- 1College of Food Engineering and Nutritional Science, Shaanxi Normal University, Xi’an, China
- 2College of Food Science and Technology, Hainan University, Haikou, China
Drugs used to treat liver diseases have serious side effects; it is important to search for safe functional foods with hepatoprotective functions and few side effects. In this study, potential hepatoprotective effects of goats’ milk and cows’ milk on mice with CCl4-induced acute hepatic injury were evaluated. We also elucidated the role of goats’ and cows’ milk on the regulation of CCl4-induced gut microbiota imbalance. In mice with liver damage induced by CCl4, administration of goats’ milk for 7 days prior to injection of CCl4 had beneficial effects on the indicators of liver damage within 1 day: the area of liver necrosis was small; activity of alanine transaminase (ALT) and aspartate transaminase (AST) and expression of the genes CYP2E1 and TNF-α were lower than that of model group of mice. By 7 days after CCl4 injection, there were no significant differences in liver damage indicators (ALT, AST, malondialdehyde, superoxide dismutase, and glutathione) between the goats’ milk group, which continued to receive goats’ milk, and the untreated control group of mice showing that goats’ milk continued to protect against liver damage. Throughout the entire experiment, the community of gut microbes from mice in the goats’ milk treatment was more similar to the untreated control group than to the cows’ milk group and the model group, indicating that intake of goats’ milk prior and post-CCl4 injection effectively prevented and alleviated the intestinal microbial disorder that caused by CCl4 in mice. Our research suggests that goats’ milk could be developed as a potential functional food to prevent/protect against liver injury.
Introduction
Liver disease is a major cause of mortality and morbidity worldwide. Liver function can be impacted by gut function and vice versa (1). Following liver injury, the secretion of bile can be altered by the gut–liver axis; this reduces blood supply and peristalsis in the intestine, resulting in disruption of the intestinal mucosa and disturbance of the gut microbiota (2). Gut secretions such as hormones, inflammatory mediators, and digestive absorption products also affect liver function directly (3). The microbiome of the gut, especially in the large intestine, comprised numerous microorganisms that play an important role in food digestion and also in other important processes within the organism (4). Evidence suggests that some gut-derived microbial components activate the inflammatory cascade of immune cells in the liver and regulate the function and response of liver parenchymal cells (5). Imbalance in the gut microbiota can result in severe systemic infections, and alterations in microbial composition and abundance are critically influential factors in hepatic dysfunction (6). Severity of liver disease can be directly related to the severity of dysbiosis of gut microbiota. Sustaining a dynamic balance in gut microbiota could maintain a healthy state, while an imbalance in gut microbiota may promote liver injury. Thus, it is important to fully elucidate the relationship between gut microbiota and acute liver injury that could lead to chronic liver disease.
Some drugs that are used for the treatment of liver diseases have side effects (7). Therefore, it is essential to search for potential functional foods that prevent/protect against liver injury but have no (or few) side effects. Milk is rich in nutrients, especially goats’ milk, which is easier to digest and absorb than cows’ milk due to its smaller casein micelles and fat globules, and the high levels of medium- and short-chain fatty acids (SCFAs) (8). Consumption of goats’ milk can protect cells from injury and has been used as a treatment for hepatic adipose infiltration in children (9). This indicates that goats’ milk may have potential hepatoprotective qualities that should be further investigated.
Carbon tetrachloride (CCl4)-induced hepatic injury in animal models has been used to explore the potential for natural compounds to protect the liver against damage (10). CCl4 transforms into a trichloromethyl radical that reacts with molecular oxygen to form a highly toxic trichloromethyl peroxyl radical; this free radical disrupts polyunsaturated fatty acids in membrane lipids causing the membrane structures to rupture, leading to the disruption of cell energy processes and the synthesis of protein, which leads to lipid peroxidation in liver cells (11). Furthermore, the community structure of the gut microbiota is altered following oral administration or intraperitoneal injection of CCl4 (12, 13). This means that CCl4 directly induces hepatic injury, while simultaneously modulating the gut microbiota. Mazagova et al. and Wang et al. were the first to demonstrate the protective role of gut microbiota against CCl4-induced chronic liver injury in germ-free mice (14); they demonstrated that commensal microbiota were important for maintenance of liver health. Subsequent studies have also proven the beneficial effect of gut microbiota on hepatic function (15). Changes in the gut microbiota could result in changes in liver function. An important component of the gut microbiota is probiotics (e.g., Lactobacillus, Bifidobacterium, Clostridium, and Saccharomyces species), which are also known to alleviate the symptoms of CCl4-induced hepatic injury (12, 16). Previous study showed that goats’ milk had beneficial effects on rats with hepatotoxicity induced by the drugs isoniazid, rifampicin, and pyrazinamide (17). In this study, the preventative and protective effects of goats’ milk and cows’ milk, administered prior to and post the use of on CCl4 to induce acute liver injury mice, were investigated. We also used metagenomics-wide high-throughput sequencing of the V3–V4 regions of the 16S rRNA gene to study the beneficial roles of the milk on the regulation of CCl4-induced gut microbiota imbalance. Our research suggests that goats’ milk could be developed as a potential functional food to prevent/protect against liver injury and provides the preliminary data necessary for future in-depth studies on the relationships amongst goat milk, gut microbiota, and acute liver injury.
Materials and Methods
Mice and Experimental Protocol
C57BL/6 male mice (12 weeks old) were allowed to acclimate to the animal facility for 1 week before starting the experiment. Mice were maintained in individual ventilated cages in climate-controlled rooms (12 h light/dark cycles; 20–24°C; 45–55%RH; individual filter-sterilized air) with access to food and water ad libitum. All food, water, and experimental equipment used were sterilized or sanitized. The animals received humane care from trained staff, and all experiments were performed in accordance with the guidelines of the Shaanxi Normal University Scientific Ethics Committee.
After 1 week of acclimatization, the mice were divided randomly into eight groups, each comprised six mice (48 in total) and fed the same diet throughout the experimental period. Four groups were used to evaluate the preventative effects of milk consumption for 7 days prior to challenge with CCl4, and the other four groups to evaluate the protective effects of continued milk consumption in the 7 days following challenge with CCl4. The eight groups were set up at the same time and run alongside each other; the experimental design is fully described in Figure 1A. For each part of the experiment (preventive effects and protective effects), there was an untreated control group of mice (U; receiving only food and water), a model group (M; receiving CCl4 on day 7), a goats’ milk group (G; receiving CCl4 on day 7 and goats’ milk daily throughout), and a cows’ milk group (C; receiving CCl4 on day 7 and cows’ milk daily throughout). On day 8 of the experiment (1 day after injection of CCl4 in the M, G, and C groups), the first four groups of mice, designated as UPrev, MPrev, GPrev, and CPrev, were sacrificed and assessed for the preventive role of milk on liver injury prior to challenge with CCl4. After a further 7 days, the remaining groups were sacrificed and assessed for the protective role of continued administration of milk against liver injury following challenge with CCl4 and these were designated as UProt, MProt, GProt, and CProt, respectively.
