLR activation impacts aGVHD development, with the goal of developing novel prophylaxis and therapeutics. Utilizing TLR4 wildtype or deficient mice, Zhao et al. demonstrated that inactivation of TLR4 in either the donor or recipient is protective against aGVHD, with recipient mice having reduced aGVHD symptoms and delayed mortality (57). These finding were also supported by Brennan et al. who showed that administration of heparan sulfate (a TLR4 agonist) promotes aGVHD development while administration of α1-antitrypsin [a serine protease inhibitor which functions as a TLR4 antagonist by disrupting the LPS-TLR4-NF-κB axis (58–60)] reduced aGVHD severity (61). Similar to TLR4, TLR9 inactivation in recipient mice through global deletion, significantly reduces aGVHD severity and mortality (62). Further supporting this data, Taylor et al. demonstrated that repeated administration of CpG oligodeoxynucleotides, a main ligand of TLR9, accelerated aGVHD lethality (63). There are other TLRs such as TLR7 that may accelerate or ameliorate aGVHD depending on time and duration of administration. Chakraverty et al. showed that topical application of R-848, a TLR7/8 agonist, induced severe donor T cell infiltration into the skin of recipient mice 64. Similar results were obtained independently by another research group who repeatedly administered either 3M-011 (a TLR7/8 agonist) or drug vehicle and observed that mice receiving 3M-011 had higher overall mortality when compared to mice receiving vehicle (63). In contrast, from data Jasperson et al. indicated that a single administration of 3M-011 between lethal irradiation conditioning and allo-HSCT induced the tryptophan catabolic pathway in APCs, leading to significantly reduced lethality and colonic pathology scores (65). While the pharmacologic studies described here are all based on well-studied methods of TLR activation or inhibition, researchers are also actively investigating other novel mechanisms by which TLRs are activated and can be pharmacologically manipulated.
MiRs as TLR Ligands
MicroRNAs (miRs) are small, non-coding RNAs that are approximately 19–24 nucleotides long and are found in nearly all plants and animals (66). They function in regulating gene expression for critical cellular processes such as cell development, differentiation, expansion, survival, and function (67). The canonical function of miRs involves the mature miR, loaded into the RISC complex, interacting with target mRNA or proteins in the cell nucleus or cytoplasm, leading to altered gene expression and/or protein function (66, 68, 69). In contrast to canonical biogenesis of miRs, emerging data supports the findings that miRs can also be stably found in a variety of body fluids, such as saliva (70, 71), urine (72), and blood (73, 74) either packaged within exosomes or in complexes with RNA-binding proteins such as Ago or high-density lipoprotein. These miRs, referred to as cell-free miRs (75), may serve as biomarkers of disease (71, 72, 74) and/or facilitators of disease pathogenesis through cell-to-cell communication (75–78).
Viral single-stranded RNA oligonucleotides serve as the primary PAMPs recognized by TLR7 (mice) and TLR8 (humans) located within endosomes (79). Since miRs are short single-stranded RNA molecules, it is conceivable that miRs could function as ligands for these specific TLRs. Indeed, over the past 7 years, a small number miRs have been shown to function as TLR ligands in a variety of diseases, which will be discussed briefly. A summary of the mechanism by which miRs can function as TLR ligands is shown in Figure 1.
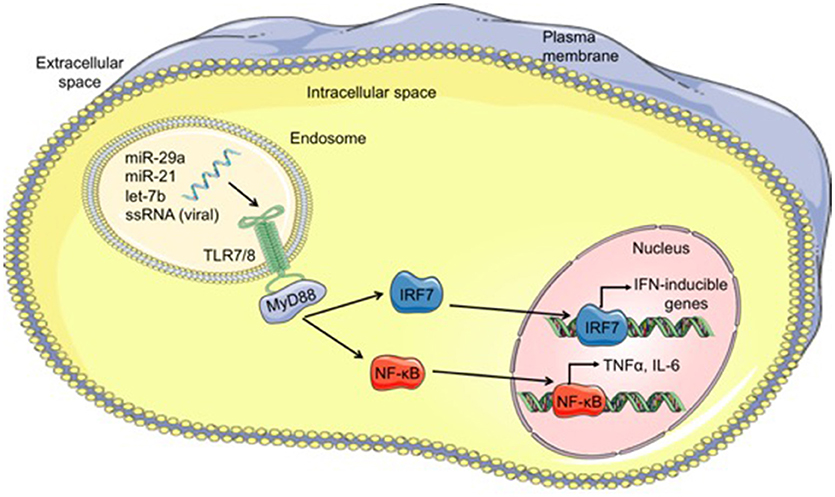
Figure 1. Mechanism of microRNAs functioning as TLR ligands. Cell-derived exosomes containing microRNAs such as let-7b, miR-21, and miR-29a, are taken into cells through endocytosis and fuse with TLR-containing endosomes within cells. The GU-rich microRNA bind to TLR7/8, activating TLR signaling through MyD88 and leading to translocation of IRF7 and NF-κB from the cytoplasm into the nucleus. Once in the nucleus, these transcription factors bind to DNA, resulting in transcription of interferon (IFN)-inducible genes and proinflammatory cytokines such as TNFα and IL-6, respectively. MicroRNA (miR), single-stranded RNA (ssRNA), Toll-like receptor 7/8 (TLR7/8), myeloid differentiation primary response 88 (MyD88), interferon regulatory factor 7 (IRF7), nuclear factor kappa-light-chain-enhancer of activated B cells (NF-κB).
