Corrigendum: Phloroglucinol-Mediated Hsp70 Production in Crustaceans: Protection against Vibrio parahaemolyticus in Artemia franciscana and Macrobrachium rosenbergii
- 1Laboratory of Aquaculture & Artemia Reference Center, Department of Animal Sciences and Aquatic Ecology, Faculty of Bioscience Engineering, Ghent University, Ghent, Belgium
- 2ICAR-Central Inland Fisheries Research Institute (CIFRI), Barrackpore, India
- 3Department of Crop Protection, Faculty of Bioscience Engineering, Ghent University, Ghent, Belgium
- 4Laboratory of Animal Nutrition and Animal Product Quality, Department of Animal Sciences and Aquatic Ecology, Faculty of Bioscience Engineering, Ghent University, Ghent, Belgium
The halophilic aquatic bacterium, Vibrio parahaemolyticus, is an important aquatic pathogen, also capable of causing acute hepatopancreatic necrosis disease (AHPND) in shrimp resulting in significant economic losses. Therefore, there is an urgent need to develop anti-infective strategies to control AHPND. The gnotobiotic Artemia model is used to establish whether a phenolic compound phloroglucinol is effective against the AHPND strain V. parahaemolyticus MO904. We found that pretreatment with phloroglucinol, at an optimum concentration (30 µM), protects axenic brine shrimp larvae against V. parahaemolyticus infection and induced heat shock protein 70 (Hsp70) production (twofolds or more) as compared with the control. We further demonstrated that the Vibrio-protective effect of phloroglucinol was caused by its prooxidant effect and is linked to the induction of Hsp70. In addition, RNA interference confirms that phloroglucinol-induced Hsp70 mediates the survival of brine shrimp larvae against V. parahaemolyticus infection. The study was validated in xenic Artemia model and in a Macrobrachium rosenbergii system. Pretreatment of xenic brine shrimp larvae (30 µM) and Macrobrachium larvae (5 µM) with phloroglucinol increases the survival of xenic brine shrimp and Macrobrachium larvae against subsequent V. parahaemolyticus challenge. Taken together, our study provides substantial evidence that the prooxidant activity of phloroglucinol induces Hsp70 production protecting brine shrimp, A. franciscana, and freshwater shrimp, M. rosenbergii, against the AHPND V. parahaemolyticus strain MO904. Probably, phloroglucinol treatment might become part of a holistic strategy to control AHPND in shrimp.
Introduction
The acute hepatopancreatic necrosis disease (AHPND), originally known as early mortality syndrome, is a newly emerging shrimp disease causing havoc in the shrimp industry (1). The disease is caused by a specific virulent strain of Gram-negative marine bacterium, Vibrio parahaemolyticus, ubiquitous in estuarine and coastal waters (2). Since the AHPND outbreak first appeared in China in 2009, it has spread to Vietnam (2010), Malaysia (2011), Thailand (2012), Mexico (2013), Philippines (2015), and South America (2016) (3, 4). The shrimp production in AHPND-affected regions has dropped to ~60%, and the disease has caused global loss of $1 billion per year to the shrimp farming industry (5, 6). The V. parahaemolyticus becomes virulent VPAHPND by acquiring a 63- to 70-kb plasmid (pVA1) encoding the binary toxin PirAVp/PirBVp, which consist of two subunits PirA and PirB, and is homologous to the Photorhabdus luminescens insect-related (Pir) toxins PirA/PirB (7–9). Therefore, to enable the prevention of V. parahaemolyticus, an approach that focuses on understanding the host immune system and develops effective anti-infective strategies, in general, will have the highest chance of decreasing the risk of pathogenic V. parahaemolyticus infection in shrimps.
The conventional approach so far applied in the mitigation or cure of V. parahaemolyticus such as antibiotics and disinfectants had limited success (10). In addition, their usage in the food-producing sector is under severe scientific and public scrutiny due to the development of multiple resistances (11). Because of such concerns, there is an urgent need for the development of polyphenol plant-derived compounds or natural products that protect the shrimp and enhance the immune reactivity to V. parahaemolyticus. However, the critical problem in studying the anti-pathogenic effects of a polyphenol plant-based compound in vivo is the difficulty to either eliminate or extricate the effect of the microbial communities that occur naturally in the system (12). In addition, in germ-associated conditions, the compound of interest is either metabolized by microbial communities or influences the physiology of host-associated microbes, thereby making it difficult to understand the host response toward tested compound (13). Consequently, the selection of appropriate animal model system that permits better delineation of the biological effects of polyphenol plant-based compounds is paramount, for instance, on the induction of resistance to disease.
The brine shrimp (Artemia franciscana), an aquatic invertebrate that can be reared under gnotobiotic conditions (allowing full control over the host-associated microbial communities), is characteristically small, highly osmotolerant, branchiopod crustacean reported from a variety of harsh environment worldwide (14). Apart from its unusual life history, relatively low space and cost requirement for culture, rapid generation cycle (cyst to adult in 20–30 days), well-characterized developmental stages, the ability to form cyst that can be stored and used, and established molecular techniques like RNA interference (RNAi) make A. franciscana an exceptional experimental system to study the biological activity of polyphenol plant-based compounds as it allows to distinguish the direct effect on the host (by pre-exposing axenic brine shrimp larvae to the compound for a certain duration) from indirect effects (15–18).
Given the potential of polyphenol plant-based compound, phloroglucinol, as an anti-infective agent, understanding the underlying mechanism of action of this compound is considered vital. In this study, using a highly controlled gnotobiotic brine shrimp model system, we aimed to investigate whether phloroglucinol potentiates the generation of prooxidant activity and heat shock protein 70 (Hsp70) production in vivo and whether this putative effect could contribute to the induction of protective responses in Artemia against pathogenic V. parahaemolyticus MO904 strain. Furthermore, we also unraveled the mechanism behind the possible Hsp70-inducing effect of phloroglucinol. The results obtained in the gnotobiotic brine shrimp model system were validated in a xenic brine shrimp and Macrobrachium larvae, and we present novel finding which demonstrates that phloroglucinol is indeed a potent in vivo enhancer of Hsp70 and this effect mediates the induction of resistance in xenic brine shrimp and Macrobrachium larvae against V. parahaemolyticus.
Materials and Methods
Bacterial Strains for In Vivo Challenge Tests
In total, seven bacterial strains were used in the study: V. parahaemolyticus (AHPND strains) KM, MO605, RY, MO903, MO904, non-AHPND strain CAIM170, and Aeromonas hydrophila LVS3. LVS3 (autoclaved) were used as feed for brine shrimp larvae and MO904 as a pathogen for the challenge assay. The V. parahaemolyticus strains were confirmed for AHPND bacteria by PCR using AP3 primers (Table 1). Based on the amplification of DNA sequence of V. parahaemolyticus strains, it was confirmed as AHPND-causing strain that is not present in non-AHPND V. parahaemolyticus (Figure S1 in Supplementary Material) (19, 20). Before the challenge assay, the virulence of V. parahaemolyticus AHPND strains (KM, MO605, RY, MO903, and MO904) and non-AHPND strain (CAIM170) was evaluated using axenic Artemia as host. The V. parahaemolyticus MO904 strain provoking significant mortality in brine shrimp larvae up to 80% after 48 h was selected for the experimental challenge (Figure S2 in Supplementary Material). The MO904 strain used in the experiment was collected from the collection of aquatic important microorganism, CIAD, A.C. Mazatlàn unit of Aquaculture, Mazatlàn, Sinaloa, Mexico, and the isolates have been transferred to the Laboratory of Aquaculture and Artemia Reference Center, Ghent University, Belgium, under material transfer agreement in 2013. The stock culture of MO904 was streaked onto Luria Bertani agar plates (Himedia, India) containing 2% NaCl (LB+) to obtain pure colonies. A single colony was inoculated into LB+ broth (Himedia, USA), incubated overnight at 28°C under constant agitation (100/min), and the stocked culture was prepared in 40% glycerol and stored in −80°C. The LVS3 stock culture collected from the Laboratory of Aquaculture and Artemia Reference Center, Ghent University, Belgium, was streaked onto Marine agar plates (Difco Laboratories, Detroit, MI, USA). For cultivation, a single colony was inoculated in Marine broth (Difco Laboratories, Detroit, MI, USA).
Reagents
Phloroglucinol (≥99%) purchased from Sigma-Aldrich (Diegem, Belgium) was dissolved in sterile distilled water at 0.4 g/l (3.17 mM). Superoxide dismutase (SOD) (2,000–6,000 units/mg protein) and catalase (2,000–5,000 units/mg protein) were also obtained from Sigma-Aldrich. Catalase was dissolved in sterile distilled water at 0.5 g/l as stock solution. All the stock solutions were prepared fresh for each experiment.
Gnotobiotic Brine Shrimp Rearing System
The gnotobiotic brine shrimp larvae were produced by hatching brine shrimp cysts axenically (germ-free) following decapsulation and hatching procedures as described by Baruah et al. (21). Briefly, 1.5 g of cysts (EG® type, batch 21452, INVE Aquaculture, Dendermonde, Belgium) were hydrated in 89 ml of distilled water for 1 h. Sterile cysts and larvae were obtained via decapsulation using 3.3 ml NaOH (32%) and 50 ml NaOCl (50%). During the reaction, 0.2-µm filtered aeration was provided. All manipulation was carried out under a laminar flow hood and all tools were sterilized. The decapsulation was stopped after 2 min by adding 50 ml Na2S2O3 at 10 g/l. The decapsulated cysts were washed with filtered autoclaved seawater (FASW) containing 35 g/l of instant ocean® synthetic sea salt (Aquarium Systems, Sarrebourg, France). The cysts, suspended in 1-l glass bottles containing FASW, were incubated at 28°C for 28 h for hatching with a constant illumination of approximately 27 μE/m2 s. The hatched larvae at developmental stage instar II (mouth is opened to ingest particles) were collected, and the axenicity was verified by spread plating (100 ml) as well as by adding (500-µl) hatching water on Marine Agar and Marine Broth, respectively, followed by incubation at 28°C for 5 days (22). Experiments that started with non-axenic larvae were discarded.
