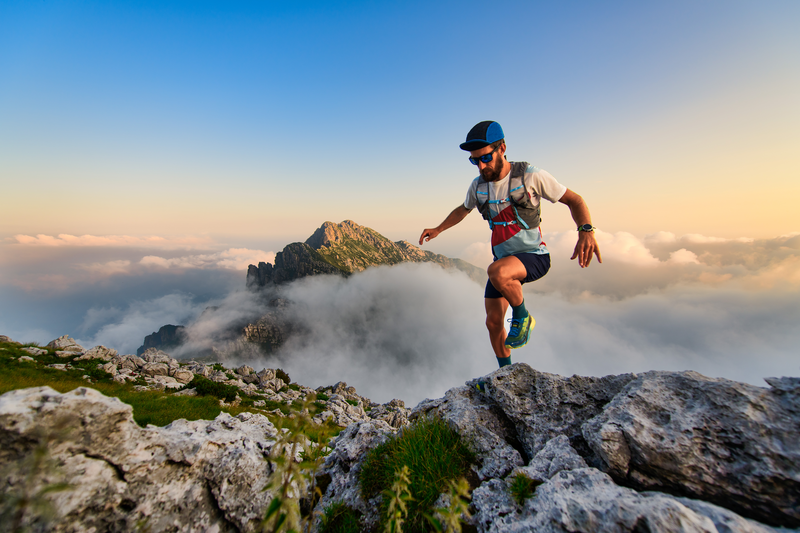
94% of researchers rate our articles as excellent or good
Learn more about the work of our research integrity team to safeguard the quality of each article we publish.
Find out more
PERSPECTIVE article
Front. Immunol. , 10 April 2018
Sec. Molecular Innate Immunity
Volume 9 - 2018 | https://doi.org/10.3389/fimmu.2018.00764
This article is part of the Research Topic C1q: A Molecular Bridge to Innate and Adaptive Immunity View all 10 articles
Complement component C1q plays an important recognition role in adaptive, and innate, immunity through its ability to interact, via its six globular head regions, with both immunoglobulin and non-immunoglobulin activators of the complement system, and also in the clearance of cell debris, and by playing a role in regulation of cellular events by interacting with a wide range of cell surface molecules. The presence of collagen-like triple-helical structures within C1q appears crucial to the presentation, and multivalent binding, of the globular heads of C1q to targets, and also to its association with the proenzyme complex of C1r2–C1s2, to yield the C1 complex. The possible role that movement of these collagen-like structures may play in the activation of the C1 complex is a controversial area, with there still being no definitive answer as to how the first C1r proenzyme molecule becomes activated within the C1 complex, thus allowing it to activate proenzyme C1s, and initiate and the consequent cascade of events in the activation of the classical pathway of complement. The globular heads of C1q are similar to domains found within the tumor necrosis factor (TNF) superfamily of proteins, and have been shown to bind to a very wide range of ligands. In addition to its well-defined roles in infection and immunity, a variety of other functions associated with C1q include possible roles, in the development of problems in the central nervous system, which occur with aging, and perhaps in the regulation of tumor growth.
Prior to the formal proof of there being collagen triple helical coils present in the C1q molecule (1), it was considered that any such protein, containing that feature, would most likely play a structural role in the extracellular matrix, rather than being involved in the activation of the serum complement system. However, it was recognized by the end of the 1980s (2) that several other serum proteins, besides subcomponent C1q, also contained collagen-like regions, and were likely to be involved in innate immune effector systems. These included the C-type lectins, mannose binding lectin (MBL), bovine conglutinin, and lung surfactant protein A. Indeed, conglutinin was the first vertebrate lectin to be characterized by virtue of its function to promote the agglutination of erythrocytes coated with activated complement components (3). It is now known that there are several other lectins, such as lung surfactant protein D, the ficolins, bovine serum lectin (CL-43), in a growing family of proteins, containing collagen-like regions, which are involved in immune defense. These proteins display a wide range of binding properties, via both their globular head regions and collagen-like triple-helical regions, toward both immune targets and cell surface receptors, and participate as a bridge between innate and adaptive immunity. The relationship between structure and function with respect to the many binding, and triggering, properties shown by C1q should now be able to be even more fully explored, by generation of point mutation variants, as a result of the major achievement of expression of the functionally fully active recombinant form of this structurally complicated protein, composed of three different polypeptide chains, in a mammalian cell system (4).
