- 1Key Laboratory of Respiratory Diseases of the Ministry of Health, Department of Respiratory and Critical Care Medicine, Union Hospital, Tongji Medical College, Huazhong University of Science and Technology, Wuhan, China
- 2Department of Thoracic Surgery, Union Hospital, Tongji Medical College, Huazhong University of Science and Technology, Wuhan, China
Retinoic acid receptor-related orphan receptors (RORs) include RORα (NR1F1), RORβ (NR1F2), and RORγ (NR1F3). These receptors are reported to activate transcription through ligand-dependent interactions with co-regulators and are involved in the development of secondary lymphoid tissues, autoimmune diseases, inflammatory diseases, the circadian rhythm, and metabolism homeostasis. Researches on RORs contributing to cancer-related processes have been growing, and they provide evidence that RORs are likely to be considered as potential therapeutic targets in many cancers. RORα has been identified as a potential therapeutic target for breast cancer and has been investigated in melanoma, colorectal colon cancer, and gastric cancer. RORβ is mainly expressed in the central nervous system, but it has also been studied in pharyngeal cancer, uterine leiomyosarcoma, and colorectal cancer, in addition to neuroblastoma, and recent studies suggest that RORγ is involved in various cancers, including lymphoma, melanoma, and lung cancer. Some studies found RORγ to be upregulated in cancer tissues compared with normal tissues, while others indicated the opposite results. With respect to the mechanisms of RORs in cancer, previous studies on the regulatory mechanisms of RORs in cancer were mostly focused on immune cells and cytokines, but lately there have been investigations concentrating on RORs themselves. Thus, this review summarizes reports on the regulation of RORs in cancer and highlights potential therapeutic targets in cancer.
Introduction
Cancer incidence and mortality rates are increasing worldwide with the growing and aging of the population, as well as risk factors such as outdoor pollution, tobacco smoke, and physical inactivity (1). Due to early detection and advanced treatments, cancer survival rates continue to grow, although a better understanding of carcinogenesis may lead to more effective treatment options for cancer.
The nuclear receptors (NRs) have been demonstrated to play essential roles in cancer-related progresses and to be potential therapeutic targets for many malignancies (2–5). The retinoic acid receptor-related orphan receptors (RORs) are a subfamily of the thyroid hormone receptor, which is a subfamily of the NRs and belonging to the orphan NR family (6). The ROR subfamily contains three members: RORα (NR1F1), RORβ (NR1F2), and RORγ (NR1F3).
Members of the RORs are typically regarded as noteworthy in inflammation, autoimmune diseases, metabolism disorders, circadian rhythms, development of neuron cells, and immune cell differentiation. Although RORs share some common sequences, the three RORs present a wide assortment of features. RORα and RORγ are important regulators of the immune system. For instance, the development and differentiation of Th17 cells are dependent on these factors (7–9). Moreover, studies show that RORγ is expressed in lymphoid tissue inducer cells, innate lymphoid cells, invariant natural killer T cells, and γδ T cells, which contribute to inflammation and autoimmune disease (10).
RORα, RORβ, and RORγ are all involved in the modulation of circadian rhythms. RORα functions as a positive regulator of the circadian modulator Bmal1 through binding to ROR-responsive elements (ROREs) (11, 12). RORβ mRNA expression levels were found to oscillate with true circadian rhythms, peaking at night-time (13), and modulation of circadian rhythms was disrupted in RORβ-deficient mice (14). Recent studies have proposed that RORγ1, but not RORα, is periodically expressed, and RORγ regulates several clock genes, such as Cry1, Bmal1, and Npas2, directly in a Zeitgeber time-dependent manner through these ROREs (15, 16).
Accumulating evidence shows that RORα and RORγ are involved in lipid/glucose metabolism, insulin sensitivity, and cardiometabolic control (17). A report showed that RORα could repress the transcriptional activity of PPARγ, leading to dysregulation of hepatic lipid metabolism (18). Recently, studies have shown that metabolic disorders affected by circadian rhythms might be attributed to RORα and RORγ, partly because of their modulation in both circadian and metabolic diseases. Moreover, earlier studies suggested that RORα was directly involved in melatonin-mediated anti-fibrotic processes (19) and beneficial manipulation in diabetic cardiomyopathy (20).
The expression sites and producing cells of RORs are also distinct from each other, consistent with their functions in the various diseases mentioned above. RORα and RORγ are expressed in all skin cell types, including epidermal keratinocytes, melanocytes, dermal fibroblasts, and several established lines of malignant melanomas. The expression levels of RORα/γ are dependent on the skin cell type and can be regulated by hydroxy derivatives of vitamin D3 (5, 21–24). Vitamin D3 formation is regulated by UVB (25); vitamin D3 metabolites are inverse agonists for RORα/γ; therefore, RORα and RORγ expression level could be regulated by UVB (5).
Other expression sites of RORα include the liver, skin, pancreas, brain, adipose tissue, islet cells, and the pineal gland. In addition to its expression and modulation in melanoma described above, RORα has been researched in breast cancer (BC) (26), melanoma (5), hepatocellular carcinoma (HCC) (27), and colon cancer (28). RORβ is mainly expressed in the brain and pineal gland (29). RORβ is upregulated or downregulated in cancers such as primary leiomyosarcoma of the uterus (30), a pharyngeal cancer cell line (31), and colorectal cancer (28). RORγ is expressed in the thymus and lymphoid organs, and RORγ production in cancer cells is detected in lung cancer (4), lymphoma (32), melanoma (5), and BC (33).
The RORs have been widely investigated in cancer and have shown varying influences in cancer-related processes, these differences may be due to their structures and their tissue-specific expression. Some studies suggest that RORα is a tumor suppressor and a potential therapeutic target for BC; and based on the limited researches on RORβ in cancer, RORβ might be a tumor suppressor as well. Others have proposed that activating RORγ may exert antitumor immunity (34), while RORγ is considered as protumor candidates in prostate cancer and lung cancer (4, 35). In this review, we summarize and discuss the structures of RORs and their roles in cancer-related processes, highlighting the potential therapeutic targets for cancer treatment.