Brine Shrimp Lethality Test and Challenge Assay
In total, four separate challenge tests were performed. The first test determined the dose–response relationship (protective effect) of phloroglucinol. Briefly, hatched brine shrimp larvae (at developmental stage II) were collected and counted volumetrically. Firstly, the sub-samples (1 ml) were tallied, and this number was multiplied by the total measured volume (ml) of hatched larvae. Thereafter, the larvae were transferred to sterile 50-ml falcon tubes containing 30 ml. The axenic larvae were pretreated with increasing concentrations of phloroglucinol (5, 10, 20, 30, 40, 50, and 100 µM) for 2 h at 28°C. They were repeatedly rinsed with FASW to wash away the compound and then allowed to recover for 2 h at 28°C. Following recovery period, a group of 20 larvae were transferred to sterile 40-ml glass tubes containing 10-ml FASW. Afterward, it was verified whether the pretreatment of phloroglucinol can protect the larvae against subsequent challenge with V. parahaemolyticus MO904 strain at 107 cells/ml. The survival of Artemia was scored 48 h after the addition of the pathogen. The non-pretreated larvae that were either challenged with V. parahaemolyticus or not were maintained as negative and positive controls. Each treatment and control were done in quintuplicate.
In the second test, the cytotoxic effect of phloroglucinol was determined in the axenic brine shrimp larvae as previously described by Baruah et al. (13). The axenic larvae were pretreated with an increasing concentration of phloroglucinol as described above in the dose–response study. Subsequently following recovery period, toxicity assay was performed in sterile 40-ml glass tubes with 20 larvae in 10-ml FASW per tube. The toxicity of the compound was determined after 48 h by scoring the number of survivors as previously described (13). Brine shrimp larvae that did not receive the phloroglucinol pretreatment served as control group. Five replicates were maintained for each treatment and control groups.
The third and fourth tests were performed to determine the mode of action of phloroglucinol. The axenic brine shrimp larvae were pretreated with an optimized dose of phloroglucinol (a dose that gave maximum protection to challenged larvae in the dose–response assay), a mixture of the antioxidant enzymes catalase (10 mg/l) and SOD (75 units), or a combination of phloroglucinol and antioxidant enzymes mixture in a similar manner as described above. The larvae in the control group did not undergo any pretreatment. Groups of 20 larvae were counted and distributed in sterile 40-ml glass tubes and then challenged with V. parahaemolyticus MO904 strain as described in the dose–response study. The survival of larvae was scored 2 days after the addition of pathogen. Brine shrimp larvae that did not receive phloroglucinol pretreatment either challenged with V. parahaemolyticus or not served as negative and positive controls. Each treatment and control were performed in five replicates.
Protein Extraction and Detection of Hsp70 in Brine Shrimp Larvae
Following pretreatment with phloroglucinol, antioxidant enzymes, and a mixture of antioxidant enzymes and phloroglucinol, live brine shrimp larvae (0.1 g w/v) were collected, rinsed in distilled water, immediately frozen in liquid nitrogen, and stored in −80°C. Artemia samples were homogenized in cold buffer K (150 mM sorbitol, 70 mM potassium gluconate, 5 mM MgCl2, 5 mM NaH2PO4, 40 mM HEPES, pH 7.4) (23), supplemented with protease inhibitor cocktail (Sigma-Aldrich®, USA). Subsequently, the samples were centrifuged at 2,200 × g for 1 min at 4°C, and the supernatant protein concentration was determined by the Bradford method (24) using bovine serum albumin as standard. Supernatant samples were then combined with loading buffer, vortexed, heated for 5 min at 95°C, and electrophoresed in 10% SDS-PAGE gel (BioRad, Belgium), with each lane receiving an equivalent amount of protein. HeLa (heat-shocked) cells (Enzo Life Sciences, USA) (6 µg) were loaded on to one well to serve as a positive control. Gels were then either stained with Coomassie Biosafe (BioRad Laboratories) or transferred to polyvinylidene fluoride membrane (BioRad Immuno-Blot™ PVDF) for antibody probing. Membrane was incubated with a blocking buffer [50 ml of 1× phosphate-buffered saline containing 0.2% (v/v) tween 20 and 5% (w/v) bovine serum albumin] for 60 min at room temperature and then with mouse monoclonal anti-Hsp70 antibody (3 A3) (Affinity BioReagents Incl., Golden, CO, USA), at the recommended dilution of 1:5,000 (22). Horseradish peroxidase-conjugated donkey anti-mouse IgG was used as secondary antibody at the recommended dilution of 1:2,500 (Affinity BioReagents Incl., Golden, CO, USA). The membrane was then incubated with clarity western ECL substrate (chemiluminescence reagent) (BioRad Laboratories) for 5 min, and the signals were detected by a ChemiDoc MP imaging system (BioRad, Belgium).
Lipid Peroxidation
The extent of lipid peroxidation was measured by the methods of Grotto et al. (25), and malondialdehyde (MDA) was determined calorimetrically. In brief, gnotobiotic brine shrimp larvae were pretreated with an optimized dose (30 µM) of phloroglucinol for 2 h at 28°C. They were repeatedly rinsed with FASW to wash away the compound and then allowed to recover for 2 h at 28°C. Following the recovery period, the samples containing 0.1 g (w/v) of live larvae were harvested from treatment and control and immediately frozen in liquid nitrogen and stored at −80°C for further MDA determination. The samples were hydrolyzed in mild alkaline conditions (1.5 M NaOH) at 60°C for 30 min in order to release bound MDA. The mixture was incubated with 6% H3PO4 and 0.8% thiobarbituric acid at 90°C for 45 min. After cooling, 10% SDS was added and the chromophore was extracted with n-butanol and absorbance was measured at 532 nm. The results were expressed as nmol MDA/g of Artemia.
Culture of A. franciscana for Microinjection
Approximately, 1.5 g of brine shrimp cysts was hydrated in 89 ml of distilled water for 1 h. Sterile cysts and larvae were collected following decapsulation as described above. The cysts, suspended in 1-l glass bottles containing FASW, were incubated at 28°C for 28 h for hatching with a constant illumination of approximately 27 μE/m2 s. Hatched larvae at developmental stage instar II (stage at which mouth is open to ingest particles) were collected and transferred to 2-l glass bottles containing FASW. The larvae were fed daily with non-axenic green algae (Tetraselmis suecica) and grown to adults in a controlled temperature room (28°C) with a constant illumination of approximately 27 μE/m2 s (26).
Preparation of dsRNA of A. franciscana hsp70 and Green Fluorescence Protein (gfp)
The dsRNA specific to the A. franciscana hsp70 (GenBank: AF427596.1) gene transcript was amplified (436 bp) using gene-specific primers (forward primer: 5′ GATGCAGGTGCCATTGC 3′ and reverse primer: 5′ AGCTCCTCAAAACGGGC 3′); each primer included the T7 promoter (5′ TAATACGACTCACTATAGGG 3′) at their 5′ ends. The PCR was performed at 94°C for 3 min, followed by 35 cycles of 94°C for 25 s, 53°C for 25 s, and 72°C for 2 min, and then the final extension at 72°C for 5 min. The purified PCR product was used as a template to generate dsRNA by using the MEGAscript RNAi kit (Ambion, USA), and the in vitro transcription reaction was performed according to the manufacturer’s instructions. Briefly, PCR-purified product was incubated overnight at 37°C with four ribonucleotides (ATP, CTP, GTP, and UTP), T7 enzyme mix, and reaction buffer. Then, the dsRNA was incubated at 37°C for 1 h with DNase I and RNAse for nuclease digestion to remove any template DNA and ssRNA that did not anneal. The hsp70 dsRNA, quantified in Nanodrop spectrophotometer (ThermoFisher Scientific, Belgium), was subjected to 1.5% agarose gel electrophoresis in order to check the integrity and efficiency of duplex formation. In addition, a gfp fragment (455bp) was amplified to prepare dsRNA of gfp, which served as a negative control using primers (forward 5′ AGAGCGCTTCTCGTTGGGG 3′ and reverse 5′ AGACCTGAAGTTCATCTGC 3′); each primer included the T7 promoter (5′ TAATACGACTCACTATAGGG 3′) at their 5′ ends.
Microinjection of Artemia Females With dsRNA
The dsRNA specific to hsp70 and gfp was mixed separately in 1:10 ratio (v/v) with 0.5% phenol red in Dulbecco’s phosphate-buffered saline (Sigma-Aldrich). The diluted dsRNA either hsp70 or gfp (250 nl of solution containing approximately 80 ng dsRNA) was injected with a FemtoJet® microinjector (Eppendorf, USA) using Femtotips II microinjection capillary tips (Eppendorf, USA) to egg sacs of adult Artemia female while viewing under stereomicroscope (Figure S3 in Supplementary Material) (18). A total of thirty numbers of females were injected with dsRNA for each hsp70 and gfp. Injected females were observed for 2 h, and the animals that retained dye, remained healthy, and could swim properly were employed for further experiments (Figure S4 in Supplementary Material). Mating pairs, i.e., injected adult female and male, were transferred to individual well in a 6-well plate containing 35 ppt artificial sea water. The Artemia pairs were fed daily with green algae, T. suecica, and maintained in a controlled temperature room (28°C) with a constant illumination of approximately 27 μE/m2 s. After 5 days, larvae were collected from each mating pairs (injected with dsRNA hsp70 and gfp) for further analysis.
Brine Shrimp Challenge Assay
The brine shrimp larvae obtained from a single female injected with dsRNA specific to either hsp70 or gfp were collected, counted, and thereafter transferred to sterile 50-ml falcon tubes containing 30-ml FASW. The larvae were pretreated with an optimized dose of phloroglucinol for 2 h at 28°C. They were rinsed repeatedly with FASW to wash away the compound and then allowed to recover for 2 h at 28°C. Following recovery, the larvae were transferred to sterile 40-ml glass tubes containing 10 ml of FASW. Subsequently, the tubes were inoculated with V. parahaemolyticus MO904 strain at 107 cells/ml. The survival of Artemia was scored 48 h after the addition of a pathogen. The brine shrimp larvae, obtained from either hsp70 or gfp dsRNA-injected females, which did not receive phloroglucinol pretreatment, and either challenged with V. parahaemolyticus or not, served as negative and positive controls.