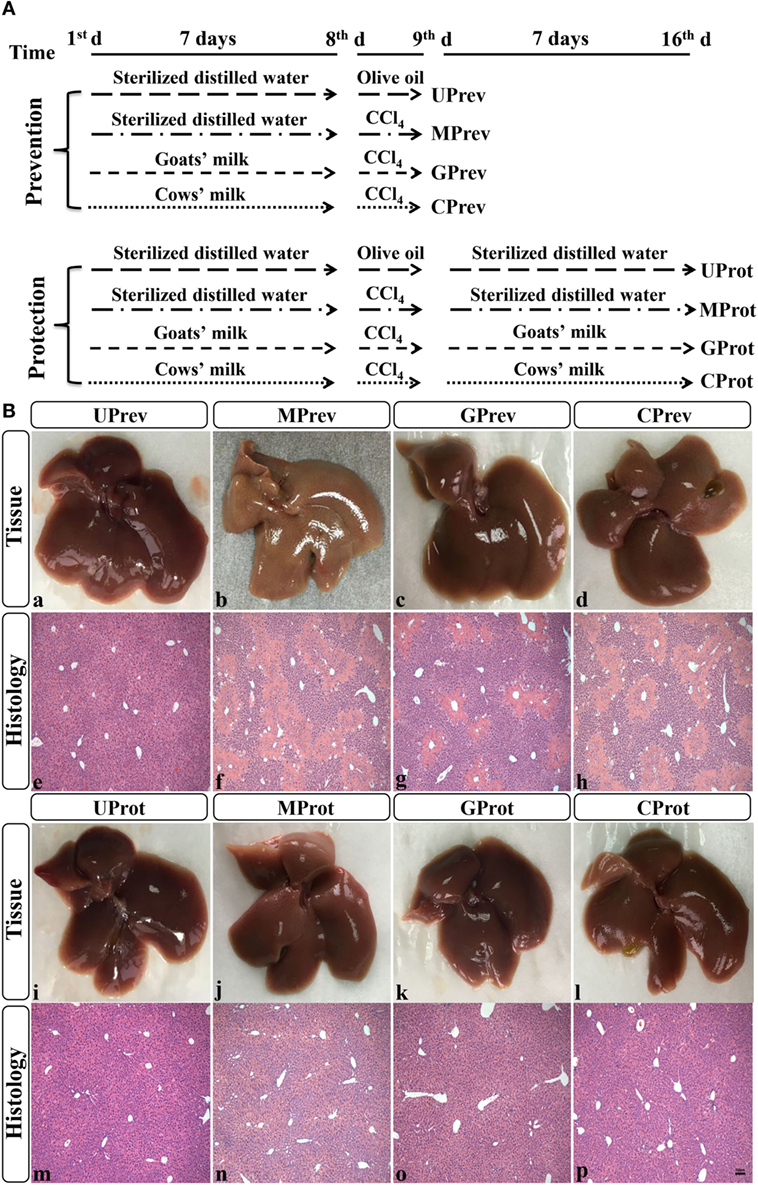
Figure 1. Experimental design (A) and histological analysis of livers from the eight different treatment groups of mice (see Figure 1A) (B) (1). Liver samples taken 1 day after administration of CCl4 (to determine preventive effects of milk consumption on induced liver injury) from untreated control mice UPrev (a), model mice MPrev (b), mice receiving goats’ milk GPrev (c), and mice receiving cows’ milk CPrev (d) and 8 days after administration of CCl4 (to determine protective effects of continued milk consumption on induced liver injury) from untreated control mice UProt (i), model mice MProt (j), mice receiving goats’ milk GProt (k), and mice receiving cows’ milk CProt (l) (2). Liver histology [hematoxylin and eosin (H&E)] of UPrev (e), MPrev (f), GPrev (g), CPrev (h), UProt (m), MProt (n), GProt (o), and CProt (p) mice. Scale bar: 100 µm for e–h and m–p.
Evaluating the Preventive Role of Milk on CCl4-Induced Liver Damage
From days 1–7, mice in treatment groups UPrev and MPrev received 10 mL/kg body weight sterilized distilled water by intra-gastric administration twice a day; mice in treatment groups GPrev and CPrev received 10 mL/kg body weight of commercial UHT-goats’ milk and 10 mL/kg body weight of commercial UHT-cows’ milk, respectively, again twice a day by intra-gastric administration.
On the 8th day, mice in the UPrev group received an intraperitoneal injection of 1 mL/kg body weight olive oil; mice in groups MPrev, GPrev, and CPrev received an intraperitoneal injection of 1 mL/kg body weight CCl4 (1:4, v/v, in olive oil). After 24 h (day 9), all mice were anesthetized with isoflurane gas, and under aseptic conditions, laparotomies performed via a midline incision and samples collected from the colon for gut microbiota evaluation. Blood samples were taken and the livers harvested, frozen in liquid nitrogen, and stored at −80°C for further analysis.
Evaluating the Protective Effects of Milk on CCl4-Induced Liver Damage
Mice in the other four groups were used to evaluate the protective effects of continued milk consumption and were treated the same as the mice in the preventive experiment, until day 9 but then, rather than being sacrificed, UProt, MProt, GProt, and CProt mice continued to receive the sterilized distilled water, goats’ milk, or cows’ milk for a further 7 days. On the 16th day, all remaining mice were sacrificed and samples were collected as described earlier.
Histological Analysis
Samples from the left lobe of the liver were fixed in 4% paraformaldehyde (4°C), dehydrated in graded alcohol, and embedded in paraffin wax. Embedded tissues were cut into 4 µm thick sections and stained with hematoxylin and eosin for morphological analysis.
Alanine Transaminase (ALT) and Aspartate Transaminase (AST) Analyses
ALT and AST are mainly distributed in liver cells, and when the membranes of liver cells are damaged or undergo necrosis, these enzymes enter the serum in large quantities. Determination of serum or plasma enzyme activity can sensitively reflect the extent of liver injury (18). Serum was collected by centrifugation (2,000 × g for 15 min, 4°C). ALT and AST levels were determined using the commercial ALT Activity Assay Kit (MAK052) and the AST Activity Assay Kit (MAK055) according to the manufacturer’s instructions (Sigma-Aldrich, Inc.). Each sample was assayed three times.
Malondialdehyde (MDA), Superoxide Dismutase (SOD), and Glutathione (GSH) Analyses
Samples of liver were weighed and homogenized for 1 min in phosphate buffer (pH 7.4). The homogenates were centrifuged at 2,000 × g and 4°C for 20 min, and the supernatant was collected carefully. MDA, SOD, and GSH concentrations in the supernatants were determined using mouse MDA, SOD, and GSH ELISA kits according to the manufacturer’s instructions (MDA, ml931407; SOD, ml643059; GSH, ml643115; Shanghai Enzyme-linked Biotechnology Co., Ltd). Each sample was assayed three times.
Quantitative RT-PCR Assay
We measured the mRNA expression levels of the genes for the key enzymes CYP2E1, which influences the activation of CCl4 in vivo, and TNF-α, which is a pro-inflammatory cytokine. Total RNA was extracted from 50 mg samples of liver tissue using TRIzol® according to the manufacturer’s protocol. Sample RNA was transcribed into cDNA using the SuperScript III RT-PCR kit, and then quantitative PCR was performed in a fluorescent temperature cycler with SYBR Green and specific primers for each of the genes. Each reaction was repeated independently at least three times. Glyceraldehyde-3-phosphate dehydrogenase (GAPDH) was amplified as an internal control. The primer sequences used for each gene are listed in Table 1. pcr array data were calculated using the 2−(ΔΔCt) method.
Metagenomic DNA Extraction and High-Throughput Sequencing of V3–V4 Regions of 16S rRNA Gene
Extraction and analysis of metagenomic DNA from the microbiome present in colon samples was performed using our previously described methods (19). The V3–V4 regions of the 16S rRNA gene were amplified using specific primers (forward primer 338F and reverse primer 806R; Table 1) (20). PCR products were purified using the Qiagen Gel Extraction Kit. A TruSeq® DNA PCR-Free Sample Preparation Kit was used to construct the DNA library. The DNA library was quantified using a Qubit Fluorometer and an Agilent Bioanalyzer 2100; sequencing was done using an Illumina HiSeq 2500 System.
Bioinformatics Analysis of Sequence Data
The 250 bp paired-end reads were generated through sequencing; the bioinformatics analysis we used for these sequence data has been fully described previously (8, 19, 20). Briefly, Qiime pipeline (v1.7.0) was used to filter out low-quality tags. Uparse software (v7.0.1001) was used to cluster effective tags to the operational taxonomic unit (OTU) based on 97% sequence similarity of sequences. Representative OTUs with the high frequency of occurrence were selected and annotated for taxonomic information using the Mothur method and SSUrRNA database in SILVA with a threshold of 0.8–1, to obtain community compositions at different taxonomic levels (phylum and genus). Multiple sequence alignments were performed using MUSCLE software to study phylogenetic relationships amongst different OTUs and the predominant bacteria in the gut microbiota.
Alpha diversity was determined as a measure of the diversity of the microbial community within a sample; Shannon index was calculated by Qiime to measure the alpha diversity of the gut microbiota (8, 19, 20). Beta diversity is a comparative analysis of microbial community composition and complexity between sample pairs (8, 19, 20); Weighted UniFrac distances between microbial communities from the colon samples were calculated by Qiime to study beta diversity metrics (8, 19, 20). Based on the UniFrac distances between gut microbial communities, the samples located in the first principal coordinates analysis at different time points were used to fit the curve of changes in microbial community structure and to dynamically analyze differences between the treatment groups in their microbial community structure; this allowed us to identify groups with community structures closest to the untreated control group and thus reveal the treatments providing the best prevention and mitigation effects in the CCl4-induced mice. Phylogenetic Investigation of Communities by Reconstruction of Unobserved States (PICRUSt) was used to predict the function of the 16S rRNA gene based on the sequencing data (21). Z scores for each of the specific metabolic pathway in pairwise group were calculated using R software. Relationships amongst goats’ milk, different genera, metabolisms, and liver damage indicators were calculated using the Spearman Rank Correlation Coefficient and visualized as a network in Cytoscape software (v3.2.1). R software and GraphPad Prism were used for plotting in this study.