Structure and Ligands of RORs
The three ROR family members contain sequences similar to the retinoic acid receptor, with certain differences. The three ROR family members contain sequences similar to the retinoic acid receptor, but in minor details, the structures of each are distinct (36). The RORα gene maps to human chromosome 15q22.2, covering a large genomic region of 730 kb and generating four human RORα isoforms: RORα1—RORα4, while only RORα1 and RORα4 are found in mice (17). The RORβ and RORγ genes map to human q21.13 and 1q21.3, covering 188 and 24 kb, respectively. RORβ and RORγ each generate two isoforms: RORβ1/RORβ2 and RORγ1/RORγ2 (RORC2 in human and RORγt in mice). The isoforms of RORs differ in their amino terminals due to alternative exon splicing and promoter usage and their distinct expression and function in different tissues. However, if cells co-express RORs, the co-expressed RORs may overlap in several functions.
Receptor-related orphan receptor genes encode proteins of similar amino sequences ranging from 459 to 556 amino acids according to the different isoforms, and they all consist of four domains. These domains include an N-terminal domain, a highly conserved DNA-binding domain, a ligand-binding domain (LBD), and a hinge between the domains. Transcription is regulated by binding to RORE as a monomer (36).
No cognate ligands of RORs had been identified until crystallography studies on the LBD of RORα indicated that cholesterol and cholesterol sulfate function as natural ligands (37). Several retinoids, including all-trans retinoic acid and the synthetic retinoid ALRT 1550 (ALRT), have been identified to bind RORβ, reversibly and with high affinity (38). Thus, the retinoids have been identified as ligands of RORβ, although their specific regulation is not clearly understood. RORγ has been found to be co-expressed with RORα, and the ligands of RORα and RORγ have been reported as sterols or their derivatives and secosteroids (5, 6). Endogenously produced novel D3 hydroxy derivatives can act as both “biased” agonists of the vitamin D receptor and inverse agonists of RORα/γ (22), and hydroxylumisterols can act as ligands of RORα and RORγ (39). Melatonin was once considered a ligand for RORα (40, 41). However, contrasting reports showed that melatonin was not a natural ligand for RORα because melatonin could not activate RORα directly (42, 43). The docking scores calculated from molecular modeling of interactions between melatonin and its metabolites with RORα and RORγ predicted weak binding affinities (5), and the structures of melatonin and its metabolites were not similar to the sterols that were identified as natural ligands (37).
Except for the natural ligands of RORs mentioned above, there are also some synthetic RORγ ligands with therapeutic potential identified in literatures (6, 44). For instance, the inverse agonists of RORα and RORγ, SR2211 has been reported to inhibit the expression of IL-17A and cell viability in lung cancer (4) and suppress inflammation in a collagen-induced arthritis mouse model (45). And RORα and RORγ agonist SR1078 can induce cancer cell apoptosis and p53 stability (46). Inverse agonists or agonists like these two are promising therapeutic reagents for the diseases that RORs involved in, but there are still lack of studies to investigate their treatment potentials in cancer.
Cancer Relevance
As illustrated above, RORs have been implicated in autoimmune or immune-mediated disease, the circadian rhythm, and metabolic disorders. RORs are also important regulators in various cancers due to their pivotal roles in immunity, the circadian rhythm, and metabolic homeostasis, which contribute to tumor progression.
RORα has been found to be downregulated in keratinocyte-derived skin cancer (47) and is expressed in prostate cancer cells (48), melanoma cell lines (5, 49), and BC (50) (Table 1). Decreased expression of RORα is positively related with melanoma progression and shorter disease-free and overall survival (23, 24). RORα is also involved in inhibiting cell proliferation as a tumor suppressor (51). In human hepatoma cells, RORα was found to be upregulated after hypoxia induction (52), while RORα expression was lower in tumor tissues than in adjacent tumor tissues. It was also determined to be involved in the reprogramming of glucose metabolism and inhibiting hepatoma growth both in vitro and in a xenograft model in vivo (53). However, in one report, the production of RORα mRNA in colorectal cancer patients was unchanged (54), while RORα phosphorylation was found reduced and might be involved in colon cancer progression (55). In another report about BC, RORα was found to be downregulated, and low expression of RORα mRNA was associated with a poor prognosis (26). RORα is commonly considered a repressor (Figure 1), according to investigations into its role in cancer illustrated above.