Detection of hsp70 mRNA and Hsp70 Protein in Brine Shrimp Larvae
The larvae released from Artemia females injected with either hsp70 or gfp dsRNA were transferred to sterile 50-ml falcon tubes containing 30-ml FASW. The larvae were pretreated with an optimized dose of phloroglucinol for 2 h at 28°C. They were rinsed repeatedly with FASW to wash away the compound and then allowed to recover for 2 h at 28°C. Subsequently, the larvae were washed with sterile distilled water, counted (20 nos.), and collected in 1.5-ml sterile eppendorf tubes. The larvae were homogenized with a sterile microfuge pestle in 100 µl of TRIzol® reagent (Invitrogen, USA) (15, 18). The homogenate was centrifuged at 12,000 × g for 10 min at 4°C, and the supernatant was transferred to new sterile eppendorf tubes prior to RNA isolation according to the manufacturer’s protocols. An equal amount of larvae RNA (determined by NanoDrop spectrophotometer, ThermoFisher Scientific, Belgium) was used for cDNA synthesis, using Superscript® III First-Strand synthesis supermix for qRT-PCR kit (Invitrogen, USA). Subsequently, 5 µl of cDNA product was used for PCR amplification of hsp70 using the forward and reverse primers (as described above). PCR products were resolved in 1.5% (w/v) agarose gel, stained with GelRed™ nucleic acid gel stain, and visualized by ChemiDoc MP imaging system (BioRad, Belgium).
For the detection of Hsp70 protein in larvae from females injected with dsRNA specific to either hsp70 or gfp, the larvae were pretreated with an optimized dose of phloroglucinol as described above. Following pretreatment, 20 larvae (washed in sterile DW) were collected in 1.5-ml sterile eppendorf tubes. The larvae were homogenized in an equal volume of loading buffer (5 µl), vortexed, heated for 5 min at 95°C, and 15 µl of each sample was resolved in 10% SDS-PAGE gel (BioRad, Belgium) (15). Subsequently, Hsp70 protein is detected as described previously in Section “Protein Extraction and Detection of Hsp70 in Brine Shrimp Larvae.”
Xenic Brine Shrimp Challenge Assay
Brine shrimp cysts were hatched in xenic (germ-associated) condition, following decapsulation and hatching procedures as described by Sorgeloos et al. (27) and Baruah et al. (21), and brine shrimp larvae were collected after 28 h, at developmental stage II. The dose–response relationship of phloroglucinol was performed as described previously by Ref. (22) with slight modification. Briefly, the hatched brine shrimp larvae were collected, counted volumetrically, and thereafter transferred to 50-ml falcon tubes containing 30-ml seawater. The larvae were pretreated with increasing doses of phloroglucinol (1, 2.5, 5, 10, 20, 30, 40, 50, and 100 µM) for 2 h at 28°C. The larvae were rinsed repeatedly with seawater to wash away the compound and then allowed to recover for 2 h. Following recovery period, a group of 20 larvae were transferred to 40-ml glass tubes containing 10 ml of seawater. Subsequently, the tubes were inoculated with V. parahaemolyticus MO904 strain at 107 cells/ml, and the Artemia therein was fed with autoclaved LVS3 (107 cells/ml). The survival of Artemia was scored 2 days after the addition of pathogen, and each treatment was done in quintuplicate.
Macrobrachium rosenbergii Rearing System
The experiments were carried out at the Laboratory of Aquaculture & Artemia Reference Center, Ghent University, Belgium, in a controlled temperature room. Acclimatized adult freshwater shrimp (M. rosenbergii) obtained from laboratory and maintained in four separate freshwater recirculation units were used as brooders. For each experiment, larvae from single ovigerous female breeder were used. Matured female with fully ripe fertilized eggs (indicated by dark gray eggs) were transferred to the hatching tanks (30 l) containing brackish water (6 g/l salinity). Twenty-four hours after hatching, the larvae were collected and stocked in two separate brackish water (12 g/l salinity) recirculation units and fed with newly hatched axenic brine shrimp larvae. The broodstock management techniques were followed as previously described (28, 29).
Macrobrachium Lethality Test and Challenge Assay
In total, four separate experiments were performed in a controlled temperature room. In the first experiment, it was determined whether V. parahaemolyticus MO904 strain could cause infection in Macrobrachium larvae, and to this end, a dose–response study was determined. A group of 10 Macrobrachium larvae (8 days old) were collected and transferred to 150-ml glass tubes containing 100-ml sterile brackish water (12 g/l salinity) (29). Subsequently, the larvae were exposed to four doses (104, 105, 106, and 107 cells/ml of total rearing water) of V. parahaemolyticus MO904 strain. Macrobrachium larvae that were not challenged with V. parahaemolyticus served as negative control. Survival was scored at 12, 24, 36, and 48 h after the addition of the pathogen. The V. parahaemolyticus dose-inducing mortality of prawn larvae near to 80% in 24 h was considered as an optimum infectious dose and selected for the experimental challenge.
In the second experiment, the cytotoxic effect of phloroglucinol was determined in M. rosenbergii larvae as previously described by Baruah et al. (13). The larvae were pretreated with an increasing concentration of phloroglucinol as described above in the M. rosenbergii dose–response study. Subsequently following recover period, toxicity assay was performed in sterile 150-ml glass tubes with 10 larvae in 100-ml sterile brackish water (12 g/l salinity) per tube. The toxicity of compound was determined after 12, 24, 36, and 48 h by scoring the number of survivors as previously described (13). The larvae that did not receive the phloroglucinol pretreatment served as negative control. Each treatment and control were performed in three replicates.
In third and fourth experiments, the protective effect of phloroglucinol was determined. In brief, Macrobrachium larvae (8 days old) were collected, counted, and transferred to 500-ml sterile glass bottle containing sterile brackish water (12 g/l salinity). The larvae were pretreated with increasing concentrations of phloroglucinol (1, 2.5, 5, 10, 20, and 30 µM) for 2 h at 28°C. They were rinsed repeatedly with brackish water to wash away the compound and then allowed to recover for 2 h at 28°C. Following the recovery period, a group of 10 larvae were transferred to sterile 150-ml glass tubes containing 100-ml sterile brackish water (12 g/l salinity). Subsequently, the tubes were inoculated with V. parahaemolyticus MO904 strain at 106 cells/ml. The survival of larvae was scored 12, 24, 36, and 48 h after the addition of pathogen. Macrobrachium larvae that did not receive phloroglucinol pretreatment either challenged or not challenged with V. parahaemolyticus served as negative and positive controls.
Detection of Hsp70 in Macrobrachium Larvae
Following pretreatment with phloroglucinol, live Macrobrachium larvae (0.1 g w/v) were rinsed in sterile distilled water, collected in 1.5-ml sterile eppendorf tubes, and immediately frozen in liquid nitrogen and stored in −80°C. The samples were homogenized and processed for protein extraction as described above. The protein concentration was determined by the Bradford method (24) using bovine serum albumin as standard. The supernatant samples of Macrobrachium larvae were then electrophoresed in 10% SDS-PAGE gel (BioRad, Belgium) and transferred to polyvinylidene fluoride membrane (BioRad Immuno-Blot™ PVDF) for antibody probing to detect Hsp70 protein as described previously in the above section.
Statistical Analysis
Survival data were arcsin transformed to satisfy normality and homoscedasticity requirements as necessary. The data were then subjected to one-way analysis of variances (ANOVA) followed by Duncan’s multiple range test using statistical software statistical package for the social sciences version 24.0. The effect of RNAi on survival of brine shrimp larvae was analyzed by two-way ANOVA. P-values of ≤0.001 were considered significant. Survival data of M. rosenbergii were subjected to logistic regression analysis using GenStat 16 (VSN International, Hemel Hempstead, UK) to determine significant differences between the control and the treatment.
Results
Phloroglucinol Pretreatment of Axenic Brine Shrimp Larvae Protects Them Against Subsequent V. parahaemolyticus Challenge and Is Associated With Hsp70 Induction
In the first experiment, we investigated whether phloroglucinol could confer protection to the host Artemia against V. parahaemolyticus MO904 strain, which can cause AHPND in shrimp (Figure S5 in Supplementary Material). We found that brine shrimp larvae that received phloroglucinol pretreatment in the range of 5–100 µM exhibited a significant increase in the survival compared to positive control. However, the maximum survival (11-folds or more) was observed at a concentration of 30 µM (Figure 1A). By contrast, the survival of brine shrimp larvae pretreated with phloroglucinol higher than 30 µM appeared to decrease. Therefore, we next investigated whether it was due to a toxic effect of phloroglucinol. Brine shrimp larvae exposed with phloroglucinol in the range of 5–100 µM did not exhibit any significant difference in survival when compared with unexposed, control larvae (Figure 1B). This result indicates that phloroglucinol appeared to be nontoxic to the experimental animal under the present experimental conditions. Next, to determine whether the induction of Hsp70 may be a mechanism supporting the resistance of phloroglucinol-treated brine shrimp larvae against V. parahaemolyticus challenge, we analyzed the temporal induction profile of Hsp70 in vivo, since the role of inducible Hsp70 in eliciting protective immunity against bacterial infection has been previously described (12, 13, 17, 20, 30, 31). Interestingly, we found that phloroglucinol pretreatment significantly increased Hsp70 production (twofolds or more) in brine shrimp larvae as compared with the control (Figure 1C). This result suggests that phloroglucinol is an effective inducer of Hsp70 and the protective effect of phloroglucinol is paralleled with the Hsp70 induction.
