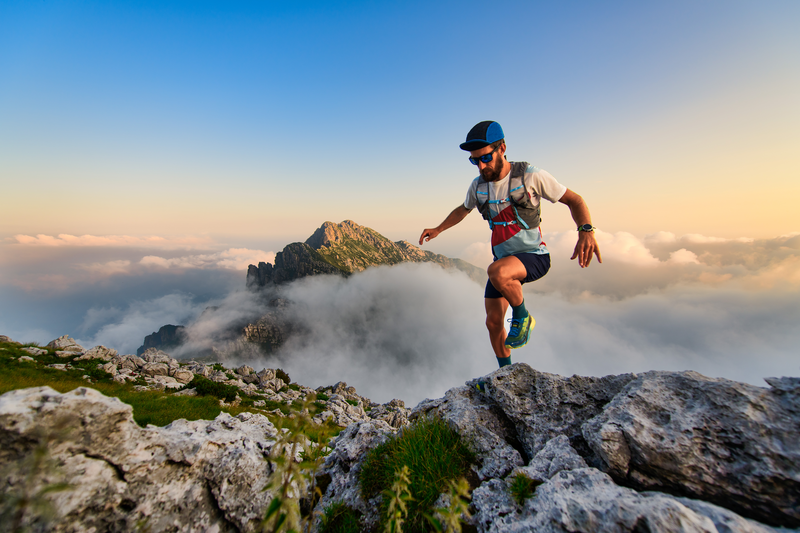
95% of researchers rate our articles as excellent or good
Learn more about the work of our research integrity team to safeguard the quality of each article we publish.
Find out more
REVIEW article
Front. Immunol. , 22 June 2018
Sec. Viral Immunology
Volume 9 - 2018 | https://doi.org/10.3389/fimmu.2018.01452
This article is part of the Research Topic Immune Surveillance of the HIV Reservoir: Mechanisms, Therapeutic Targeting and New Avenues for HIV Cure View all 9 articles
Antiretroviral therapy regimens durably suppress HIV replication, but do not cure infection. This is partially attributable to the persistence of long-lived pools of resting CD4+ T-cells harboring latent replication-competent virus. Substantial clinical and pre-clinical research is currently being directed at purging this viral reservoir by combining pharmacological latency reversal with immune effectors, such as HIV-specific CD8+ T-cells, capable of eliminating reactivated targets—the so-called “shock-and-kill” approach. However, several studies indicate that the latency-reversing agents (LRAs) may affect CD8+ T-cell function. The current review aims to frame recent advances, and ongoing challenges, in implementing “shock-and-kill” strategies from the perspective of effectively harnessing CD8+ T-cells. We review and contextualize findings indicating that LRAs often have unintended impacts on CD8+ T-cell function, both detrimental and beneficial. We identify and attempt to bridge the gap between viral reactivation, as measured by the detection of RNA or protein, and bona fide presentation of viral antigens to CD8+ T-cells. Finally, we highlight factors on the effector (CD8+) and target (CD4+) cell sides that contribute to whether or not infected-cell recognition results in killing/elimination. These perspectives may contribute to an integrated view of “shock-and-kill,” with implications for therapeutic development.
Antiretroviral therapy (ART) has transformed the lives of people living with HIV; however, early hopes that ART might provide a sterilizing cure have proven unfounded (1). A barrier to cure are long-lived populations of latently infected cells harboring integrated provirus, which are not actively producing virions (and, therefore, are impervious to both ART and the immune system) but retain the capacity to do so (2–4). If ART is interrupted, spontaneous activation of these latent cells can lead to viral rebound (5–7).
Recent findings have reignited hopes that a cure for HIV may be possible. Several putative latency-reversing agents (LRAs) have been shown to induce viral RNA production in participants with undetectable viral load on ART (8–10). However, to date most studies have not reported a reduction in the frequency of latently infected cells (as measured by cell-associated replication-competent virus or HIV DNA) following LRA treatment, suggesting that latency reversal alone is unlikely to clear the reservoir. Instead, reactivated cells may need to be actively eliminated by the immune system, a strategy that is known as “shock-and-kill” but may be more precisely characterized as “latency reversal and clearance” (11–13). Supporting this notion, a study using an in vitro model of HIV latency demonstrated that latent cells reactivated using Vorinostat did not die from viral cytopathic effects, but could be killed by HIV-specific CD8+ T-cells (14).
CD8+ T-cells can detect and kill virally infected cells with exquisite sensitivity, can be boosted by immunization, and form long-lived “memory” populations capable of rapidly responding to subsequent viral encounters (15, 16). In acute HIV infection, the emergence of HIV-specific CD8+ T-cells coincides with the decline of virus load from peak to set point (17–19), and CD8+ T-cells targeting conserved regions of the HIV proteome (from which the virus is unable to escape without a fitness cost) have been associated with superior virus control in long-term non-progressors (20–25). Furthermore, in a presentation to the 2017 Conference on Retroviruses and Opportunistic Infections, Mothe et al. reported delayed viral rebound following ART interruption in clinical trial participants who received the LRA Romidepsin in combination with a vaccine designed to elicit HIV-specific CD8+ T-cells (26). The vaccine regimen boosted HIV-specific T-cell responses in all participants, and 4 out of 11 were able to maintain viral loads below 2,000 copies/ml for at least 7 weeks after ART interruption, suggesting that the regimen may have impacted the viral reservoir. Thus, HIV-specific CD8+ T-cells are excellent candidates for a HIV cure strategy. However, we and others have reported that some LRAs may have detrimental effects on CD8+ T-cell function, potentially compromising the clearance of reactivated cells. Here, we summarize the current literature, focusing on two leading classes of LRAs: histone deacetylase inhibitors (HDACis) and protein kinase C agonists (PCKa, sometimes also referred to as PKC modulators).
Histone deacetylase inhibitors block the removal of selected histone acetylation marks, which both allows the recruitment of transcriptional coactivators and inhibits the recruitment of chromosomal silencing complexes (27). Three HDACis (Vorinostat, Romidepsin, and Panobinostat) have been tested as LRAs in clinical trials. PKCa bind to and activate various protein kinase C isoforms, triggering multiple signaling cascades that result in the activation of transcription factors, such as NFκB and ERK1/2 (28). We will discuss three subclasses of PKCa, Bryostatin-1, Prostratin, and Ingenols [primarily Ingenol-B and Ingenol 3,20-dibenzoate (Ingenol-db), two of several Ingenol derivatives proposed as candidate HIV LRAs]. To date, only Bryostatin-1 has been tested as an LRA in clinical trials; the drug failed to enhance PKC activity or increase detection of cell-associated unspliced HIV RNA, indicating that the infusion did not achieve an effective in vivo exposure (29). We will summarize both in vivo and in vitro findings, focusing mostly on studies utilizing primary T-cells and clones, and considering all stages of the T-cell response, from presentation of viral peptides by the infected cell to killing orchestrated by HIV-specific CD8+ T-cells (Figure 1).
Figure 1. Summary of the effects of latency-reversing agents (LRAs) on antigen-specific CD8+ T-cells in vitro. LRAs can either enhance (blue arrows) or inhibit (red bars) multiple facets of the CD8+ T-cell response. Some of these observations have yet to be confirmed by clinical studies.