Statistical Analysis
Analysis of differences between two or more groups was performed using the non-parametric Wilcoxon rank-sum test and the Kruskal–Wallis H test. The data and error bars are presented as mean ± standard error of the mean. Statistical significance was assumed when the P value was less than 0.05. All statistical analysis was performed in R software.
Nucleotide Sequence Accession Number
The high-throughput sequencing data reported in this study were deposited in the NCBI database, and the accession number is PRJNA437795.
Results
Histological Analysis
Preventive Role of Milk on CCl4-Induced Liver Damage
Liver tissue appeared reddish brown and shiny in the UPrev mice 24 h after olive oil injection (Figure 1B-a); the hepatic lobule structure was clear and complete, the liver cells were large, the nuclei were round and located in the middle of the cell, and there was no degeneration, necrosis, or infiltration of inflammatory cells (Figure 1B-e). However, in the MPrev mice, the liver tissue was pale and infiltrated with white spots that indicated injury inside the hepatic cells (Figure 1B-b); large areas of necrosis were apparent in the central parts of the hepatic lobules, and the necrotic area was characterized by fragmentation, dissolution, and disappearance of the nucleus. There was a necrotic band between the two central veins, and normal liver tissue between the necrotic areas (Figure 1B-f). Liver tissues from the GPrev mice (Figure 1B-c) were slightly paler than tissue from the UPrev mice but with fewer scattered white spots compared with the MPrev mice. There was a little necrosis of the hepatic lobule in liver tissue from GPrev mice (Figure 1B-g); the necrotic area was intermediate in size between the UPrev mice and the CPrev mice (Figure 1B-d,h). This indicated that GPrev mice had the least liver injury following CCl4 challenge, showing that goats’ milk had a protective effect against acute liver injury.
Protective Role of Milk on CCl4-Induced Liver Damage
Eight days after CCl4 injection, the liver tissue and hepatic lobule structure of MProt mice (Figure 1B-j,n), GProt mice (Figure 1B-k,o), and CProt mice (Figure 1B-l,p) were similar to those from the UProt mice (Figure 1B-i,m). The necrotic area of liver tissue disappeared, and the structure of the liver lobule returned to normal, indicating that the tissue had been repaired.
ALT and AST Analyses
Preventive Role of Milk on CCl4-Induced Liver Damage
Twenty-four hours after administration of CCl4, ALT levels in serum from MPrev and GPrev mice were 72 and 35 times higher than that of UPrev mice (Figure 2A). AST levels in serum from MPrev and GPrev mice were 17 and 9 times higher than that of UPrev mice (Figure 2B). All differences between the groups presented above were statistically significant (P < 0.01).
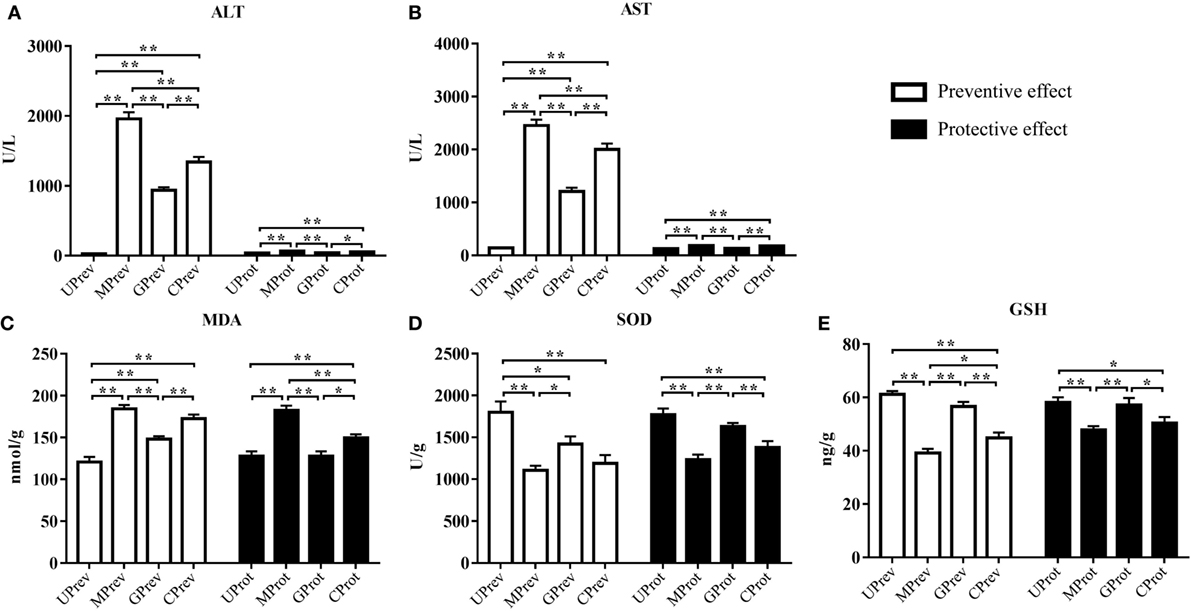
Figure 2. Levels of five biochemical indices in samples from mice in the eight different treatment groups (see Figure 1A). Samples were taken 1 day after administration of CCl4 (to determine preventive effects of milk consumption on induced liver injury) from: untreated control mice (UPrev), model mice (MPrev), mice receiving goats’ milk (GPrev), and mice receiving cows’ milk (CPrev) and 8 days after administration of CCl4 (to determine protective effects of continued milk consumption on induced liver injury) from untreated control mice (UProt), model mice (MProt), mice receiving goats’ milk (GProt), and mice receiving cows’ milk (CProt). The indices were as follows: alanine transaminase (ALT) (A), aspartate transaminase (AST) (B), malondialdehyde (MDA) (C), superoxide dismutase (SOD) (D), and glutathione (GSH) (E). The data and error bars are presented as mean ± SEM. *P < 0.05; **P < 0.01.
Protective Role of Milk on CCl4-Induced Liver Damage
Eight days after injection of CCl4, ALT and AST levels in serum from MProt mice were 1.8 and 1.4 times higher than in serum from UProt mice (P < 0.01), respectively. However, there were no significant differences in ALT and AST levels between GProt and UProt mice (P > 0.05). This shows that peak liver injury occurred 24 h after injection of CCl4 and that the degree of liver damage in the goats’ milk group of mice was significantly lower than in the model group and the cows’ milk group, which paralleled the histopathological findings. This indicates that goats’ milk protects against acute CCl4-induced liver damage and could accelerate the recovery of damaged tissue.
MDA, SOD, and GSH Analyses
Preventive Role of Milk on CCl4-Induced Liver Damage
Twenty-four hours after injection of CCl4, MDA levels in the liver tissues of MPrev mice were significantly higher than in the liver tissues of UPrev and GPrev mice (P < 0.01; Figure 2C); MDA levels were significantly higher in the liver tissues of GPrev mice compared with UPrev mice (P < 0.01; Figure 2C). SOD and GSH levels in liver tissues of MPrev mice were significantly lower than those in UPrev mice (P < 0.01; Figures 2D,E), but there was no significant difference in GSH levels in GPrev mice compared with UPrev mice (P > 0.05).
Protective Role of Milk on CCl4-Induced Liver Damage
MDA levels in the liver tissues of MProt mice were significantly higher than in the liver tissues of UProt and GProt mice (P < 0.01; Figure 2C). There was no significant difference between MDA levels in GProt and UProt mice (P > 0.05). SOD and GSH levels were significantly lower in MProt mice than in UProt mice (P < 0.01; Figures 2D,E), but there were no significant differences in SOD and GSH levels between GProt mice and UProt mice (P > 0.05).
Quantitative RT-PCR Assay of CYP2E1 and TNF-α
Preventive Role of Milk on CCl4-Induced Liver Damage
Twenty-four hours after the administration of CCl4, the relative expression levels of CYP2E1 were 2.2, 1.3, and 1.8 times higher in the liver tissues of MPrev, GPrev, and CPrev mice, respectively, than those in the liver tissues of UPrev mice (P < 0.05; Figure 3A). The relative mRNA expression levels of TNF-α in liver tissues of MPrev, GPrev, and CPrev mice were 3.5, 1.7, and 3.0 times higher than those in UPrev mice, respectively (P < 0.05, Figure 3B).