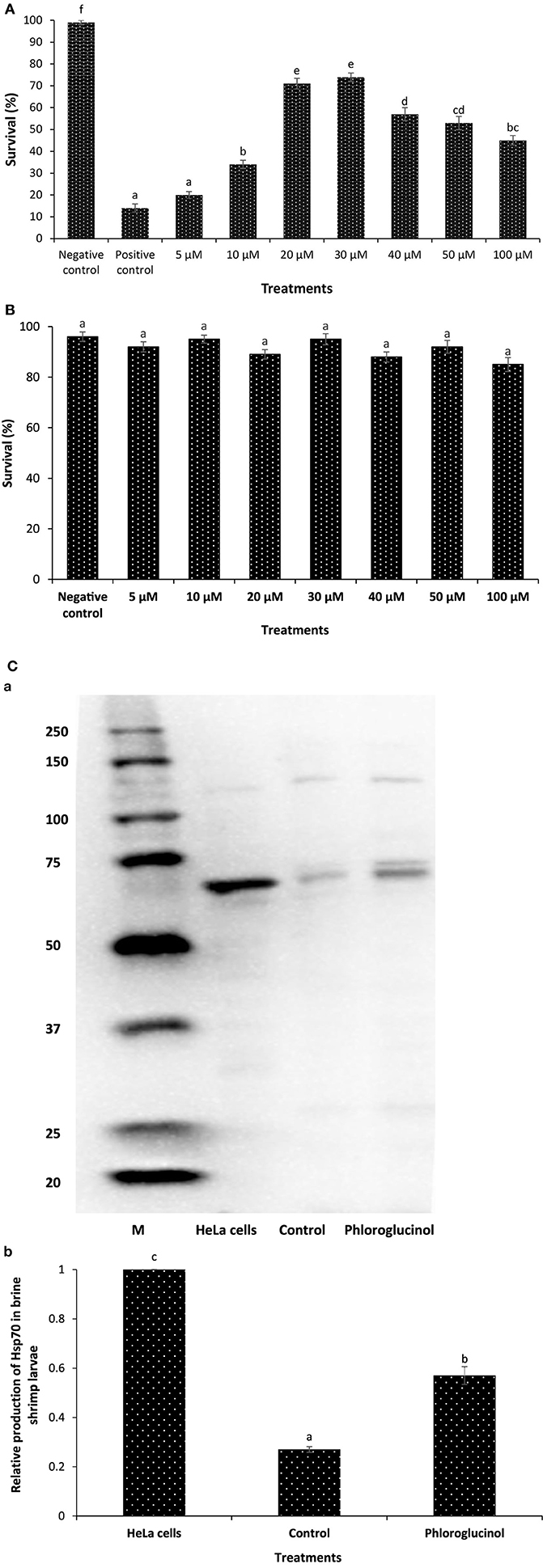
Figure 1. Phloroglucinol pretreatment of axenic brine shrimp larvae protects them against subsequent Vibrio parahaemolyticus challenge and is associated with heat shock protein (Hsp70) induction. (A) Survival (%) of phloroglucinol-pretreated brine shrimp larvae after 48 h of challenge with V. parahaemolyticus MO904. The larvae were pretreated with phloroglucinol at the indicated doses for 2 h, rinsed to wash away the compound, and then allowed to recover for 2 h. The larvae were subsequently challenged with V. parahaemolyticus at 107 cells/ml of rearing water. Non-pretreated larvae that were either challenged with V. parahaemolyticus (positive control) or unchallenged (negative control) served as controls. Error bars represent the standard error of five replicates; different letters indicate significant differences (P < 0.001). (B) Toxicity of phloroglucinol to gnotobiotic brine shrimp larvae. The larvae were pretreated with phloroglucinol at the indicated doses for 2 h, rinsed to wash away the compound, and then allowed to recover for 2 h. Non-pretreated larvae served as negative controls. Survival was recorded 48 h after the recovery period. Error bars represent the standard error of five replicates; different letters indicate significant differences (P < 0.001). (C) Induction of Hsp70 protein in brine shrimp larvae pretreated with phloroglucinol (30 µM). Non-pretreated larvae were maintained as control. (a) Protein extracted from control and treatment groups was resolved on an SDS-PAGE gel and then transferred to a polyvinylidene fluoride membrane and probed with an antibody to brine shrimp Hsp70. Molecular mass standards (M) in kilodaltons (protein ladder) are shown on the left. The induction of Hsp70 in HeLa cells was regarded as 1. (b) Quantitative analysis of Hsp70 levels in brine shrimp larvae is presented relative to Hsp70 production in HeLa cells. Error bars represent the standard error of three replicates; different letters indicate significant differences (P < 0.001).
Phloroglucinol-Generated Prooxidant Activity Mediates the Protective Response in Brine Shrimp Larvae
We next sought to investigate the mechanism of action of phloroglucinol in inducing Hsp70 within Artemia. Since reactive oxygen species (e.g., hydrogen peroxide, H2O2) generated from the polyphenolic compounds has been linked with Hsp70 induction (17, 32), we hypothesized that phloroglucinol induced Hsp70 by the mechanism of generating ROS and that eliminating these molecules by using ROS-scavenging enzymes, the phloroglucinol-mediated Hsp70 induction can be neutralized. We verified these findings by two alternative approaches. We found that brine shrimp larvae pretreated with phloroglucinol showed a significant increase in the survival and Hsp70 production (Figures 2A,B). However, co-pretreatment of larvae with phloroglucinol and antioxidant enzyme mixture resulted in a significant decrease in the survival and Hsp70 production as compared to the larvae pretreated with phloroglucinol (Figures 2A,B). We also further examined the prooxidant characteristics (i.e., generation of ROS: hydrogen peroxide, H2O2) of the phloroglucinol through lipid peroxidation by analyzing MDA content in the phloroglucinol-treated brine shrimp larvae. We found that pretreated larvae have significantly higher MDA content/g Artemia when compared with the negative control larvae (Figure 2C). These results indicate that prooxidant activity generated by phloroglucinol in the Artemia appeared to be, at least in part, involved in the induction of Hsp70 within brine shrimp larvae.
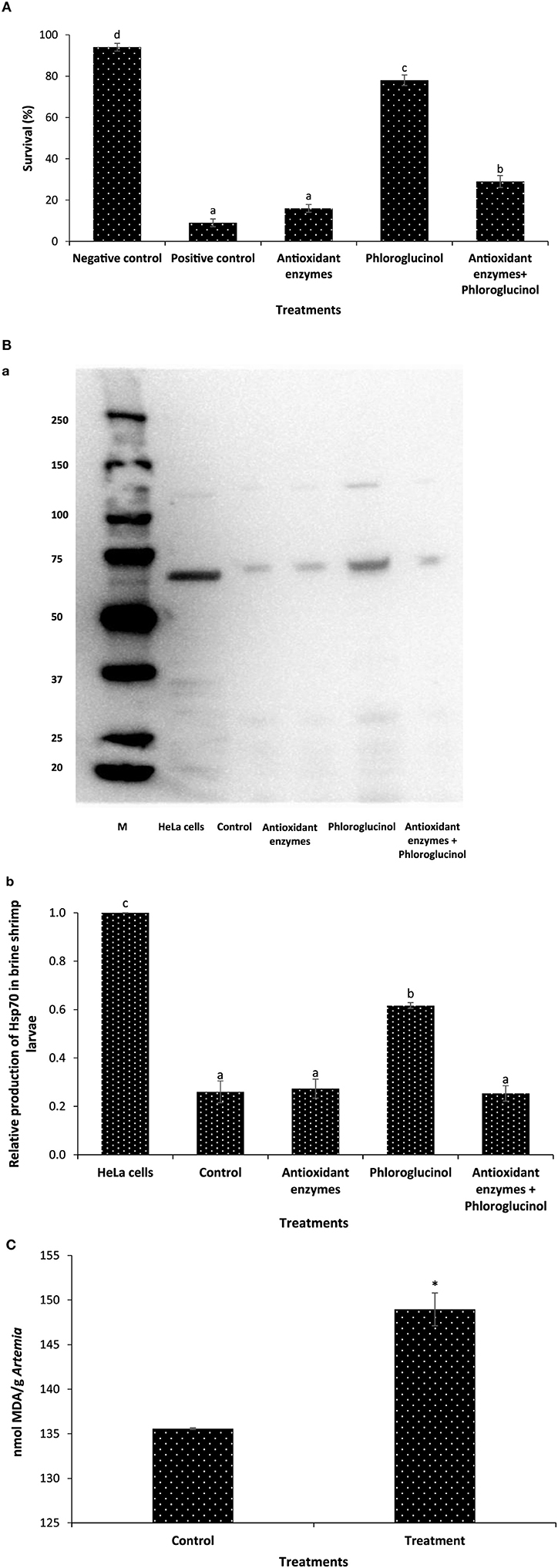
Figure 2. Phloroglucinol-generated prooxidant activity mediates the protective response in brine shrimp larvae. (A) Survival (%) of brine shrimp larvae pretreated with antioxidant enzymes, phloroglucinol, or in combination with both (antioxidant enzymes and phloroglucinol) after 48 h of challenge with Vibrio parahaemolyticus MO904. The larvae were pretreated with antioxidant enzymes (catalase, 10 mg/l and superoxide dismutase (SOD), 75 units), phloroglucinol (30 µM), and mixture of antioxidant enzymes and phloroglucinol for 2 h, rinsed to wash away the compound, and then allowed to recover for 2 h. The larvae were subsequently challenged with V. parahaemolyticus at 107 cells/ml of rearing water. Non-pretreated larvae that were either challenged with V. parahaemolyticus (positive control) and those not challenged with V. parahaemolyticus (negative control) served as controls. Error bars represent the standard error of five replicates; different letters indicate significant differences (P < 0.001). (B) Induction of heat shock protein (Hsp70) protein in brine shrimp larvae. The brine shrimp larvae were pretreated with antioxidant enzymes, phloroglucinol, or in combination with both (antioxidant enzymes and phloroglucinol) as described in Figure 2A. Non-pretreated larvae were maintained as control. (a) Protein extracted from different treatment groups was resolved on an SDS-PAGE gel and then transferred to a polyvinylidene fluoride membrane and probed with an antibody to brine shrimp Hsp70. Molecular mass standards (M) in kilodaltons are shown on the left. The induction of Hsp70 in HeLa cells was regarded as 1. (b) Quantitative analysis of Hsp70 levels in the brine shrimp larvae is presented relative to Hsp70 production in HeLa cells. Error bars represent the standard error of three replicates; different letters indicate significant differences (P < 0.001). (C) Effect of phloroglucinol on lipid peroxidation in brine shrimp larvae. Lipid peroxidation is expressed as malondialdehyde (MDA) content in each sample. The larvae were pretreated with phloroglucinol (30 µM) for 2 h, rinsed to wash away the compound, and then allowed to recover for 2 h. The larvae were subsequently collected and analyzed for MDA/g of Artemia. Non-pretreated larvae were maintained as control. Error bars represent the standard error of two independent samples; asterisks represent significant difference between the control and the treatment, *P < 0.001.