CD8+ T-cells detect virally infected cells via their T-cell receptor (TCR), which recognizes viral peptide (antigen) presented at the infected-cell surface by major histocompatibility class I (MHC-I) molecules (30, 31). Each T-cell population recognizes a specific peptide-MHC combination. For clearance of latently infected cells by CD8+ T-cells to occur, a LRA must induce expression of viral protein that is appropriately presented by MHC-I for a sufficient period of time to be recognized by functional HIV-specific CD8+ T-cells. Notably, HIV virion production is not a prerequisite for viral antigen expression, as resting CD4+ T-cells can transcribe and translate HIV proteins without producing infectious virions, and we and others have previously observed killing of targets infected with replication-defective virus by HIV-specific CD8+ T-cell clones (32–34). The degree to which current latency-reversing regimens induce viral protein production remains uncertain, as the first clinical studies demonstrating latency reversal by HDACis reported increases in viral RNA but did not measure protein (8–10, 35). However, subsequent studies have documented at least some virion release (36).
It is currently unclear whether HDACis such as Vorinostat induce sufficient viral antigen production for recognition of latently infected cells by HIV-specific CD8+ T-cells. Conflicting results have been reported, possibly due to differences in model systems and methods of quantification. For example, using a primary cell latency model, we (Jones and colleagues) recently reported no increase in HIV Gag p24 levels, as measured by ELISA, following Vorinostat treatment (37). However, using a new, highly sensitive digital ELISA method, quantifiable increases in p24 were detected ex vivo in resting CD4+ T-cells from ART-suppressed study participants who had received Vorinostat or Panobinostat (36). This suggests that Vorinostat may induce low-level antigen expression below the limit of detection of traditional assays. In contrast, Prostratin treatment induced HIV p24 production at sufficient levels to be measured by standard ELISA (37). Collectively, these studies suggest that LRAs are capable of inducing viral protein expression, but whether this expression occurs at a sufficient level to enable detection by CD8+ T-cells remains inconclusive.
Viral protein expression may not lead to effective antigen presentation. To be detected by HIV-specific CD8+ T-cells, viral antigen must be appropriately processed and presented at the infected-cell surface by MHC-I molecules. The HDACis Panobinostat and Romidepsin transiently reduce MHC-I expression on CD4+ T-cells in vitro, whereas PKCa increase expression over several days (38). This may be related to differential activity of peptidases (enzymes that trim peptides prior to loading onto MHC molecules) under these conditions, and thus availability of peptides for MHC-I loading. HDACi treatment of primary CD4+ T-cells results in a 20–60% reduction in peptidase activity compared with untreated cells, whereas this activity is enhanced in cells treated with the PKCa Bryostatin-1 (Boucau et al. NIAID Strategies for an HIV Cure Meeting, 2016). These effects may dampen the abilities of HDACis to induce T-cell recognition of infected cells, while further boosting antigen presentation by PKCa-treated CD4+ T-cells. An alternative approach is to directly quantify antigen presentation by the infected cell. Specific peptide-MHC combinations can be visualized using fluorescently tagged synthetic TCRs (39). Using this method, Yang et al. reported that up to 50 MHC-I complexes presenting the HIV Gag SL9 peptide could be detected on the surface of CD4+ T-cells that had been infected with HIV in vitro (40). This method could be utilized to investigate whether antigen is presented at a comparable level on reactivated latently infected cells, whether there are differences in the level of antigen presentation induced by different LRAs, and, crucially, the timing and duration of antigen presentation following LRA administration.
The third consideration when assessing the impact of putative LRAs on HIV antigen expression is whether enough peptide-antigen/MHC complexes are present on the cell surface to facilitate recognition and killing of the infected cell by HIV-specific CD8+ T-cells. Imaging studies of individual cells have shown that three or fewer peptide-MHC complexes can trigger T-cell cytokine production and killing (16, 41, 42), raising the possibility that reactivated infected cells may be recognized by HIV-specific CD8+ T-cells even if expression of the corresponding protein cannot be directly measured by conventional assays. Investigators have begun to address this issue by using CD8+ T-cells as “biosensors” for antigen expression, providing a readout that is directly relevant to “shock-and-kill” strategies. Latently infected CD4+ T-cells are exposed to LRAs, washed, and then co-cultured with CD8+ T-cells to determine whether the cells have been reactivated sufficiently to trigger cytokine production, virus inhibition, or killing. Since HIV-infected cells are typically present at very low frequencies in ART-treated individuals, investigators have usually employed primary cell latency models (where CD4+ T-cells from participants are superinfected and then allowed to recover to a latent state over several days) to assess CD8+ T-cell recognition and infected-cell elimination. Using such a model, Shan et al. demonstrated that antigen-pre-stimulated HIV-specific CD8+ T-cell lines effectively eliminated Vorinostat-reactivated infected cells (14). This finding was supported by an ex vivo study showing that Vorinostat sensitized resting CD4+ T-cells from HIV-infected individuals on ART to elimination by autologous CD8+ T-cells (43). In contrast, we (Jones and colleagues) were unable to observe recognition of Vorinostat- or Panobinostat-treated cells by HIV-specific CD8+ T-cell clones, whereas the PKCa Prostratin effectively primed latently infected cells for CD8+ T-cell recognition, as measured by IFN-γ production (37). These conflicting results could be explained by the differences in the latency model, reporter viruses, and HIV-specific CD8+ T-cells (cell lines versus clones) used in the two in vitro studies. Interestingly, HDACis induced detectable HIV protein expression in the first in vitro study (where Vorinostat-activated cells could be cleared by CD8+ T-cells) but not the second, suggesting that measurable protein expression is a useful indicator of the vulnerability of latently infected cells to clearance in vitro.
Even if LRAs induce appropriate antigen production and presentation, clearance could be limited by insufficient frequencies of HIV-specific CD8+ T-cells in HIV-infected individuals on ART [Xu et al., manuscript in preparation (44)]. Additionally, in chronically infected individuals, we and others have observed that HIV-specific CD8+ T-cells exhibit signs of dysfunction that may impair their capacities to eliminate reactivated cells (45–48). To overcome these limitations, researchers have developed bispecific molecules combining a HIV-recognizing component with an element that binds CD3 (part of the TCR complex), so that T-cells of any specificity can be recruited to eliminate latent cells. Using a high-avidity HIV-specific TCR fused with a CD3-specific single chain antibody fragment, Yang et al. reported that resting CD4+ T-cells from HIV-infected individuals on ART pre-treated with Romidepsin plus Bryostatin-1 could be killed by CD8+ T-cells from HIV-seronegative donors (who lack HIV-specific CD8+ T-cells) (40). Similarly, a bispecific antibody targeting HIV gp120 and CD3 facilitated clearance of Vorinostat- or Panobinostat-exposed CD4+ T-cells from HIV+ ART-treated participants by autologous CD8+ T-cells (36). This suggests that these LRA regimens stimulated sufficient antigen presentation for killing in a situation where TCR recognition is optimal and CD8+T-cell numbers are not limiting.
Finally, antigen-presenting cells (and other cells) can modulate antigen-induced T-cell activation by expressing costimulatory or inhibitory molecules (referred to as signal 2) and by producing cytokines (signal 3) (49–51). HDACis and PKCa have been shown to impact signal 2 and signal 3 in cancer and other settings. Vorinostat inhibits expression of the costimulatory molecule CD80 on antigen-presenting cells, though only at supraphysiological doses (52). Vorinostat and Romidepsin can also blunt TLR agonist-induced cytokine production by dendritic cells (52, 53). In contrast, PKCa can enhance costimulatory molecule expression (54), though again, these exposures may not mimic in vivo drug pharmacokinetics. We (Clutton and colleagues) observed that Bryostatin-1 also induced a modest but sustained reduction in expression of PD-1, an “immune checkpoint” molecule that attenuates signaling downstream of the TCR, on CD8+ T-cells in vitro (38). While HDACis generally induce limited cytokine production in vitro when administered at physiological doses, Bryostatin-1 and Ingenol can trigger production of IL-12, which could boost effector CD8+ T-cell responses (38, 55).