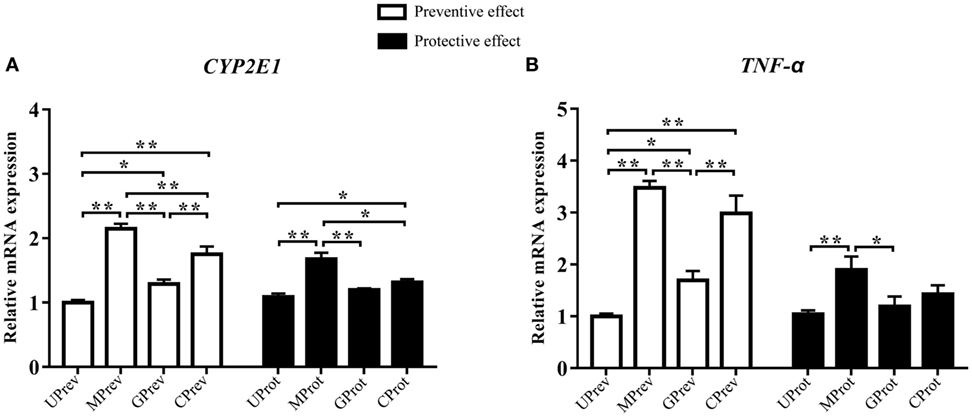
Figure 3. Relative expression levels of two key genes CYP2E1 (A) and TNF-α (B) in liver tissue from mice in the different treatment groups (see Figure 1A). Samples were taken 1 day after administration of CCl4 (to determine preventive effects of milk consumption on induced liver injury) from: untreated control mice (UPrev), model mice (MPrev), mice receiving goats’ milk (GPrev), and mice receiving cows’ milk (CPrev) and 8 days after administration of CCl4 (to determine protective effects of continued milk consumption on induced liver injury) from untreated control mice (UProt), model mice (MProt), mice receiving goats’ milk (GProt), and mice receiving cows’ milk (CProt). The data and error bars are presented as mean ± SEM. *P < 0.05; **P < 0.01.
Protective Role of Milk on CCl4-Induced Liver Damage
Eight days after CCl4 administration, the relative mRNA expression levels of CYP2E1 in liver tissues of MProt mice were 1.5 times higher than those in UProt mice (P < 0.01), while there were no significant differences in expression between GProt and UProt mice (P > 0.05). The results suggest that mRNA expression levels of CYP2E1 tend to normalize following goats’ milk intervention and that expression was repressed by goats’ milk. The relative mRNA expression levels of TNF-α in the liver tissue of MProt mice were 1.8 times higher than those in UProt mice (P < 0.01), while expression levels in GProt mice were only 1.1 times higher than those in UProt mice (P > 0.05). The results showed that the expression of TNF-α tended to normalize in mice following goats’ milk intervention.
Diversity of Gut Microbiota from Different Mice Treatment Groups
Shannon index was used to determine the alpha diversity of the microbiota in samples. The average value of this index for the microbiota was significantly higher in the UPrev and GPrev groups than in the MPrev group (P < 0.05; Figure 4A), indicating that the alpha diversity of the microbiota in UPrev and GPrev was higher than that in MPrev. However, the difference amongst the alpha diversity of the gut microbiota of the four groups at day 16 was not significantly different (P > 0.05). The alpha diversities of the microbiota in the MProt, GProt, and CProt groups of mice were all higher than in the values for the MPrev, GPrev, and CPrev groups.
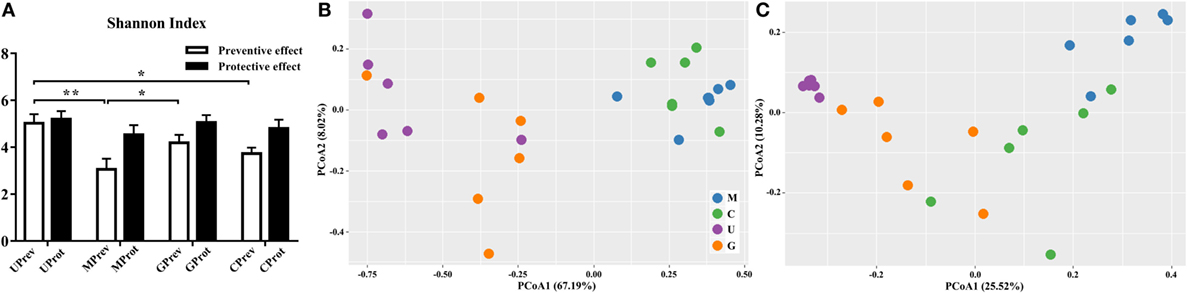
Figure 4. Diversity of the gut microbiota. (A) Alpha diversity of the gut microbiota of U, M, G, and C mice as measured by the Shannon index and (B,C) beta diversity analyzed using principal components analysis (PCoA) score plots based on weighted UniFrac metrics for the gut microbiota from the eight treatment groups of mice: (B) samples taken 1 day after the administration of CCl4 (to determine preventive effects of milk consumption on induced liver injury) from untreated control mice (UPrev), model mice (MPrev), mice receiving goats’ milk (GPrev), and mice receiving cows’ milk (CPrev) and (C) samples taken 8 days after administration of CCl4 (to determine protective effects of continued milk consumption on induced liver injury) from untreated control mice (UProt), model mice (MProt), mice receiving goats’ milk (GProt), and mice receiving cows’ milk (CProt). The data and error bars are presented as mean ± SEM. *P < 0.05; **P < 0.01.
There were significant differences in the beta diversity of gut microbiota between the UPrev and MPrev groups (P < 0.05), the GPrev and MPrev groups (P < 0.05), the UPrev and CPrev groups (P < 0.05), and the GPrev and CPrev groups (P < 0.05); no significant differences in beta diversity were detected between the UPrev and GPrev groups (P > 0.05), or between the CPrev and MPrev groups (P > 0.05). The results were the same for the UProt, MProt, GProt, and CProt data. Principal components analysis showed that the Weighted UniFrac distances between the bacterial communities in the UPrev and GPrev groups were small (Figure 4B). The CProt group tended to be closer to the UProt and GProt groups; the MPrev and MProt groups remained distant from the other groups throughout the experimental period (Figure 4C). Bioinformatics analysis showed that microbial communities of mice in the goats’ milk groups and the untreated control groups were more similar to each other than to communities from mice in the cows’ milk and model groups; this was both in their proximity and their trends in variation. These results indicate that intake of goats’ milk effectively prevented and alleviated intestinal microbial disorder caused by CCl4 in mice.
Taxonomic Annotation of Gut Microbiota From Different Mice Treatment Groups
Preventive Role of Milk on CCl4-Induced Liver Damage
At the phylum level, Firmicutes, Verrucomicrobia, Actinobacteria, Bacteroidetes, and Proteobacteria were detected in all gut microbiota samples (Figures 5A,B); Firmicutes was the predominant phylum, representing more than 43.29% of all bacteria present. Relative abundance of Firmicutes was significantly lower in the MPrev group than in the UPrev and GPrev groups (P < 0.05), but Bacteroidetes were significantly more abundant in the MPrev group than in the UPrev and GPrev groups (P < 0.05). At the genus level, significant differences in abundance of different microbial genera were found between the MPrev group and the UPrev group. The genera Enterococcus, Enterorhabdus, Oscillibacter, and Oscillospira were significantly more abundant in MPrev mice than in UPrev mice (P < 0.05), while the genera Adlercreutzia, Flavonifractor, Lactobacillus, and Turicibacter were more abundant in UPrev mice than in MPrev mice (P < 0.05; Figure 6).
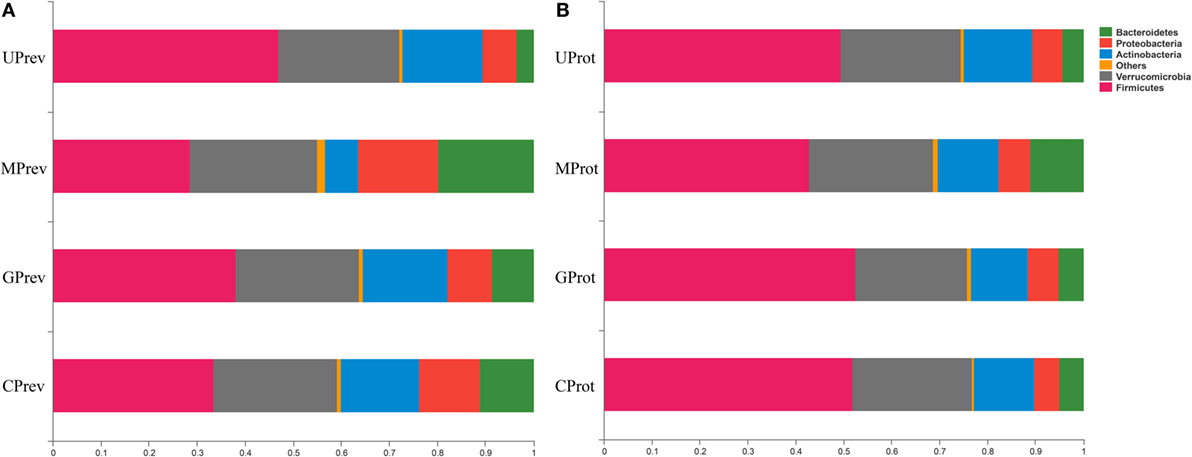
Figure 5. Relative abundance of different phyla in the gut microbiota of mice from the eight treatment groups: (A) samples taken 1 day after administration of CCl4 (to determine preventive effects of milk consumption on induced liver injury) from: untreated control mice (UPrev), model mice (MPrev), mice receiving goats’ milk (GPrev), and mice receiving cows’ milk (CPrev) and (B) samples taken 8 days after administration of CCl4 (to determine protective effects of continued milk consumption on induced liver injury) from untreated control mice (UProt), model mice (MProt), mice receiving goats’ milk (GProt), and mice receiving cows’ milk (CProt).