The C1q protein was first, accurately, described, in 1961, as a “11s thermolabile serum protein which precipitates γ-globulin aggregates and participates in immune hemolysis” (5), thus highlighting interesting features about its large size (460 kDa) and its binding properties (to immunoglobulin complexes) and its function (participation in complement-mediated hemolysis of antibody-coated red cells). In 1963 (6), Lepow et al. showed that the euglobulin fraction of human serum (proteins precipitated in low ionic strength buffer, at pH 5.5), which contained the then defined C1component of the complement system, could be fractionated, by ion-exchange chromatography into three subcomponents, which were defined, based on their elution positions, from an ion-exchange column, as C1q, C1r, and C1s (the nomenclature a, b, and c…, was not used, in order to avoid confusion with “C1a” being used for activated C1, at that time). It was shown that all three subcomponents were required to reconstitute the original C1 hemolytic activity. The use of further, new at the time, techniques, such as gel-filtration and affinity chromatography, allowed the isolation of highly purified C1q to perform detailed structural and functional studies.
Early chemical studies of human and rabbit C1q (7–9) provided indirect evidence, that there may be collagen-like structures within C1q, since it was reported to have an unusually high glycine content, to contain hydroxylysine and hydroxyproline residues and disaccharide units of glucosylgalactose, linked to the hyroxylysine, and it had a great susceptibility to collagenase. The first direct evidence for the presence of collagen-like amino acid sequence, in the A-chain of C1q, was obtained in 1974 (10).
It was then shown that the preparation of the collagen-like regions of C1q could be achieved by limited proteolysis of the native intact molecule with pepsin at pH 4.45, when the globular head regions are digested to small peptides leaving the large, 190 kDa, collagen-like region intact (1, 11). When viewed in the electron microscope, C1q was seen to be composed of six peripheral globular “head” regions, which are each joined by a collagen-like connecting strand to a fibril-like central portion/stalk (1, 12, 13).
All these studies allowed the proposal in 1976 (14) of a molecular model for subcomponent C1q (Figure 1), which has stood the test of time, in which there are 18 polypeptide chains (6 A-, 6 B-, and 6 C-chains), with disulfide bonds between the A- and B- chains and between pairs of C-chains, thus yielding nine dimers, i.e., six A–B dimers and three C–C dimers. The complete derived amino acid sequence, along with the characterization and organization of the genes encoding all three polypeptide chains of C1q, was completed in 1991 (15).
Figure 1. Proposed model of human subcomponent C1q. Initial diagram, drawn up in 1975, of the first published molecular model proposed for C1q (14). It was based on the electron microscopy measurements (12, 13), the amino acid sequencing and physical chemistry results of the studies on the 190 kDa pepsin-resistant fragment of C1q (1, 11), and the assumption that the collagen-like regions in the A-, B-, and C-chains of C1q form a triple helical collagen-type structure (denoted by the solid, broken, and wavy lines), and the C-terminal approximately 140 amino acid residues, in each of the A-, B-, and C-chains, form a globular heterotrimeric structure of 47.8 kDa (which should, more correctly, be shown as globular units, rather than the tulip-flower shapes as shown in the initial diagram). From the dimensions shown, which are averages of those given in the electron microscopy studies (12), the following comparisons can be made: length of collagen-like fiber plus fibril-like end piece = 115 + 112 = 227 Å. Length of triple helix proposed from amino acid sequences = 78 × 2.9 = 226 Å (2.9 Å is the rise of collagen helix per residue, and there are 78 residues, over the collagen-like regions, in each of the three chains of C1q).
