- Department of Respiratory Medicine, Union Hospital, Tongji Medical College, Huazhong University of Science and Technology, Wuhan, China
Tumor necrosis factor (TNF) is a pleiotropic cytokine that has both pro-inflammatory and anti-inflammatory functions. The biological functions of TNF are mediated by two receptors, TNF receptor type I (TNFR1) and TNF receptor type II (TNFR2). TNFR1 is expressed universally on almost all cell types and has been extensively studied, whereas TNFR2 is mainly restricted to immune cells and some tumor cells and its role is far from clarified. Studies have shown that TNFR2 mediates the stimulatory activity of TNF on CD4+Foxp3+ regulatory T cells (Tregs) and CD8+Foxp3+ Tregs, and is involved in the phenotypic stability, proliferation, activation, and suppressive activity of Tregs. TNFR2 can also be expressed on CD8+ effector T cells (Teffs), which delivers an activation signal and cytotoxic ability to CD8+ Teffs during the early immune response, as well as an apoptosis signal to terminate the immune response. TNFR2-induced abolition of TNF receptor-associated factor 2 (TRAF2) degradation may play an important role in these processes. Consequently, due to the distribution of TNFR2 and its pleiotropic effects, TNFR2 appears to be critical to keeping the balance between Tregs and Teffs, and may be an efficient therapeutic target for tumor and autoimmune diseases. In this review, we summarize the biological functions of TNFR2 expressed on CD8+Foxp3+ Tregs and CD8+ Teffs, and highlight how TNF uses TNFR2 to coordinate the complex events that ultimately lead to efficient CD8+ T cell-mediated immune responses.
Introduction
Tumor necrosis factor (TNF) is a pleiotropic cytokine involved in regulating diverse functions, including cell growth modulation, viral replication, septic shock, tumorigenesis, inflammation, and autoimmunity (1, 2). These functions hinge upon the binding of TNF to two distinct membrane receptors on target cells: TNF receptor (TNFR) 1and TNFR2. TNFR1 is expressed universally on almost all cell types, whereas TNFR2 is restricted to immune cells (2–6) and some tumor cells (7–13). Since TNFR1 and TNFR2 were identified (14), multiple studies have been carried out to characterize their structures and functions. While TNFR1 has been extensively characterized, the biological functions of TNFR2 have remained elusive (15). There is mounting evidence to suggest that TNFR2 is expressed on and has critical roles in immune cells, including CD4+ regulatory T cells (Tregs) (16), CD4+ effector T cells (Teffs) (4), CD8+ Tregs (17), and CD8+ Teffs (18). This implies that TNFR2 is involved in various T cell-mediated immune responses. TNFR2 expressed on CD4+ T cells has been studied in depth with many studies indicating that TNFR2 mediates the stimulatory activity of TNF on CD4+ Treg cells, resulting in their phenotypic stability, proliferation, and activation (3, 19–22). Furthermore, TNFR2 can be used to identify the maximally suppressive subset of CD4+ Tregs (20). However, studies on TNFR2 expression on CD8+ T cells are relatively deficient. Several studies have identified TNFR2 as a potent costimulatory molecule on CD8+ T cells required to sustain cell survival and protect from apoptosis, while TNFR2 expressing CD8+Foxp3+ Tregs exhibited highly suppressive activity (17, 23, 24).
The restricted distribution of TNFR2 has identified it as a potential target for immunotherapy. Targeting TNFR2 for cancer immunotherapy has seen remarkable success. Treatment of OVVAR3, an ovarian cancer cell line with surface expression of TNFR2, with a TNFR2 antagonist induced significant tumor cell death. Furthermore, the TNFR2 antagonist preferentially suppressed the activity of tumor-associated CD4+ Treg cells, but had little inhibitory effects on peripheral CD4+ Treg cells or cells from healthy donors (25). This result indicates that patients treated with a TNFR2 antagonist can maintain immunological homeostasis and mitigate the collateral damage to healthy tissues (20, 25). While the potential effects of TNFR2 antagonists on tumors have been documented, major questions remain unanswered, including how much the effects of therapeutically targeting TNFR2 in vivo are directly related to modulating T cell activity. Better knowledge of the fundamental biological processes, such as signaling pathway activation and the molecular mechanism underlying the T cell response to TNFR2 stimulation, especially in Treg cells, may help design safer and more effective targeted therapeutics. As TNFR2 expression on CD4+ T cells has been documented in detail, in this review, we mainly summarize and discuss the biological effects of TNFR2 expression on CD8+Foxp3+ Tregs and CD8+ Teffs.
TNFR2 Expressed on CD8+ Tregs
The suppressive effects of CD8+ Tregs on normal and pathologic immune responses are well described (Figure 1) (26–28). Previous study demonstrated that human CD8+CD25+ Tregs share many features with CD4+CD25+ Tregs in the thymus, such as phenotype, function, and mechanisms of action (23). Increasing evidence suggests that TNFR2 is a significant biomarker for highly potent suppressive Tregs, because TNFR2 promotes the activation, expansion, and survival of CD4+ Tregs by mediating the effect of TNF (29). However, most studies on TNFR2 expression on Tregs have focused on the CD4+ Tregs population, rather than CD8+ Tregs. Current results suggest that TNFR2 might also be a critical suppressive maker of the functional CD8+Foxp3+ Tregs. However, CD8+ Tregs are not the CD8+ counterpart of CD4+ Tregs. There are multiple subsets of CD8+ Tregs reported in both humans and mice (30), such as CD8+CD122+ Tregs (31), CD8+CD28− Tregs (32, 33), and CD8+CD103+ Tregs (34, 35). Unfortunately, the published studies on TNFR2 expression on CD8+Tregs all focused on CD8+Foxp3+ Tregs. As a consequence, we can only summarize the biological effects of TNFR2 expressed on CD8+Foxp3+ Tregs.