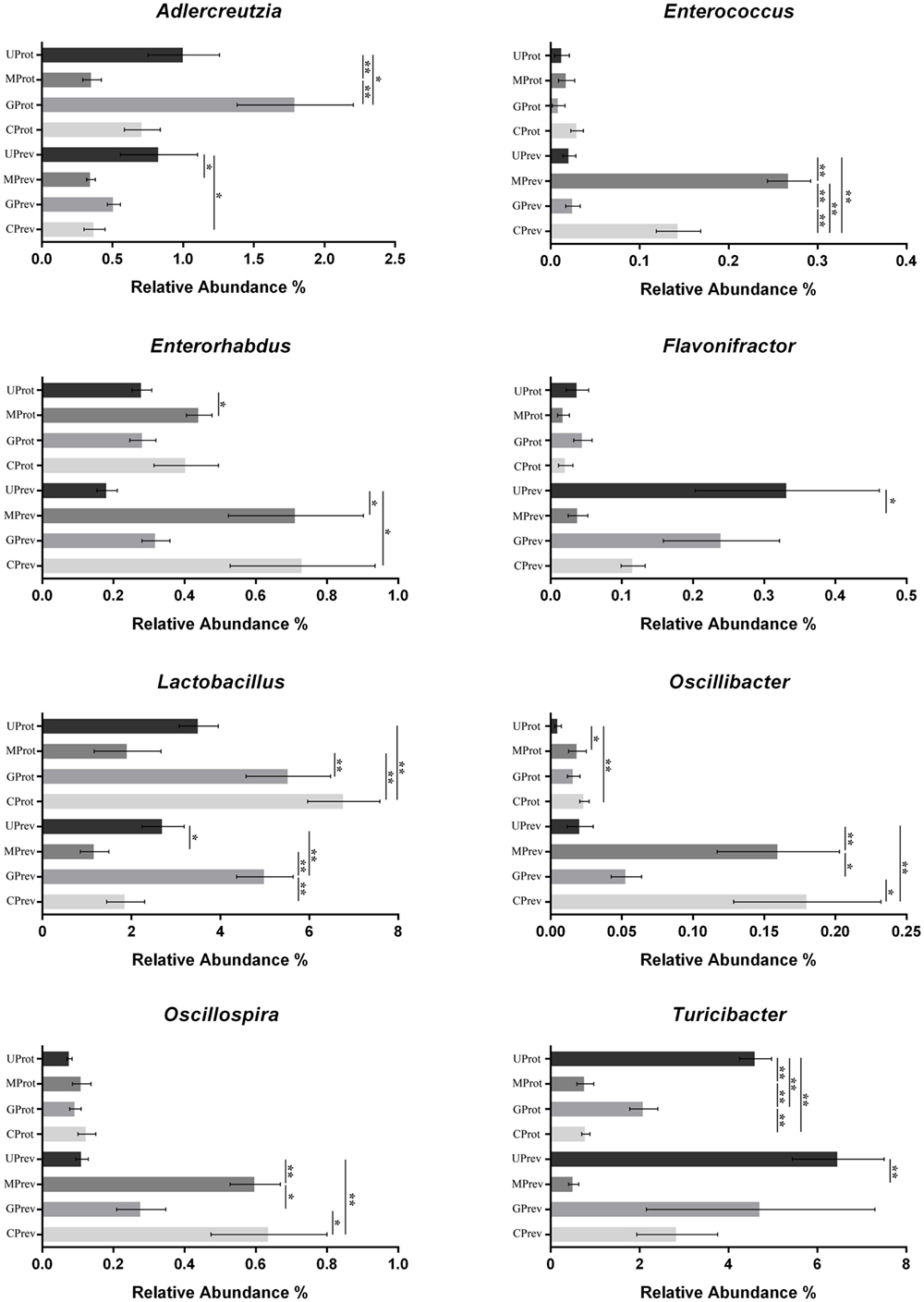
Figure 6. Abundance of the eight microbial genera significantly affected by the experimental treatments, in the gut microbiota of mice from the eight treatment groups: Samples were taken 1 day after the administration of CCl4 (to determine preventive effects of milk consumption on induced liver injury) from untreated control mice (UPrev), model mice (MPrev), mice receiving goats’ milk (GPrev), and mice receiving cows’ milk (CPrev) and 8 days after the administration of CCl4 (to determine protective effects of continued milk consumption on induced liver injury) from untreated control mice (UProt), model mice (MProt), mice receiving goats’ milk (GProt), and mice receiving cows’ milk (CProt). The data and error bars are presented as mean ± SEM. *P < 0.05; **P < 0.01.
Protective Role of Milk on CCl4-Induced Liver Damage
At the phylum level, there was no significant difference in the relative abundance of Verrucomicrobia, Actinobacteria, and Proteobacteria amongst the four groups (P > 0.05). However, relative abundance of Firmicutes was significantly lower in the MProt group than in the UProt and GProt groups (P < 0.05), but Bacteroidetes were significantly more abundant in the MProt group than in the UProt and GProt groups (P < 0.05). At the genus level, differences in distribution and abundance of the six previously mentioned microbial genera (i.e., Enterococcus, Enterorhabdus, Flavonifractor, Lactobacillus, Oscillibacter, and Oscillospira) were not significantly different in the gut microbiota of the GProt mice and the UProt mice (P > 0.05; Figure 6); distribution and abundance of the eight genera in the gut microbiota of CProt mice were more different to the UProt mice than to the GProt mice.
Overall, intraperitoneal administration of CCl4 induced an increase in the abundance of Enterococcus, Enterorhabdus, Oscillibacter, and Oscillospira and a decrease in the abundance of Adlercreutzia, Flavonifractor, Lactobacillus, and Turicibacter. Disparity amongst the eight genera gradually decreased over time between the MPrev and UPrev groups and between the MProt and UProt groups, indicating that mice are able to self-heal and recover intestinal microbial capability. Intake of goats’ milk could prevent imbalance in the gut microbiota and promote self-healing and recovery.
Enrichment of Functional Genes and Metabolic Pathways in Intestinal Microflora
The phosphotransferase system (PTS), glyoxylate and dicarboxylate metabolism, fructose and mannose metabolism, peptidoglycan biosynthesis, d-alanine metabolism, and selenocompound metabolism were enriched in the gut microbiota of UPrev mice (Table 2). Furthermore, glyoxylate and dicarboxylate metabolism, flagellar assembly, PTS, peptidoglycan biosynthesis, synthesis and degradation of ketone bodies, selenocompound metabolism, and d-alanine metabolism were enriched in the gut microbiota of GPrev mice. This shows that functional features of the gut microbiota of the GPrev mice and the UPrev mice were similar and different to the gut microbiota of the MPrev. More metabolic pathways were enriched in the goats’ milk group than in the untreated group, including histidine metabolism, cysteine and methionine metabolism, starch and sucrose metabolism, alanine, aspartate, glutamate metabolism, and energy metabolism. Enrichment of these carbohydrate and amino acids metabolisms in the gut microbiota of the goats’ milk group may be as a result of intra-gastric administration and the gut bacteria played important roles in nutrients and energy metabolism.