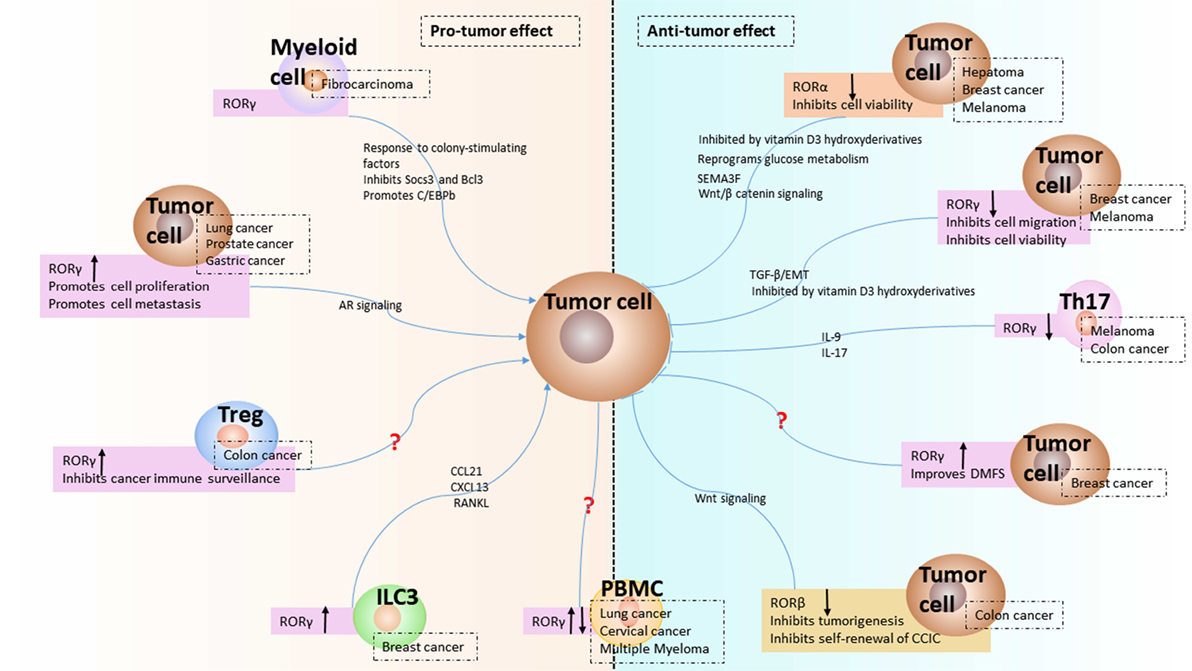
Figure 1. Expression and function of receptor-related orphan receptors (RORs) in tumor microenvironment. The expression of RORα and RORβ from tumor cell and the modulated expression of RORγ in group 3 innate lymphoid cells (ILC3), Th17, regulatory T cell (Treg), myeloid cell, and tumor cell from tumor microenvironment are presented as reviewed in the text. The downregulation of RORα and RORβ induce antitumor effect in hepatoma, breast cancer (BC), melanoma, and colon cancer. The upregulation of RORγ in ILC3 leads to protumor effect by chemokines in BC. The downregulation of RORγ in Th17 indicates antitumor effect by IL-17 in colon cancer. The upregulation of RORγ in Treg shows protumor effect in colon cancer. The expression of RORγ in myeloid cell has protumor effects via Socs3, Bcl3, and C/EBPb. The expression of RORγ in tumor cell is either increased or decreased depending on the cancer type. Increased expression of RORγ in lung cancer, prostate cancer, and gastric cancer results in protumor effect, while decreased expression of RORγ in BC and melanoma could induce antitumor effect via TGFβ/epithelial–mesenchymal transition (EMT) or vitamin D3 derivatives. The question mark refers to unknown mechanisms. The up or down black arrow refers to upregulation or downregulation. Antitumor: inhibits tumor progression; protumor: promotes tumor progression.
The natural expression of RORβ is exclusively restricted to neuronal tissues; therefore, activation of RORβ transcription is predominantly found in neuroblastoma cell lines (56), and literature on the role of RORβ in cancer is not much. Nevertheless, primary uterine leiomyosarcoma showed high RORβ expression (30), pharyngeal carcinoma cells and colorectal cancer cells showed modulated RORβ expression (29, 31), and RORβ was related to metastasis in a metastatic colorectal cancer cell model (28), which are summarized in Table 1. Based on the studies mentioned above, RORβ shows features of a tumor suppressor (Figure 1), but the potential roles of RORβ in various cancers related processes such as tumor proliferation and metastasis warrant further investigation.
RORγ in Various Cancers
On the contrary, RORγ and its isoforms are extensively found in various kinds of malignancies. The diverse roles of RORγ in distinct cancers are specifically described below and summarized in Table 1 and Figure 1.
Hematological Malignancies
RORγ was found to function as an important element in lymphatic tumors (32), and mice deficient in RORγ were shown to have a high incidence of lymphoma metastasis and death within 4 months (57). Moreover, RORγ is frequently studied in tumor-infiltrating immune cells. RORγ mRNA expression in total lymphocytes was found unchanged between multiple myeloma and healthy controls (58, 59), but it was identified upregulation in peripheral blood monocyte cell (PBMC) from multiple myeloma comparing with healthy controls (60).
Breast Cancer
RORγ was found to be significantly overexpressed among infiltrating IL-17+ T cells, which drive immunosuppression in BC (61), and in breast tumor tissues compared with control tissues (62). An investigation related to group 3 innate lymphoid cells (ILC3) in BC revealed a role for RORγt + ILC3 in promoting lymph node metastasis by modulating chemokines in the tumor microenvironment (63). RORγ was found to be decreased in basal-like and grade 3 BCs, and inhibition of RORγ blocked cell viability, migration, and epithelial–mesenchymal transition (EMT) (64). However, an earlier study suggested that high expression of RORγ1, but not RORγt, by cancer cells was related to a high distance metastasis-free survival and was inversely correlated with decreased expression of PRMT2, which could suppress cell migration in BC (33). Accordingly, the different functions of RORγ in BC may be due to distinct cell origins and isotypes. For instance, when expressed by immune cells, RORγ acts as an immune suppressor, although when produced by cancer cells, it acts as a potential survival factor.
Skin Cancer
RORγ1 regulated tumor-promoting “emergency” granulo-monocytopoiesis by suppressing negative (Socs3 and Bcl3) and promoting positive (C/EBPb) regulators of granulopoiesis and RORγ1 promoted expansion of tumor-promoting MDSCs and TAM in fibrocarcinoma mice models (65). In a study exploring the function of Th17 cells in antitumor immunity, RORγt was found to be expressed by tumor-infiltrating Th17 cells. Th17 cells did not exhibit in vitro tumor cell killing activity, although CD8+ cytotoxic T cells stimulated by Th17 cells could activate the tumor killing response in a mouse B16 melanoma model (66).
In another study, RORγ-deficient mice showed inhibited melanoma growth, and this effect was identified to be IL-9 dependent (67). Together with RORα, RORγ was found to be expressed in melanoma cell lines and could bind with vitamin D3 derivatives, including 20(OH)D3 and 20,23(OH)2D3 (5), active forms of secosteroids and lumisterol can have anti-melanoma activity through action on RORα and RORγ (22, 24, 25, 39). In another study, RORγ and RORα expression levels were decreased during melanoma progression, with the lowest expression levels in stages III and IV primary melanomas and in melanoma (68). These studies of RORα and RORγ in melanoma suggest that RORα and RORγ could be important modulators affecting melanomagenesis, contributing to the anti-melanoma activity of vitamin D3 and act as potential therapeutic targets in adjuvant melanoma therapy (23, 24). The investigation of RORγ in skin cancer seems to be concentrated on melanoma and the isotype RORγ1, thus, there is a need for further exploration focusing on the regulation of RORγ and its roles in other types of skin cancer.