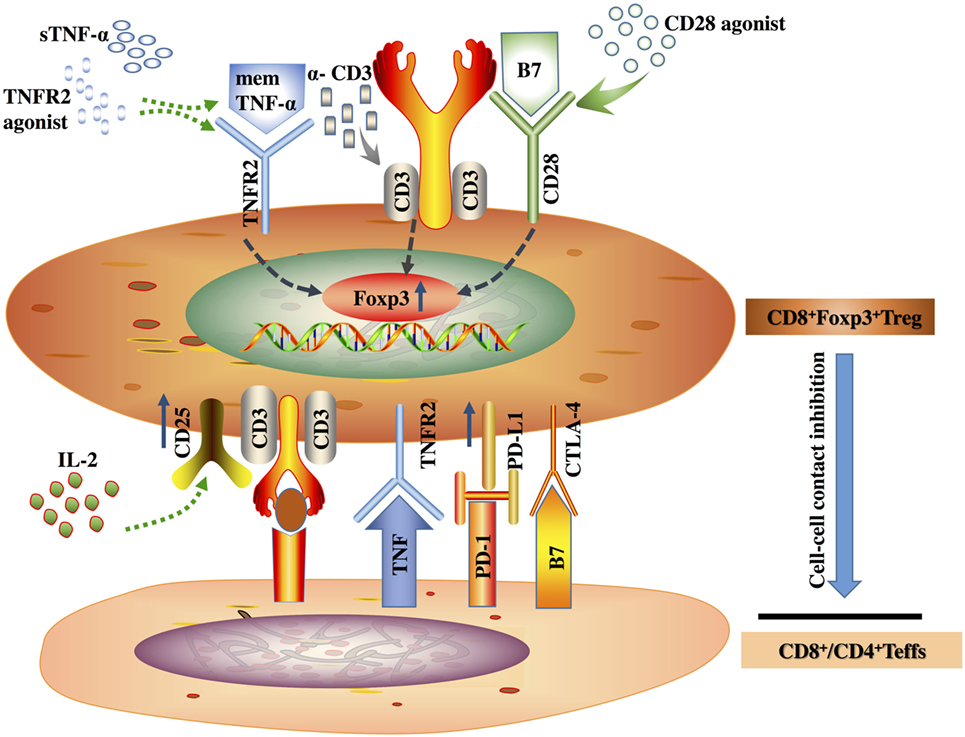
Figure 1. Tumor necrosis factor (TNF) receptor type II (TNFR2) acts as a suppressive marker for CD8+ regulatory T (Tregs) cells. The TNF/TNFR2 interaction, as well as TNFR2 and CD28 agonists, could promote the induction of Foxp3 in the presence of anti-CD3. Additionally, the TNF/TNFR2 interaction could also upregulate CD25 and PD-L1, the negative molecules on the surface of CD8+ Tregs, to mediate a contact-dependent inhibition to CD4+ and CD8+ effector T cells, cooperation with other negative molecules on the surface of CD8+ Tregs, such as CTLA-4.
TNFR2 Is a Better Functional Treg Cell Marker Than CD25 for CD8+Foxp3+ Tregs
CD8+Foxp3+ Tregs can be generated in vitro with anti-CD3 antibodies (17, 36, 37) or anti-CD3/28 beads (24). These cells expressed CD25, Foxp3, TNFR2, and the negative co-stimulatory receptors CTLA-4, PD-1, PDL-1, and Tim-3 (24). When CD8+ T cells were isolated from peripheral blood mononuclear cells (PBMCs) from healthy donors and cultured with anti-CD3 mAb for 5 days, the TNFR2+CD25+ cells were identified as the main subset that expressed Foxp3 (17). Similarly, human CD25 and TNFR2-coexpressing CD4+ Tregs were identified as a potent subpopulation of Tregs (22, 38–40). Interestingly, when these CD8+Tregs were sorted into four subsets, CD25+TNFR2+, CD25+TNFR2−, CD25−TNFR2+, and CD25−TNFR2−, to identify their respective ability to inhibit proliferation of target CD4+ Teffs, the results identified that both CD8+CD25+ and CD8+CD25− cells were more potent inhibitors of proliferation if they coexpressed TNFR2, suggesting that TNFR2 is a more important marker than CD25 on CD8+Foxp3+ Tregs (17). Additionally, in vitro-induced CD8+Foxp3+ Tregs expressed both TNFR2 and PDL-1. When sorting CD8+ T cells into TNFR2+PDL-1+, TNFR2+PDL-1−, TNFR2−PDL-1+, or TNFR2−PDL-1−, it was observed that TNFR2-PDL-1 double positive cells exhibited much stronger suppressive activity than control sham sorted cells. TNFR2 or PDL-1 single positive cells had modest suppressive activity, while the double negative cells had none (24). Once more, these data emphasized that TNFR2 might be a characteristic expression marker for functional CD8+Foxp3+ Tregs and the coexpression of TNFR2 and PDL-1 on CD8+Foxp3+ Tregs may represent cells with stronger suppressive activity.
TNF/TNFR2 Interaction Delivers a Co-Stimulatory Signal to Induce Foxp3 by CD8+Foxp3+ Tregs
It was shown that Foxp3 appears to function as a master regulator of the regulatory pathway in the development and function of Tregs (41–43). Interestingly, the TNF/TNFR2 interaction on the surface of CD8+ T cells could promote the induction of Foxp3 in the presence of anti-CD3/CD28 beads to generate more CD8+Foxp3+ Tregs. Previous studies have shown that when PBMCs from rheumatoid arthritis (RA) patients were cultured with anti-CD3 for 24 h, a greater percentage of CD8+Foxp3+ Tregs were generated and expressed high levels of CD25 and TNFR2 (44). However, when anti-TNF monoclonal antibodies (mAb) were added into the in vitro culture system, the percentage of Foxp3 expression on CD8+ Tregs decreased significantly (44). Furthermore, experimental results show that membrane TNF/TNFR2 interactions, in combination with CD80/CD28 interactions between monocytes and CD8+ T cells from RA patients, could also promote the induction of CD8+Foxp3+ Tregs in vitro, while combined CD86 and TNF blockade completely ablated the process (44). These data all indicated that the effect mediated by TNFR2 expression on CD8+ T cells played a prominent role for the generation of CD8+Foxp3+ Tregs in the presence of anti-CD3 in vitro. However, a defined mechanism remains elusive and the corresponding process in vivo remains to be studied.