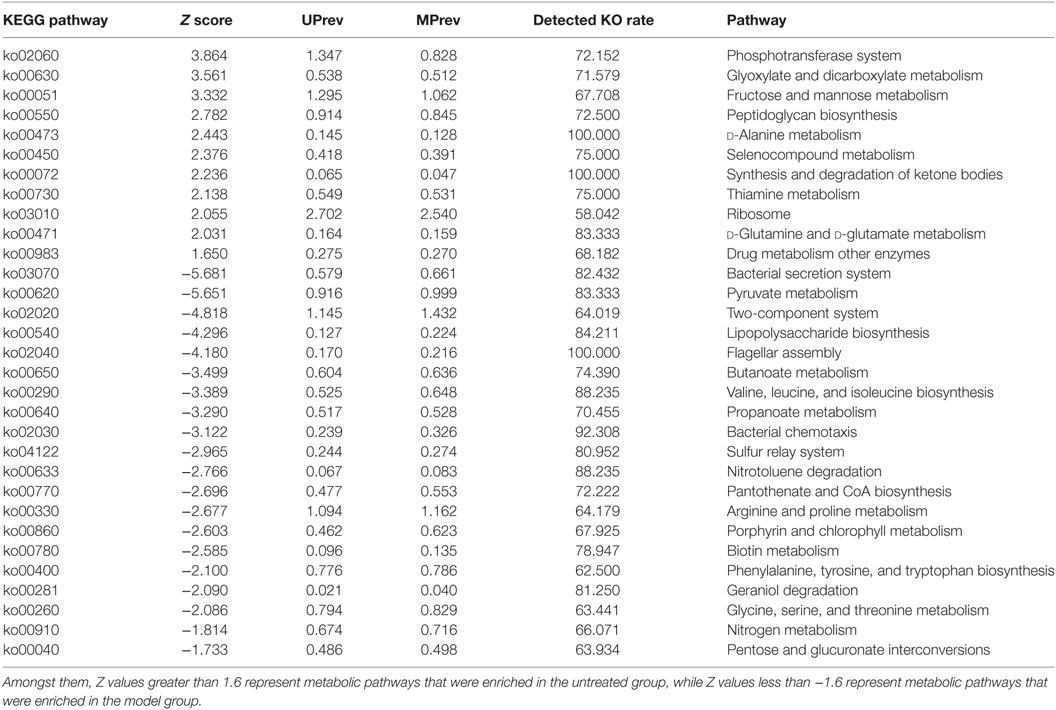
Table 2. Different metabolic pathways found in the gut microbiota of mice from the untreated (UPrev) and model (MPrev) treatment groups.
We found that metabolism of lipopolysaccharide (LPS) biosynthesis was enriched in model group (Z score = −4.296; Table 2). Moreover, a comparison amongst the different groups of mice found that the gut microbiota of the model group was more abundant in drug metabolism-cytochrome P450 and bile secretion than the untreated group (Table S1 in Supplementary Material). Greater enrichment of drug metabolism pathways in the gut microbiota of the model group compared with the other groups may be due to selection of genera that have a functional role in decomposition of the harmful chemical CCl4. Low levels of drug metabolism pathways were found in both the goats’ milk group and the untreated group. This may suggest that intake of goats’ milk had some protective effect in mice suffering the challenge of CCl4. Correlations amongst goats’ milk, varied significantly amongst bacterial genera, G group-enriched metabolisms, and the seven indicators of liver damage (Figure 7) showed that the G group of mice was positively correlated with Adlercreutzia (R = 0.35), Flavonifractor (R = 0.17), Lactobacillus (R = 0.58), and Turicibacter (R = 0.71) but negatively correlated with Enterococcus (R = −0.22), Enterorhabdus (R = −0.17), Oscillibacter (R = −0.28), and Oscillospira (R = −0.23). The G group of mice was significantly negatively correlated with drug metabolism-cytochrome P450 (R = −0.60; P < 0.05) and bile secretion (R = −0.35; P < 0.05).
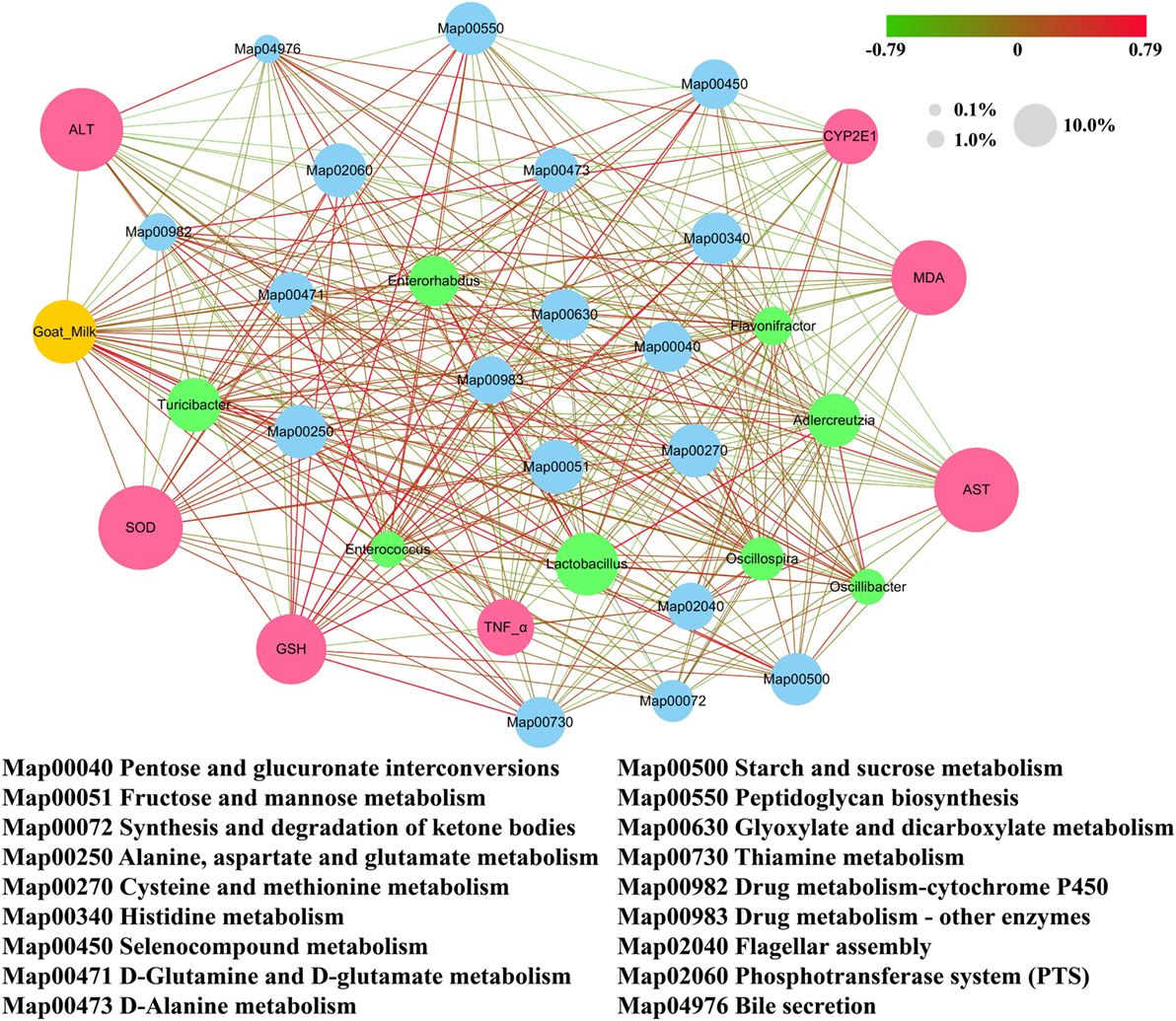
Figure 7. Correlation network established based on goats’ milk, bacterial genera that varied significantly in abundance, G group-enriched metabolisms, and the seven indicators of liver damage. Values of 0.1, 1.0, and 10.0% represent the relative abundance of the same category of elements (i.e., bacterial genera, metabolisms, and liver damage indicators) presented in the network. Positive correlations are connected by red lines (R > 0), and negative correlations are connected by gray lines (R < 0). The gradually changing color presented in the figure illustrates the degree of the correlation.
Discussion
Liver function can be affected by the food eaten; it is important to search for safe functional foods with potential hepatoprotective abilities. Food can alter the composition and community structure of the gut microbiota, and some foods can promote probiotics; previous studies have demonstrated a beneficial role of commensal gut microbiota and probiotics on liver function. Consequently, we need to take changes in gut microbiota into account when undertaking functional studies of particular foods. In this study, we investigated the hepatoprotective effects of goats’ milk and cows’ milk on mice with CCl4-induced acute hepatic injury. We also investigated the regulatory effects of these milks on CCl4-induced gut microbiota imbalance. Potential correlation networks amongst milk administration, indicators of acute liver injury, and gut microbiota and their functional features were established.