Lung Cancer
Our previous study showed that RORγ2 was highly expressed in non-small cell lung cancer (NSCLC) cells and also served as a prognostic factor (4). The expression of RORγt mRNA and protein was found to be downregulated in PBMCs from NSCLC patients compared with controls (69). However, RORγt mRNA was found to be upregulated in the peripheral blood of patients with NSCLC compared with that of healthy controls (70), which was confirmed in other studies (71, 72). Moreover, in a recent report, RORγt, together with Th17/IL-6R/pSTAT3/BATF, was upregulated in the tumor region of adenocarcinomas, except for squamous carcinomas of lung cancer (73). Studies focused on cancer cell-derived RORγt are infrequent and require additional attention.
Hepatocellular Carcinoma
RORγt mRNA was shown to be increased in HCC compared with a normal control group (74). By contrast, RORγt mRNA expression was found to be significantly lower in patients with steatosis/steatohepatitis, liver fibrosis, and HCC (27). Investigations into RORγt in HCC are rare, although RORγt is known to be expressed in hepatocytes. There could be additional modulatory roles for RORγt in HCC progression, and further studies are warranted.
Gastrointestinal Cancer
The gene expression of IL-17A and RORγ was not altered in gastric cancer (75). Foxp3+IL-17+ cells in colorectal cancer were found to express RORγt (76). Another study described RORγt-expressing regulatory T cells that were linked with the inability of these cells to suppress inflammation and were directly associated with the stage of human colon cancer (77). RORγt was also found to be involved in inhibiting colon carcinogenesis through binding with an E3 ubiquitin ligase, Itch, for ubiquitination (78). However, RORγt was not expressed within colorectal cancer tissues or by colorectal cancer-infiltrating CD4+ T cells (79). The expression and regulation of RORγt in gastric and colorectal cancer remain controversial, which makes it difficult to conclude the extent of RORγ/RORγt expression or the involvement in tumorigenesis. However, the differences in results from different studies might be attributable to the diversity of detection methods from tissue samples when considering individual variation.
Genitourinary Cancer
In castration-resistant prostate cancer (CRPC), RORγ was examined as a therapeutic target due to its overexpression and was found to directly drive androgen receptor (AR) hyperactivity through binding to an exonic RORE and partly through the NR coactivators SRC-1 and -3 (35). Therefore, inhibition of RORγ may represent a possible treatment option for CRPC. The transcriptional expression of RORγ mRNA from PBMCs exhibited high levels in cervical cancer compared with healthy controls (80). Additional observations are needed to elucidate the functions of RORγ in genitourinary cancer, where it may serve as a valuable therapeutic target.
Perspective
The three ROR family members are regarded as important regulators of the circadian rhythm, metabolism, and tumorigenesis. As discussed in this review, the protumor or antitumor effects of RORα and RORβ in cancer have not been intensively explored, requiring further study and evidence. However, as the main transcription factor in IL-17-expressing immune cells, RORγ has been investigated in various cancer cells and tumor-infiltrating cells (Figure 1), indicating that it might be a promising prognostic factor in lung and BC and a potential therapeutic target in prostate cancer.
Moreover, according to this review, we could conclude that the roles that RORs family members play in tumorigenesis vary in different cancers and, to some extent, depend on producing cells in the tumor microenvironment. Further concentration on the relationships between RORs and tumorigenesis should be meticulously organized and should deeply explore the clinical significance and the underlying mechanisms. More importantly, each RORs family members consists of several isoforms, and some previous studies have showed that different RORs isoforms present different biological functions (6). Thus, prospective reports on therapeutic targets of RORs in cancer should identify all isoforms of specific RORs.
Since RORα and RORγ are dysregulated in multiple cancer types based on published articles, they likely participate in carcinogenesis through modulating molecules such as IL-17, PRMT2, and AR or as receptors for sterols, such as vitamin D3 derivatives. Intriguingly, IL-17, AR, and vitamin D3 are therapeutic targets in rheumatoid arthritis and have potential, as a frontline treatment option for advanced prostate cancer and an adjuvant in melanoma management. Agonists or inverse agonists for RORα and RORγ might be efficiently inhibiting tumor growth and progression through activation or inactivation so that their ligands or targets, such as vitamin D3 derivatives and AR, become valid or invalid. Another promising new strategy for anticancer therapy might involve directly targeting tumor cells with RORα- and RORγ-specific modulators due to the correlations between high or low expression of RORα and RORγ and tumor progression. Third, RORs are sometimes produced by immune cells in tumor microenvironments and then induce antitumor or protumor activity by regulating tumor-related cytokines or chemokines. Accordingly, therapies targeting RORs producing immune cells could be novel treatments for certain cancers.
Author Contributions
JF, ZL and GY wrote the draft. YJ revised the manuscript. JF, TL, JX, and FW designed the figures. QH, MG, GH, MZ, LD and SL commented and added extra information.
Conflict of Interest Statement
The authors declare that the research was conducted in the absence of any commercial or financial relationships that could be construed as a potential conflict of interest.
Funding
This paper was supported by the National Natural Science Foundation of China (no. 81572942, no. 81770096), Hubei province technical innovation special major project (2017ACA094), the Natural Science Foundation of Hubei Province (no. 2014CFA057), the Health and Planning Commission Fund of Hubei Province (WJ2017M098), the Science and Technology Support Program of Hubei Province (YSF2015001294), the Wuhan Planning Project of Science and Technology (no. 2014060101010036), the Special Fund for Industrial Transformation and Upgrading, the Independent Innovation Research Fund for Huazhong University of Science and Technology (no. 017KFYXJJ253), the Scientific Training Program for Young Talents from Union Hospital of Tongji Medical College, Huazhong University of Science and Technology (to JF), and the National major new drug discovery technology major special projects (2018ZX09301001001).