TNF/TNFR2 Interactions Mediate the Suppressive Activity of CD8+Foxp3+ Tregs
Tumor necrosis factor was also found to be responsible for the induction of CD8+Foxp3+ Tregs, as anti-TNF monoclonal antibodies (mAb) could dramatically abrogate the proliferation of CD8+Foxp3+ Tregs, prevent the upregulation of CD25 in response to anti-CD3 in vitro on CD8+ Tregs, and interfere with the suppressive activity of CD8+Foxp3+ Tregs. Furthermore, TNFR2 expression was upregulated significantly after CD8+Foxp3+ Tregs were stimulated with anti-CD3 mAb in vitro, whereas the TNFR1 level was relatively low (17), indicating that the effect of TNF was more potent via TNFR2 to mediate the downstream signal. Additionally, TNF could upregulate PDL-1 expression on CD8+Foxp3+ Tregs via TNFR2 and which was greatly decreased by blocking with soluble TNF receptors (TNFR2-Fc) (17). Therefore, upregulating PDL-1 expressing on CD8+ Tregs might be a specific mechanism for TNF/TNFR2 mediating CD8+ Treg suppressive activation (45). Compared with TNFR2 expressed on CD4+ Tregs, little is known about the significance of TNFR2 on CD8+ Tregs. The available evidence indicates that TNFR2 expression on CD8+Foxp3+ Tregs is beneficial for their function, and defects in their suppressive function occurred when TNFR2 was neutralized. CD8+ Tregs have been shown to exhibit different phenotypes in different diseases, including viral infection (46), autoimmune diseases (47), graft-versus-host disease (GVHD) (48, 49), and cancer (44, 50). However, it is unclear whether TNFR2 can be used as a suppressive marker for all the reported CD8+ Treg subsets.
TNFR2 Expressed on CD8+ Effector T Cells
Studies on TNFR2 expressed on CD8+ Teffs are relatively more sufficient than studies on TNFR2 expressed on CD8+ Tregs (Figure 2). Numerous reports have shown that CD8+ Teffs are critical players involved in various immune responses (51–53). Efficient induction of CD8+ Teffs requires coordinated signaling through a number of pathways, including T cell receptor (TCR) ligation with peptide in the context of major histocompatibility complex class I (MHC I), costimulatory molecules, and cytokines(53). TNFR2, but not TNFR1, has been previously shown to be the predominant TNF receptor on activation CD8+ Teffs (54, 55). Thus, the direct effects of TNF on CD8+ Teffs are mainly mediated through TNFR2 (54, 55). Typically, T-cell-mediated immune responses can be divided into three parts: (1) antigen recognition; (2) proliferation and differentiation; and (3) activation-induced cell death (AICD). Once an activation signal has been received, primary CD8+ T cells undergo proliferation, expansion, and differentiation. It has been reported that TNFR2 expression was involved in CD8+ Teffs activation in certain phases of an immune response. For instance, it has been found that TNFR2 not only lowered the threshold for T cell activation, but also provided early costimulatory signals during T cell activation (56–58). Additionally, TNFR2 also plays a critical role in regulating AICD in activated CD8+ Teffs (59).
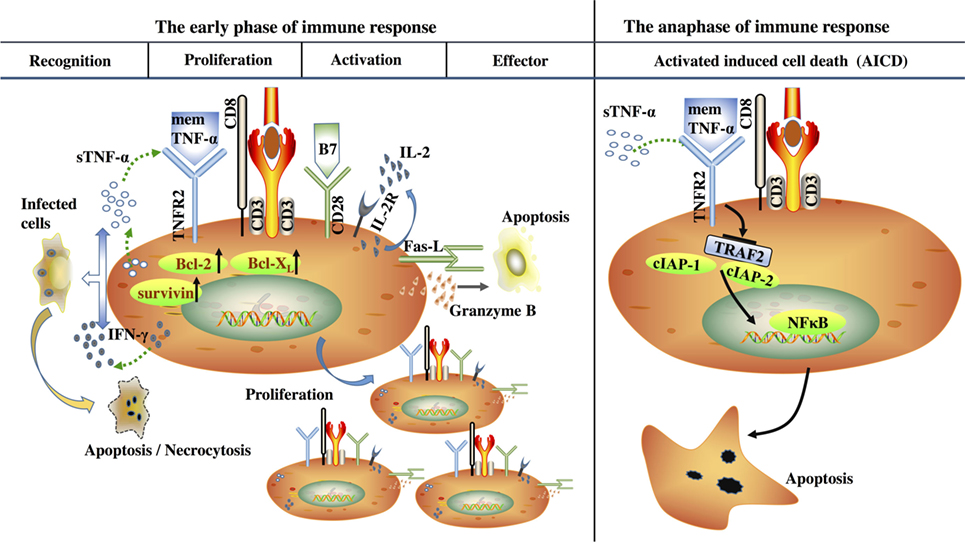
Figure 2. Tumor necrosis factor (TNF) receptor type II (TNFR2) modulates the process of immune response mediated by CD8+ effector T cells (Teffs). In the early phase of immune response, TNFR2 is a CD8+ Teff costimulatory molecule for IL-2, survivin, Bcl-2, and Bcl-xL induction to promote CD8+ Teffs survival, proliferation, and activation and involve in controlling the cell fate during TCR/CD28-mediated stimulation. TNFR2 is essential for the production of IFN-γ and TNF-α in CD8+ Teffs to promote antigen clearance. Additionally, TNFR2 is also required for CD8+ Teffs to upregulate Fas-L and granzyme B to enhance their cytotoxic activity. However, in the anaphase of immune response, TNFR2 can induce activated CD8+ T cells programmed cell death to terminate the immune response via the degradation of a pro-survival signal—TRAF2, which is required for the recruitment of cellular inhibitor of apoptosis proteins cIAP-1 and cIAP-2 to the TNFR2 signaling complex and activates nuclear factor-κB.