Collectively, these observations suggest that some LRAs may induce viral protein production and antigen presentation; however, whether this occurs at a sufficient level to activate HIV-specific CD8+ T-cells has yet to be conclusively established. Adding further complexity, we (Jones and colleagues) have recently reported that while cells harboring defective proviruses can be eliminated, latent cells infected with replication-competent virus appear to be resistant to killing even in the face of powerful LRAs (including mitogens) and potent HIV-specific CD8+ T-cells (34). Potential mechanisms underlying this resistance include HIV Nef-mediated downmodulation of antigen presentation and intrinsic resistance of productively infected cells to killing. In support of the latter hypothesis, Cohn et al. observed that latent cells harboring intact provirus display a distinct, pro-survival transcriptional signature upon reactivation, and Kuo et al. reported that the anti-apoptotic protein BIRC5 can promote survival of HIV-infected CD4+ T-cells (56, 57). More studies are needed to further elucidate the effects of LRAs on antigen processing and presentation and expression of survival-related genes in productively infected latent cells.
Following successful induction of viral transcription, translation, and antigen presentation, the next critical stage of “shock-and-kill” is the recruitment of HIV-specific CD8+ T-cells capable of recognizing viral antigen and responding appropriately by proliferating, secreting antiviral cytokines, and inducing apoptosis of the infected cell. Recent studies have suggested that LRAs may have effects on numerous aspects of CD8+ T-cell function.
Toxicity is an important consideration when screening LRAs; HDACis such as Vorinostat are used in cancer therapy partly because they can increase expression of pro-apoptotic genes in cancer cells (though not in non-transformed cells) (58). HDACis have been administered to HIV-infected study participants without serious adverse events (8–10, 35, 59, 60), whereas administration of the PKCa Bryostatin has been associated with toxicities including severe myalgia and nausea in patients with persistent or advanced cancers (61, 62). Agents that do not cause clinical toxicity may still have effects on T-cell viability that could compromise the ability to detect and eliminate reactivated HIV-infected cells.
The effects of LRAs on T-cell viability in vitro depend on the dose, culture duration, cellular subset, and activation status of the cells. For example, we and others have reported that HDACis are disproportionately toxic to activated T-cells and CD8+ T-cell clones, whereas resting CD4+ T-cells are less susceptible to LRA toxicity (63, 64). Vorinostat is not toxic at doses up to 1 µM (exceeding the maximum plasma concentration reported in clinical studies), whereas we observed that Romidepsin and Panobinostat reduce T-cell and PBMC viability in a dose-dependent manner (37, 38). The effects of PKCa on viability vary between compounds; Ingenols do not appear to be toxic (38, 65), whereas we have observed that Bryostatin-1 and Prostratin induce PBMC and CD8+ T-cell death at higher doses (10 nM or higher for Bryostatin-1 and over 300 nM for Prostratin) (38, 64, 66). Since it is difficult to determine in vitro doses that reflect clinically relevant exposures for these compounds, more research is needed to determine whether PKCa would induce significant T-cell death in vivo.
Following latency reversal in vivo, antigen-specific proliferation of HIV-specific CD8+ T-cells will likely be required to generate sufficient effectors to detect and eliminate the extremely low frequency latently infected cells dispersed in multiple tissues in the body. However, certain LRAs may inhibit T-cell proliferation; indeed HDACis are used to treat various cancers in part because of their anti-proliferative effects (67, 68). We observed that clinically relevant exposures to Romidepsin and Panobinostat can substantially reduce HIV-specific CD8+ T-cell proliferation in vitro (38, 63). Vorinostat inhibits proliferation at supraphysiological but not clinically achievable doses (38, 63). The effects of PKCa also differ by drug. Prostratin and Bryostatin-1 induce non-specific CD8+ T-cell proliferation. Since altered PKC signaling has been implicated in leukemic cell growth and differentiation, this observation raises concerns about possible protumorigenic effects of PKCa (69). We (Clutton and colleagues) reported that Prostratin and Bryostatin-1 also limit antigen-specific proliferation, perhaps as a result of toxicity, but more likely by limiting the number of proliferative cycles undergone by antigen-specific CD8+ T-cells. Ingenol-db does not induce non-specific proliferation but also reduces the number of proliferative cycles that undergone by antigen-stimulated CD8+ T-cells (38). Thus, many of the compounds under consideration as LRAs may impair antigen-specific CD8+ T-cell proliferation. The critical questions are whether this occurs in vivo, whether the effect would be sufficient to impair clearance, and whether immunization could be used to override these effects by boosting the population of HIV-specific CD8+ T-cells prior to LRA treatment.
Upon encountering viral antigen, HIV-specific CD8+ T-cells secrete cytokines and chemokines to inhibit viral replication, and release granules containing cytotoxic mediators that can induce apoptosis in the infected cell (70, 71). This process is tightly controlled to prevent non-specific effects that could trigger immune pathology [reviewed in Ref. (72)]. We and others have observed that HDACis induce minimal non-specific cytokine production (38, 64). In contrast, the PKCa Prostratin, Ingenol-db, and Bryostatin induce non-specific release of inflammatory cytokines by CD8+ T-cells ex vivo, raising the possibility that these agents could trigger immune pathology or increase the susceptibility of bystander CD4+ T-cells to infection by HIV virions released from reactivated cells (38). However, PKCa also reduce expression of the HIV entry co-receptors CD4+ and CCR5, which may render cells less susceptible to infection (38, 65). If tightly monitored and controlled, a modest increase in inflammation might be beneficial in reactivating cells from latency; nevertheless, clinical testing of these agents should be performed with great caution.
The effects of LRAs on antigen-specific CD8+ T-cell function vary even between drugs of the same class. We and others have reported that Vorinostat has no measurable effect on antigen-specific IFN-γ production and degranulation after a pharmacologically relevant exposure in vitro, and does not impact ex vivo HIV-specific IFN-γ production after multiple in vivo doses (35, 38). However, Panobinostat and Romidepsin reduce antigen-specific cytokine production and degranulation in vitro, though the effects of Romidepsin appear to be delayed until 10 or more hours after dosing (38, 63). Clinical studies have yet to conclusively support or refute an impact of Romidepsin on HIV-specific CD8+ T-cell responses in vivo. In a pilot study of five HIV-infected participants on ART, Romidepsin treatment was associated with a modest reduction in cytokine production by HIV-specific CD8+ T-cells that did not reach statistical significance (10). More recently, Mothe et al. reported a transient decline of 35% in the magnitude of HIV-specific CD8+ T-cell responses following Romidepsin in a therapeutic vaccine plus Romidepsin combination study, though this was a preliminary analysis (26). Collectively, these data suggest that Romidepsin could limit CD8+ T-cell function but that the duration of this impairment may be limited. It will be critical to determine whether viral reactivation and antigen presentation occurs within this timeframe. We (Clutton and colleagues) recently reported that exposure to the PKCa Prostratin and Bryostatin-1 in vitro does not affect antigen-specific CD8+ T-cell cytokine production and degranulation, whereas Ingenol-db modestly boosts antigen-specific responses (38). Though this observation will need to be confirmed in further studies, if potential off-target effects can be mitigated, Ingenol derivatives may be attractive candidates capable of both reversing latency and boosting HIV-specific CD8+ T-cell responses against reactivated cells.