References
1. Siegel RL, Miller KD, Jemal A. Cancer statistics, 2017. CA Cancer J Clin (2017) 67(1):7–30. doi:10.3322/caac.21387
2. Vacca M, Degirolamo C, Massafra V, Polimeno L, Mariani-Costantini R, Palasciano G, et al. Nuclear receptors in regenerating liver and hepato cellular carcinoma. Mol Cell Endocrinol (2013) 368(1–2):108–19. doi:10.1016/j.mce.2012.06.025
3. Li XB, Jiao S, Sun H, Xue J, Zhao WT, Fan L, et al. The orphan nuclear receptor EAR2 is overexpressed in colorectal cancer and it regulates survivability of colon cancer cells. Cancer Lett (2011) 309(2):137–44. doi:10.1016/j.canlet.2011.05.025
4. Huang Q, Fan J, Qian X, Lv Z, Zhang X, Han J, et al. Retinoic acid-related orphan receptor C isoform 2 expression and its prognostic significance for non-small cell lung cancer. J Cancer Res Clin Oncol (2016) 142(1):263–72. doi:10.1007/s00432-015-2040-0
5. Slominski AT, Kim TK, Takeda Y, Janjetovic Z, Brozyna AA, Skobowiat C, et al. RORalpha and ROR gamma are expressed in human skin and serve as receptors for endogenously produced noncalcemic 20-hydroxy- and 20,23-dihydroxyvitamin D. FASEB J (2014) 28(7):2775–89. doi:10.1096/fj.13-242040
6. Jetten AM, Takeda Y, Slominski A, Kang HS. Retinoic acid-related orphan receptor gamma (RORgamma): connecting sterol metabolism to regulation of the immune system and autoimmune disease. Curr Opin Toxicol (2018) 8:66–80. doi:10.1016/j.cotox.2018.01.005
7. Kojima H, Takeda Y, Muromoto R, Takahashi M, Hirao T, Takeuchi S, et al. Isoflavones enhance interleukin-17 gene expression via retinoic acid receptor-related orphan receptors alpha and gamma. Toxicology (2015) 329:32–9. doi:10.1016/j.tox.2015.01.007
8. Solt LA, Kumar N, Nuhant P, Wang Y, Lauer JL, Liu J, et al. Suppression of TH17 differentiation and autoimmunity by a synthetic ROR ligand. Nature (2011) 472(7344):491–4. doi:10.1038/nature10075
9. Dong C. TH17 cells in development: an updated view of their molecular identity and genetic programming. Nat Rev Immunol (2008) 8(5):337–48. doi:10.1038/nri2295
10. Cua DJ, Tato CM. Innate IL-17-producing cells: the sentinels of the immune system. Nat Rev Immunol (2010) 10(7):479–89. doi:10.1038/nri2800
11. Guillaumond F, Dardente H, Giguere V, Cermakian N. Differential control of Bmal1 circadian transcription by REV-ERB and ROR nuclear receptors. J Biol Rhythms (2005) 20(5):391–403. doi:10.1177/0748730405277232
12. Akashi M, Takumi T. The orphan nuclear receptor RORalpha regulates circadian transcription of the mammalian core-clock Bmal1. Nat Struct Mol Biol (2005) 12(5):441–8. doi:10.1038/nsmb925
13. Andre E, Conquet F, Steinmayr M, Stratton SC, Porciatti V, Becker-Andre M. Disruption of retinoid-related orphan receptor beta changes circadian behavior, causes retinal degeneration and leads to vacillans phenotype in mice. EMBO J (1998) 17(14):3867–77. doi:10.1093/emboj/17.14.3867
14. Masana MI, Sumaya IC, Becker-Andre M, Dubocovich ML. Behavioral characterization and modulation of circadian rhythms by light and melatonin in C3H/HeN mice homozygous for the RORbeta knockout. Am J Physiol Regul Integr Comp Physiol (2007) 292(6):R2357–67. doi:10.1152/ajpregu.00687.2006
15. Takeda Y, Jothi R, Birault V, Jetten AM. RORgamma directly regulates the circadian expression of clock genes and downstream targets in vivo. Nucleic Acids Res (2012) 40(17):8519–35. doi:10.1093/nar/gks630
16. Takeda Y, Kang HS, Angers M, Jetten AM. Retinoic acid-related orphan receptor gamma directly regulates neuronal PAS domain protein 2 transcription in vivo. Nucleic Acids Res (2011) 39(11):4769–82. doi:10.1093/nar/gkq1335
17. Jetten AM, Kang HS, Takeda Y. Retinoic acid-related orphan receptors alpha and gamma: key regulators of lipid/glucose metabolism, inflammation, and insulin sensitivity. Front Endocrinol (2013) 4:1. doi:10.3389/fendo.2013.00001
18. Kim K, Boo K, Yu YS, Oh SK, Kim H, Jeon Y, et al. RORalpha controls hepatic lipid homeostasis via negative regulation of PPARgamma transcriptional network. Nat Commun (2017) 8(1):162. doi:10.1038/s41467-017-00215-1
19. Shajari S, Laliena A, Heegsma J, Tunon MJ, Moshage H, Faber KN. Melatonin suppresses activation of hepatic stellate cells through RORalpha-mediated inhibition of 5-lipoxygenase. J Pineal Res (2015) 59(3):391–401. doi:10.1111/jpi.12271
20. Zhao Y, Xu L, Ding S, Lin N, Ji Q, Gao L, et al. Novel protective role of the circadian nuclear receptor retinoic acid-related orphan receptor-alpha in diabetic cardiomyopathy. J Pineal Res (2017) 62(3):e12378. doi:10.1111/jpi.12378
21. Slominski A, Fischer TW, Zmijewski MA, Wortsman J, Semak I, Zbytek B, et al. On the role of melatonin in skin physiology and pathology. Endocrine (2005) 27(2):137–48. doi:10.1385/ENDO:27:2:137
22. Slominski AT, Kim TK, Hobrath JV, Oak ASW, Tang EKY, Tieu EW, et al. Endogenously produced nonclassical vitamin D hydroxy-metabolites act as “biased” agonists on VDR and inverse agonists on RORalpha and RORgamma. J Steroid Biochem Mol Biol (2017) 173:42–56. doi:10.1016/j.jsbmb.2016.09.024