TNFR2 as an Activator Molecule in the Early Phase of Immune Response
TNFR2 Is Required for Primary CD8+ T Cell Survival and Proliferation
CD28 is a key costimulatory molecule for IL-2 induction, based on its ability to substantially augment expression in T cells stimulated via the TCR (60). However, the effects mediated by CD28 were found to be insufficient to sustain long-term T cell survival (61). Nevertheless, TNFR2 plays a critical role in promoting activation and survival of naive T cells during a primary response (5, 62). CD8+ T cells deficient in TNFR2 possessed a marked defect in IL-2 production, a critical T cell growth factor (63, 64), resulting in a decreased proliferative response (57), suggesting that TNFR2 is a CD8+ T cell costimulatory molecule involved in controlling the cell fate during TCR/CD28-mediated stimulation (57). Additionally, TNFR2 deficiency in CD8+ T cells increased the requirements for a TCR agonist, approximately fivefold to achieve a proliferative response equivalent to wild-type CD8+ T cells in several infection models (5, 56–58). Additionally, in a mouse tumor model, the proportion of proliferating transgenic tumor-specific CD8+ T cells in TNFR2 deficient mice were significant reduced in tumor-draining lymph nodes (54). These data indicated that TNFR2 sustained the early proliferative phase during CD8+ T cell cells activation. Moreover, during CD8+ T cell activation in response to antigen in vitro, TNFR2 deficiency was related to a reduction of anti-apoptotic molecules, such as survivin, Bcl-2, and Bcl-xL (57, 58), indicating the critical roles of TNFR2 in CD8+ T cell survival.
TNFR2 Is Required for the Secretion of Effector Molecules by CD8+ Teffs
One of the key effector functions of activated CD8+ T cells is the ability to produce antiviral and pro-inflammatory cytokines, including interferon (IFN)-γ and TNF-α (65). Typically, cytokine production by antiviral CD8+ T cells occurs in a hierarchical fashion, with the majority producing IFN-γ, and a subset of those producing TNF-α (66–68). During infection, such as respiratory influenza or C. muridarum infection, the production of IFN-γ was significantly decreased in TNFR2−/−CD8+ T cell, with significantly delayed antigen clearance in TNFR2−/− mice (69, 70). These results suggest that TNFR2 primarily promotes the activation of CD8+ T cells and enhances the ability of CD8+ T cells to clear antigen. When tumor-special CD8+ T cells, isolated from TNFR2−/− mice, TNFR1−/−, or wild-type mice, were cultured with specific antigens in vitro, IFN-γ levels produced by TNFR2−/−CD8+ T cells was less than TNFR1−/− or wild-type CD8+ T cells (54), indicating that TNFR2 was also necessary for the optimal production of IFN-γ to clear tumor antigens during the T cell activation phase.
Second, TNF-α is increased during CD8+ T cell activation following antigenic stimulation (71, 72). Similar to IFN-γ, TNF-α is critically required for efficient CD8+ T cell-mediated responses from initiation to pathogen clearance. However, TNF-α levels produced by CD8+ T cells were not always in line with INF-γ production. During colitis, CD8+ T cells from TNFR2−/− mice expressed significantly higher levels of TNF-α compared with wild-type mice, which was sufficient to worsen colonic inflammation (73). Similarly, after intranasal challenge with HKx31 influenza A virus, TNF-α production was also increased in TNFR2−/− mice, compared with wild-type mice (73). It is possible that the increased TNF-α in TNFR2 deficient mice may be due to a negative feedback loop in the TNF-TNFR2 signaling (5, 59, 62, 73).
TNFR2 Is Required for CD8+ T Memory Cells Recovery
After encountering with microbial antigen, T cells can differentiate into memory cells to provide long-lasting protection against subsequent pathogens (18, 74, 75). During transplantation, microbe-elicited T memory cells can also cross-react with allogeneic antigen and mediate graft rejection, a process termed allogeneic heterologous immunity. TCR affinity is hypothesized to be critically important in the context of allogeneic heterologous immunity (18, 76, 77). Notably, TNFR2 plays an important role for low-affinity-primed memory CD8+ T cells mediating optimum recall responses. During heterologous rechallenge, low-affinity-primed memory effectors upregulated TNFR2 surface expression to mediated graft rejection, whereas blockade of TNFR2 significantly attenuated graft rejection and prolonged graft survival (18). These data indicated that TNFR2 is required and critical for memory CD8+ T cells recovery in immune responses.
TNFR2 Is Required for Cytotoxic T Lymphocyte (CTL) Activity
Granzyme B is a serine protease expressed by CTL and together with the pore forming protein, perforin, mediates apoptosis in target cells (78). Notably, TNFR2 engagement with TNF-α induces the expression of granzyme B in CD8+ T cells, when costimulation with CD86 is provided simultaneously. TNFR2 was also shown to be upregulated on granzyme B+CD8+ T cells in aging mice and humans (79), indicating that the TNF/TNFR2 signaling pathway in CD8+ T cell could reinforce the cells’ cytotoxic activity to induce target cells apoptosis via the release of granzyme B.
A second way for CTL to induce apoptosis is via cell-surface Fas–Fas ligand (FasL) interactions between CTL and infected cells. FasL is expressed predominantly on activated lymphocytes and is able to induce programmed cell death in most Fas-expressing cells (80, 81). The number of FasL-expressing CD8+ intrahepatic lymphocytes isolated from various strains of hepatic adenovirus-infected TNFR2−/− mice were found to be significantly reduced compared with wild-type mice (82). Furthermore, TNFR2−/− intrahepatic lymphocytes were significantly less efficient in killing adenovirus-infected hepatocyte target cells than intrahepatic lymphocytes obtained from adenovirus-infected wild-type mice (82). These data provide evidence suggesting that TNFR2 can potentiate FasL-mediated cytotoxicity for CD8+ Teffs.
TNFR2 Is as an Apoptosis Signal on Activated CD8+ Teffs
Tumor necrosis factor receptor type II is essential for both optimal proliferation during CD8+ T cell activation and for the induction of AICD that terminates the proliferative response (59). Previous study had shown that TNFR2−/−CD8+ T cells exhibited consistently high resistance to AICD, leading to worsen colonic inflammation (73), indicating that TNFR2 is a critical negative regulator of activated CD8+ T cells by promoting AICD to terminate the immune response. Moreover, TNFR2 signaling was reported to lead to the degradation of TNF receptor-associated factor 2 (TRAF2) (83), which were important in the regulation of the receptor signaling (83–86). Notably, TRAF2 is known as a pro-survival signal (87), which is required for the recruitment of cellular inhibitor of apoptosis proteins (cIAP)-1 and -2 to the TNFR2 signaling complex (88) and activates nuclear factor (NF)-κB to mediate its anti-apoptotic effects (89–91). The overexpression of TRAF2 in wild-type CD8+ T cells did not affect the percentage of apoptotic cells, whereas the silencing of TRAF2 in activated TNFR2−/−CD8+ T cells could render them as sensitive to AICD as activated wild-type CD8+ T cells (59). Collectively, these results provide evidence that the TNFR2 signaling pathway is involved in regulating AICD and that TRAF2 depletion induced by TNFR2 is critical to this process.