In 2012, Lehmann et al. demonstrated that secreted miR let-7b functions as a TLR7 ligand in murine microglia and bone marrow-derived, leading to the induction of TNFα release and subsequent neurodegeneration (80). The authors demonstrate that all let-7 family members, not just let-7b, are able to activate TLR7 on murine microglia and propose that this is due to the presence of a conserved 3' GU-rich motif on all let-7 family members that is also present on a known TLR7 ligand, HIV ssRNA40 (79). Interestingly, let-7b was shown to be released from dying neurons in vitro, which then functioned in a paracrine manner to accelerate neuronal injury of surrounding neurons. The neuronal damage caused by let-7b release from surrounding degenerative neurons could be ameliorated by pre-treatment with a let-7b inhibitor both in vitro and in vivo when administered intrathecally. Lastly, the authors also showed elevated let-7b levels in the cerebrospinal fluid of patients with Alzheimer's disease when compared to those who did not have disease. Taken together, these findings demonstrate that let-7b serves as a novel TLR7 ligand on murine microglia, functions as a DAMP to surrounding neurons during times of neuronal injury and could be a novel therapeutic target to reduce neuronal damage.
Kim et al. investigated the role of synovial fluid let-7b in the pathogenesis of an autoimmune disease; rheumatoid arthritis (81). First, the authors identified that let-7b is markedly upregulated in synovial fluid of patients with rheumatoid arthritis. Similar to Lehmann et al., Kim et al. identified that let-7b functions as an endogenous ligand for both TLR7 and TLR8 within synovial fluid macrophages in patients with rheumatoid arthritis. Additionally, let-7b strongly stimulated TLR7-positive myeloid cells found within synovial fluid, driving their development toward pro-inflammatory M1 macrophages in a murine model of rheumatoid arthritis.
In 2012, Fabbri et al. showed that miR-21 and miR-29a are both secreted from lung cancer cell lines into cell-derived exosomes and interact with TLR-containing endosomes within macrophages at the interface between neoplastic and non-neoplastic tissues (82). Once within macrophage endosomes, miR-21, and miR-29a function as TLR ligands to activate murine TLR7 and human TLR8. In contrast to Lehmann et al. which show indirect interaction between let-7b and murine TLR7, Fabbri et al. utilized co-immunoprecipitation assays to demonstrate the direct binding of miR-21 and miR-29a to TLR7/TLR8. Functionally, the binding of miR-21 and miR-29a to TLR8 on human peripheral blood mononuclear cells induces NF-κB-dependent production of both TNFα and IL-6. Similar to let-7b and TLR7, specific short GU-rich motifs found on miR-21 and miR-29a are critical for modulating their binding to and activation of TLR8. Utilizing the inflammation-induced Lewis lung cancer mouse model, the authors demonstrate that tumor-secreted exosomal miRs, including miR-21 and miR-29a, induce murine TLR7 activation and increase the formation of lung multiplicities. Furthermore, treatment of mice with a locked nucleic acid (LNA) antimiR-21/29a significantly reduced pulmonary tumor multiplicities. Altogether, these findings directly demonstrate that miRs can function as TLR ligands, are important regulators of prometastatic inflammation, and warrant additional investigation as novel cancer therapeutic targets.
MiR29a as TLR Ligand in aGVHD
While there are many studies which describe the importance of miRs in aGVHD pathogenesis, the focus is primarily on intrinsic miR expression in immune cells such as T cells and dendritic cells (83–86). Recent data, however, suggests that miRs in circulation may serve as important modulators of pathogenesis in aGVHD.