In a study using a primary cell latency model, Shan et al. demonstrated that following reactivation, latently infected CD4+ T-cells do not die by cytopathic effects, but can be killed by HIV-specific CD8+ T-cells (14). In addition to providing a rationale for using T-cell-boosting therapies (such as a vaccine) as part of HIV cure, this study showed that it will be critical to determine whether LRAs have an effect on the killing capacity of CD8+ T-cells. In studies that have addressed this question, it is not always clear whether the effect observed was due to LRAs acting on the infected cell (e.g., affecting antigen processing or presentation) or on HIV-specific CD8+ T-cells directly; however, some have examined effects on CD8+ T-cell killing in isolation. We and others have observed that all three HDACis that have been tested in clinical trials (Vorinostat, Romidepsin, and Panobinostat) can impair killing of infected cells by CD8+ T-cells in vitro (37, 63, 66). However, the effect of Vorinostat appeared to be restricted to recently activated T-cells, such as CTL clones, as in vitro exposure of primary CD8+ T-cells to the drug did not reduce their viral suppressive capacity, and repeated in vivo Vorinostat dosing did not impair the viral suppressive capacity of CD8+ T-cells ex vivo (43, 73). Similarly, we (Jones and colleagues) reported that the PKCa Prostratin completely abrogated the viral suppressive capacity of HIV-specific CD8+ T-cell clones, but Blankson and colleagues demonstrated that Prostratin did not reduce suppression by CD8+ T-cells from elite controllers (37, 66, 74). In contrast to Prostratin, Bryostatin-1 impaired the viral suppressive capacity of primary CD8+ T-cells from elite suppressors in a dose-dependent manner, with the addition of Romidepsin further reducing killing (66, 74). Since latently infected cells pre-exposed to Bryostatin-1 plus Romidepsin can be killed by (LRA-unexposed) CD8+ T-cells in the presence of a bispecific fusion molecule [(40); see above], these data suggest that this combination effectively reverses latency but also induces T-cell dysfunction, acting as a “double-edged sword.” In contrast to the other PKCa, the Ingenol derivative Ingenol-B did not reduce the viral suppressive capacity of CD8+ T-cells from elite suppressors (74). Collectively, these observations suggest that T-cell clones, or recently activated CD8+ T-cells, may be more vulnerable to the negative effects of LRAs (in terms of toxicity, proliferative capacity, and viral suppressive capacity) than primary T-cells. The effects of LRAs on CD8+ killing may also depend on the culture conditions in vitro, with lower doses and shorter exposures having fewer deleterious effects (37, 66). Once again, it will be vital to determine in vitro conditions that reflect clinically relevant exposures.
A direct way of assessing whether combinations of LRAs and T-cells are capable of driving the elimination of cells harboring infectious HIV proviruses from the CD4+ T-cells of HIV-infected individuals on long-term ART is to perform ex vivo co-culture experiments, followed by quantitative viral outgrowth assays. We are aware of only three such studies that have measured replication-competent virus (several others having measured viral RNA, which can also be produced by defective proviruses). Two of these studies reported significant reductions in replication-competent virus in a “latency clearance assay” (43, 73), while we (Jones and colleagues) reported an inability to reduce replication-competent virus in a related “HIV eradication assay” (34). There are a number of differences in terms of methodology, LRAs, immune effectors, and study participant populations that could have contributed to these contrasting observations; and work is underway to understand these. Based on a process of elimination of known barriers to infected-cell elimination, our study suggested that cells harboring infectious proviruses may possess some degree of intrinsic-resistance to elimination by T-cells (discussed above). This draws a parallel with the cancer field, where differential susceptibilities of target cells to killing by T-cells is well known as a limiting factor in immunotherapy (75). While further study is needed to evaluate this possibility in the HIV setting, we raise the possibility that in addition to: (i) antigen presentation on infected cells, (ii) functional (cytotoxic) HIV-specific T-cells, and (iii) the intrinsic susceptibility of target cells to cytotoxic effectors (ex. perforin/granzyme or Fas/FasL) may be an important consideration for infected cells to be efficiently eliminated by “shock-and-kill” strategies.
As described above, most LRAs that have been tested to date in viral suppression assays have had detrimental effects. However, we (Jones and colleagues) have observed that the IL-15 superagonist ALT-803 and the TLR-2 ligand Pam3CSK4 enhance killing of HIV-infected cells by both CD8+ T-cell clones and (in the case of ALT-803) primary CD8+ T-cells from an ART-treated HIV+ participant (37). These agents are exciting candidates that will be examined further in future studies.
A growing body of work demonstrates that LRAs of the HDACi and PKCa classes can negatively impact CD8+ T-cell function in vitro, with more recent clinical studies suggesting that some detrimental effects may also occur in vivo (26). Even within the same class, LRAs can vary in their effects; for example, the HDACis Romidepsin and Panobinostat impair antigen-specific CD8+ T-cell function to a greater extent than Vorinostat. However, several outstanding questions must be addressed before we can confidently conclude whether these agents will substantially alter CD8+ T-cell function in a clinical setting. Further studies are needed to assess the impact on T-cells of in vivo exposure to LRAs, particularly PKCa, though it is interesting to note a degree of concordance between in vitro and in vivo studies to date (Table 1). More work is needed to determine in vitro conditions that mimic physiological exposures, particularly in tissues other than the blood. This is particularly important for PCKa, which are metabolized so rapidly following infusion that it may be difficult to achieve an effective concentration in vivo without unacceptable toxicity (29, 76, 77). Multiple doses of an LRA (or LRAs) will likely be required to substantially deplete the latent reservoir, but few studies to date have examined the effect of repeated exposures on T-cells. The effects of LRAs on HIV-specific CD8+ T-cells must also be balanced with the different efficacies of the various drugs in reversing latency. The current data suggest that, while HDACis may be relatively benign in terms of their effects on CD8+ T-cells, they are less effective latency reversers compared with maximal stimulation (64, 78). PKCa are capable of more robust latency reactivation in vitro, but have yet to demonstrate efficacy in a clinical setting (29). Combination therapy with drugs of two or more classes may allow lower doses of the individual agents to be used, preserving their potency as latency reversers while reducing some of their undesirable effects on CD8+ T-cells, though this approach requires further validation (63, 64).
Table 1. The effects of histone deacetylase inhibitor (HDACi) and PKCa, administered in vitro or in vivo, on CD8+ T-cells.
A major hurdle to the successful implementation of a “shock-and-kill” strategy is the difficulty of reactivating all cells harboring replication-competent virus without triggering a potentially life-threatening systemic inflammatory response (81, 82). Possible approaches to overcome this issue include reducing systemic effects by targeted delivery of LRAs or other therapeutic drugs to latently infected target cells, or using immunosuppressive drugs such as rapamycin to blunt inflammatory cytokine release without compromising latency reversal (83, 84). However, a more achievable goal may be a so-called “functional cure,” whereby the pool of replication-competent virus is not entirely eradicated but the individual can cease ART permanently without viral rebound or risk of transmission to others (85). In this case, an LRA (or combination of LRAs) would need only to be of sufficient potency to shrink the latent reservoir to the extent that any residual viral replication could be controlled by, for example, potent HIV-specific CD8+ T-cells induced by vaccination or other means (86). It is in this context that the short- and long-term effects of LRAs on CD8+ T-cell function are particularly pertinent.
One intriguing finding of the studies to date is that the timing and duration of effects on antigen-specific CD8+ T-cells varies even between LRAs of the same class (38, 63). Whether this impairment coincides with the period during which antigen is presented by reactivated cells could be the major determinant of whether HIV-specific CD8+ T-cells are able to eliminate latent cells. Of note, Sung et al. recently reported that CD8+ T-cells from HIV-infected study participants on ART could suppress viral replication in autologous CD4+ T-cells treated with Vorinostat within 24 h, suggesting that both antigen presentation and CD8+ T-cell killing can occur within this timeframe (43). It is hoped that additional studies examining the kinetics of antigen presentation (or “window of vulnerability”) following LRA treatment will continue to shed light on this issue.