The use of large fragments of C1q, produced by limited proteolysis with either collagenase or pepsin, allowed direct analysis of the primary functions of the two, very different, regions of the C1q molecule. The globular head regions of C1q can be prepared by digestion with collagenase at pH 7.4, which results in a rapid loss of C1q function (9, 16). These preparations, of globular subunits, with a molecular weight of 47 kDa, were shown (16) to be able to inhibit the binding of C1q to IgG immune complexes, thus directly illustrating the role of the peripheral globular head units in binding to IgG. The determination of the crystal structure globular head region of C1q (17), also prepared by collagenase digestion, has shown that it is an almost spherical heterotrimeric assembly (formed from the C-terminals regions of the A-, B-, and C-chains). This allowed molecular modeling with two of its well-defined targets, IgG and C-reactive protein (CRP), providing a good illustration of the versatility in binding shown by the globular heads of C1q. It was found that the large pepsin-resistant fragment of C1q, composed almost entirely of the collagen-like regions of the molecule, could act as an effective inhibitor of the reconstitution of whole C1 hemolytic activity, when intact C1q was mixed with C1r2–C1s2 (18). It has also been found that the pepsin-resistant fragment of C1q bound the unactivated C1r2–C1s2 with approximately the same strength as that of the intact C1q molecule (19). These studies provided strong functional evidence that the C1r2–C1s2 interacted primarily with the collagen-like regions of C1q, and was in agreement with electron microscopy studies of the whole C1 complex (20) which suggested that the C1r2–C1s2 binding site is in the middle of the triple helical collagen-like regions, close to where the collagen-like strands diverge out from the central fibril-like region (Figure 1).
It is clear from electron microscopy, and all the protein structural data available, that the 460 kDa C1q molecule adopts a bouquet of flowers shape, comprising six heterotrimeric collagen-like triple helices that associate in their N-terminal half to form a fibril-like structure, then diverge at a bend, or “kink,” approximately half-way along the collagen-like region to form six individual “stalks,” each terminating in a C-terminal heterotrimeric globular domain (Figure 1). Other serum proteins (MBLs, Ficolins) have an overall structural similarity to C1q, and also interact with their associated proteases via their collagen-like regions. It was noted that, on alignment of all the known, collagen-like sequences, present in various chains of C1q, MBL, and Ficolin, from several species, that there is a conserved amino acid sequence (-Hyp-Gly-Lys-Xaa-Gly-Pro-) in which it was shown, by site-directed mutagenesis of Ficolin A, that the lysine is the critical residue involved in interaction with its associated protease, MASP (21). This conserved site, also present in each of the three chains of C1q, is six Gly-Xaa-Yaa- triplets C-terminal to the link region of C1q, and it was, therefore, postulated that this is likely to be the major binding site for C1r2–C1s2 on the stalks of C1q (21). The importance of these lysine residues, in the B- and C- chains, and lesser extent the A-chain, for the interaction, and activation, of C1r2–C1s2 was elegantly formally proved by their mutation to alanine residues, with consequent loss-of-function. Further studies, involving the determination of the crystal structure of the CUB1-EGF-CUB2 region of C1s bound to a short triple helical collagen-like peptide, containing the important conserved lysine, provided strong direct structural evidence for the proposed, precise interaction site between C1q and C1r2–C1s2 (22).
The structural studies on globular head region of C1q show that it is member of the growing TNF superfamily (17, 23) and functional studies indicate that the C1q heads can bind a wide range of self and non-self ligands.
Primary targets for the six globular heads of C1q are the multiple Fc regions presented within immune complexes, containing IgG or IgM antibodies. Well-defined non-immunoglobulin targets, for the C1q heads, include CRP (24) and pentraxin 3, as well as lipopolysaccharides and bacterial porins. Apoptotic cells also form a major target (25–28), probably via C1q binding to phosphatidylserine and double-stranded DNA, thus allowing for opsonization and effective phagocytosis of cell debris (28, 29), and enhancement of the immunosuppressive nature of the apoptotic cells.
One of the first molecules to be proposed as a receptor, for the globular heads of C1q, is gC1qR/p33 (30), but this molecule, although found at the surface of a wide variety of cells, has been shown to be present mainly in mitochondria. Since it does not possess a transmembrane domain, or a lipid anchor, it appears that it must always have to interact with other cell surface molecules, in order to modulate intracellular functions.
After activation of the C1 complex, control of the activated C1r and C1s is mediated by C1-inhibitor which forms covalent complexes with both the activated C1r and C1s, rapidly removing them from the C1 complex, leaving the entire collagen-like region of C1q free to interact with potential cell-surface receptors. One such putative receptor was a 60 kDa molecule isolated from Raji cell membranes (31) and to bind to the collagen region of C1q (and thus became to be defined as cC1qR). The NH2-terminal amino acid sequence of this molecule, isolated from endothelial cells (32) was found to be identical to that of C1qR, isolated from tonsil lymphocytes, and also to that of calreticulin (33) The C1qR preparation was shown to bind to the collagen-like regions of C1q and several other collagen-like proteins (34). Although cC1qR/C1qR/calreticulin can be found at the surfaces of many cell types, it is primarily found in the endoplasmic reticulum, and lacks a transmembrane domain. Thus care must be taken to define both gC1qR and cC1qR as “C1q-binding proteins,” rather than as true “transmembrane receptors.”