Our group demonstrated the a novel role for serum miR-29a in aGVHD pathogenesis as a ligand for TLR7/ TLR8 on dendritic cells following allo-HSCT (84). Using two independent cohorts of patients who received allo-HSCT, we showed that miR-29a is upregulated in the serum of patients who develop aGVHD when compared to those who do not. These findings were also validated in murine models of aGVHD and it was further shown that the miR-29a was localized within serum exosomes. Using liposomal-conjugated miR-29a, we showed that murine bone marrow derived dendritic cells (BMDCs) were potently activated, as indicated by upregulation of maturation markers CD40, CD80, CD86, MHC II, and CCR7 as well as significant secretion of pro-inflammatory TNFα and IL-6. BMDCs activated by miR-29a migrated more efficiently toward CCR7 ligand CCL19 and induced stronger T cell proliferation when compared to BMDCs treated with miR-16 which served as a negative control. Because TLR signaling results in activation of My-D88-dependent transcription factors including NF-κB and IRF7, we also showed that miR-29a stimulation of murine BMDCs leads to nuclear translocation of both phosphorylated IRF7 and NF-κB-p65. Lastly, utilizing healthy donor human peripheral blood mononuclear cells (PBMCs) and monocyte-derived DCs, we demonstrated that exosomal miR-29a activates human PBMCs and DCs. To conclusively show that these findings were due to direct binding of miR-29a to human TLR8, Flag antibody tagging, and RNA immunoprecipitation were performed and confirmed marked enrichment of miR-29a in TLR8 transfected DCs only. Finally, we showed that administration of LNA antimiR-29a resulted in reduced circulating serum miR-29a, significantly improved survival and decreased clinical aGVHD severity while maintaining beneficial graft-vs.-leukemia effects in murine models of aGVHD. These findings are the first and only to demonstrate miRs as TLR ligands in the context of aGVHD and provide an exciting novel therapeutic target to prevent or treat aGVHD.
Future Direction
Allo-HSCT remains a curative modality a variety of diseases, including hematologic malignancies, myelodysplastic disorders, myeloproliferative neoplasms, and aplastic anemia (3, 87, 88). Despite this, aGVHD remains a frequent and lethal complication of allo-HSCT, underscoring the need for better understanding of aGVHD pathogenesis Furthermore, given the high morbidity and mortality associated with aGVHD, scientists and clinicians are seeking not just novel therapeutics but also novel prophylaxis. TLRs are highly conserved PRRs which are a critical aspect of the innate immune system. Traditionally, TLRs are activated by DAMPs and PAMPs, many of which are involved in the initiation of aGVHD. MiRs, being small ssRNA strands, have recently been documented as serving as ligands for TLR7 and TLR8, propagating such diseases as Alzheimer's disease, rheumatoid arthritis, and aGVHD. With the identification of miR-29a as a soluble mediator of TLR activation in aGVHD, many additional questions arise. Are there other secreted miRs which activate TLRs in the context of aGVHD? While miR-153-3p has recently been identified as a plasma miR which is upregulated and disrupts tryptophan synthesis during aGVHD, the authors do not evaluate the role of miR-153-3p on specific immune cell activation such as T cells or APCs (78). Secondly, do these miRs interact with their associated TLR similarly with specificity dependent on GU-rich motifs and how much specificity does this confer? As miRs are < 25 nt long and thousands of miRs are currently recognized, the potential for other miRs to have similar GU-rich motifs which could bind to TLRs seems highly likely. Third, can these miRs serve as novel therapeutic or prophylactic targets in diseases in which TLR7/8 play a pivotal role in pathogenesis, including aGVHD? TLR agonists and antagonists are actively being investigated as novel therapeutics for a broad range of diseases, including cancer, inflammatory disease, allergies, and infectious agents such as HIV and hepatitis C (89–92), although TLR modulation as an aGVHD therapeutic is still very much in its infancy. With this, we have the potential for gaining a better understanding aGVHD pathogenesis and identifying novel prophylactic and therapeutic targets. With all of these questions still needing answers, we have likely only brushed the surface, opening a completely new avenue of study for scientists not only in aGVHD but across many diseases in which TLR7/8 is implicated in their initiation and development.
Author Contributions
All authors listed have made a substantial, direct and intellectual contribution to the work, and approved it for publication. NZ and PR wrote the manuscript together. RG edited the manuscript.
Conflict of Interest Statement
The authors declare that the research was conducted in the absence of any commercial or financial relationships that could be construed as a potential conflict of interest.