The ultimate question with regards to detrimental effects of LRAs on CD8+ T-cells is whether they will be of sufficient magnitude to critically compromise “shock-and-kill” HIV cure efforts. As ongoing research has furthered our knowledge of the latent reservoir, it has become increasingly clear that a successful “shock-and-kill” strategy will likely require a multi-pronged approach including not only latency reversal but also strategies such as immunization and/or “immune checkpoint” blockade to boost HIV-specific CD8+ T-cell responses. It will be important to examine the effects of these combinatorial strategies on CD8+ T-cells. For example, “immune checkpoint” inhibitors block inhibitory signals from proteins such as PD-1, and have been demonstrated to enhance HIV-specific CD8+ T-cell function (45, 87). These inhibitors could negate the detrimental effects of some LRAs on antigen-specific T-cell proliferation and killing but could also potentially exacerbate the non-specific T-cell responses induced by agents such as Bryostatin-1, therefore, increasing the risk of serious adverse events (38). More promisingly, a recent clinical trial combining Romidepsin with a HIV vaccine showed that although the frequency of vaccine-specific CD8+ T-cells transiently declined after Romidepsin infusion, these responses were efficiently boosted by a second immunization. Furthermore, one-third of the participants experienced delayed viral rebound following ART interruption (26). Though these results were preliminary, and further studies are needed to confirm the findings, this trial suggested that, when fully optimized, a protocol combining LRAs and HIV-specific CD8+ T-cells could provide durable viral suppression in vivo. Finally, it may be necessary to harness additional immune effectors such as NK cells and γδ T-cells to achieve optimal clearance of reactivated latent cells (88, 89). To date, there have been fewer studies of the effects of LRAs on these cell populations, though currently available data suggest that HDACis may have detrimental effects on NK cell function (52, 53, 90).
The search for a cure for HIV has been underway since the start of the epidemic, with numerous hurdles and disappointments as well as promising breakthroughs (91). However, the discovery of compounds that can reverse latency has re-energized the field. If latency reversal can be combined with effective, durable HIV-specific CD8+ T cell responses, this elusive goal may finally be attainable.
All authors listed have made a substantial, direct, and intellectual contribution to the work and approved it for publication.
The authors declare that the research was conducted in the absence of any commercial or financial relationships that could be construed as a potential conflict of interest.
We thank Drs. Nilu Goonetilleke and David Margolis for their constructive feedback, which was invaluable in the preparation of this manuscript. Research reported in this publication was supported by the National Institute of Allergy and Infectious Diseases of the National Institutes of Health under award numbers UM1AI126619 (CARE) and UM1AI126617 (BELIEVE), with co-funding support from the National Institute on Drug Abuse, the National Institute of Mental Health, and the National Institute of Neurological Disorders and Stroke. Additional support was received from the Creative and Novel Ideas in HIV Research Program (CNIHR) through a supplement to the University of California at San Francisco (UCSF) Center for AIDS Research funding (P30 AI027763). This funding was made possible by collaborative efforts of the Office of AIDS Research, the National Institute of Allergy and Infectious Diseases, and the International AIDS Society. RBJ also received support from R01 AI31798, R01 AI111860, R21 MH112224, and the District of Columbia Center for AIDS Research, an NIH funded program (AI117970). GTC is a UNC HIV Cure Center investigator. The content is solely the responsibility of the authors and does not necessarily represent the official views of the National Institutes of Health.
1. Perelson AS, Essunger P, Cao Y, Vesanen M, Hurley A, Saksela K, et al. Decay characteristics of HIV-1-infected compartments during combination therapy. Nature (1997) 387:188–91. doi:10.1038/387188a0
2. Wong JK, Hezareh M, Günthard HF, Havlir DV, Ignacio CC, Spina CA, et al. Recovery of replication-competent HIV despite prolonged suppression of plasma viremia. Science (1997) 278:1291–5. doi:10.1126/science.278.5341.1291
3. Chun TW, Finzi D, Margolick J, Chadwick K, Schwartz D, Siliciano RF. In vivo fate of HIV-1-infected T cells: quantitative analysis of the transition to stable latency. Nat Med (1995) 1:1284–90. doi:10.1038/nm1295-1284
4. Finzi D, Hermankova M, Pierson T, Carruth LM, Buck C, Chaisson RE, et al. Identification of a reservoir for HIV-1 in patients on highly active antiretroviral therapy. Science (1997) 278:1295–300. doi:10.1126/science.278.5341.1295
5. Ruiz L, Martinez-Picado J, Romeu J, Paredes R, Zayat MK, Marfil S, et al. Structured treatment interruption in chronically HIV-1 infected patients after long-term viral suppression. AIDS (2000) 14:397–403. doi:10.1097/00002030-200003100-00013
6. Oxenius A, Price DA, Günthard HF, Dawson SJ, Fagard C, Perrin L, et al. Stimulation of HIV-specific cellular immunity by structured treatment interruption fails to enhance viral control in chronic HIV infection. Proc Natl Acad Sci U S A (2002) 99:13747–52. doi:10.1073/pnas.202372199
7. Li JZ, Etemad B, Ahmed H, Aga E, Bosch RJ, Mellors JW, et al. The size of the expressed HIV reservoir predicts timing of viral rebound after treatment interruption. AIDS (2016) 30:343–53. doi:10.1097/QAD.0000000000000953
8. Archin NM, Liberty AL, Kashuba AD, Choudhary SK, Kuruc JD, Crooks AM, et al. Administration of vorinostat disrupts HIV-1 latency in patients on antiretroviral therapy. Nature (2012) 487:482–5. doi:10.1038/nature11286
9. Rasmussen TA, Tolstrup M, Brinkmann CR, Olesen R, Erikstrup C, Solomon A, et al. Panobinostat, a histone deacetylase inhibitor, for latent-virus reactivation in HIV-infected patients on suppressive antiretroviral therapy: a phase 1/2, single group, clinical trial. Lancet HIV (2014) 1:e13–21. doi:10.1016/S2352-3018(14)70014-1
10. Søgaard OS, Graversen ME, Leth S, Olesen R, Brinkmann CR, Nissen SK, et al. The depsipeptide romidepsin reverses HIV-1 latency in vivo. PLoS Pathog (2015) 11:e1005142. doi:10.1371/journal.ppat.1005142
11. Hamer DH. Can HIV be cured? Mechanisms of HIV persistence and strategies to combat it. Curr HIV Res (2004) 2:99–111. doi:10.2174/1570162043484915
12. Archin NM, Margolis DM. Emerging strategies to deplete the HIV reservoir. Curr Opin Infect Dis (2014) 27:29–35. doi:10.1097/QCO.0000000000000026
13. Margolis DM, Garcia JV, Hazuda DJ, Haynes BF. Latency reversal and viral clearance to cure HIV-1. Science (2016) 353:aaf6517. doi:10.1126/science.aaf6517
14. Shan L, Deng K, Shroff NS, Durand CM, Rabi SA, Yang HC, et al. Stimulation of HIV-1-specific cytolytic T lymphocytes facilitates elimination of latent viral reservoir after virus reactivation. Immunity (2012) 36:491–501. doi:10.1016/j.immuni.2012.01.014
15. Wherry EJ, Ahmed R. Memory CD8 T-cell differentiation during viral infection. J Virol (2004) 78:5535–45. doi:10.1128/JVI.78.11.5535-5545.2004
16. Purbhoo MA, Irvine DJ, Huppa JB, Davis MM. T cell killing does not require the formation of a stable mature immunological synapse. Nat Immunol (2004) 5:524–30. doi:10.1038/ni0604-658a
17. Borrow P, Lewicki H, Hahn BH, Shaw GM, Oldstone MB. Virus-specific CD8+ cytotoxic T-lymphocyte activity associated with control of viremia in primary human immunodeficiency virus type 1 infection. J Virol (1994) 68:6103–10.