23. Brozyna AA, Jozwicki W, Roszkowski K, Filipiak J, Slominski AT. Melanin content in melanoma metastases affects the outcome of radiotherapy. Oncotarget (2016) 7(14):17844–53. doi:10.18632/oncotarget.7528
24. Slominski AT, Brozyna AA, Skobowiat C, Zmijewski MA, Kim TK, Janjetovic Z, et al. On the role of classical and novel forms of vitamin D in melanoma progression and management. J Steroid Biochem Mol Biol (2018) 177:159–70. doi:10.1016/j.jsbmb.2017.06.013
25. Slominski AT, Brozyna AA, Zmijewski MA, Jozwicki W, Jetten AM, Mason RS, et al. Vitamin D signaling and melanoma: role of vitamin D and its receptors in melanoma progression and management. Lab Invest (2017) 97(6):706–24. doi:10.1038/labinvest.2017.3
26. Xiong G, Wang C, Evers BM, Zhou BP, Xu R. RORalpha suppresses breast tumor invasion by inducing SEMA3F expression. Cancer Res (2012) 72(7):1728–39. doi:10.1158/0008-5472.CAN-11-2762
27. Ou Z, Shi X, Gilroy RK, Kirisci L, Romkes M, Lynch C, et al. Regulation of the human hydroxysteroid sulfotransferase (SULT2A1) by RORalpha and RORgamma and its potential relevance to human liver diseases. Mol Endocrinol (2013) 27(1):106–15. doi:10.1210/me.2012-1145
28. Chen Q, Chen L, Zhao R, Yang XD, Imran K, Xing CG. Microarray analyses reveal liver metastasis-related genes in metastatic colorectal cancer cell model. J Cancer Res Clin Oncol (2013) 139(7):1169–78. doi:10.1007/s00432-013-1424-2
29. Wen Z, Pan T, Yang S, Liu J, Tao H, Zhao Y, et al. Up-regulated NRIP2 in colorectal cancer initiating cells modulates the Wnt pathway by targeting RORbeta. Mol Cancer (2017) 16(1):20. doi:10.1186/s12943-017-0590-2
30. Davidson B, Abeler VM, Forsund M, Holth A, Yang Y, Kobayashi Y, et al. Gene expression signatures of primary and metastatic uterine leiomyosarcoma. Hum Pathol (2014) 45(4):691–700. doi:10.1016/j.humpath.2013.11.003
31. Matijevic T, Pavelic J. The dual role of TLR3 in metastatic cell line. Clin Exp Metastasis (2011) 28(7):701–12. doi:10.1007/s10585-011-9402-z
32. Liljevald M, Rehnberg M, Soderberg M, Ramnegard M, Borjesson J, Luciani D, et al. Retinoid-related orphan receptor gamma (RORgamma) adult induced knockout mice develop lymphoblastic lymphoma. Autoimmun Rev (2016) 15(11):1062–70. doi:10.1016/j.autrev.2016.07.036
33. Oh TG, Bailey P, Dray E, Smith AG, Goode J, Eriksson N, et al. PRMT2 and RORgamma expression are associated with breast cancer survival outcomes. Mol Endocrinol (2014) 28(7):1166–85. doi:10.1210/me.2013-1403
34. Hu X, Liu X, Moisan J, Wang Y, Lesch CA, Spooner C, et al. Synthetic RORgamma agonists regulate multiple pathways to enhance antitumor immunity. Oncoimmunology (2016) 5(12):e1254854. doi:10.1080/2162402X.2016.1254854
35. Wang J, Zou JX, Xue X, Cai D, Zhang Y, Duan Z, et al. Corrigendum: ROR-gamma drives androgen receptor expression and represents a therapeutic target in castration-resistant prostate cancer. Nat Med (2016) 22(6):692. doi:10.1038/nm0616-692b
36. Solt LA, Griffin PR, Burris TP. Ligand regulation of retinoic acid receptor-related orphan receptors: implications for development of novel therapeutics. Curr Opin Lipidol (2010) 21(3):204–11. doi:10.1097/MOL.0b013e328338ca18
37. Kallen J, Schlaeppi JM, Bitsch F, Delhon I, Fournier B. Crystal structure of the human RORalpha ligand binding domain in complex with cholesterol sulfate at 2.2 A. J Biol Chem (2004) 279(14):14033–8. doi:10.1074/jbc.M400302200
38. Stehlin-Gaon C, Willmann D, Zeyer D, Sanglier S, Van Dorsselaer A, Renaud JP, et al. All-trans retinoic acid is a ligand for the orphan nuclear receptor ROR beta. Nat Struct Biol (2003) 10(10):820–5. doi:10.1038/nsb979
39. Slominski AT, Kim TK, Hobrath JV, Janjetovic Z, Oak ASW, Postlethwaite A, et al. Characterization of a new pathway that activates lumisterol in vivo to biologically active hydroxylumisterols. Sci Rep (2017) 7(1):11434. doi:10.1038/s41598-017-10202-7
40. Wiesenberg I, Missbach M, Kahlen JP, Schrader M, Carlberg C. Transcriptional activation of the nuclear receptor RZR alpha by the pineal gland hormone melatonin and identification of CGP 52608 as a synthetic ligand. Nucleic Acids Res (1995) 23(3):327–33. doi:10.1093/nar/23.3.327
41. Lardone PJ, Guerrero JM, Fernandez-Santos JM, Rubio A, Martin-Lacave I, Carrillo-Vico A. Melatonin synthesized by T lymphocytes as a ligand of the retinoic acid-related orphan receptor. J Pineal Res (2011) 51(4):454–62. doi:10.1111/j.1600-079X.2011.00909.x
42. Slominski AT, Zmijewski MA, Jetten AM. RORalpha is not a receptor for melatonin (response to DOI 10.1002/bies.201600018). Bioessays (2016) 38(12):1193–4. doi:10.1002/bies.201600204
43. Ram PT, Dai J, Yuan L, Dong C, Kiefer TL, Lai L, et al. Involvement of the mt1 melatonin receptor in human breast cancer. Cancer Lett (2002) 179(2):141–50. doi:10.1016/S0304-3835(01)00873-4