Histological analysis showed that the area of hepatic cell lobule lesions in the goats’ milk group was smaller than in the model and cows’ milk groups and this was because the expression of CYP2E1 was inhibited in the liver cells of mice in the goats’ milk group. In fact, CCl4 is converted into toxic substances in mice, mainly through activation and induction or over-expression of CYP2E1; the CYP2E1 protein is located mainly in the central region of the hepatic lobule, which explains why hepatocyte injury is typically in the centrilobular region after CCl4 administration (6). Histological analysis indicated that goats’ milk had beneficial effects on CCl4-induced acute hepatic injury; these hepatoprotective abilities of goats’ milk were confirmed by measuring the activity of ALT and AST in serum, which was significantly lower in mice receiving goats’ milk than in the model group of mice.
CCl4 is able to induce lipid peroxidation of the liver cell membrane, which is a key factor causing hepatocyte injury (6). Our results showed that ingestion of goats’ milk also prevented the increase in MDA induced by CCl4. This would greatly reduce lipid peroxidation injury and prevent a strong inflammatory response (22). Various antioxidant enzymes with high endogenous expression in the liver, such as SOD and GSH, are the major defense mechanisms against reactive oxygen species (23). Current results show that consumption of goats’ milk significantly elevated the activity of SOD and GSH in CCl4-damaged livers, indicating the ability of goats’ milk to restore and preserve the activity of these two enzymes.
Kupffer cells are activated by CCl4 and could mediate hepatic inflammation by producing TNF-α, which is one of the most potent pro-inflammatory cytokines released by innate immune cells (24). Goats’ milk significantly inhibited the expression of TNF-α, suggesting that it plays an important role in reducing the CCl4-induced inflammatory cascade in the liver. The mechanism for this protective effect may also be due to the scavenging effect of free radicals, the inhibition of lipid peroxidation, and the enhancement of antioxidant activity. In the goats’ milk group of mice, we observed that the liver tissue was less damaged 24 h after injection of CCl4 compared with the other groups receiving CCl4; the necrotic area of liver tissue had almost disappeared, and the structure of the liver lobule was restored to normal after 8 days of injection. There are two possible reasons for this phenomenon: the degree of tissue damage was slight and the ability of the tissues to repair was strong. Overall, our findings indicate that goats’ milk has a protective effect against CCl4-induced acute hepatic injury in mice, which is achieved by the scavenging of free radicals, inhibiting lipid peroxidation, enhancing the antioxidative defense system, and suppressing cell apoptosis and the inflammatory response of the liver.
Imbalance in the gut microbiota can affect liver function, which could lead to various liver diseases (25). Gut microbiota influence the progression of chronic liver diseases such as alcoholic fatty liver disease (AFLD), cirrhosis, and hepatocellular carcinoma (HCC) (26). AFLD patients have significantly fewer fecal Bifidobacterium, Lactobacillus, and Enterococcus but more Escherichia coli (27). Liver cirrhosis patients have fewer Faecalibacterium and Coprococcus but more Fusobacteria (28). Furthermore, HCC patients have higher proportions of Helicobacter (29). Chronic liver diseases develop from acute liver injury, so it is important to understand the relationship between gut microbiota and the severity of acute liver injury. Future studies should always include an accurate assessment of the gut microbiota using sophisticated molecular and microbiological methods. This would facilitate more effective selection of potentially useful functional foods or bacterial isolates that may contribute to the prevention of liver injury. The gut microbiota could be altered through dietary changes or administration of drugs. In this study, mice with CCl4-induced acute liver injury had fewer Adlercreutzia, Flavonifractor, Lactobacillus, and Turicibacter and more Enterococcus, Enterorhabdus, Oscillibacter, and Oscillospira. Intestinal Enterococcus species are known to promote liver disease (30), even though they are lactic acid bacteria. Diets rich in saturated fat can cause the development of a lipogenic liver and is associated with a decrease in the abundance of Lactobacillus and an increase in the abundance of Oscillibacter in the gut microbiota (31). Gram-negative LPS-producing bacteria, such as Oscillibacter, may activate bacterial translocation, leading to liver disease; LPS could also elevate levels of TNF-α by activating inflammatory signaling pathways, leading to chronic liver disease (32, 33). In contrast, SCFA- and butyrate-producing bacteria, such as Lactobacillus and Flavonifractor, inhibit harmful bacteria and promote balance in the gut microbiota (33, 34). We found that, regardless of whether mice were suffering from acute liver injury or chronic liver disease, the abundance of some probiotic genera decreased while other symbiotic bacteria increased. In this study, we found that intake of goats’ milk could prevent imbalance in the gut microbiota by promoting the probiotics and reducing harmful bacteria in the gut microbiota.
We also found that there were links amongst goats’ milk consumption, metabolism of the gut microbiota, and biochemical indices. Abundance of the drug metabolism-cytochrome P450 was lower in the gut microbiota of the goats’ milk group of mice than in the model group. This is consistent with down-regulation of the mRNA expression of CYP2E1 (CYP2E1 is a subtype of CYP450), which we observed in the liver tissue of mice in the goats’ milk group. We also demonstrated that the gut microbiota in the goats’ milk group of mice was involved in amino acid metabolism and energy metabolism in the KEGG pathways, indicating that goats’ milk may provide the energy source required for regulating and improving immunity. This is consistent with our observations on the indicators of liver tissue damage in the goats’ milk group of mice. The interesting point is that the gut microbiota of the goats’ milk group of mice was involved in bile secretion through the KEGG pathways and may thus protect the liver by regulation of bile secretion.
Goats’ milk is an important nutrient source, but the beneficial effects of goats’ milk on organisms with acute hepatic injury induced by CCl4 were previously unknown. In our research, we found that consumption of goats’ milk could protect mice from CCl4-induced acute hepatic injury and also improved the gut microbiota imbalance caused by CCl4. To the best of our knowledge, this is the first time that the hepatoprotective effects of goats’ milk have been demonstrated; goats’ milk has the potential to be developed as a new prospective functional food for alleviation of liver injury.
Ethics Statement
Animal experiments were performed in accordance with the guidelines of Shaanxi Normal University Scientific Ethics Committee.
Author Contributions
JZ and YS contributed to the experimental design and data analysis. ZW and DH performed the experiments. YS and ZW wrote the article.
Conflict of Interest Statement
The authors declare that the research was conducted in the absence of any commercial or financial relationships that could be construed as a potential conflict of interest.
Funding
The research was supported by the National Natural Science Foundation of China (No. 31701577), the Fundamental Research Funds for the Central Universities (Grant No. GK201603097), the Science and Technology Research and Development Program of Shaanxi Province, China (No. 2016NY-207), and the Scientific Research Foundation of Hainan University (No. KYQD1548).
Supplementary Material
The Supplementary Material for this article can be found online at https://www.frontiersin.org/articles/10.3389/fimmu.2018.01034/full#supplementary-material.
Table S1. The abundances of the metabolic pathways (Level 3) in gut microbiota of the four groups of mice (UPrev, MPrev, GPrev and CPrev).