References
1. Martin PJ, Rizzo JD, Wingard JR, Ballen K, Curtin PT, Cutler C, et al. First- and second-line systemic treatment of acute graft-versus-host disease: recommendations of the American Society of Blood and Marrow Transplantation. Biol Blood Marrow Transplant. (2012) 18:1150–63. doi: 10.1016/j.bbmt.2012.04.005
2. Ferrara JL, Levine JE, Reddy P, Holler E. Graft-versus-host disease. Lancet (2009) 373:1550–61. doi: 10.1016/S0140-6736(09)60237-3
3. , D'Souza A, Fretham C. Current Uses and Outcomes of Hematopoietic Cell Transplantation (HCT): CIBMTR Summary Slides (2017). Available online at: http://www.cibmtr.org
4. Malard F, Chevallier P, Guillaume T, Delaunay J, Rialland F, Harousseau JL, et al. Continuous reduced nonrelapse mortality after allogeneic hematopoietic stem cell transplantation: a single-institution's three decade experience. Biol Blood Marrow Transplant. (2014) 20:1217–23. doi: 10.1016/j.bbmt.2014.04.021
5. Khoury HJ, Wang T, Hemmer MT, Couriel D, Alousi A, Cutler C, et al. Improved survival after acute graft-versus-host disease diagnosis in the modern era. Haematologica (2017) 102:958–66. doi: 10.3324/haematol.2016.156356
6. Jamani K, Russell JA, Daly A, Stewart D, Savoie L, Duggan P, et al. Prognosis of grade 3-4 acute GVHD continues to be dismal. Bone Marrow Transplant. (2013) 48:1359–61. doi: 10.1038/bmt.2013.59
7. Castilla-Llorente C, Martin PJ, McDonald GB, Storer BE, Appelbaum FR, Deeg HJ, et al. Prognostic factors and outcomes of severe gastrointestinal GVHD after allogeneic hematopoietic cell transplantation. Bone Marrow Transplant. (2014) 49:966–71. doi: 10.1038/bmt.2014.69
8. Zeiser R, Blazar BR. Acute graft-versus-host disease — biologic process, prevention, and therapy. N Engl J Med. (2017) 377:2167–79. doi: 10.1056/NEJMra1609337
9. Storb R, Deeg HJ, Whitehead J, Appelbaum F, Beatty P, Bensinger W, et al. Methotrexate and cyclosporine compared with cyclosporine alone for prophylaxis of acute graft versus host disease after marrow transplantation for leukemia. N Engl J Med. (1986) 314:729–35. doi: 10.1056/NEJM198603203141201
10. Ruutu T, Van Biezen A, Hertenstein B, Henseler A, Garderet L, Passweg J, et al. Prophylaxis and treatment of GVHD after allogeneic haematopoietic SCT: a survey of centre strategies by the European Group for Blood and Marrow Transplantation. Bone Marrow Transplant. (2012) 47:1459–64. doi: 10.1038/bmt.2012.45
11. Quellmann S, Schwarzer G, Hübel K, Engert A, Bohlius J. Corticosteroids in the prevention of graft-vs-host disease after allogeneic myeloablative stem cell transplantation: a systematic review and meta-analysis. Leukemia (2008) 22:1801–3. doi: 10.1038/leu.2008.105
12. Ruutu T, Gratwohl A, de Witte T, Afanasyev B, Apperley J, Bacigalupo A, et al. Prophylaxis and treatment of GVHD: EBMT-ELN working group recommendations for a standardized practice. Bone Marrow Transplant. (2014) 49:168–73. doi: 10.1038/bmt.2013.107
13. Dignan FL, Clark A, Amrolia P, Cornish J, Jackson G, Mahendra P, et al. Diagnosis and management of acute graft-versus-host disease. Br J Haematol. (2012) 158:30–45. doi: 10.1111/j.1365-2141.2012.09129.x
14. Deeg HJ. How I treat refractory acute GVHD. Blood (2007) 109:4119–26. doi: 10.1182/blood-2006-12-041889
15. Pavletic SZ, Fowler DH. GVHD - are we making progress in GVHD prophylaxis and treatment? Hematology Am Soc Hematol Educ Prog. (2012) 2012:251–64. doi: 10.1182/asheducation-2012.1.251
16. Ram R, Storb R. Pharmacologic prophylaxis regimens for acute graft-versus-host disease: past, present and future. Leuk Lymphoma (2013) 54:1591–601. doi: 10.3109/10428194.2012.762978
17. Murphy S, Nguyen VH. Role of gut microbiota in graft-versus-host disease. Leuk Lymphoma (2011) 52:1844–56. doi: 10.3109/10428194.2011.580476
18. Kornblit B, Müller K. Sensing danger: Toll-like receptors and outcome in allogeneic hematopoietic stem cell transplantation. Bone Marrow Transplant. (2017) 52:499–505. doi: 10.1038/bmt.2016.263
19. Blazar BR, Murphy WJ, Abedi M. Advances in graft-versus-host disease biology and therapy. Nat Rev Immunol. (2012) 12:443–58. doi: 10.1038/nri3212
20. Welniak LA, Blazar BR, Murphy WJ. Immunobiology of allogeneic hematopoietic stem cell transplantation. Annu Rev Immunol. (2007) 25:139–70. doi: 10.1146/annurev.immunol.25.022106.141606
21. Janeway CA. Approaching the asymptote? evolution and revolution in immunology. Cold Spring Harb Symp Quant Biol. (1989) 54:1–13. doi: 10.1101/SQB.1989.054.01.003
22. Hashimoto C, Hudson KL, Anderson K V. The Toll gene of drosophila, required for dorsal-ventral embryonic polarity, appears to encode a transmembrane protein. Cell (1988) 52:269–79. doi: 10.1016/0092-8674(88)90516-8
23. Belvin MP, Anderson K V. A conserved signaling pathway: the Drosophila toll-dorsal pathway. Annu Rev Cell Dev Biol. (1996) 12:393–416. doi: 10.1146/annurev.cellbio.12.1.393
24. Lemaitre B, Nicolas E, Michaut L, Reichhart JM, Hoffmann JA. The dorsoventral regulatory gene cassette spatzle/Toll/Cactus controls the potent antifungal response in Drosophila adults. Cell (1996) 86:973–83. doi: 10.1016/S0092-8674(00)80172-5
25. Medzhitov R, Preston-Hurlburt P, Janeway CAJ. A human homologue of the Drosophila activation of adaptive immunity. Nature (1997) 388:394–7.