Knockdown of Hsp70 by RNAi Reduces the Tolerance of Brine Shrimp Larvae to V. parahaemolyticus Infection
There exists a correlation between the amount of induced Hsp70 and the degree of improved protective response against V. parahaemolyticus. To gain insight into the molecular bases for the observed effects, we used RNAi to assess the role of Hsp70 in protecting brine shrimp larvae against V. parahaemolyticus MO904 strain. As RNAi-mediated gene-silencing technique has been very frequently used to investigate the function of critical genes involved in the immune response (18, 33–36), we have prepared dsRNA specific to Hsp70 and injected A. franciscana females in order to eliminate Hsp70 mRNA and protein from larvae released from these females. At first, we have examined the effect of Hsp70 knockdown on the survival of larvae released from females injected with either hsp70 dsRNA or gfp. We found that larvae lacking or containing Hsp70 survived equally after being released from injected females and Hsp70 knockdown did not affect the viability of brine shrimp larvae. Furthermore, our analysis revealed that phloroglucinol pretreatment to larvae collected from females injected with either hsp70 dsRNA (−Hsp70) or gfp (+Hsp70) showed a significant difference in the survival against V. parahaemolyticus (Figure 3A). The survival was significantly increased (~6-folds) in the pretreated larvae containing Hsp70 when compared with larvae lacking Hsp70 (Table S1). We therefore reasoned that the difference in the survival of larvae might be due to the difference in the expression of Hsp70. To test the hypothesis that dsRNA may have knockdown hsp70 mRNA and protein, we have analyzed hsp70 mRNA and Hsp70 protein in A. franciscana larvae collected from females injected with dsRNA. We found that females injected with hsp70 dsRNA knockdown the hsp70 mRNA in larvae (Figure 3B). Furthermore, the Western blot analysis of Hsp70 protein showed that Hsp70 was absent in the larvae released by females injected with dsRNA hsp70, but not in the larvae from females injected with gfp dsRNA (Figure 3C). These results therefore confirm that phloroglucinol-mediated Hsp70 production plays an essential role in providing protection against V. parahaemolyticus MO904 strain.
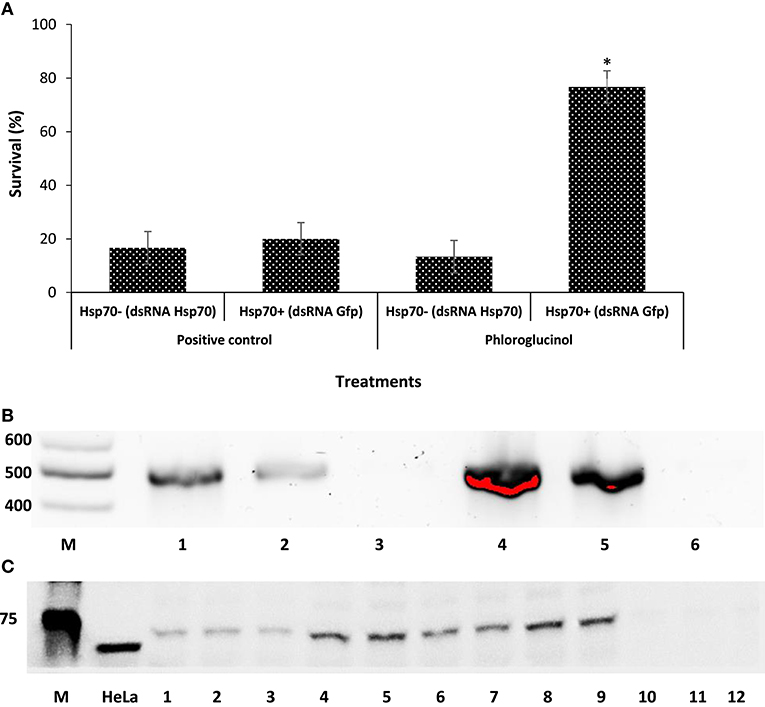
Figure 3. Knockdown of heat shock protein 70 (Hsp70) by RNA interference (RNAi) reduces the tolerance of brine shrimp larvae to Vibrio parahaemolyticus infection. (A) Hsp70 protects brine shrimp larvae against V. parahaemolyticus MO904. Brine shrimp larvae obtained from females injected with either gfp (+Hsp70) or hsp70 dsRNA (−Hsp70) were pretreated with phloroglucinol (30 µM) for 2 h, rinsed to wash away the compound, and then allowed to recover for 2 h. The larvae were subsequently challenged with V. parahaemolyticus at 107 cells/ml. Phloroglucinol non-pretreated larvae with and without hsp70 that were challenged with V. parahaemolyticus served as positive control. Error bars represent the standard error of six replicates; asterisks represent significant difference between the control and the treatment, *P < 0.001. (B) Knockdown of hsp70 mRNA in brine shrimp larvae. Equal amounts of RNA from brine shrimp larvae were amplified by RT-PCR and the products were resolved by electrophoresis in agarose gels. M—100 bp DNA ladder, Lane 1—wild-type brine shrimp larvae, Lane 2—brine shrimp larvae injected with dsRNA gfp, Lane 3—brine shrimp larvae injected with dsRNA hsp70, Lane 4—wild-type brine shrimp larvae pretreated with phloroglucinol, Lane 5—brine shrimp larvae injected with dsRNA gfp and pretreated with phloroglucinol, Lane 6—brine shrimp larvae injected with dsRNA hsp70 and pretreated with phloroglucinol. (C) Knockdown of Hsp70 protein in brine shrimp larvae. The brine shrimp larvae from females injected with dsRNA for either gfp or hsp70 were resolved by electrophoresis in SDS polyacrylamide gels, blotted to nitrocellulose, and probed with Hsp70 antibody (3A3). The secondary antibody was HRP-conjugated donkey anti-mouse IgG. M—Protein ladder in kilodaltons, Lanes 1–3—wild-type brine shrimp larvae, Lanes 4–6—wild-type brine shrimp larvae pretreated with phloroglucinol, Lanes 7–9—brine shrimp larvae injected with dsRNA gfp and pretreated with phloroglucinol, Lanes 10–12—brine shrimp larvae injected with dsRNA hsp70 and pretreated with phloroglucinol.
Pretreatment of Xenic Brine Shrimp Larvae and Macrobrachium Larvae With Phloroglucinol Protects Them Against Subsequent V. parahaemolyticus Challenge
To further confirm the contribution of phloroglucinol on the survival of xenic brine shrimp and Macrobrachium larvae against V. parahaemolyticus, we conducted additional in vivo survival assay as described in Figure 4. We assumed that phloroglucinol imparts protection to xenic brine shrimp larvae against V. parahaemolyticus infection and does not have any significant difference when compared with gnotobiotic brine shrimp larvae. Second, we have used M. rosenbergii system to further verify that phloroglucinol mediates the production of Hsp70 and induces resistance in Macrobrachium larvae against pathogenic V. parahaemolyticus MO904 strain.