44. Kojetin DJ, Burris TP. REV-ERB and ROR nuclear receptors as drug targets. Nat Rev Drug Discov (2014) 13(3):197–216. doi:10.1038/nrd4100
45. Chang MR, Lyda B, Kamenecka TM, Griffin PR. Pharmacologic repression of retinoic acid receptor-related orphan nuclear receptor gamma is therapeutic in the collagen-induced arthritis experimental model. Arthritis Rheumatol (2014) 66(3):579–88. doi:10.1002/art.38272
46. Wang Y, Solt LA, Kojetin DJ, Burris TP. Regulation of p53 stability and apoptosis by a ROR agonist. PLoS One (2012) 7(4):e34921. doi:10.1371/journal.pone.0034921
47. Dai J, Brooks Y, Lefort K, Getsios S, Dotto GP. The retinoid-related orphan receptor RORα promotes keratinocyte differentiation via FOXN1. PLoS One (2013) 8(7):e70392. doi:10.1371/journal.pone.0070392
48. Moretti RM, Montagnani Marelli M, Sala A, Motta M, Limonta P. Activation of the orphan nuclear receptor RORalpha counteracts the proliferative effect of fatty acids on prostate cancer cells: crucial role of 5-lipoxygenase. Int J Cancer (2004) 112(1):87–93. doi:10.1002/ijc.20387
49. Fischer TW, Zmijewski MA, Zbytek B, Sweatman TW, Slominski RM, Wortsman J, et al. Oncostatic effects of the indole melatonin and expression of its cytosolic and nuclear receptors in cultured human melanoma cell lines. Int J Oncol (2006) 29(3):665–72. doi:10.3892/ijo.29.3.665
50. Odawara H, Iwasaki T, Horiguchi J, Rokutanda N, Hirooka K, Miyazaki W, et al. Activation of aromatase expression by retinoic acid receptor-related orphan receptor (ROR) alpha in breast cancer cells: identification of a novel ROR response element. J Biol Chem (2009) 284(26):17711–9. doi:10.1074/jbc.M109.009241
51. Du J, Xu R. RORalpha, a potential tumor suppressor and therapeutic target of breast cancer. Int J Mol Sci (2012) 13(12):15755–66. doi:10.3390/ijms131215755
52. Chauvet C, Bois-Joyeux B, Danan JL. Retinoic acid receptor-related orphan receptor (ROR) alpha4 is the predominant isoform of the nuclear receptor RORalpha in the liver and is up-regulated by hypoxia in HepG2 human hepatoma cells. Biochem J (2002) 364(Pt 2):449–56. doi:10.1042/bj20011558
53. Byun JK, Choi YK, Kang YN, Jang BK, Kang KJ, Jeon YH, et al. Retinoic acid-related orphan receptor alpha reprograms glucose metabolism in glutamine-deficient hepatoma cells. Hepatology (2015) 61(3):953–64. doi:10.1002/hep.27577
54. Leon J, Casado J, Carazo A, Sanjuan L, Mate A, Munoz de Rueda P, et al. Gender-related invasion differences associated with mRNA expression levels of melatonin membrane receptors in colorectal cancer. Mol Carcinog (2012) 51(8):608–18. doi:10.1002/mc.20832
55. Lee JM, Kim IS, Kim H, Lee JS, Kim K, Yim HY, et al. RORalpha attenuates Wnt/beta-catenin signaling by PKCalpha-dependent phosphorylation in colon cancer. Mol Cell (2010) 37(2):183–95. doi:10.1016/j.molcel.2009.12.022
56. Gawlas K, Stunnenberg HG. Differential transcription of the orphan receptor RORbeta in nuclear extracts derived from Neuro2A and HeLa cells. Nucleic Acids Res (2001) 29(16):3424–32. doi:10.1093/nar/29.16.3424
57. Ueda E, Kurebayashi S, Sakaue M, Backlund M, Koller B, Jetten AM. High incidence of T-cell lymphomas in mice deficient in the retinoid-related orphan receptor RORgamma. Cancer Res (2002) 62(3):901–9.
58. Wang M, Chen P, Jia Y, He N, Li D, Ji C, et al. Elevated Th22 as well as Th17 cells associated with therapeutic outcome and clinical stage are potential targets in patients with multiple myeloma. Oncotarget (2015) 6(20):17958–67. doi:10.18632/oncotarget.4641
59. Braga WM, da Silva BR, de Carvalho AC, Maekawa YH, Bortoluzzo AB, Rizzatti EG, et al. FOXP3 and CTLA4 overexpression in multiple myeloma bone marrow as a sign of accumulation of CD4(+) T regulatory cells. Cancer Immunol Immunother (2014) 63(11):1189–97. doi:10.1007/s00262-014-1589-9
60. Feng P, Yan R, Dai X, Xie X, Wen H, Yang S. The alteration and clinical significance of Th1/Th2/Th17/Treg cells in patients with multiple myeloma. Inflammation (2015) 38(2):705–9. doi:10.1007/s10753-014-9980-4
61. Thibaudin M, Chaix M, Boidot R, Vegran F, Derangere V, Limagne E, et al. Human ectonucleotidase-expressing CD25(high) Th17 cells accumulate in breast cancer tumors and exert immunosuppressive functions. Oncoimmunology (2016) 5(1):e1055444. doi:10.1080/2162402X.2015.1055444
62. Benevides L, Cardoso CR, Tiezzi DG, Marana HR, Andrade JM, Silva JS. Enrichment of regulatory T cells in invasive breast tumor correlates with the upregulation of IL-17A expression and invasiveness of the tumor. Eur J Immunol (2013) 43(6):1518–28. doi:10.1002/eji.201242951
63. Irshad S, Flores-Borja F, Lawler K, Monypenny J, Evans R, Male V, et al. RORgammat(+) innate lymphoid cells promote lymph node metastasis of breast cancers. Cancer Res (2017) 77(5):1083–96. doi:10.1158/0008-5472.CAN-16-0598