26. Qureshi ST, Larivière L, Leveque G, Clermont S, Moore KJ, Gros P, et al. Endotoxin-tolerant mice have mutations in toll-like receptor 4 ( Tlr4 ). J Exp Med. (1999) 189:615–25. doi: 10.1084/jem.189.4.615
27. Poltorak A, He X, Smirnova I, Liu MY, Van Huffel C, Du X, et al. Defective LPS signaling in C3H/HeJ and C57BL/10ScCr mice: mutations in Tlr4 gene. Science (1998) 282:2085–8. doi: 10.1126/science.282.5396.2085
28. Blasius AL, Beutler B. Intracellular toll-like receptors. Immunity (2010) 32:305–15. doi: 10.1016/j.immuni.2010.03.012
29. Kawai T, Akira S. The role of pattern-recognition receptors in innate immunity: update on toll-like receptors. Nat Immunol. (2010) 11:373–84. doi: 10.1038/ni.1863
30. Zeiser R, Socié G, Blazar BR. Pathogenesis of acute graft-versus-host disease: from intestinal microbiota alterations to donor T cell activation. Br J Haematol. (2016) 175:191–207. doi: 10.1111/bjh.14295
31. Paczesny S, Hanauer D, Sun Y, Reddy P. New perspectives on the biology of acute GVHD. Bone Marrow Transplant. (2010) 45:1–11. doi: 10.1038/bmt.2009.328
32. Gyurkocza B, Sandmaier BM. Conditioning regimens for hematopoietic cell transplantation : one size does not fit all. Blood (2014) 124:344–53. doi: 10.1182/blood-2014-02-514778
33. Boussios S, Pentheroudakis G, Katsanos K, Pavlidis N. Systemic treatment-induced gastrointestinal toxicity: incidence, clinical presentation and management. Ann Gastroenterol Ann Gastroenterol. (2012) 25:106–18.
34. Rubin P, Casarett GW. Clinical radiation pathology as applied to curative radiotherapy. Cancer (1968) 22:767–78.
35. Fajardo LF. The pathology of ionizing radiation as defined by morphologic patterns. Acta Oncol. (2005) 44:13–22. doi: 10.1080/02841860510007440
36. Mitchell EP. Gastrointestinal toxicity of chemotherapeutic agents. Semin Oncol. (2006) 33:106–20. doi: 10.1053/j.seminoncol.2005.12.001
37. Van der Velden WJ, Herbers AH, Netea MG, Blijlevens NM. Mucosal barrier injury, fever and infection in neutropenic patients with cancer: introducing the paradigm febrile mucositis. Br J Haematol. (2014) 167:441–52. doi: 10.1111/bjh.13113
38. Guzman-Stein G, Bonsack M, Liberty J, Delaney JP. Abdominal radiation causes bacterial translocation. J Surg Res. (1989) 46:104–7. doi: 10.1016/0022-4804(89)90211-4
39. Xun BCQ, Thompson JS, Jennings CD, Brown SA, Widmer MB. Effect of total body irradiation, busulfan-cyclophosphamide, or cyclophosphamide conditioning on inflammatory cytokine release and development of acute and chronic graft-versus-host disease in H-2-incompatible transplanted SCID mice. Blood (1994) 83:2360–7.
40. Ramadan A, Paczesny S. Various forms of tissue damage and danger signals following hematopoietic stem-cell transplantation. Front Immunol. (2015) 6:14. doi: 10.3389/fimmu.2015.00014
41. Toubai T, Mathewson ND, Magenau J, Reddy P. Danger signals and graft-versus-host disease: current understanding and future perspectives. Front Immunol. (2016) 7:539. doi: 10.3389/fimmu.2016.00539
42. Zeiser R. Activation of innate immunity in graft-versus-host disease: Implications for novel targets? Oncol Res Treat. (2015) 38:239–43. doi: 10.1159/000381296
44. Chen GY, Nuñez G. Sterile inflammation: sensing and reacting to damage. Nat Rev Immunol. (2010) 10:826–37. doi: 10.1038/nri2873
45. Quintana FJ, Cohen IR. Heat shock proteins as endogenous adjuvants in sterile and septic inflammation. J Immunol. (2005) 175:2777–82. doi: 10.4049/jimmunol.175.5.2777
46. Bours MJ, Swennen EL, Di Virgilio F, Cronstein BN, Dagnelie PC. Adenosine 5′-triphosphate and adenosine as endogenous signaling molecules in immunity and inflammation. Pharmacol Ther. (2006) 112:358–404. doi: 10.1016/j.pharmthera.2005.04.013
47. Kelsh RM, McKeown-Longo PJ. Topographical changes in extracellular matrix: activation of TLR4 signaling and solid tumor progression. Trends Cancer Res. (2013) 29:997–1003.