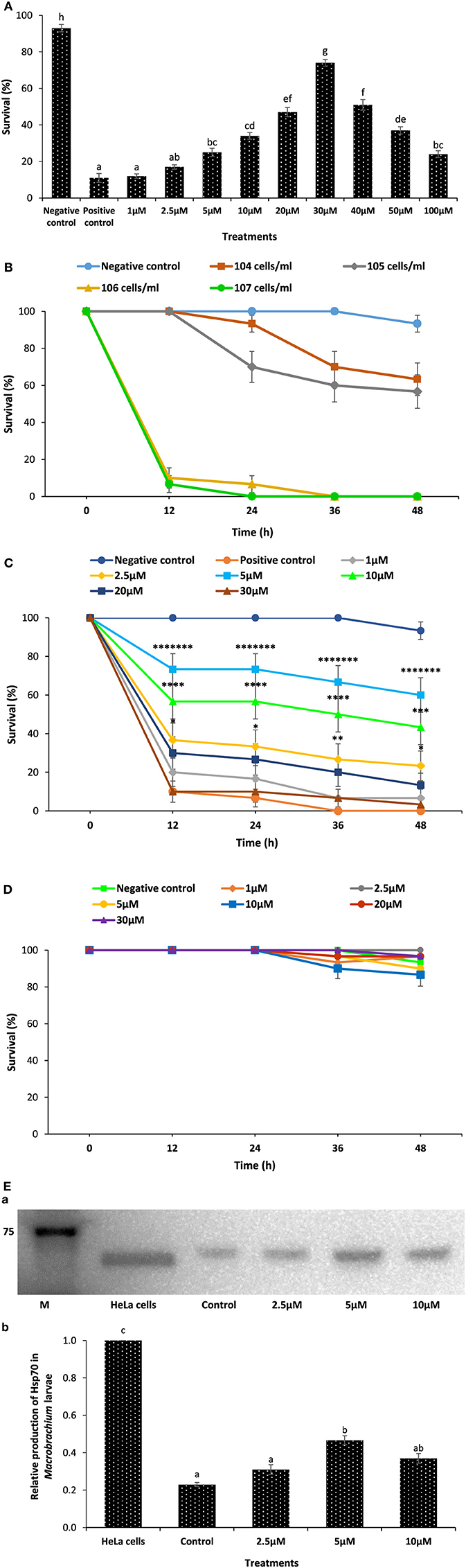
Figure 4. Pretreatment of xenic brine shrimp larvae and Macrobrachium larvae with phloroglucinol protects them against subsequent Vibrio parahaemolyticus challenge. (A) Survival (%) of phloroglucinol-pretreated xenic brine shrimp larvae after 48 h of challenge with V. parahaemolyticus MO904. The larvae were pretreated with phloroglucinol at the indicated doses for 2 h, rinsed to wash away the compound, and then allowed to recover for 2 h. The larvae were subsequently challenged with V. parahaemolyticus at 107 cells/ml of rearing water. Non-pretreated larvae that were either challenged with V. parahaemolyticus (positive control) or unchallenged (negative control) served as controls. Error bars represent the standard error of five replicates; different letters indicate significant differences (P < 0.001). (B) Proportion of survived of Macrobrachium larvae (8 days old) after 12, 24, 36, and 48 h post challenge with V. parahaemolyticus MO904 strain. Four infectious doses (104, 105, 106, and 107 cells/ml) were tested in the Macrobrachium larvae. The V. parahaemolyticus dose provoking significant mortality near to 80% at 24 h in Macrobrachium larvae was considered an optimum dose and selected for the experimental challenge. The unchallenged was served as negative controls. Values are presented as mean ± SE (n = 3). (C) The effect of phloroglucinol pretreatment on the survival of Macrobrachium larvae (8 days old) after 12, 24, 36, and 48 h post challenge with V. parahaemolyticus. The larvae were pretreated with phloroglucinol at the indicated doses for 2 h, rinsed to wash away the compound, and then allowed to recover for 2 h. The Macrobrachium larvae were subsequently challenged with V. parahaemolyticus at 106 cells/ml of rearing water. Non-pretreated larvae that were either challenged with V. parahaemolyticus (positive control) and those not challenged with V. parahaemolyticus (negative control) served as controls. Values are presented as mean ± SE (n = 3). Asterisks represent significant difference between the control and the treatment. *P < 0.05, **P < 0.01, ***P < 0.001, ****P < 0.0001, *****P < 0.00001, ******P < 0.000001, *******P < 0.0000001. (D) Toxicity of phloroglucinol to Macrobrachium larvae (8 days old). The larvae were pretreated with phloroglucinol at the indicated doses for 2 h, rinsed to wash away the compound, and then allowed to recover for 2 h. Non-pretreated larvae served as controls. Survival was recorded 48 h after the recovery period. Values are presented as mean ± SE (n = 3). (E) Induction of Hsp70 protein in Macrobrachium larvae (8 days old) pretreated with phloroglucinol at indicated doses. Non-pretreated larvae were maintained as control. (a) Protein extracted from different treatment groups was resolved on an SDS-PAGE gel and then transferred to a polyvinylidene fluoride membrane and probed with an antibody to brine shrimp Hsp70. Molecular mass standards (M) in kilodaltons are shown on the left. The induction of Hsp70 in HeLa cells was regarded as 1. (b) Quantitative analysis of Hsp70 levels in the brine shrimp larvae is presented relative to Hsp70 production in HeLa cells. Error bars represent the standard error of three replicates; different letters indicate significant differences (P < 0.001).
In Vivo Confirmation in Xenic Brine Shrimp Larvae
We investigated whether phloroglucinol contributes to the induction of protective response in xenic brine shrimp larvae against V. parahaemolyticus MO904 strain. In agreement with the gnotobiotic brine shrimp larvae survival results, we found that xenic brine shrimp larvae pretreated with varying concentrations of phloroglucinol protect larvae when subsequently challenged with pathogenic V. parahaemolyticus (Figure 4A). Moreover, the survival was recorded maximum (six times or more) at a concentration of 30 µM when compared with the control larvae. The phloroglucinol pretreatment at a concentration higher than 30 µM did not further improve the larvae survival. By contrast, survival tended to decrease with further increase in the concentration.
Further Validation in M. rosenbergii
In the validation experiment, the efficiency of phloroglucinol to protect Macrobrachium larvae from V. parahaemolyticus infection was investigated. At first, we have examined the optimum dose of V. parahaemolyticus MO904 strain required for the dose–response study. We found that M. rosenbergii post larvae (8 days old) when exposed to four infectious doses of V. parahaemolyticus (104, 105, 106, and 107 cells/ml) showed a significant difference in the survival of larvae (Figure 4B). The V. parahaemolyticus infectious dose, 106 cells/ml, provoking a significant mortality near to 80% at 24 h, was considered an optimum dose and selected for the experimental challenge.
Earlier in the study, it is shown that phloroglucinol induces the Hsp70 production and protects brine shrimp larvae from V. parahaemolyticus infection. This has led to the suggestion that phloroglucinol might have a protective response in other shrimp species, e.g., freshwater shrimp, M. rosenbergii. To test the hypothesis, we first investigated whether phloroglucinol pretreatment has a significant role in the survival of Macrobrachium larvae, and we found that larvae pretreated with phloroglucinol showed a significant increase (sevenfolds or more) in the survival as compared to the control (positive control) (Figure 4C). We have also investigated whether the phloroglucinol has some toxic effect on the larvae of Macrobrachium, since the survival of larvae pretreated above 5 µM phloroglucinol concentration appeared to decrease. We found that phloroglucinol pretreatment does not have any toxic effect on the experimental animals (Figure 4D). In the next experiment, we examined whether an observed increase in the survival of larvae is related to the increase in the Hsp70 production. We found that Hsp70 production increased significantly (twofolds or more) in the Macrobrachium larvae pretreated with phloroglucinol as compared to the control (Figure 4E). These results indicate that phloroglucinol stimulates the Hsp70 production in larvae, which may be involved in eliciting immune response and protects Macrobrachium larvae from pathogenic V. parahaemolyticus infection.
Discussion
The bacterial species, V. parahaemolyticus and especially AHPND-causing strains, such as MO904 are a major cause of infectious disease and mortality in wild and cultured shrimp species (1, 3). The AHPND strains of V. parahaemolyticus after acquiring a plasmid (pVA1) (63–70 kb) express deadly toxin PirAVP and PirBVP that damages the shrimp tissue, primarily the hepatopancrease (Hp) R (resorptive) cells, B (blister) cells, F (fibrillar) cells, and E (embryonic cells), resulting in the shrinkage of Hp tubular epithelial cells and sloughing of Hp tubular lumen (36). The larval stages of shrimp species, in general, are more susceptible to the pathogenic V. parahaemolyticus, and the infection develops quickly, starting approximately after 8 days of post-larvae stocking, and severe mortalities occur during the first 20–30 days of culture (37). This motivated us to develop an infection model for AHPND using gnotobiotic Artemia, and we found that V. parahaemolyticus MO904 strain indeed could cause mortality in axenic Artemia. Subsequent studies were carried out to validate these findings under xenic condition, and results showed that MO904 strain was virulent to both xenic Artemia and Macrobrachium larvae. However, it remains to be established whether the mortality is PirAVP and PirBVP mediated. It would especially be of interest to establish which cells would be affected by PirAVP and PirBVP.
The ability to stimulate defense system of invertebrate is considered to be central to control the pathogenesis of many microbial pathogens (17). In case of brine shrimp that lacks acquired immune system and depends on innate immune factors to build up resistance against microbial pathogens (like other invertebrate species), eliciting innate immune response might be a potential preventive modality for the control of biotic and abiotic stressors in aquaculture production system (33, 34, 38–41). Previous works have shown that the ability of natural/plant-based compounds to increase the resistance of host against bacterial infection is probably facilitating enhanced immune response (12, 20, 27, 38). Although the functional significance of natural or plant-based compounds in providing protection to Artemia against bacterial infection by a mechanism of inducing Hsp70 is reported in these studies, the role of natural/plant-based compounds against V. parahaemolyticus and the molecular mechanism behind the protective effect in host remain to be established. In this study, using gnotobiotic Artemia model system, it was shown that phloroglucinol, a polyphenol plant-based compound, isolated from marine brown algae belonging to the family Laminariaceae, containing 1,3,5-trihydroxy benzene as the basic moiety (42), provides protection to brine shrimp larvae against V. parahaemolyticus MO904 strain.
The ability of polyphenol plant-based compounds to induce resistance in host and prevent the microbial infection is functionally dependent on antioxidant property, prooxidant activity, and antimicrobial effects (12, 43, 44). Our results indicate that pretreatment of phloroglucinol exerted a protective effect in brine shrimp larvae against V. parahaemolyticus by mechanism of its prooxidant activity (e.g., generation of hydrogen peroxide, H2O2) (Figures 2A–C). In fact, such protective effect of phloroglucinol was not observed if the generation of reactive oxygen species was counteracted by the addition of antioxidant enzyme mixture (SOD and catalase) (Figure 2A) (17). In addition, we have also provided evidence for a link between the phloroglucinol prooxidant action and an increase in the Hsp70 protein levels, which might stimulate the immune response instigating resistance against V. parahaemolyticus in the brine shrimp larvae. This assumption is supported by the observation that the protective phenomenon was not observed when the induction of Hsp70 was inhibited by the ROS-scavenging enzymes mixture (Figures 2A,B) and consistent with the known beneficial roles that Hsp70 plays in defining the tolerance of organism to bacterial infection by eliciting the protective immunity (33, 45, 46).
However, phloroglucinol-mediated Hsp70 induction may represent only one of the possible underlying mechanisms. Hence, we used RNAi-mediated gene-silencing technique, to unravel the molecular mechanism behind the observed protective effect of the compound in protecting the brine shrimp larvae against V. parahaemolyticus (16, 18, 36). Our results indicate that increased survival in brine shrimp larvae pretreated with phloroglucinol is most likely due to a significant increase in Hsp70 production (Figures 3A–C). This is due to the fact that such protective effect of phloroglucinol was not observed if the adult Artemia females were injected with hsp70 dsRNA. Therefore, our result provides here new insight on the mode of Hsp70-inducing action of phloroglucinol, in which the initial generation of prooxidant activity by the compound plays a key role in Hsp70 induction and protection of brine shrimp larvae against V. parahaemolyticus infection.