64. Oh TG, Wang SM, Acharya BR, Goode JM, Graham JD, Clarke CL, et al. The nuclear receptor, RORgamma, regulates pathways necessary for breast cancer metastasis. EBioMedicine (2016) 6:59–72. doi:10.1016/j.ebiom.2016.02.028
65. Strauss L, Sangaletti S, Consonni FM, Szebeni G, Morlacchi S, Totaro MG, et al. RORC1 regulates tumor-promoting “emergency” granulo-monocytopoiesis. Cancer Cell (2015) 28(2):253–69. doi:10.1016/j.ccell.2015.07.006
66. Ankathatti Munegowda M, Deng Y, Mulligan SJ, Xiang J. Th17 and Th17-stimulated CD8(+) T cells play a distinct role in Th17-induced preventive and therapeutic antitumor immunity. Cancer Immunol Immunother (2011) 60(10):1473–84. doi:10.1007/s00262-011-1054-y
67. Purwar R, Schlapbach C, Xiao S, Kang HS, Elyaman W, Jiang X, et al. Robust tumor immunity to melanoma mediated by interleukin-9-producing T cells. Nat Med (2012) 18(8):1248–53. doi:10.1038/nm.2856
68. Brozyna AA, Jozwicki W, Skobowiat C, Jetten A, Slominski AT. RORalpha and RORgamma expression inversely correlates with human melanoma progression. Oncotarget (2016) 7(39):63261–82. doi:10.18632/oncotarget.11211
69. Zhao L, Yang J, Wang HP, Liu RY. Imbalance in the Th17/Treg and cytokine environment in peripheral blood of patients with adenocarcinoma and squamous cell carcinoma. Med Oncol (2013) 30(1):461. doi:10.1007/s12032-013-0461-7
70. Duan MC, Han W, Jin PW, Wei YP, Wei Q, Zhang LM, et al. Disturbed Th17/Treg balance in patients with non-small cell lung cancer. Inflammation (2015) 38(6):2156–65. doi:10.1007/s10753-015-0198-x
71. Duan M, Ning Z, Fu Z, Zhang J, Liu G, Wei Q, et al. Decreased IL-27 negatively correlated with Th17 cells in non-small-cell lung cancer patients. Mediators Inflamm (2015) 2015:802939. doi:10.1155/2015/802939
72. Li S, Li Y, Qu X, Liu X, Liang J. Detection and significance of TregFoxP3(+) and Th17 cells in peripheral blood of non-small cell lung cancer patients. Arch Med Sci (2014) 10(2):232–9. doi:10.5114/aoms.2014.42573
73. Balabko L, Andreev K, Burmann N, Schubert M, Mathews M, Trufa DI, et al. Increased expression of the Th17-IL-6R/pSTAT3/BATF/RorgammaT-axis in the tumoural region of adenocarcinoma as compared to squamous cell carcinoma of the lung. Sci Rep (2014) 4:7396. doi:10.1038/srep07396
74. Lin ZW, Wu LX, Xie Y, Ou X, Tian PK, Liu XP, et al. The expression levels of transcription factors T-bet, GATA-3, RORgammat and FOXP3 in peripheral blood lymphocyte (PBL) of patients with liver cancer and their significance. Int J Med Sci (2015) 12(1):7–16. doi:10.7150/ijms.8352
75. Kennedy CL, Najdovska M, Jones GW, McLeod L, Hughes NR, Allison C, et al. The molecular pathogenesis of STAT3-driven gastric tumourigenesis in mice is independent of IL-17. J Pathol (2011) 225(2):255–64. doi:10.1002/path.2933
76. Yang S, Wang B, Guan C, Wu B, Cai C, Wang M, et al. Foxp3+IL-17+ T cells promote development of cancer-initiating cells in colorectal cancer. J Leukoc Biol (2011) 89(1):85–91. doi:10.1189/jlb.0910506
77. Blatner NR, Mulcahy MF, Dennis KL, Scholtens D, Bentrem DJ, Phillips JD, et al. Expression of RORgammat marks a pathogenic regulatory T cell subset in human colon cancer. Sci Transl Med (2012) 4(164):164ra59. doi:10.1126/scitranslmed.3004566
78. Kathania M, Khare P, Zeng M, Cantarel B, Zhang H, Ueno H. Itch inhibits IL-17-mediated colon inflammation and tumorigenesis by ROR-gammat ubiquitination. Nat Immunol (2016) 17(8):997–1004. doi:10.1038/ni.3488
79. Punkenburg E, Vogler T, Buttner M, Amann K, Waldner M, Atreya R, et al. Batf-dependent Th17 cells critically regulate IL-23 driven colitis-associated colon cancer. Gut (2016) 65(7):1139–50. doi:10.1136/gutjnl-2014-308227
Keywords: retinoic acid receptor-related orphan receptors, RORα, RORβ, RORγ, cancer
Citation: Fan J, Lv Z, Yang G, Liao Tt, Xu J, Wu F, Huang Q, Guo M, Hu G, Zhou M, Duan L, Liu S and Jin Y (2018) Retinoic Acid Receptor-Related Orphan Receptors: Critical Roles in Tumorigenesis. Front. Immunol. 9:1187. doi: 10.3389/fimmu.2018.01187
Received: 24 January 2018; Accepted: 14 May 2018;
Published: 31 May 2018
Edited by:
Robin Anderson, Olivia Newton-John Cancer Research Institute, AustraliaReviewed by:
Thomas Burris, Washington University in St. Louis, United StatesAmorette Barber, Longwood University, United States
Andrzej T. Slominski, University of Alabama at Birmingham, United States
Copyright: © 2018 Fan, Lv, Yang, Liao, Xu, Wu, Huang, Guo, Hu, Zhou, Duan, Liu and Jin. This is an open-access article distributed under the terms of the Creative Commons Attribution License (CC BY). The use, distribution or reproduction in other forums is permitted, provided the original author(s) and the copyright owner are credited and that the original publication in this journal is cited, in accordance with accepted academic practice. No use, distribution or reproduction is permitted which does not comply with these terms.
*Correspondence: Yang Jin, d2h1aGp5JiN4MDAwNDA7MTI2LmNvbQ==
†These authors have contributed equally to this work.