48. Maeda Y. Pathogenesis of graft-versus-host disease: innate immunity amplifying acute alloimmune responses. Int J Hematol. (2013) 98:293–9. doi: 10.1007/s12185-013-1421-x
49. Land WG. Emerging role of innate immunity in organ transplantation. Part I: evolution of innate immunity and oxidative allograft injury. Transplant Rev. (2012) 26:60–72. doi: 10.1016/j.trre.2011.05.001
50. Heidegger S, van den Brink MR, Haas T, Poeck H. The role of pattern-recognition receptors in graft-versus-host disease and graft-versus-leukemia after allogeneic stem cell transplantation. Front Immunol. (2014) 5:337. doi: 10.3389/fimmu.2014.00337
51. Chakraverty R, Sykes M. The role of antigen-presenting cells in triggering graft-versus-host disease and graft-versus-leukemia. Blood (2007) 110:9–17. doi: 10.1182/blood-2006-12-022038
52. Tu S, Zhong D, Xie W, Huang W, Jiang Y, Li Y. Role of toll-like receptor signaling in the pathogenesis of graft-versus-host diseases. Int J Mol Sci. (2016) 17:E1288. doi: 10.3390/ijms17081288
53. Lorenz E, Schwartz DA, Martin PJ, Gooley T, Lin MT, Chien JW, et al. Association of TLR4 mutations and the risk for acute GVHD after HLA- matched-sibling hematopoietic stem cell transplantation. Biol Blood Marrow Transplant. (2001) 7:384–7. doi: 10.1053/bbmt.2001.v7.pm11529488
54. Elmaagacli AH, Koldehoff M, Hindahl H, Steckel NK, Trenschel R, Peceny R, et al. Mutations in innate immune system NOD2/CARD 15 and TLR-4 (Thr399Ile) genes influence the risk for severe acute graft-versus-host disease in patients who underwent an allogeneic transplantation. Transplantation (2006) 81:247–54. doi: 10.1097/01.tp.0000188671.94646.16
55. Xiao HW, Luo Y, Lai XY, Shi JM, Tan YM, He JS, et al. Donor TLR9 gene tagSNPs influence susceptibility to aGVHD and CMV reactivation in the allo-HSCT setting without polymorphisms in the TLR4 and NOD2 genes. Bone Marrow Transplant. (2014) 49:241–7. doi: 10.1038/bmt.2013.160
56. Sivula J, Cordova ZM, Tuimala J, Jaatinen T, Partanen J, Volin L, et al. Toll-like receptor gene polymorphisms confer susceptibility to graft-versus-host disease in allogenic hematopoietic stem cell transplantation. Scand J Immunol. (2012) 76:336–41. doi: 10.1111/j.1365-3083.2012.02737.x
57. Zhao Y, Liu Q, Yang L, He D, Wang L, Tian J, et al. TLR4 inactivation protects from graft-versus-host disease after allogeneic hematopoietic stem cell transplantation. Cell Mol Immunol. (2013) 10:165–75. doi: 10.1038/cmi.2012.58
58. Janciauskiene S, Larsson S, Larsson P, Virtala R, Jansson L, Stevens T. Inhibition of lipopolysaccharide-mediated human monocyte activation, in vitro, by α1-antitrypsin. Biochem Biophys Res Commun. (2004) 321:592–600. doi: 10.1016/j.bbrc.2004.06.123
59. Jonigk D, Al-Omari M, Maegel L, Muller M, Izykowski N, Hong J, et al. Anti-inflammatory and immunomodulatory properties of 1-antitrypsin without inhibition of elastase. Proc Natl Acad Sci USA. (2013) 110:15007–12. doi: 10.1073/pnas.1309648110
60. Nita IM, Serapinas D, Janciauskiene SM. α1-Antitrypsin regulates CD14 expression and soluble CD14 levels in human monocytes in vitro. Int J Biochem Cell Biol. (2007) 39:1165–76. doi: 10.1016/j.biocel.2007.02.017
61. Brennan TV, Lin L, Huang X, Cardona DM, Li Z, Dredge K, et al. Heparan sulfate, an endogenous TLR4 agonist, promotes acute GVHD after allogeneic stem cell transplantation. Blood (2012) 120:2899–908. doi: 10.1182/blood-2011-07-368720
62. Calcaterra C, Sfondrini L, Rossini A, Sommariva M, Rumio C, Ménard S, et al. Critical role of TLR9 in acute graft-versus-host disease. J Immunol. (2008) 181:6132–9. doi: 10.4049/jimmunol.181.9.6132