As for the gnotobiotic A. franciscana, we found that phloroglucinol has a protective response against V. parahaemolyticus. This led to postulate us that phloroglucinol might be contributing in the protection of xenic brine shrimp larvae and other shrimp species (e.g., M. rosenbergii) against V. parahaemolyticus. However, it is hard to generalize from the results of gnotobiotic A. franciscana alone because, first under germ-associated condition, the physiological response (increased prooxidant activity and Hsp70 production) in the host due to exposure of tested compound could simply reflect the influence of various microbial communities (LPS or other cellular component) rather than a genuine biological effect of the compound (46, 47). Second, it may be possible that the protective effect of phloroglucinol might be different in another host–pathogen model (i.e., M. rosenbergii–V. parahaemolyticus). Therefore, to address this question, we have analyzed xenic brine shrimp larvae and Macrobrachium larvae response against phloroglucinol and V. parahaemolyticus. We found that xenic brine shrimp larvae pretreated with phloroglucinol displayed a significantly increased survival of larvae against pathogenic V. parahaemolyticus infection (Figure 4A), and in agreement with gnotobiotic A. franciscana results, we therefore suggest that phloroglucinol induces resistance in brine shrimp larvae in both gnotobiotic and germ-associated conditions against V. parahaemolyticus. Furthermore, in case of M. rosenbergii, we provided evidence that phloroglucinol exerted a protective effect against V. parahaemolyticus by the induction of Hsp70, which might be involved in eliciting immune response in the host (Figures 4C,E) (32, 48, 49).
In conclusion, the results presented here provide new insight on the mode of action of phloroglucinol, as a novel inducer of prooxidant activity and Hsp70. Our finding also provides strong evidences that phloroglucinol-mediated Hsp70 production seems to be responsible for generating resistance against a pathogenic V. parahaemolyticus MO904 strain that can cause AHPND in shrimps. The ability of phloroglucinol to boost immunity and induce resistance in A. franciscana and M. rosenbergii makes phloroglucinol a potent biocontrol agent that may be valuable to avert V. parahaemolyticus infection in other shrimp species. The results obtained add new information about the mode of action of phloroglucinol and advance our knowledge of this compound as a potential disease-mitigating agent.
Author Contributions
VK, KB, and PB conceived and designed the experiment. VK performed the experiments and drafted the figures and manuscript. VK, DN, EV, and GS made the laboratory analysis, statistics, and interpreted data. The manuscript is reviewed and edited by VK, KB, and PB. All the authors approved the final manuscript.
Conflict of Interest Statement
The authors declare that the research was conducted in the absence of any commercial or financial relationships that could be construed as a potential conflict of interest.
Acknowledgments
We thank Prof. Bruno Gomez-Gil of CIAD, A.C. Mazatlàn unit of Aquaculture, Mazatlàn, Sinaloa, Mexico, for providing V. parahaemolyticus MO904 AHPND strain. We would also like to acknowledge the technical assistance provided by Brigitte Van Moffaert and Anita De Haese. In addition, the authors also would like to thank Jorg De Smeyter for his technical support in M. rosenbergii broodstock management and larvae collection and Mathieu Wille and Spyros Nikolakakis for statistical advice.
Funding
The authors acknowledge financial support from the Research Foundation Flanders, FWO-Vlaanderen, Belgium (FWO13/PDO/005), GOA project entitled “Host microbial interaction in aquatic production” (BOF12/GOA/022), and the Belgian Science Policy Office (BELSPO) project entitled AquaStress, project number: IUAPVII/64/Sorgeloos. We highly acknowledge the Special Research Fund of Ghent University (BOF12/BAS/042) for procuring the chemiluminescence detection system. VK is supported by Netaji Subhas international doctoral fellowship from Indian Council of Agricultural Research (ICAR), New Delhi.
Supplementary Material
The Supplementary Material for this article can be found online at https://www.frontiersin.org/articles/10.3389/fimmu.2018.01091/full#supplementary-material.
References
1. Li P, Kinch LN, Ray A, Dalia AB, Cong Q, Nunan LM, et al. Acute hepatopancreatic necrosis disease-causing Vibrio parahaemolyticus strains maintain an antibacterial type VI secretion system with versatile effector repertoires. Appl Environ Microbiol (2017) 83(13):e00737–17. doi:10.1128/AEM.00737-17
2. Tran L, Nunan L, Redman RM, Mohney LL, Pantoja CR, Fitzsimmons K, et al. Determination of the infectious nature of the agent of acute hepatopancreatic necrosis syndrome affecting penaeid shrimp. Dis Aquat Organ (2013) 105:45–55. doi:10.3354/dao02621
3. Lee C-T, Chen I-T, Yang Y-T, Ko T-P, Huang Y-T, Huang J-Y, et al. The opportunistic marine pathogen Vibrio parahaemolyticus becomes virulent by acquiring a plasmid that expresses a deadly toxin. Proc Natl Acad Sci U S A (2015) 112:10798–803. doi:10.1073/pnas.1503129112
4. Nunan L, Lightner D, Pantoja C, Gomez-Jimenez S. Detection of acute hepatopancreatic necrosis disease (AHPND) in Mexico. Dis Aquat Organ (2014) 111:81–6. doi:10.3354/dao02776
5. FAO. Report of the FAO/MARD Technical Workshop on Early Mortality Syndrome (EMS) or Acute Hepatopancreatic Necrosis Syndrome (AHPNS) of Cultured Shrimp (under TCP/VIE/3304). Rome: FAO (Food and Agriculture Organization of the United Nations) (2013).
6. De Schryver P, Defoirdt T, Sorgeloos P. Early mortality syndrome outbreaks: a microbial management issue in shrimp farming? PLoS Pathog (2014) 10:10–1. doi:10.1371/journal.ppat.1003919
7. Lai HC, Ng TH, Ando M, Lee CT, Chen IT, Chuang JC, et al. Pathogenesis of acute hepatopancreatic necrosis disease (AHPND) in shrimp. Fish Shellfish Immunol (2015) 47:1006–14. doi:10.1016/j.fsi.2015.11.008
8. Lightner DV, Redman RM, Pantoja CR, Noble BI, Tran L. Early mortality syndrome affects shrimp in Asia. Global Aquacult Advocate (2012) 15(1):40.
9. Sirikharin R, Taengchaiyaphum S, Sanguanrut P, Chi TD, Mavichak R, Proespraiwong P, et al. Characterization and PCR detection of binary, pir-like toxins from Vibrio parahaemolyticus isolates that cause acute hepatopancreatic necrosis disease (AHPND) in shrimp. PLoS One (2015) 10:1–16. doi:10.1371/journal.pone.0126987
10. Hong X, Lu L, Xu D. Progress in research on acute hepatopancreatic necrosis disease (AHPND). Aquac Int (2016) 24:577–93. doi:10.1007/s10499-015-9948-x
11. Smith P. Antimicrobial resistance: the use of antimicrobials in the livestock sector. Rev Sci Tech (2008) 27:243–64. doi:10.20506/rst.27.1.1799
12. Baruah K, Norouzitallab P, Phong HPPD, Smagghe G, Bossier P. Enhanced resistance against Vibrio harveyi infection by carvacrol and its association with the induction of heat shock protein 72 in gnotobiotic Artemia franciscana. Cell Stress Chaperones (2017) 22:377–87. doi:10.1007/s12192-017-0775-z
13. Baruah K, Huy TT, Norouzitallab P, Niu Y, Gupta SK, De Schryver P, et al. Probing the protective mechanism of poly-ß-hydroxybutyrate against vibriosis by using gnotobiotic Artemia franciscana and Vibrio campbellii as host-pathogen model. Sci Rep (2015) 5:1–8. doi:10.1038/srep09427
14. Triantaphyllidis G, Abatzopoulos T, Sorgeloos P. Review of the biogeography of the genus Artemia (Crustacea, Anostraca). J Biogeogr (1998) 25:213–26. doi:10.1046/j.1365-2699.1998.252190.x
15. King AM, MacRae TH. The small heat shock protein p26 aids development of encysting Artemia embryos, prevents spontaneous diapause termination and protects against stress. PLoS One (2012) 7:e43723. doi:10.1371/journal.pone.0043723
16. King AM, Toxopeus J, Macrae TH. Functional differentiation of small heat shock proteins in diapause-destined Artemia embryos. FEBS J (2013) 280:4761–72. doi:10.1111/febs.12442
17. Baruah K, Duy Phong HPP, Norouzitallab P, Defoirdt T, Bossier P. The gnotobiotic brine shrimp (Artemia franciscana) model system reveals that the phenolic compound pyrogallol protects against infection through its prooxidant activity. Free Radic Biol Med (2015) 89:593–601. doi:10.1016/j.freeradbiomed.2015.10.397
18. Iryani MTM, MacRae TH, Panchakshari S, Tan J, Bossier P, Wahid MEA, et al. Knockdown of heat shock protein 70 (Hsp70) by RNAi reduces the tolerance of Artemia franciscana nauplii to heat and bacterial infection. J Exp Mar Bio Ecol (2017) 487:106–12. doi:10.1016/j.jembe.2016.12.004
19. Sirikharin R, Taengchaiyaphum S, Sritunyalucksana K, Thitamadee S, Flegel TW, Mavichak R, et al. A New and Improved PCR Method for Detection of AHPND Bacteria. Network of Aquaculture Centres in Asia-Pacific (NACA) (2014). p. 7–9. Available from: http://www.enaca.org/modules/news/article.php