A wide range of other cell surface molecules have now been shown to interact with the either the heads, or collagen-like regions, of C1q, and occasionally with both. This area has recently been thoroughly and critically reviewed (35). The putative receptors were neatly placed in four main groups, based on the nature of their extracellular domains: (i) large multi-modular ectodomains involved in interaction with multiple ligands (CR1, LRP1, and the scavenger receptors SR-F1 and SR-F3); (ii) integrins (α2-β1 and CR3/αM-β2); (iii) Ig-like receptors (RAGE, LAIR-1, and CD33); and (iv) the C-type lectin receptors, DC-SIGN and DC-SIGNR.
There are two recent publications (36, 37), which address the possible mechanism by which it is considered the first steps of activation of proenzyme C1r, within the C1 complex, may take place, and they come to significantly different conclusions.
One view, the intramolecular view (36), is that the proenzyme C1r domains must be linked together at the center of the heterotetramer C1r2–C1s2, and that autoactivation of proenzyme C1r occurs as soon as the contacts between the catalytic domains are broken, possibly by flexibility of the collagen-like stems of C1q, on contact of the globular heads with targets. These conclusions derive from previous observations and are associated with studies on the interactions, in solution, of fragments (the CUB1-EGF-CUB2 fragments) of the C1r and C1s involved in binding to C1q, and also the crystal structure of a complex of these two fragments, allowing a close analysis of the C1s–C1r interface.
The other view, the intermolecular view (37), is that the C1 complex cross-activates by interacting with neighboring C1 complexes. This model is based on synchrotron small-angle X-ray scattering and electron microscopy studies and concludes that there is cleavage of proenzyme C1r in one C1 complex by C1r in a neighboring complex. The two models differ in the precise manner in which the C1r2–C1s2 complex is aligned, at the now generally agreed position, within the collagenous stems of C1q. The intramolecular view (36) does appear to be consistent with the first-order kinetics, reported by several groups, as regards activation of C1, but does rely upon contacts between the catalytic domains being broken, presumably by flexibility within the collagen-like stems on binding to a target, and this has not yet been formally proven. However, although collagen-like structures are seen as structures with only limited flexibility, all observations, made on C1q, suggest flexible hinge movements at the level of the “kink,” which can modulate the positions of the six globular domains. The intermolecular model (37), in which the serine protease domains are considered to be located at the periphery of the C1r2–C1s2 complex is consistent with the fact that C1 can bind to a structurally diverse range of activators and allows intermolecular activation between neighboring complexes. In a very recent study (38), cryo-electron microscopy was used to examine C1 bound to monoclonal antibodies, and the authors observed heterogeneous structures of single and clustered C1-IgG1 hexamer complexes. This structural data was interpreted as showing that, upon antibody binding, the C1q arms condense, thus inducing rearrangements of the C1r2–C1s2 complex and tilting the C1q’s cone-shaped stalk. Thus, it was concluded that C1r perhaps could activate C1s within single, strained C1 complexes, or between neighboring C1 complexes on surfaces (38).
Final general acceptance of one, or other, or indeed a combination, of these models of C1 activation awaits further study.
Unlike most of the other complement proteins, which are mainly liver-derived, C1q is synthesized primarily by macrophages, as demonstrated by the fact that bone marrow transplantation from wild-type mice into C1q-deficient (C1qa−/−) mice was able to restore the normal serum levels of C1q (39). This finding has prompted the use of hematopoietic stem cell transplantation in the remarkable, and successful, treatment of genetic human C1q deficiency (40), a condition where the complete absence of C1q function results in an exceptionally high risk of severe lupus erythematosus, and complications with skin and renal diseases.