63. Taylor PA, Ehrhardt MJ, Lees CJ, Panoskaltsis-Mortari A, Krieg AM, Sharpe AH, et al. TLR agonists regulate alloresponses and uncover a critical role for donor APCs in allogeneic bone marrow rejection. Blood (2008) 112:3508–16. doi: 10.1182/blood-2007-09-113670
64. Chakraverty R, Côté D, Buchli J, Cotter P, Hsu R, Zhao G, et al. An inflammatory checkpoint regulates recruitment of graft-versus-host reactive T cells to peripheral tissues. J Exp Med. (2006) 203:2021–31. doi: 10.1084/jem.20060376
65. Jasperson LK, Bucher C, Panoskaltsis-Mortari A, Mellor AL, Munn DH, Blazar BR. Inducing the tryptophan catabolic pathway, indoleamine 2,3-dioxygenase (IDO), for suppression of graft-versus-host disease (GVHD) lethality. Blood (2009) 114:5062–70. doi: 10.1182/blood-2009-06-227587
66. Bartel DP. MicroRNAs: genomics, biogenesis, mechanism, and function. Cell (2004) 116:281–97. doi: 10.1016/S0092-8674(04)00045-5
67. Garzon R, Marcucci G, Croce CM. Targeting microRNAs in cancer: rationale, strategies and challenges. Nat Rev Drug Discov. (2010) 9:775–89. doi: 10.1038/nrd3179
68. Lin S, Gregory RI. MicroRNA biogenesis pathways in cancer. Nat Rev Cancer (2015) 15:321–33. doi: 10.1038/nrc3932
69. Garzon R, Calin GA, Croce CM. MicroRNAs in Cancer. Annu Rev Med. (2009) 60:167–79. doi: 10.1146/annurev.med.59.053006.104707
70. Gallo A, Tandon M, Alevizos I, Illei GG. The majority of microRNAs detectable in serum and saliva is concentrated in exosomes. PLoS ONE (2012) 7:e30679. doi: 10.1371/journal.pone.0030679
71. Michael A, Bajracharya SD, Yuen PS, Zhou H, Star RA, Illei GG, et al. Exosomes from human saliva as a source of microRNA biomarkers. Oral Dis. (2010) 16:34–8. doi: 10.1111/j.1601-0825.2009.01604.x
72. Lv LL, Cao Y, Liu D, Xu M, Liu H, Tang RN, et al. Isolation and quantification of MicroRNAs from urinary exosomes/microvesicles for biomarker discovery. Int J Biol Sci. (2013) 9:1021–31. doi: 10.7150/ijbs.6100
73. Mitchell PS, Parkin RK, Kroh EM, Fritz BR, Wyman SK, Pogosova-Agadjanyan EL, et al. Circulating microRNAs as stable blood-based markers for cancer detection. Proc Natl Acad Sci USA. (2008) 105:10513–8. doi: 10.1073/pnas.0804549105
74. Gilad S, Meiri E, Yogev Y, Benjamin S, Lebanony D, Yerushalmi N, et al. Serum microRNAs are promising novel biomarkers. PLoS ONE (2008) 3:e3148. doi: 10.1371/journal.pone.0003148
75. Schwarzenbach H, Nishida N, Calin GA, Pantel K. Clinical relevance of circulating cell-free microRNAs in cancer. Nat Rev Clin Oncol. (2014) 11:145–56. doi: 10.1038/nrclinonc.2014.5
76. Ha TY. The role of MicroRNAs in regulatory T cells and in the immune response. Immune Netw. (2011) 11:11–41. doi: 10.4110/in.2011.11.1.11
77. Hannafon BN, Ding WQ. Intercellular communication by exosome-derived microRNAs in cancer. Int J Mol Sci. (2013) 14:14240–69. doi: 10.3390/ijms140714240
78. Zhao X, Wang Y, Lv M, Kong Y, Luo H, Ye X, et al. miR-153-3p, a new bio-target, is involved in the pathogenesis of acute graft-versus-host disease via inhibition of indoleamine- 2,3-dioxygenase. Oncotarget (2016) 7:48321–34. doi: 10.18632/oncotarget.10220
79. Heil F. Species-specific recognition of single-stranded RNA via toll-like receptor 7 and 8. Science (2004) 303:1526–9. doi: 10.1126/science.1093620
80. Lehmann SM, Krüger C, Park B, Derkow K, Rosenberger K, Baumgart J, et al. An unconventional role for miRNA: let-7 activates toll-like receptor 7 and causes neurodegeneration. Nat Neurosci. (2012) 15:827–35. doi: 10.1038/nn.3113
81. Kim S, Chen Z, Essani AB, Elshabrawy HA, Volin MV, Volkov S, et al. Identification of a novel TLR7 endogenous ligand in RA synovial fluid that can provoke arthritic joint inflammation. Arthritis Rheumatol. (2015) 68:1099–110. doi: 10.1002/art.39544