Conclusion
Tumor necrosis factor receptor type II is an attractive molecular marker to identify both CD8+Foxp3+ Tregs and CD8+ Teffs. For CD8+Foxp3+ Tregs, TNFR2 is necessary for the induction of Foxp3 and regarded as a functional marker of their suppressive ability. For CD8+ Teffs, TNFR2 serves as an activator for proliferation and cytotoxic ability in the early stage of an immune response and as an apoptosis signal for activated CD8+ Teffs to terminate the immune response. Both CD8+Foxp3+ Tregs and CD8+ Teffs could express high levels of TNFR2 and were involved in various diseases. It is noteworthy that there is an antagonistic relationship between CD8+ Tregs and CD8+ Teffs. The TNF-TNFR2 signaling pathway potentially activates both of them, so targeting TNFR2 may impair the function of protective Tregs or Teffs as a side effect in the treatment of diseases (4). Furthermore, studies have shown that TNFR2 is a potential therapeutic target with remarkable success in cancer immunotherapy. A TNFR2 antagonists could specifically inhibit CD4+Foxp3+ Tregs expansion in the tumor microenvironment, whereas it had little inhibitory effects on CD4+ Tregs in periphery or from healthy donors, and killed human ovarian tumor cells directly. However, little is known about TNFR2 agonists or antagonists aimed at altering TNFR2 expression on tumor-associated CD8+ Tregs and CD8+ Teffs. Further understanding of TNFR2 expression on CD8+ T cells and the pathways that are active and important in different disease-related microenvironments will provide better understanding of its impacts on TNF-mediated pathology, and may help in the development of more effective targeted therapeutics.
Furthermore, recent evidence indicated that the relationship between TNF/TNFR2 and T cell responses is complex and, at times, paradoxical. There is controversy to the specific effects of TNF on different T cell subsets (92). The explanation for such contradictory outcomes may lay in how downstream signaling pathways are activated and drive disease (92). Consequently, a precise understanding of the level and/or ratio of TNFR2 expressed on different T cell subsets will help in the use of TNFR2 agonists or antagonists as therapies.
Author Contributions
L-LY and QZ contributed to the design and writing of this review. X-SW, MZ, and Y-RN contributed to collection of references.
Conflict of Interest Statement
The authors declare that the research was conducted in the absence of any commercial or financial relationships that could be construed as a potential conflict of interest.
Funding
This work was supported by grants from National Natural Science Foundation of China (No. 81770090 and 81470274).
References
1. Aggarwal BB, Gupta SC, Kim JH. Historical perspectives on tumor necrosis factor and its superfamily: 25 years later, a golden journey. Blood (2012) 119(3):651–65. doi:10.1182/blood-2011
2. Faustman DL, Davis M. TNF receptor 2 and disease: autoimmunity and regenerative medicine. Front Immunol (2013) 4:478. doi:10.3389/fimmu.2013.00478
3. Chen X, Wu X, Zhou Q, Howard OM, Netea MG, Oppenheim JJ. TNFR2 is critical for the stabilization of the CD4+Foxp3+ regulatory T cell phenotype in the inflammatory environment. J Immunol (2013) 190(3):1076–84. doi:10.4049/jimmunol.1202659
4. Chen X, Nie Y, Xiao H, Bian Z, Scarzello AJ, Song NY, et al. TNFR2 expression by CD4 effector T cells is required to induce full-fledged experimental colitis. Sci Rep (2016) 6:32834. doi:10.1038/srep32834
5. Kim EY, Teh SJ, Yang J, Chow MT, Teh HS. TNFR2-deficient memory CD8 T cells provide superior protection against tumor cell growth. J Immunol (2009) 183(10):6051–7. doi:10.4049/jimmunol.0803482
6. Siegmund D, Kums J, Ehrenschwender M, Wajant H. Activation of TNFR2 sensitizes macrophages for TNFR1-mediated necroptosis. Cell Death Dis (2016) 7(9):e2375. doi:10.1038/cddis.2016.285
7. Tanimura Y, Kokuryo T, Tsunoda N, Yamazaki Y, Oda K, Nimura Y, et al. Tumor necrosis factor alpha promotes invasiveness of cholangiocarcinoma cells via its receptor, TNFR2. Cancer Lett (2005) 219(2):205–13. doi:10.1016/j.canlet.2004.07.027
8. Chen YJ, Chang LS. Arecoline-induced death of human leukemia K562 cells is associated with surface up-modulation of TNFR2. J Cell Physiol (2012) 227(5):2240–51. doi:10.1002/jcp.22963
9. Ungewickell A, Bhaduri A, Rios E, Reuter J, Lee CS, Mah A, et al. Genomic analysis of mycosis fungoides and Sezary syndrome identifies recurrent alterations in TNFR2. Nat Genet (2015) 47(9):1056–60. doi:10.1038/ng.3370
10. Nakayama S, Yokote T, Tsuji M, Akioka T, Miyoshi T, Hirata Y, et al. Expression of tumour necrosis factor-alpha and its receptors in Hodgkin lymphoma. Br J Haematol (2014) 167(4):574–7. doi:10.1111/bjh.13015
11. Wang J, Al-Lamki RS. Tumor necrosis factor receptor 2: its contribution to acute cellular rejection and clear cell renal carcinoma. Biomed Res Int (2013) 2013:821310. doi:10.1155/2013/821310
12. Rauert H, Stuhmer T, Bargou R, Wajant H, Siegmund D. TNFR1 and TNFR2 regulate the extrinsic apoptotic pathway in myeloma cells by multiple mechanisms. Cell Death Dis (2011) 2:e194. doi:10.1038/cddis.2011.78
13. Uhlen M, Bjorling E, Agaton C, Szigyarto CA, Amini B, Andersen E, et al. A human protein atlas for normal and cancer tissues based on antibody proteomics. Mol Cell Proteomics (2005) 4(12):1920–32. doi:10.1074/mcp.M500279-MCP200
14. Brockhaus M, Schoenfeld HJ, Schlaeger EJ, Hunziker W, Lesslauer W, Loetscher H. Identification of two types of tumor necrosis factor receptors on human cell lines by monoclonal antibodies. Proc Natl Acad Sci U S A (1990) 87(8):3127–31. doi:10.1073/pnas.87.8.3127