In normal healthy human sera, C1q has a concentration of around 80 µg/ml (0.17 µM in serum) and thus is present in an approximately equimolar concentration to that of the C1r2–C1s2 complex (50 µg/ml of each of C1r and C1s, thus 0.15 µM C1r2–C1s2). The concentration of C1q rises quite steeply with aging, reaching 161 µg/ml, in the 60–81, years old, age group (41). It is of interest that the high serum level of C1q could surprisingly be reduced by a simple exercise regime, down to almost normal levels, which may be of significance to health in old age. An even more dramatic (10- to 300-fold) increase in C1q levels in the central nervous system (42) has been reported in mouse and human brains, with the highest levels being seen in close proximity to synapses and central regions of the brain. Interestingly, aged C1q-deficient (C1qa−/−) mice showed less cognitive and memory decline in hippocampus-dependent behavior tests compared to their wild-type litter mates (42), thus suggesting that C1q may play a role in the development of problems, during aging, that are seen in the central nervous system.
Another connection between C1q and aging has emerged from studies that there is elevated Wnt signaling in aging mice, where muscle stem cells have an increased tendency to fibroblastic differentiation (43). This appears to be due to the binding by one, or more, serum factors, to the Frizzled family of cell surface receptors, thus causing Wnt receptor signaling, and one Frizzled-binding protein has been identified to be C1q (44). It has been proposed that when C1q, within the C1 complex, binds to Frizzled then activated C1s cleaves lipoprotein receptor 6, which is a Wnt co-receptor, thus causing canonical Wnt signaling and accelerated aging. Thus abnormally high, or low, C1q levels could possibly play a role in various disease states caused by increased, or decreased, Wnt signaling.
There is growing evidence that C1q binding, leading to activation of C1r and C1s, may trigger other functions via activated C1s, unexpectedly, cleaving nuclear antigens, MHC class I antigens and other proteins, as well as lipoprotein receptor 6 (45), thus opening up many other biological pathways, besides complement, that may be triggered by C1q binding.
A recent study has indicated that non-bone marrow derived, locally synthesized, C1q may play a role in enhancing tumor progression by facilitating cancer cell seeding and promoting angiogenesis (46). This view is consistent with the finding of increased expression of the genes of the chains of C1q correlating with a poor prognosis in breast cancers (47). However, other studies indicate that C1q may possibly play a protective role, as judged by C1q-enhanced apoptosis in an ovarian cancer cell line (48) and activation of a tumor suppressor to induce apoptosis in prostate cancer cells (49).
There have been many publications concerned with the possible functions mediated via C1q, since its first description in 1961 (5), and perhaps more the use of the well-defined, globular, and collagen-like, fragments of C1q, monoclonal antibodies against these regions, or mutation of specific residues within the expressed whole recombinant molecule (4), will aid understanding of how exactly C1q is interacting with the large number of targets (in the region of 100, to date) to which it has been reported to bind. The use of a short synthetic triple helical peptide, corresponding to the binding site on C1q for the C1r2–C1s2 complex (22), to define the precise region on C1q involved in that interaction shows there are possibly many other innovative ways, utilizing protein fragments or synthetic peptides, which will allow the exploration, at the molecular level, of the functions of this versatile molecule.
The author confirms being the sole contributor of this work and approved it for publication.
The author declares that the research was conducted in the absence of any commercial or financial relationships that could be construed as a potential conflict of interest.
CTRP, C1q/TNF-related protein; MBL, mannose binding lectin; MASP, MBL-associated serine protease; CRP, C-reactive protein; CUB, complement C1r/C1s; EGF, epidermal growth factor.
1. Brodsky-Doyle B, Leonard KR, Reid KB. Circular-dichroism and electron microscopy studies of human subcomponent C1q before and after limited proteolysis by pepsin. Biochem J (1976) 159(2):279–86. doi:10.1042/bj1590279
2. Thiel S, Reid KB. Structures and functions associated with the group of mammalian lectins containing collagen-like sequences. FEBS Lett (1989) 250:78–84. doi:10.1016/0014-5793(89)80689-1
3. Bordet J, Streng O. Les phenomenes d’absorption et la conglutinine du serum de boeuf. Zentrabl Bactriol (1909) 49:260–76.