18. Borrow P, Lewicki H, Wei X, Horwitz MS, Peffer N, Meyers H, et al. Antiviral pressure exerted by HIV-1-specific cytotoxic T lymphocytes (CTLs) during primary infection demonstrated by rapid selection of CTL escape virus. Nat Med (1997) 3:205–11. doi:10.1038/nm0297-205
19. Koup RA, Safrit JT, Cao Y, Andrews CA, McLeod G, Borkowsky W, et al. Temporal association of cellular immune responses with the initial control of viremia in primary human immunodeficiency virus type 1 syndrome. J Virol (1994) 68:4650–5.
20. Pereyra F, Heckerman D, Carlson JM, Kadie C, Soghoian DZ, Karel D, et al. HIV control is mediated in part by CD8+ T-cell targeting of specific epitopes. J Virol (2014) 88:12937–48. doi:10.1128/JVI.01004-14
21. Mothe B, Llano A, Ibarrondo J, Zamarreño J, Schiaulini M, Miranda C, et al. CTL responses of high functional avidity and broad variant cross-reactivity are associated with HIV control. PLoS One (2012) 7:e29717. doi:10.1371/journal.pone.0029717
22. Miura T, Brockman MA, Schneidewind A, Lobritz M, Pereyra F, Rathod A, et al. HLA-B57/B*5801 human immunodeficiency virus type 1 elite controllers select for rare gag variants associated with reduced viral replication capacity and strong cytotoxic T-lymphocyte [corrected] recognition. J Virol (2009) 83:2743–55. doi:10.1128/JVI.02265-08
23. Schneidewind A, Brockman MA, Yang R, Adam RI, Li B, Le Gall S, et al. Escape from the dominant HLA-B27-restricted cytotoxic T-lymphocyte response in Gag is associated with a dramatic reduction in human immunodeficiency virus type 1 replication. J Virol (2007) 81:12382–93. doi:10.1128/JVI.01543-07
24. Goulder PJ, Bunce M, Krausa P, McIntyre K, Crowley S, Morgan B, et al. Novel, cross-restricted, conserved, and immunodominant cytotoxic T lymphocyte epitopes in slow progressors in HIV type 1 infection. AIDS Res Hum Retroviruses (1996) 12:1691–8. doi:10.1089/aid.1996.12.1691
25. Migueles SA, Sabbaghian MS, Shupert WL, Bettinotti MP, Marincola FM, Martino L, et al. HLA B*5701 is highly associated with restriction of virus replication in a subgroup of HIV-infected long term nonprogressors. Proc Natl Acad Sci U S A (2000) 97:2709–14. doi:10.1073/pnas.050567397
26. Mothe B, Molto J, Manzardo C, et al. Viral control induced by HIVconsv vaccines & romidepsin in early treated individuals. Conference on Retroviruses and Opportunistic Infections. Boston, MA, USA (2017).
27. Marks PA, Richon VM, Miller T, Kelly WK. Histone deacetylase inhibitors. Adv Cancer Res (2004) 91:137–68. doi:10.1016/S0065-230X(04)91004-4
28. Jiang G, Dandekar S. Targeting NF-kappaB signaling with protein kinase C agonists as an emerging strategy for combating HIV latency. AIDS Res Hum Retroviruses (2015) 31:4–12. doi:10.1089/aid.2014.0199
29. Gutierrez C, Serrano-Villar S, Madrid-Elena N, et al. Bryostatin-1 for latent virus reactivation in HIV-infected patients on antiretroviral therapy: a phase I, double-blind clinical trial. AIDS (2016) 30:1385–92. doi:10.1097/QAD.0000000000001064
30. Zinkernagel RM, Doherty PC. Restriction of in vitro T cell-mediated cytotoxicity in lymphocytic choriomeningitis within a syngeneic or semiallogeneic system. Nature (1974) 248:701–2. doi:10.1038/248701a0
31. Krogsgaard M, Davis MM. How T cells ‘see’ antigen. Nat Immunol (2005) 6:239–45. doi:10.1038/ni1173
32. Pollack RA, Jones RB, Pertea M, Bruner KM, Martin AR, Thomas AS, et al. Defective HIV-1 proviruses are expressed and can be recognized by cytotoxic T lymphocytes, which shape the proviral landscape. Cell Host Microbe (2017) 21:494–506.e4. doi:10.1016/j.chom.2017.03.008
33. Pace MJ, Graf EH, Agosto LM, Mexas AM, Male F, Brady T, et al. Directly infected resting CD4+T cells can produce HIV Gag without spreading infection in a model of HIV latency. PLoS Pathog (2012) 8:e1002818. doi:10.1371/journal.ppat.1002818
34. Huang SH, Ren Y, Thomas AS, Chan D, Mueller S, Ward AR, et al. Latent HIV reservoirs exhibit inherent resistance to elimination by CD8+ T cells. J Clin Invest (2018) 128:876–89. doi:10.1172/JCI97555
35. Elliott JH, Wightman F, Solomon A, Ghneim K, Ahlers J, Cameron MJ, et al. Activation of HIV transcription with short-course vorinostat in HIV-infected patients on suppressive antiretroviral therapy. PLoS Pathog (2014) 10:e1004473. doi:10.1371/journal.ppat.1004473
36. Wu G, Swanson M, Talla A, Graham D, Strizki J, Gorman D, et al. HDAC inhibition induces HIV-1 protein and enables immune-based clearance following latency reversal. JCI Insight (2017) 2(16). doi:10.1172/jci.insight.92901
37. Jones RB, Mueller S, O’Connor R, Rimpel K, Sloan DD, Karel D, et al. A subset of latency-reversing agents expose HIV-infected resting CD4+ T-cells to recognition by cytotoxic T-lymphocytes. PLoS Pathog (2016) 12:e1005545. doi:10.1371/journal.ppat.1005545
38. Clutton G, Xu Y, Baldoni PL, Mollan KR, Kirchherr J, Newhard W, et al. The differential short- and long-term effects of HIV-1 latency-reversing agents on T cell function. Sci Rep (2016) 6:30749. doi:10.1038/srep34430
39. Bossi G, Buisson S, Oates J, Jakobsen BK, Hassan NJ. ImmTAC-redirected tumour cell killing induces and potentiates antigen cross-presentation by dendritic cells. Cancer Immunol Immunother (2014) 63:437–48. doi:10.1007/s00262-014-1525-z
40. Yang H, Buisson S, Bossi G, Wallace Z, Hancock G, So C, et al. Elimination of latently HIV-infected cells from antiretroviral therapy-suppressed subjects by engineered immune-mobilizing T-cell receptors. Mol Ther (2016) 24:1913–25. doi:10.1038/mt.2016.114
41. Huang J, Brameshuber M, Zeng X, Xie J, Li QJ, Chien YH, et al. A single peptide-major histocompatibility complex ligand triggers digital cytokine secretion in CD4(+) T cells. Immunity (2013) 39:846–57. doi:10.1016/j.immuni.2013.08.036
42. Irvine DJ, Purbhoo MA, Krogsgaard M, Davis MM. Direct observation of ligand recognition by T cells. Nature (2002) 419:845–9. doi:10.1038/nature01076
43. Sung JA, Sholtis K, Kirchherr J, Kuruc JD, Gay CL, Nordstrom JL, et al. Vorinostat renders the replication-competent latent reservoir of human immunodeficiency virus (HIV) vulnerable to clearance by CD8 T cells. EBioMedicine (2017) 23:52–8. doi:10.1016/j.ebiom.2017.07.019