References
1. Stärkel P, Schnabl B. Bidirectional communication between liver and gut during alcoholic liver disease. Semin Liver Dis (2016) 36:331–9. doi:10.1055/s-0036-1593882
2. Ley RE, Turnbaugh PJ, Klein S, Gordon JI. Microbial ecology: human gut microbes associated with obesity. Nature (2006) 444:1022–3. doi:10.1038/4441022a
3. Brandl K, Kumar V, Eckmann L. Gut-liver axis at the frontier of host-microbial interactions. Am J Physiol Gastrointest Liver Physiol (2017) 312:G413–9. doi:10.1152/ajpgi.00361.2016
4. Barratt MJ, Lebrilla C, Shapiro HY, Gordon JI. The gut microbiota, food science, and human nutrition: a timely marriage. Cell Host Microbe (2017) 22:134–41. doi:10.1016/j.chom.2017.07.006
5. Tilg H, Cani PD, Mayer EA. Gut microbiome and liver diseases. Gut (2016) 65:2035. doi:10.1136/gutjnl-2016-312729
6. Zamparelli MS, Compare D, Coccoli P, Rocco A, Nardone OM, Marrone G, et al. The metabolic role of gut microbiota in the development of nonalcoholic fatty liver disease and cardiovascular disease. Int J Mol Sci (2016) 17:1225. doi:10.3390/ijms17081225
7. Muriel P, Rivera-Espinoza Y. Beneficial drugs for liver diseases. J Appl Toxicol (2008) 28:93–103. doi:10.1002/jat.1310
8. Zhang F, Wang Z, Lei F, Wang B, Jiang S, Peng Q, et al. Bacterial diversity in goats’ milk from the Guanzhong area of China. J Dairy Sci (2017) 100:7812–24. doi:10.3168/jds.2017-13244
9. Minervini F, Bilancia MT, Siragusa S, Gobbetti M, Caponio F. Fermented goats’ milk produced with selected multiple starters as a potentially functional food. Food Microbiol (2009) 26:559–64. doi:10.1016/j.fm.2009.03.008
10. Ali S, Khan MR, Sajid M. Protective potential of parrotiopsis jacquemontiana (decne) rehder on carbon tetrachloride induced hepatotoxicity in experimental rats. Biomed Pharmacother (2017) 95:1853–67. doi:10.1016/j.biopha.2017.09.003
11. Weber LW, Boll M, Stampfl A. Hepatotoxicity and mechanism of action of haloalkanes: carbon tetrachloride as a toxicological model. Crit Rev Toxicol (2003) 33:105–36. doi:10.1080/713611034
12. Liu J, Fu Y, Zhang H, Wang J, Zhu J, Wang Y, et al. The hepatoprotective effect of the probiotic Clostridium butyricum against carbon tetrachloride-induced acute liver damage in mice. Food Funct (2017) 8:4042–52. doi:10.1039/c7fo00355b
13. Gómez-Hurtado I, Santacruz A, Peiró G, Zapater P, Gutiérrez A, Pérez-Mateo M, et al. Gut microbiota dysbiosis is associated with inflammation and bacterial translocation in mice with CCl4-induced fibrosis. PLoS One (2011) 6:e23037. doi:10.1371/journal.pone.0023037
14. Mazagova M, Wang L, Anfora AT, Wissmueller M, Lesley SA, Miyamoto Y, et al. Commensal microbiota is hepatoprotective and prevents liver fibrosis in mice. FASEB J (2015) 29:1043–55. doi:10.1096/fj.14-259515
15. Wang WW, Zhang Y, Huang XB, You N, Zheng L, Li J. Fecal microbiota transplantation prevents hepatic encephalopathy in rats with carbon tetrachloride-induced acute hepatic dysfunction. World J Gastroenterol (2017) 23:6983–94. doi:10.3748/wjg.v23.i38.6983
16. Li M, Zhu L, Xie A, Yuan J. Oral administration of Saccharomyces boulardii ameliorates carbon tetrachloride-induced liver fibrosis in rats via reducing intestinal permeability and modulating gut microbial composition. Inflammation (2015) 38:170–9. doi:10.1007/s10753-014-0019-7
17. Miglani S, Patyar RR, Patyar S, Reshi MR. Effect of goat milk on hepatotoxicity induced by antitubercular drugs in rats. J Food Drug Anal (2016) 24:716–21. doi:10.1016/j.jfda.2016.03.012
18. Ying W, Wan Y, Ye G, Pu W, Xue X, Wu G, et al. Hepatoprotective effects of adiporon against D-galactosamine-induced liver injury in mice. Eur J Pharm Sci (2016) 93:123–31. doi:10.1016/j.ejps.2016.08.017
19. Shao Y, Huo D, Peng Q, Pan Y, Jiang S, Liu B, et al. Lactobacillus plantarum HNU082-derived improvements in the intestinal microbiome prevent the development of hyperlipidaemia. Food Funct (2017) 8:4508–16. doi:10.1039/c7fo00902j
20. Wang Z, Shao Y. Effects of microbial diversity on nitrite concentration in pao cai, a naturally fermented cabbage product from China. Food Microbiol (2018) 72:185–92. doi:10.1016/j.fm.2017.12.003
21. Langille MG, Zaneveld J, Caporaso JG, McDonald D, Knights D, Reyes JA, et al. Predictive functional profiling of microbial communities using 16S rRNA marker gene sequences. Nat Biotechnol (2013) 8:814–21. doi:10.1038/nbt.2676
22. Tao W, Li J, Yong L, Song H. Antioxidant and hepatoprotective effect of swertiamarin on carbon tetrachloride-induced hepatotoxicity via the Nrf2/HO-1 pathway. Cell Physiol Biochem (2017) 42:2242–54. doi:10.1159/000475639
23. Lim DW, Kim H, Park JY, Kim JE, Moon JY, Park SD, et al. Amomum cardamomum L. ethyl acetate fraction protects against carbon tetrachloride-induced liver injury via an antioxidant mechanism in rats. BMC Complement Altern Med (2016) 16:155. doi:10.1186/s12906-016-1121-1
24. Hamid M, Liu D, Abdulrahim Y, Khan A, Gang Q, Huang K. Inactivation of kupffer cells by selenizing astragalus polysaccharides prevents CCl4-induced hepatocellular necrosis in the male Wistar rat. Biol Trace Elem Res (2017) 3:1–11. doi:10.1007/s12011-017-0970-x
25. Abushanab A, Quigley EMM. The role of the gut microbiota in nonalcoholic fatty liver disease. Nat Rev Gastroenterol Hepatol (2010) 7:691–701. doi:10.1038/nrgastro.2010.172
26. Haque TR, Barritt AS. Intestinal microbiota in liver disease. Best Pract Res Clin Gastroenterol (2016) 30:133–42. doi:10.1016/j.bpg.2016.02.004
27. Kirpich IA, Solovieva NV, Leikhter SN, Shidakova NA, Lebedeva OV, Sidorov PI, et al. Probiotics restore bowel flora and improve liver enzymes in human alcohol-induced liver injury: a pilot study. Alcohol (2008) 42:675. doi:10.1016/j.alcohol.2008.08.006
28. Qin N, Yang F, Li A, Prifti E, Chen Y, Shao L, et al. Alterations of the human gut microbiome in liver cirrhosis. Nature (2014) 513:59–64. doi:10.1038/nature13568
29. Rocha M, Avenaud P, Menard A, Le BB, Balabaud C, Bioulac-Sage P, et al. Association of Helicobacter species with hepatitis C cirrhosis with or without hepatocellular carcinoma. Gut (2005) 54:396–401. doi:10.1136/gut.2004.042168
30. Llorente C, Jepsen P, Inamine T, Wang L, Bluemel S, Wang HJ, et al. Gastric acid suppression promotes alcoholic liver disease by inducing overgrowth of intestinal Enterococcus. Nat Commun (2017) 8:837. doi:10.1038/s41467-017-00796-x
31. Lam YY, Ha CW, Campbell CR, Mitchell AJ, Dinudom A, Oscarsson J, et al. Increased gut permeability and microbiota change associate with mesenteric fat inflammation and metabolic dysfunction in diet-induced obese mice. PLoS One (2012) 7:e34233. doi:10.1371/journal.pone.0034233
32. Festi D, Schiumerini R, Eusebi LH, Marasco G, Taddia M, Colecchia A. Gut microbiota and metabolic syndrome. World J Gastroenterol (2014) 20:16079–94. doi:10.3748/wjg.v20.i43.16079
33. Song JJ, Tian WJ, Kwok LY, Wang YL, Shang YN, Menghe B, et al. Effects of microencapsulated Lactobacillus plantarum LIP-1 on the gut microbiota of hyperlipidaemic rats. Br J Nutr (2017) 118:481–92. doi:10.1017/S0007114517002380
Keywords: animal milk, gut microbiome, hepatoprotective effect, acute liver injury, carbon tetrachloride
Citation: Zhang J, Wang Z, Huo D and Shao Y (2018) Consumption of Goats’ Milk Protects Mice From Carbon Tetrachloride-Induced Acute Hepatic Injury and Improves the Associated Gut Microbiota Imbalance. Front. Immunol. 9:1034. doi: 10.3389/fimmu.2018.01034
Received: 23 January 2018; Accepted: 25 April 2018;
Published: 15 May 2018
Edited by:
Pinyi Lu, Biotherapeutics, Inc., United StatesReviewed by:
Fangyan Wang, Wenzhou Medical University, ChinaLushen Li, University of Maryland, Baltimore, United States
Heitor Affonso Paula Neto, Universidade Federal do Rio de Janeiro, Brazil
Phillipp Hartmann, University of California, San Diego, United States
Copyright: © 2018 Zhang, Wang, Huo and Shao. This is an open-access article distributed under the terms of the Creative Commons Attribution License (CC BY). The use, distribution or reproduction in other forums is permitted, provided the original author(s) and the copyright owner are credited and that the original publication in this journal is cited, in accordance with accepted academic practice. No use, distribution or reproduction is permitted which does not comply with these terms.
*Correspondence: Yuyu Shao, aWNoZWVzZW1ha2luZ0B5YWhvby5jb20=, eXV5dXNoYW9Ab3V0bG9vay5jb20=
†These authors have contributed equally to this work.