20. Suong NT, Van Hao N, Van Sang N, Hung ND, Tinh NTN, Phuoc LH, et al. The impact of catecholamine sensing on the virulence of Vibrio parahaemolyticus causing acute hepatopancreatic necrosis disease (AHPND). Aquaculture (2017) 470:190–5. doi:10.1016/j.aquaculture.2016.12.030
21. Baruah K, Cam DTV, Dierckens K, Wille M, Defoirdt T, Sorgeloos P, et al. In vivo effects of single or combined N-acyl homoserine lactone quorum sensing signals on the performance of Macrobrachium rosenbergii larvae. Aquaculture (2009) 288:233–8. doi:10.1016/j.aquaculture.2008.11.034
22. Baruah K, Norouzitallab P, Roberts RJ, Sorgeloos P, Bossier P. A novel heat-shock protein inducer triggers heat shock protein 70 production and protects Artemia franciscana nauplii against abiotic stressors. Aquaculture (2012) 334–337:152–8. doi:10.1016/j.aquaculture.2011.12.015
23. Clegg JS, Jackson SA, Van Hoa N, Sorgeloos P. Thermal resistance, developmental rate and heat shock proteins in Artemia franciscana, from San Francisco Bay and southern Vietnam. J Exp Mar Bio Ecol (2000) 252:85–96. doi:10.1016/S0022-0981(00)00239-2
24. Bradford MM. A rapid and sensitive method for the quantitation of microgram quantities of protein utilizing the principle of protein-dye binding. Anal Biochem (1976) 72:248–54. doi:10.1016/0003-2697(76)90527-3
25. Grotto D, Santa Maria LD, Boeira S, Valentini J, Charão MF, Moro AM, et al. Rapid quantification of malondialdehyde in plasma by high performance liquid chromatography-visible detection. J Pharm Biomed Anal (2007) 43:619–24. doi:10.1016/j.jpba.2006.07.030
26. Marques A, François JM, Dhont J, Bossier P, Sorgeloos P. Influence of yeast quality on performance of gnotobiotically grown Artemia. J Exp Mar Bio Ecol (2004) 310:247–64. doi:10.1016/j.jembe.2004.04.009
27. Sorgeloos P, Bossuyt E, Laviña E, Baeza-Mesa M, Persoone G. Decapsulation of Artemia cysts: a simple technique for the improvement of the use of brine shrimp in aquaculture. Aquaculture (1977) 12:311–5. doi:10.1016/0044-8486(77)90209-5
28. Nhan DT, Cam DTV, Wille M, Defoirdt T, Bossier P, Sorgeloos P. Quorum quenching bacteria protect Macrobrachium rosenbergii larvae from Vibrio harveyi infection. J Appl Microbiol (2010) 109:1007–16. doi:10.1111/j.1365-2672.2010.04728.x
29. Rahman MM, Wille M, Cavalli RO, Sorgeloos P, Clegg JS. Induced thermotolerance and stress resistance in larvae of the freshwater prawn, Macrobrachium rosenbergii (de Man, 1879). Aquaculture (2004) 230:569–79. doi:10.1016/j.aquaculture.2003.10.010
30. Baruah K, Norouzitallab P, Shihao L, Sorgeloos P, Bossier P. Feeding truncated heat shock protein 70s protect Artemia franciscana against virulent Vibrio campbellii challenge. Fish Shellfish Immunol (2013) 34:183–91. doi:10.1016/j.fsi.2012.10.025
31. Baruah K, Ranjan J, Sorgeloos P, MacRae TH, Bossier P. Priming the prophenoloxidase system of Artemia franciscana by heat shock proteins protects against Vibrio campbellii challenge. Fish Shellfish Immunol (2011) 31:134–41. doi:10.1016/j.fsi.2011.04.008
32. Niu Y, Norouzitallab P, Baruah K, Dong S, Bossier P. A plant-based heat shock protein inducing compound modulates host–pathogen interactions between Artemia franciscana and Vibrio campbellii. Aquaculture (2014) 430:120–7. doi:10.1016/j.aquaculture.2014.04.001
33. Baruah K, Norouzitallab P, Linayati L, Sorgeloos P, Bossier P. Reactive oxygen species generated by a heat shock protein (Hsp) inducing product contributes to Hsp70 production and Hsp70-mediated protective immunity in Artemia franciscana against pathogenic vibrios. Dev Comp Immunol (2014) 46:470–9. doi:10.1016/j.dci.2014.06.004
34. Hannon GJ, Rossi JJ. Unlocking the potential of the human genome with RNA interference. Nature (2004) 431:371–8. doi:10.1038/nature02870
35. Mao Y-B, Cai W-J, Wang J-W, Hong G-J, Tao X-Y, Wang L-J, et al. Silencing a cotton bollworm P450 monooxygenase gene by plant-mediated RNAi impairs larval tolerance of gossypol. Nat Biotechnol (2007) 25:1307–13. doi:10.1038/nbt1352
36. Junprung W, Supungul P, Tassanakajon A. HSP70 and HSP90 are involved in shrimp Penaeus vannamei tolerance to AHPND-causing strain of Vibrio parahaemolyticus after non-lethal heat shock. Fish Shellfish Immunol (2017) 60:237–46. doi:10.1016/j.fsi.2016.11.049
37. Choi M, Stevens AM, Smith SA, Taylor DP, Kuhn DD. Strain and dose infectivity of Vibrio parahaemolyticus: the causative agent of early mortality syndrome in shrimp. Aquac Res (2017) 48:3719–27. doi:10.1111/are.13197
38. Han JE, Tang KFJ, Tran LH, Lightner DV. Photorhabdus insect-related (Pir) toxin-like genes in a plasmid of Vibrio parahaemolyticus, the causative agent of acute hepatopancreatic necrosis disease (AHPND) of shrimp. Dis Aquat Organ (2015) 113:33–40. doi:10.3354/dao02830
39. Wu G, Harris MK, Guo J-Y, Wan F-H. Response of multiple generations of beet armyworm, Spodoptera exigua (Hübner), feeding on transgenic Bt cotton. J Appl Entomol (2009) 133:90–100. doi:10.1111/j.1439-0418.2008.01328.x
40. Sung YY, Dhaene T, Defoirdt T, Boon N, MacRae TH, Sorgeloos P, et al. Ingestion of bacteria overproducing DnaK attenuates Vibrio infection of Artemia franciscana larvae. Cell Stress Chaperones (2009) 14:603–9. doi:10.1007/s12192-009-0112-2
41. Baruah K, Ranjan J, Sorgeloos P, Bossier P. Efficacy of heterologous and homologous heat shock protein 70s as protective agents to Artemia franciscana challenged with Vibrio campbellii. Fish Shellfish Immunol (2010) 29:733–9. doi:10.1016/j.fsi.2010.07.011
42. Ryu J, Zhang R, Hong BH, Yang EJ, Kang KA, Choi M, et al. Phloroglucinol attenuates motor functional deficits in an animal model of Parkinson’s disease by enhancing Nrf2 activity. PLoS One (2013) 8:e71178. doi:10.1371/journal.pone.0071178
43. Burt S. Essential oils: their antibacterial properties and potential applications in foods—a review. Int J Food Microbiol (2004) 94:223–53. doi:10.1016/j.ijfoodmicro.2004.03.022
44. Ozcan T, Akpinar-Bayizit A, Yilmaz-Ersan L, Delikanli B. Phenolics in human health. Int J Chem Eng Appl (2014) 5:393–6. doi:10.7763/IJCEA.2014.V5.416
45. Roberts RJ, Agius C, Saliba C, Bossier P, Sung YY. Heat shock proteins (chaperones) in fish and shellfish and their potential role in relation to fish health: a review. J Fish Dis (2010) 33:789–801. doi:10.1111/j.1365-2761.2010.01183.x
46. Sung YY, MacRae TH, Sorgeloos P, Bossier P. Stress response for disease control in aquaculture. Rev Aquacult (2011) 3:120–37. doi:10.1111/j.1753-5131.2011.01049.x
47. Kojima K, Musch MW, Ropeleski MJ, Boone DL, Ma A, Chang EB. E. coli lipopolysaccharide induces heat shock protein 25 in intestinal epithelial cells through MAP kinase activation. Am J Physiol Gastrointest Liver Physiol (2004) 286:G645–52. doi:10.1152/ajpgi.00080.2003
48. Liu J, Yang W-J, Zhu X-J, Karouna-Renier NK, Rao RK. Molecular cloning and expression of two HSP70 genes in the prawn, Macrobrachium rosenbergii. Cell Stress Chaperones (2004) 9:313–23. doi:10.1379/CSC-40R.1
49. Xiu Y, Feng J, Lu W, Liu D, Wu T, Zhu H, et al. Identification of a novel cognate cytosolic Hsp70 gene (MnHsc70-2) from oriental river prawn Macrobrachium nipponense and comparison of its expressions with the first cognate Hsc70 (MnHsc70-1) under different stresses. Cell Stress Chaperones (2014) 19:949–61. doi:10.1007/s12192-014-0519-2
Keywords: gnotobiotic Artemia, Vibrio parahaemolyticus, phloroglucinol, heat shock protein 70, Macrobrachium rosenbergii
Citation: Kumar V, Baruah K, Nguyen DV, Smagghe G, Vossen E and Bossier P (2018) Phloroglucinol-Mediated Hsp70 Production in Crustaceans: Protection against Vibrio parahaemolyticus in Artemia franciscana and Macrobrachium rosenbergii. Front. Immunol. 9:1091. doi: 10.3389/fimmu.2018.01091
Received: 06 February 2018; Accepted: 01 May 2018;
Published: 22 May 2018
Edited by:
Chu-Fang Lo, Center for Shrimp Disease Control and Genetic Improvement, TaiwanReviewed by:
Anchalee Tassanakajon, Chulalongkorn University, ThailandIddya Karunasagar, Nitte University, India
Annalisa Pinsino, Istituto di biomedicina e di immunologia molecolare Alberto Monroy (IBIM), Italy
Copyright: © 2018 Kumar, Baruah, Nguyen, Smagghe, Vossen and Bossier. This is an open-access article distributed under the terms of the Creative Commons Attribution License (CC BY). The use, distribution or reproduction in other forums is permitted, provided the original author(s) and the copyright owner are credited and that the original publication in this journal is cited, in accordance with accepted academic practice. No use, distribution or reproduction is permitted which does not comply with these terms.
*Correspondence: Kartik Baruah, a2FydGlrLmJhcnVhaEBzbHUuc2U=
†Present address: Kartik Baruah, Department of Animal Nutrition and Management, Faculty of Veterinary Medicine and Animal Sciences, Swedish University of Agricultural Sciences, Uppsala, Sweden