15. Wortzman ME, Clouthier DL, McPherson AJ, Lin GH, Watts TH. The contextual role of TNFR family members in CD8(+) T-cell control of viral infections. Immunol Rev (2013) 255(1):125–48. doi:10.1111/imr.12086
16. Govindaraj C, Scalzo-Inguanti K, Scholzen A, Li S, Plebanski M. TNFR2 expression on CD25(hi)FOXP3(+) T cells induced upon TCR stimulation of CD4 T cells identifies maximal cytokine-producing effectors. Front Immunol (2013) 4:233. doi:10.3389/fimmu.2013.00233
17. Ablamunits V, Bisikirska B, Herold KC. Acquisition of regulatory function by human CD8(+) T cells treated with anti-CD3 antibody requires TNF. Eur J Immunol (2010) 40(10):2891–901. doi:10.1002/eji.201040485
18. Krummey SM, Chen CW, Guasch SA, Liu D, Wagener M, Larsen CP, et al. Enhanced requirement for TNFR2 in graft rejection mediated by low-affinity memory CD8+ T cells during heterologous immunity. J Immunol (2016) 197(5):2009–15. doi:10.4049/jimmunol.1502680
19. Nguyen DX, Ehrenstein MR. Anti-TNF drives regulatory T cell expansion by paradoxically promoting membrane TNF-TNF-RII binding in rheumatoid arthritis. J Exp Med (2016) 213(7):1241–53. doi:10.1084/jem.20151255
20. Chen X, Oppenheim JJ. Targeting TNFR2, an immune checkpoint stimulator and oncoprotein, is a promising treatment for cancer. Sci Signal (2017) 10(462):eaal2328. doi:10.1126/scisignal.aal2328
21. Chopra M, Biehl M, Steinfatt T, Brandl A, Kums J, Amich J, et al. Exogenous TNFR2 activation protects from acute GvHD via host T reg cell expansion. J Exp Med (2016) 213(9):1881–900. doi:10.1084/jem.20151563
22. Chen X, Subleski JJ, Kopf H, Howard OM, Mannel DN, Oppenheim JJ. Cutting edge: expression of TNFR2 defines a maximally suppressive subset of mouse CD4+CD25+FoxP3+ T regulatory cells: applicability to tumor-infiltrating T regulatory cells. J Immunol (2008) 180(10):6467–71. doi:10.4049/jimmunol.180.10.6467
23. Cosmi L, Liotta F, Lazzeri E, Francalanci M, Angeli R, Mazzinghi B, et al. Human CD8+CD25+ thymocytes share phenotypic and functional features with CD4+CD25+ regulatory thymocytes. Blood (2003) 102(12):4107–14. doi:10.1182/blood-2003-04-1320
24. Horwitz DA, Pan S, Ou JN, Wang J, Chen M, Gray JD, et al. Therapeutic polyclonal human CD8+ CD25+ Fox3+ TNFR2+ PD-L1+ regulatory cells induced ex-vivo. Clin Immunol (2013) 149(3):450–63. doi:10.1016/j.clim.2013.08.007
25. Torrey H, Butterworth J, Mera T, Okubo Y, Wang L, Baum D, et al. Targeting TNFR2 with antagonistic antibodies inhibits proliferation of ovarian cancer cells and tumor-associated Tregs. Sci Signal (2017) 10(462):eaaf8608. doi:10.1126/scisignal.aaf8608
26. Feldmann M, Beverley PC, Dunkley M, Kontiainen S. Different Ly antigen phenotypes of in vitro induced helper and suppressor cells. Nature (1975) 258(5536):614–6. doi:10.1038/258614a0
27. Cantor H, Shen FW, Boyse EA. Separation of helper T cells from suppressor T cells expressing different Ly components. II. Activation by antigen: after immunization, antigen-specific suppressor and helper activities are mediated by distinct T-cell subclasses. J Exp Med (1976) 143(6):1340–91. doi:10.1084/jem.143.6.1391
28. Kapp JA, Bucy RP. CD8+ suppressor T cells resurrected. Hum Immunol (2008) 69(11):715–20. doi:10.1016/j.humimm.2008.07.018
29. Chen X, Oppenheim JJ. The phenotypic and functional consequences of tumour necrosis factor receptor type 2 expression on CD4(+) FoxP3(+) regulatory T cells. Immunology (2011) 133(4):426–33. doi:10.1111/j.1365-2567.2011.03460.x
30. Tsai S, Clemente-Casares X, Santamaria P. CD8(+) Tregs in autoimmunity: learning “self”-control from experience. Cell Mol Life Sci (2011) 68(23):3781–95. doi:10.1007/s00018-011-0738-y
31. Rifa’I M, Shi Z, Zhang SY, Lee YH, Shiku H, Isobe K, et al. CD8+CD122+ regulatory T cells recognize activated T cells via conventional MHC class I-alphabetaTCR interaction and become IL-10-producing active regulatory cells. Int Immunol (2008) 20(7):937–47. doi:10.1093/intimm/dxn052
32. Colovai AI, Liu Z, Ciubotariu R, Lederman S, Cortesini R, Suciu-Foca N. Induction of xenoreactive CD4+ T-cell anergy by suppressor CD8+CD28- T cells. Transplantation (2000) 69(7):1304–10. doi:10.1097/00007890-200004150-00016
33. Liu Z, Tugulea S, Cortesini R, Suciu-Foca N. Specific suppression of T helper alloreactivity by allo-MHC class I-restricted CD8+CD28- T cells. Int Immunol (1998) 10(6):775–83. doi:10.1093/intimm/10.6.775
34. Koch SD, Uss E, van Lier RA, Ten BI. Alloantigen-induced regulatory CD8+CD103+ T cells. Hum Immunol (2008) 69(11):737–44. doi:10.1016/j.humimm.2008.08.281
35. Lu L, Yu Y, Li G, Pu L, Zhang F, Zheng S, et al. CD8(+)CD103(+) regulatory T cells in spontaneous tolerance of liver allografts. Int Immunopharmacol (2009) 9(5):546–8. doi:10.1016/j.intimp.2009.01.021
36. Bisikirska B, Colgan J, Luban J, Bluestone JA, Herold KC. TCR stimulation with modified anti-CD3 mAb expands CD8+ T cell population and induces CD8+CD25+ Tregs. J Clin Invest (2005) 115(10):2904–13. doi:10.1172/JCI23961
37. Ablamunits V, Henegariu O, Preston-Hurlburt P, Herold KC. NKG2A is a marker for acquisition of regulatory function by human CD8+ T cells activated with anti-CD3 antibody. Eur J Immunol (2011) 41(7):1832–42. doi:10.1002/eji.201041258
38. Chen X, Baumel M, Mannel DN, Howard OM, Oppenheim JJ. Interaction of TNF with TNF receptor type 2 promotes expansion and function of mouse CD4+CD25+ T regulatory cells. J Immunol (2007) 179(1):154–61. doi:10.4049/jimmunol.179.1.154