4. Bally I, Ancelet S, Moriscot C, Gonnet F, Mantovani A, Daniel R, et al. Expression of recombinant human complement C1q allows identification of the C1r/C1s-binding sites. Proc Natl Acad Sci U S A (2013) 110(21):8650–5. doi:10.1073/pnas.1304894110
5. Müller-Eberhard HJ, Kunkel HG. Isolation of a thermolabile serum protein which precipitates γ-globulin aggregates and participates in immune hemolysis. Proc Soc Exp Biol Med (1961) 106:291–5. doi:10.3181/00379727-106-26313
6. Lepow IH, Naff GB, Todd EW, Pensky J, Hinz CF. Chromatographic resolution of the first component of human complement into three activities. J Exp Med (1963) 117:983–1008. doi:10.1084/jem.117.6.983
7. Yonemasu K, Stroud RM, Niedermeier W, Butler WT. Chemical studies on C1q; a modulator of immunoglobulin biology. Biochem Biophys Res Commun (1971) 43:1388–94. doi:10.1016/S0006-291X(71)80028-1
8. Calcott MA, Müller-Eberhard HJ. C1q protein of human complement. Biochemistry (1972) 11:3443–50. doi:10.1021/bi00768a01
9. Reid KB, Lowe DM, Porter RR. Isolation and characterization of C1q, a subcomponent of the first component of complement, from human and rabbit sera. Biochem J (1972) 130(3):749–63. doi:10.1042/bj1300749
10. Reid KB. A collagen-like amino acid sequence in a polypeptide chain of human C1q (a subcomponent of the first component of complement). Biochem J (1974) 141(1):189–203. doi:10.1042/bj1410189
11. Reid KB. Isolation, by partial pepsin digestion, of the three collagen-like regions present in subcomponent Clq of the first component of human complement. Biochem J (1976) 155(1):5–17. doi:10.1042/bj1550005
12. Shelton E, Yonemasu K, Stroud RM. Ultrastructure of the human complement component C1q. Proc Natl Acad Sci U S A (1972) 69(1):65–8. doi:10.1073/pnas.69.1.65
13. Knobel HR, Villiger W, Isliker H. Chemical analysis and electron microscopy studies of human C1q prepared by different methods. Eur J Immunol (1975) 5(1):78–82. doi:10.1002/eji.1830050119
14. Reid KB, Porter RR. Subunit composition and structure of subcomponent C1q of the first component of human complement. Biochem J (1976) 155(1):19–23. doi:10.1042/bj1550019
15. Sellar GC, Blake DJ, Reid KB. Characterization and organization of the genes encoding the A-, B- and C-chains of human complement subcomponent C1q. The complete derived amino acid sequence of human C1q. Biochem J (1991) 274(2):481–90. doi:10.1042/bj2740481
16. Hughes-Jones NC, Gardener B. Reaction between the isolated globular sub-units of the complement component Clq and IgG-complexes. Mol Immunol (1979) 16(9):697–701. doi:10.1016/0161-5890(79)90010-5
17. Gaboriaud C, Juanhuix J, Gruez A, Lacroix M, Darnault C, Pignol D, et al. The crystal structure of the globular head of complement protein C1q provides a basis for its versatile recognition properties. J Biol Chem (2003) 278(47):46974–82. doi:10.1074/jbc.M307764200
18. Reid KB, Sim RB, Faiers AP. Inhibition of the reconstitution of the haemolytic activity of the first component of human complement by a pepsin-derived fragment of subcomponent C1q. Biochem J (1977) 161(2):239–45. doi:10.1042/bj1610239
19. Siegel C, Schumaker VN. Measurement of the association constants of the complexes formed between intact C1q or pepsin-treated C1q stalks and the unactivated or activated C1r2C1s2 tetramers. Mol Immunol (1983) 20(1):53–66. doi:10.1016/0161-5890(83)90105-0
20. Strang CJ, Siegel RC, Phillips ML, Poon PH, Schumaker VN. Ultrastructure of the first component of human complement: electron microscopy of the cross-linked complex. Proc Natl Acad Sci U S A (1982) 79(2):586–90. doi:10.1073/pnas.79.2.586
21. Girija UV, Dodds AW, Roscher S, Reid KB, Wallis R. Localization and characterization of the mannose-binding lectin (MBL)-associated-serine protease-2 binding site in rat ficolin-A: equivalent binding sites within the collagenous domains of MBLs and ficolins. J Immunol (2007) 179(1):455–62. doi:10.4049/jimmunol.179.1.455
22. Girija UV, Gingras AR, Marshall JE, Panchel R, Sheikh MA, Harper JA, et al. Structural basis of the C1q/C1s interaction and its central role in assembly of the C1 complex of complement activation. Proc Natl Acad Sci U S A (2013) 110(34):13916–20. doi:10.1073/pnas.1311113110
23. Ghai R, Waters P, Rouenia LT, Gadjeva M, Kojouharova MS, Reid KB, et al. C1q and its growing family. Immunobiology (2007) 212:253–66. doi:10.1016/j.imbio.2006.11.001
24. McGrath FD, Brouwer MC, Arlaud GJ, Daha MR, Hack CE, Roos A. Evidence that complement protein C1q interacts with C-reactive protein through its globular head region. J Immunol (2006) 176(5):2950–7. doi:10.4049/jimmunol.176.5.2950
25. Korb LC, Ahearn JM. C1q binds directly and specifically to surface blebs of apoptotic human keratinocytes: complement deficiency and systemic lupus erythematosus revisited. J Immunol (1997) 158(10):4525–8.