44. Thomas AS, Jones KL, Gandhi RT, McMahon DK, Cyktor JC, Chan D, et al. T-cell responses targeting HIV Nef uniquely correlate with infected cell frequencies after long-term antiretroviral therapy. PLoS Pathog (2017) 13:e1006629. doi:10.1371/journal.ppat.1006629
45. Day CL, Kaufmann DE, Kiepiela P, Brown JA, Moodley ES, Reddy S, et al. PD-1 expression on HIV-specific T cells is associated with T-cell exhaustion and disease progression. Nature (2006) 443:350–4. doi:10.1038/nature05115
46. Trautmann L, Janbazian L, Chomont N, Said EA, Gimmig S, Bessette B, et al. Upregulation of PD-1 expression on HIV-specific CD8+ T cells leads to reversible immune dysfunction. Nat Med (2006) 12:1198–202. doi:10.1038/nm1106-1329b
47. Jones RB, Ndhlovu LC, Barbour JD, Sheth PM, Jha AR, Long BR, et al. Tim-3 expression defines a novel population of dysfunctional T cells with highly elevated frequencies in progressive HIV-1 infection. J Exp Med (2008) 205(12):2763–79. doi:10.1084/jem.20081398
48. Day CL, Kiepiela P, Leslie AJ, van der Stok M, Nair K, Ismail N, et al. Proliferative capacity of epitope-specific CD8 T-cell responses is inversely related to viral load in chronic human immunodeficiency virus type 1 infection. J Virol (2007) 81:434–8. doi:10.1128/JVI.01754-06
49. Chen L, Flies DB. Molecular mechanisms of T cell co-stimulation and co-inhibition. Nat Rev Immunol (2013) 13:227–42. doi:10.1038/nri3405
50. Sharpe AH. Mechanisms of costimulation. Immunol Rev (2009) 229:5–11. doi:10.1111/j.1600-065X.2009.00784.x
51. Curtsinger JM, Mescher MF. Inflammatory cytokines as a third signal for T cell activation. Curr Opin Immunol (2010) 22:333–40. doi:10.1016/j.coi.2010.02.013
52. Stephen S, Morrissey KA, Benoit BM, Kim EJ, Vittorio CC, Nasta SD, et al. Inhibition of cell-mediated immunity by the histone deacetylase inhibitor vorinostat: implications for therapy of cutaneous T-cell lymphoma. Am J Hematol (2012) 87:226–8. doi:10.1002/ajh.22231
53. Kelly-Sell MJ, Kim YH, Straus S, Benoit B, Harrison C, Sutherland K, et al. The histone deacetylase inhibitor, romidepsin, suppresses cellular immune functions of cutaneous T-cell lymphoma patients. Am J Hematol (2012) 87:354–60. doi:10.1002/ajh.23112
54. Zhao D, Amria S, Hossain A, Sundaram K, Komlosi P, Nagarkatti M, et al. Enhancement of HLA class II-restricted CD4+ T cell recognition of human melanoma cells following treatment with bryostatin-1. Cell Immunol (2011) 271:392–400. doi:10.1016/j.cellimm.2011.08.007
55. Li H, Wojciechowski W, Dell’Agnola C, Lopez NE, Espinoza-Delgado I. IFN-gamma and T-bet expression in human dendritic cells from normal donors and cancer patients is controlled through mechanisms involving ERK-1/2-dependent and IL-12-independent pathways. J Immunol (2006) 177:3554–63. doi:10.4049/jimmunol.177.6.3554
56. Cohn LB, da Silva IT, Valieris R, Huang AS, Lorenzi JCC, Cohen YZ, et al. Clonal CD4(+) T cells in the HIV-1 latent reservoir display a distinct gene profile upon reactivation. Nat Med (2018) 24:604–9. doi:10.1038/s41591-018-0017-7
57. Kuo HH, Ahmad R, Lee GQ, Gao C, Chen HR, Ouyang Z, et al. Anti-apoptotic protein BIRC5 maintains survival of HIV-1-infected CD4(+) T cells. Immunity (2018). doi:10.1016/j.immuni.2018.04.004
58. Insinga A, Monestiroli S, Ronzoni S, Gelmetti V, Marchesi F, Viale A, et al. Inhibitors of histone deacetylases induce tumor-selective apoptosis through activation of the death receptor pathway. Nat Med (2005) 11:71–6. doi:10.1038/nm1160
59. Mota TM, Rasmussen TA, Rhodes A, et al. No adverse safety or virological changes two years following vorinostat in HIV-infected individuals on antiretroviral therapy. AIDS (2017) 31:1137–41. doi:10.1097/QAD.0000000000001442
60. Archin NM, Bateson R, Tripathy MK, Crooks AM, Yang KH, Dahl NP, et al. HIV-1 expression within resting CD4+ T cells after multiple doses of vorinostat. J Infect Dis (2014) 210:728–35. doi:10.1093/infdis/jiu155
61. Plimack ER, Tan T, Wong YN, von Mehren MM, Malizzia L, Roethke SK, et al. A phase I study of temsirolimus and bryostatin-1 in patients with metastatic renal cell carcinoma and soft tissue sarcoma. Oncologist (2014) 19:354–5. doi:10.1634/theoncologist.2014-0020
62. Morgan RJ Jr, Leong L, Chow W, Gandara D, Frankel P, Garcia A, et al. Phase II trial of bryostatin-1 in combination with cisplatin in patients with recurrent or persistent epithelial ovarian cancer: a California cancer consortium study. Invest New Drugs (2012) 30:723–8. doi:10.1007/s10637-010-9557-5
63. Jones RB, O’Connor R, Mueller S, Foley M, Szeto GL, Karel D, et al. Histone deacetylase inhibitors impair the elimination of HIV-infected cells by cytotoxic T-lymphocytes. PLoS Pathog (2014) 10:e1004287. doi:10.1371/journal.ppat.1004287
64. Laird GM, Bullen CK, Rosenbloom DI, Martin AR, Hill AL, Durand CM, et al. Ex vivo analysis identifies effective HIV-1 latency-reversing drug combinations. J Clin Invest (2015) 125(5):1901–12. doi:10.1172/JCI80142
65. Jiang G, Mendes EA, Kaiser P, Wong DP, Tang Y, Cai I, et al. Synergistic reactivation of latent HIV expression by Ingenol-3-Angelate, PEP005, targeted NF-kB signaling in combination with JQ1 induced p-TEFb activation. PLoS Pathog (2015) 11:e1005066. doi:10.1371/journal.ppat.1005066
66. Walker-Sperling VE, Pohlmeyer CW, Tarwater PM, Blankson JN. The effect of latency reversal agents on primary CD8+ T cells: implications for shock and kill strategies for human immunodeficiency virus eradication. EBioMedicine (2016) 8:217–29. doi:10.1016/j.ebiom.2016.04.019
67. Richon VM. Cancer biology: mechanism of antitumour action of vorinostat (suberoylanilide hydroxamic acid), a novel histone deacetylase inhibitor. Br J Cancer (2006) 95:S2–6. doi:10.1038/sj.bjc.6603463
68. Moskowitz AJ, Horwitz SM. Targeting histone deacetylases in T-cell lymphoma. Leuk Lymphoma (2017) 58:1306–19. doi:10.1080/10428194.2016.1247956
69. Redig AJ, Platanias LC. Protein kinase C signalling in leukemia. Leuk Lymphoma (2008) 49:1255–62. doi:10.1080/10428190802007726
70. Nixon DF, Townsend AR, Elvin JG, Rizza CR, Gallwey J, McMichael AJ. HIV-1 gag-specific cytotoxic T lymphocytes defined with recombinant vaccinia virus and synthetic peptides. Nature (1988) 336:484–7. doi:10.1038/336484a0