39. Chen X, Subleski JJ, Hamano R, Howard OM, Wiltrout RH, Oppenheim JJ. Co-expression of TNFR2 and CD25 identifies more of the functional CD4+FOXP3+ regulatory T cells in human peripheral blood. Eur J Immunol (2010) 40(4):1099–106. doi:10.1002/eji.200940022
40. Chen X, Oppenheim JJ. TNF-alpha: an activator of CD4+FoxP3+TNFR2+ regulatory T cells. Curr Dir Autoimmun (2010) 11:119–34. doi:10.1159/000289201
41. Hori S, Nomura T, Sakaguchi S. Control of regulatory T cell development by the transcription factor Foxp3. Science (2003) 299(5609):1057–61. doi:10.1126/science.1079490
42. Fontenot JD, Gavin MA, Rudensky AY. Foxp3 programs the development and function of CD4+CD25+ regulatory T cells. Nat Immunol (2003) 4(4):330–6. doi:10.1038/ni904
43. Fontenot JD, Rasmussen JP, Williams LM, Dooley JL, Farr AG, Rudensky AY. Regulatory T cell lineage specification by the forkhead transcription factor foxp3. Immunity (2005) 22(3):329–41. doi:10.1016/j.immuni.2005.01.016
44. Chakraborty S, Panda AK, Bose S, Roy D, Kajal K, Guha D, et al. Transcriptional regulation of FOXP3 requires integrated activation of both promoter and CNS regions in tumor-induced CD8(+) Treg cells. Sci Rep (2017) 7(1):1628. doi:10.1038/s41598-017-01788-z
45. Ou JN, Wiedeman AE, Stevens AM. TNF-alpha and TGF-beta counter-regulate PD-L1 expression on monocytes in systemic lupus erythematosus. Sci Rep (2012) 2:295. doi:10.1038/srep00295
46. Peligero C, Argilaguet J, Guerri-Fernandez R, Torres B, Ligero C, Colomer P, et al. PD-L1 blockade differentially impacts regulatory T cells from HIV-infected individuals depending on plasma viremia. PLoS Pathog (2015) 11(12):e1005270. doi:10.1371/journal.ppat.1005270
47. Berger CT, Hess C. Neglected for too long? CD8+ Tregs release NOX2-loaded vesicles to inhibit CD4+ T cells. J Clin Invest (2016) 126(5):1646–8. doi:10.1172/JCI87429
48. Le Guen V, Judor JP, Boeffard F, Gauttier V, Ferry N, Soulillou JP, et al. Alloantigen gene transfer to hepatocytes promotes tolerance to pancreatic islet graft by inducing CD8(+) regulatory T cells. J Hepatol (2017) 66(4):765–77. doi:10.1016/j.jhep.2016.11.019
49. Gutierrez-Hoya A, Lopez-Santiago R, Vela-Ojeda J, Montiel-Cervantes L, Rodriguez-Cortes O, Rosales-Garcia V, et al. Role of CD8 regulatory T cells versus Tc1 and Tc17 cells in the development of human graft-versus-host disease. J Immunol Res (2017) 2017:1236219. doi:10.1155/2017/1236219
50. Haymaker CL, Kim D, Uemura M, Vence LM, Phillip A, McQuail N, et al. Metastatic melanoma patient had a complete response with clonal expansion after whole brain radiation and PD-1 blockade. Cancer Immunol Res (2017) 5(2):100–5. doi:10.1158/2326-6066.CIR-16-0223
51. Zheng J, Liu Y, Liu Y, Liu M, Xiang Z, Lam KT, et al. Human CD8+ regulatory T cells inhibit GVHD and preserve general immunity in humanized mice. Sci Transl Med (2013) 5(168):168r–9r. doi:10.1126/scitranslmed.3004943
52. Karimi MA, Bryson JL, Richman LP, Fesnak AD, Leichner TM, Satake A, et al. NKG2D expression by CD8+ T cells contributes to GVHD and GVT effects in a murine model of allogeneic HSCT. Blood (2015) 125(23):3655–63. doi:10.1182/blood-2015-02-629006
53. Petrelli A, van Wijk F. CD8(+) T cells in human autoimmune arthritis: the unusual suspects. Nat Rev Rheumatol (2016) 12(7):421–8. doi:10.1038/nrrheum.2016.74
54. Calzascia T, Pellegrini M, Hall H, Sabbagh L, Ono N, Elford AR, et al. TNF-alpha is critical for antitumor but not antiviral T cell immunity in mice. J Clin Invest (2007) 117(12):3833–45. doi:10.1172/JCI32567
55. Zheng L, Fisher G, Miller RE, Peschon J, Lynch DH, Lenardo MJ. Induction of apoptosis in mature T cells by tumour necrosis factor. Nature (1995) 377(6547):348–51. doi:10.1038/377348a0
56. Kim EY, Teh HS. TNF type 2 receptor (p75) lowers the threshold of T cell activation. J Immunol (2001) 167(12):6812–20. doi:10.4049/jimmunol.167.12.6812
57. Kim EY, Teh HS. Critical role of TNF receptor type-2 (p75) as a costimulator for IL-2 induction and T cell survival: a functional link to CD28. J Immunol (2004) 173(7):4500–9. doi:10.4049/jimmunol.173.7.4500
58. Kim EY, Priatel JJ, Teh SJ, Teh HS. TNF receptor type 2 (p75) functions as a costimulator for antigen-driven T cell responses in vivo. J Immunol (2006) 176(2):1026–35. doi:10.4049/jimmunol.176.2.1026
59. Twu YC, Gold MR, Teh HS. TNFR1 delivers pro-survival signals that are required for limiting TNFR2-dependent activation-induced cell death (AICD) in CD8+ T cells. Eur J Immunol (2011) 41(2):335–44. doi:10.1002/eji.201040639
60. Fraser JD, Irving BA, Crabtree GR, Weiss A. Regulation of interleukin-2 gene enhancer activity by the T cell accessory molecule CD28. Science (1991) 251(4991):313–6. doi:10.1126/science.1846244
61. Song J, Salek-Ardakani S, Rogers PR, Cheng M, Van Parijs L, Croft M. The costimulation-regulated duration of PKB activation controls T cell longevity. Nat Immunol (2004) 5(2):150–8. doi:10.1038/ni1030
62. Teh HS, Seebaran A, Teh SJ. TNF receptor 2-deficient CD8 T cells are resistant to Fas/Fas ligand-induced cell death. J Immunol (2000) 165(9):4814–21. doi:10.4049/jimmunol.165.9.4814
63. Rudd CE. Upstream-downstream: CD28 cosignaling pathways and T cell function. Immunity (1996) 4(6):527–34. doi:10.1016/S1074-7613(00)80479-3
64. Abbas AK. The control of T cell activation vs. tolerance. Autoimmun Rev (2003) 2(3):115–8. doi:10.1016/S1568-9972(03)00028-4
65. Leist TP, Eppler M, Zinkernagel RM. Enhanced virus replication and inhibition of lymphocytic choriomeningitis virus disease in anti-gamma interferon-treated mice. J Virol (1989) 63(6):2813–9.