26. Navratil JS, Watkins SC, Wisnieski JJ, Ahern JM. The globular heads of C1q specifically recognise surface blebs of apoptotic vascular endothelial cells. J Immunol (2001) 166(5):3231–9. doi:10.4049/jimmunol.166.5.3231
27. Ogden CA, deCathelineau A, Hoffmann PR, Bratton D, Ghebrehiwet B, Fadok VA, et al. C1q and mannose binding lectin engagement of cell surface calreticulin and CD91 initiates macropinocytosis and uptake of apoptotic cells. J Exp Med (2001) 194:781–95. doi:10.1084/jem.194.6.781
28. Gaboriaud C, Frachet P, Thielens NM, Arlaud GJ. The human G1q globular domain: structure and recognition of non-immune self ligands. Front Immunol (2012) 2:92. doi:10.3389/fimmu.2011.00092
29. Nauta AJ, Trouw LA, Daha MR, Tijsma O, Nieuwland R, Schwaeble WJ, et al. Direct binding of C1q to apoptotic cells and cell blebs induces complement activation. Eur J Immunol (2002) 32:1726–36. doi:10.1002/1521-4141(200206)32:6<1726::AID-IMMU1726>3.0.CO;2-R
30. Ghebrehiwet B, Lim BL, Peerschke EI, Willis AC, Reid KB. Isolation, cDNA cloning, and overexpression of a 33-kD cell surface glycoprotein that binds to the globular “heads” of C1q. J Exp Med (1994) 214(12):1809–21. doi:10.1084/jem.179.6.1809
31. Ghebrehiwet B, Silvestri L, McDevitt C. Identification of the Raji cell membrane derived C1q inhibitor as a receptor for human C1q. J Exp Med (1984) 160(5):1375–89. doi:10.1084/jem.160.5.1375
32. Peerschke EI, Malhotra R, Ghebrehiwet B, Reid KB, Willis AC, Sim RB. Isolation of human endothelial cell C1q receptor (C1qR). J Leukoc Biol (1993) 53(2):179–84. doi:10.1002/jlb.53.2.179
33. Malhotra R, Willis AC, Jensenius JC, Jackson J, Sim RB. Structure and homology of human C1q receptor (collectin receptor). Immunology (1993) 78(3):341–8.