71. Walker BD, Chakrabarti S, Moss B, Paradis TJ, Flynn T, Durno AG, et al. HIV-specific cytotoxic T lymphocytes in seropositive individuals. Nature (1987) 328:345–8. doi:10.1038/328345a0
72. Huppa JB, Davis MM. T-cell-antigen recognition and the immunological synapse. Nat Rev Immunol (2003) 3:973–83. doi:10.1038/nri1245
73. Sung JA, Lam S, Garrido C, Archin N, Rooney CM, Bollard CM, et al. Expanded cytotoxic T-cell lymphocytes target the latent HIV reservoir. J Infect Dis (2015) 212(2):258–63. doi:10.1093/infdis/jiv022
74. Kwaa AK, Goldsborough K, Walker-Sperling VE, Pianowski LF, Gama L, Blankson JN. The effect of Ingenol-B on the suppressive capacity of elite suppressor HIV-specific CD8+ T cells. PLoS One (2017) 12:e0174516. doi:10.1371/journal.pone.0174516
75. Patel SJ, Sanjana NE, Kishton RJ, Eidizadeh A, Vodnala SK, Cam M, et al. Identification of essential genes for cancer immunotherapy. Nature (2017) 548:537–42. doi:10.1038/nature23477
76. Madhusudan S, Protheroe A, Propper D, Han C, Corrie P, Earl H, et al. A multicentre phase II trial of bryostatin-1 in patients with advanced renal cancer. Br J Cancer (2003) 89:1418–22. doi:10.1038/sj.bjc.6601321
77. Pavlick AC, Wu J, Roberts J, Rosenthal MA, Hamilton A, Wadler S, et al. Phase I study of bryostatin 1, a protein kinase C modulator, preceding cisplatin in patients with refractory non-hematologic tumors. Cancer Chemother Pharmacol (2009) 64:803–10. doi:10.1007/s00280-009-0931-y
78. Spina CA, Anderson J, Archin NM, Bosque A, Chan J, Famiglietti M, et al. An in-depth comparison of latent HIV-1 reactivation in multiple cell model systems and resting CD4+ T cells from aviremic patients. PLoS Pathog (2013) 9:e1003834. doi:10.1371/journal.ppat.1003834
79. Brinkmann CR, Højen JF, Rasmussen TA, Kjær AS, Olesen R, Denton PW, et al. Treatment of HIV-infected individuals with the histone deacetylase inhibitor panobinostat results in increased numbers of regulatory T cells and limits ex vivo lipopolysaccharide-induced inflammatory responses. mSphere (2018) 3:e00616-17. doi:10.1128/mSphere.00616-17
80. Ellis L, Pan Y, Smyth GK, George DJ, McCormack C, Williams-Truax R, et al. Histone deacetylase inhibitor panobinostat induces clinical responses with associated alterations in gene expression profiles in cutaneous T-cell lymphoma. Clin Cancer Res (2008) 14:4500–10. doi:10.1158/1078-0432.CCR-07-4262
81. van Praag RM, Prins JM, Roos MT, Schellekens PT, Ten Berge IJ, Yong SL, et al. OKT3 and IL-2 treatment for purging of the latent HIV-1 reservoir in vivo results in selective long-lasting CD4+ T cell depletion. J Clin Immunol (2001) 21:218–26. doi:10.1023/A:1011091300321
82. Prins JM, Jurriaans S, van Praag RM, Blaak H, van Rij R, Schellekens PT, et al. Immuno-activation with anti-CD3 and recombinant human IL-2 in HIV-1-infected patients on potent antiretroviral therapy. AIDS (1999) 13:2405–10. doi:10.1097/00002030-199912030-00012
83. Martin AR, Pollack RA, Capoferri A, Ambinder RF, Durand CM, Siliciano RF. Rapamycin-mediated mTOR inhibition uncouples HIV-1 latency reversal from cytokine-associated toxicity. J Clin Invest (2017) 127(2):651–6. doi:10.1172/JCI89552
84. Jones RB, Mueller S, Kumari S, Vrbanac V, Genel S, Tager AM, et al. Antigen recognition-triggered drug delivery mediated by nanocapsule-functionalized cytotoxic T-cells. Biomaterials (2017) 117:44–53. doi:10.1016/j.biomaterials.2016.11.048
85. International AIDS Society Scientific Working Group on HIV Cure, Deeks SG, Autran B, Berkhout B, Benkirane M, Cairns S, et al. Towards an HIV cure: a global scientific strategy. Nat Rev Immunol (2012) 12:607–14. doi:10.1038/nri3262
86. Conway JM, Perelson AS. Post-treatment control of HIV infection. Proc Natl Acad Sci U S A (2015) 112:5467–72. doi:10.1073/pnas.1419162112
87. Zhang JY, Zhang Z, Wang X, Fu JL, Yao J, Jiao Y, et al. PD-1 up-regulation is correlated with HIV-specific memory CD8+ T-cell exhaustion in typical progressors but not in long-term nonprogressors. Blood (2007) 109:4671–8. doi:10.1182/blood-2006-09-044826
88. Garrido C, Abad-Fernandez M, Tuyishime M, Pollara JJ, Ferrari G, Soriano-Sarabia N, et al. Il-15 stimulated natural killer cells clear HIV-1 infected cells following latency reversal ex vivo. J Virol (2018). doi:10.1128/JVI.00235-18
89. Soriano-Sarabia N, Archin NM, Bateson R, Dahl NP, Crooks AM, Kuruc JD, et al. Peripheral Vgamma9Vdelta2 T cells are a novel reservoir of latent HIV infection. PLoS Pathog (2015) 11:e1005201. doi:10.1371/journal.ppat.1005201
90. Garrido C, Spivak AM, Soriano-Sarabia N, Checkley MA, Barker E, Karn J, et al. HIV latency-reversing agents have diverse effects on natural killer cell function. Front Immunol (2016) 7:356. doi:10.3389/fimmu.2016.00356
Keywords: T-cells, HIV cure, shock-and-kill, histone deacetylase inhibitor, PKCa
Citation: Clutton GT and Jones RB (2018) Diverse Impacts of HIV Latency-Reversing Agents on CD8+ T-Cell Function: Implications for HIV Cure. Front. Immunol. 9:1452. doi: 10.3389/fimmu.2018.01452
Received: 19 April 2018; Accepted: 12 June 2018;
Published: 22 June 2018
Edited by:
Julia G. Prado, IrsiCaixa, SpainReviewed by:
Vijayakumar Velu, Emory University, United StatesCopyright: © 2018 Clutton and Jones. This is an open-access article distributed under the terms of the Creative Commons Attribution License (CC BY). The use, distribution or reproduction in other forums is permitted, provided the original author(s) and the copyright owner are credited and that the original publication in this journal is cited, in accordance with accepted academic practice. No use, distribution or reproduction is permitted which does not comply with these terms.
*Correspondence: Genevieve Tyndale Clutton, Z2VuX2NsdXR0b25AbWVkLnVuYy5lZHU=
Disclaimer: All claims expressed in this article are solely those of the authors and do not necessarily represent those of their affiliated organizations, or those of the publisher, the editors and the reviewers. Any product that may be evaluated in this article or claim that may be made by its manufacturer is not guaranteed or endorsed by the publisher.
Research integrity at Frontiers
Learn more about the work of our research integrity team to safeguard the quality of each article we publish.