82. Fabbri M, Paone A, Calore F, Galli R, Gaudio E, Santhanam R, et al. MicroRNAs bind to Toll-like receptors to induce prometastatic inflammatory response. Proc Natl Acad Sci USA. (2012) 109:E2110–6. doi: 10.1073/pnas.1209414109
83. Leonhardt F, Grundmann S, Behe M, Bluhm F, Dumont RA, Braun F, et al. Inflammatory neovascularization during graft-versus-host disease is regulated by v integrin and miR-100. Blood (2013) 121:3307–18. doi: 10.1182/blood-2012-07-442665
84. Ranganathan P, Ngankeu A, Zitzer NC, Leoncini P, Yu X, Casadei L, et al. Serum miR-29a is upregulated in acute graft-versus-host disease and activates dendritic cells through TLR binding. J Immunol. (2017) 198:2500–12. doi: 10.4049/jimmunol.1601778
85. Stickel N, Prinz G, Pfeifer D, Hasselblatt P, Schmitt-Graeff A, Follo M, et al. MiR-146a regulates the TRAF6/TNF-axis in donor T cells during GVHD. Blood (2014) 124:2586–95. doi: 10.1182/blood-2014-04-569046
86. Wang L, Romero M, Ratajczak P, Lebœuf C, Belhadj S, Peffault de Latour R, et al. Increased apoptosis is linked to severe acute GVHD in patients with Fanconi anemia. Bone Marrow Transplant. (2013) 48:849–53. doi: 10.1038/bmt.2012.237
87. Majhail NS, Farnia SH, Carpenter PA, Champlin RE, Crawford S, Marks DI, et al. Indications for autologous and allogeneic hematopoietic cell transplantation: guidelines from the american society for blood and marrow transplantation. Biol Blood Marrow Transplant. (2015) 21:1863–69. doi: 10.1016/j.bbmt.2015.07.032
88. Sureda A, Bader P, Cesaro S, Dreger P, Duarte RF, Dufour C, et al. Indications for allo- and auto-SCT for haematological diseases, solid tumours and immune disorders: current practice in Europe, 2015. Bone Marrow Transplant. (2015) 50:1037–56. doi: 10.1038/bmt.2015.6
89. Li K, Qu S, Chen X, Wu Q, Shi M. Promising targets for cancer immunotherapy: TLRs, RLRs, and STING-mediated innate immune pathways. Int J Mol Sci. (2017) 18:E404. doi: 10.3390/ijms18020404
90. Iribarren K, Bloy N, Buqué A, Cremer I, Eggermont A, Fridman WH, et al. Trial watch: immunostimulation with toll-like receptor agonists in cancer therapy. Oncoimmunology (2016) 5:e1088631. doi: 10.1080/2162402X.2015.1088631
91. Gao W, Xiong Y, Li Q, Yang H. Inhibition of toll-like receptor signaling as a promising therapy for inflammatory diseases: a journey from molecular to nano therapeutics. Front Physiol. (2017) 8:508. doi: 10.3389/fphys.2017.00508
Keywords: graft-vs.-host disease, Toll-like receptors, microRNAs, allogeneic stem cell transplantation, innate immunity
Citation: Zitzer NC, Garzon R and Ranganathan P (2018) Toll-Like Receptor Stimulation by MicroRNAs in Acute Graft-vs.-Host Disease. Front. Immunol. 9:2561. doi: 10.3389/fimmu.2018.02561
Received: 16 June 2018; Accepted: 17 October 2018;
Published: 05 November 2018.
Edited by:
Flavia Bazzoni, Università di Verona, ItalyPeter A. Ward, University of Michigan, United States
Reviewed by:
Claudio Lunardi, Università degli Studi di Verona, ItalyCopyright © 2018 Zitzer, Garzon and Ranganathan. This is an open-access article distributed under the terms of the Creative Commons Attribution License (CC BY). The use, distribution or reproduction in other forums is permitted, provided the original author(s) and the copyright owner(s) are credited and that the original publication in this journal is cited, in accordance with accepted academic practice. No use, distribution or reproduction is permitted which does not comply with these terms.
*Correspondence: Parvathi Ranganathan, cGFydmF0aGkucmFuZ2FuYXRoYW5Ab3N1bWMuZWR1