34. Malhotra R, Laursen SB, Willis AC, Sim RB. Localization of the receptor-binding site in the collectin family of proteins. Biochem J (1993) 293(1):15–9. doi:10.1042/bj2930015
35. Thielens NM, Tedesco F, Bohlson SS, Gaboriaud C, Tenner AJ. C1q: a fresh look upon an old molecule. Mol Immunol (2017) 89:73–83. doi:10.1016/j.molimm.2017.05.025
36. Almitairi J, Girija UV, Furze C, Simpson-Gray X, Badakshi F, Marshall J, et al. Structure of the C1r-C1s interaction of the C1 complex of complement activation. Proc Natl Acad Sci U S A (2018) 115(4):768–73. doi:10.1073/pnas.1718709115
37. Mortensen SA, Sander B, Jensen RK, Pedersen JS, Golas MM, Jensenius JC, et al. Structure and activation of C1, the complex initiating the classical pathway of the complement cascade. Proc Natl Acad Sci U S A (2017) 114(5):986–91. doi:10.1073/pnas.1616998114
38. Ugurlar D, Howes SC, de Kreuk B-J, Koning RI, de Jong RN, Beurskens FJ, et al. Structures of C1-IgG1 provide insights into how danger pattern recognition activates complement. Science (2018) 359(6377):794–7. doi:10.1126/science.aao4988
39. Petry F, Botto M, Holtappels R, Walport MJ, Loos M. Reconstitution of the complement function in C1q-deficient (C1qa-/-) mice with wild-type bone marrow cells. J Immunol (2001) 167(7):4033–7. doi:10.4049/jimmunol.167.7.4033
40. Arkwright PD, Riley P, Hughes SM, Alachkar H, Wynn RF. Successful cure of C1q deficiency in human subjects treated with hematopoietic stem cell transplantation. J Allergy Clin Immunol (2014) 133(1):265–7. doi:10.1016/j.jaci.2013.07.035
41. Watanabe S, Sato K, Hasegawa N, Kurihara T, Matsutani K, Sanada K, et al. Serum C1q as a novel biomarker of sarcopenia in older adults. FASEB J (2015) 29(3):1003–10. doi:10.1096/fj.14-262154
42. Stephan AH, Madison DV, Mateos JM, Fraser DA, Lovelett EA, Coutellier L, et al. A dramatic increase of C1q protein in the CNS during normal aging. J Neurosci (2013) 3(33):13460–74. doi:10.1523/JNEUROSCI.1333-13.2013
43. Brack AS, Conboy MJ, Roy S, Lee M, Kuo CJ, Keller C, et al. Increased Wnt signaling during aging alters muscle stem cell fate and increases fibrosis. Science (2007) 317(5839):807–10. doi:10.1126/science.1144090
44. Naito AT, Sumida T, Nomura S, Liu ML, Higo T, Nakagawa A, et al. Complement C1q activates canonical Wnt signaling and promotes aging-related phenotypes. Cell (2012) 149:1298–313. doi:10.1016/j.cell.2012.03.047
45. Lu J, Kishore U. C1 complex: an adaptable proteolytic module for complement and non-complement functions. Front Immunol (2017) 8:592. doi:10.3389/fimm.2017.00592
46. Bulla R, Tripodo C, Rami D, Ling GS, Agostinis C, Guarnotta C, et al. C1q acts in the tumour microenvironment as a cancer-promoting factor independently of complement activation. Nat Commun (2016) 7:10346. doi:10.1038/ncomms10346
47. Winslow S, Leandersson K, Edsjo A, Larsson C. Prognostic stromal gene signatures in breast cancer. Breast Cancer Res (2015) 17:23–36. doi:10.1186/s13058-015-0530-2
48. Kaur A, Sultan SHA, Murugaiah V, Pathan AA, Alhamlan FS, Karteris E, et al. Human C1q induces apoptosis in an ovarian cancer cell line via tumour necrosis factor pathway. Front Immunol (2016) 7:599. doi:10.3389/fimmu.2016.00599
Keywords: C1 activation, C1q, collagen-like structure, globular heads, tumor, aging
Citation: Reid KBM (2018) Complement Component C1q: Historical Perspective of a Functionally Versatile, and Structurally Unusual, Serum Protein. Front. Immunol. 9:764. doi: 10.3389/fimmu.2018.00764
Received: 31 January 2018; Accepted: 27 March 2018;
Published: 10 April 2018
Edited by:
Berhane Ghebrehiwet, Stony Brook University, United StatesReviewed by:
Christine Gaboriaud, UMR5075 Institut de Biologie Structurale (IBS), FranceCopyright: © 2018 Reid. This is an open-access article distributed under the terms of the Creative Commons Attribution License (CC BY). The use, distribution or reproduction in other forums is permitted, provided the original author(s) and the copyright owner are credited and that the original publication in this journal is cited, in accordance with accepted academic practice. No use, distribution or reproduction is permitted which does not comply with these terms.
*Correspondence: Kenneth B. M. Reid, a2VubmV0aC5yZWlkQGJpb2NoLm94LmFjLnVr
Disclaimer: All claims expressed in this article are solely those of the authors and do not necessarily represent those of their affiliated organizations, or those of the publisher, the editors and the reviewers. Any product that may be evaluated in this article or claim that may be made by its manufacturer is not guaranteed or endorsed by the publisher.
Research integrity at Frontiers
Learn more about the work of our research integrity team to safeguard the quality of each article we publish.