66. Almeida JR, Price DA, Papagno L, Arkoub ZA, Sauce D, Bornstein E, et al. Superior control of HIV-1 replication by CD8+ T cells is reflected by their avidity, polyfunctionality, and clonal turnover. J Exp Med (2007) 204(10):2473–85. doi:10.1084/jem.20070784
67. Darrah PA, Patel DT, De Luca PM, Lindsay RW, Davey DF, Flynn BJ, et al. Multifunctional TH1 cells define a correlate of vaccine-mediated protection against Leishmania major. Nat Med (2007) 13(7):843–50. doi:10.1038/nm1592
68. Seder RA, Darrah PA, Roederer M. T-cell quality in memory and protection: implications for vaccine design. Nat Rev Immunol (2008) 8(4):247–58. doi:10.1038/nri2274
69. Wortzman ME, Lin GH, Watts TH. Intrinsic TNF/TNFR2 interactions fine-tune the CD8 T cell response to respiratory influenza virus infection in mice. PLoS One (2013) 8(7):e68911. doi:10.1371/journal.pone.0068911
70. Manam S, Thomas JD, Li W, Maladore A, Schripsema JH, Ramsey KH, et al. Tumor necrosis factor (TNF) receptor superfamily member 1b on CD8+ T cells and TNF receptor superfamily member 1a on non-CD8+ T cells contribute significantly to upper genital tract pathology following chlamydial infection. J Infect Dis (2015) 211(12):2014–22. doi:10.1093/infdis/jiu839
71. White DW, Badovinac VP, Kollias G, Harty JT. Cutting edge: antilisterial activity of CD8+ T cells derived from TNF-deficient and TNF/perforin double-deficient mice. J Immunol (2000) 165(1):5–9. doi:10.4049/jimmunol.165.1.5
72. Brehm MA, Daniels KA, Welsh RM. Rapid production of TNF-alpha following TCR engagement of naive CD8 T cells. J Immunol (2005) 175(8):5043–9. doi:10.4049/jimmunol.175.8.5043
73. Punit S, Dube PE, Liu CY, Girish N, Washington MK, Polk DB. Tumor necrosis factor receptor 2 restricts the pathogenicity of CD8(+) T cells in mice with colitis. Gastroenterology (2015) 149(4):993–1005. doi:10.1053/j.gastro.2015.06.004
74. Kaech SM, Cui W. Transcriptional control of effector and memory CD8+ T cell differentiation. Nat Rev Immunol (2012) 12(11):749–61. doi:10.1038/nri3307
75. Jameson SC, Masopust D. Diversity in T cell memory: an embarrassment of riches. Immunity (2009) 31(6):859–71. doi:10.1016/j.immuni.2009.11.007
76. Adams AB, Williams MA, Jones TR, Shirasugi N, Durham MM, Kaech SM, et al. Heterologous immunity provides a potent barrier to transplantation tolerance. J Clin Invest (2003) 111(12):1887–95. doi:10.1172/JCI17477
77. Ai L, Xu A, Teng T, Niu J, Sun H, Qi M. Compound semiconductor nanotube materials grown and fabricated. Nanoscale Res Lett (2011) 6(1):627. doi:10.1186/1556-276X-6-627
78. Afonina IS, Cullen SP, Martin SJ. Cytotoxic and non-cytotoxic roles of the CTL/NK protease granzyme B. Immunol Rev (2010) 235(1):105–16. doi:10.1111/j.0105-2896.2010.00908.x
79. Lee-Chang C, Bodogai M, Moritoh K, Chen X, Wersto R, Sen R, et al. Aging converts innate B1a cells into potent CD8+ T cell inducers. J Immunol (2016) 196(8):3385–97. doi:10.4049/jimmunol.1502034
80. Krammer PH. CD95(APO-1/Fas)-mediated apoptosis: live and let die. Adv Immunol (1999) 71:163–210.
81. Lynch DH. The role of FasL and TNF in the homeostatic regulation of immune responses. Adv Exp Med Biol (1996) 406:135–8. doi:10.1007/978-1-4899-0274-0_14
82. Kafrouni MI, Brown GR, Thiele DL. The role of TNF-TNFR2 interactions in generation of CTL responses and clearance of hepatic adenovirus infection. J Leukoc Biol (2003) 74(4):564–71. doi:10.1189/jlb.0103035
83. Fotin-Mleczek M, Henkler F, Samel D, Reichwein M, Hausser A, Parmryd I, et al. Apoptotic crosstalk of TNF receptors: TNF-R2-induces depletion of TRAF2 and IAP proteins and accelerates TNF-R1-dependent activation of caspase-8. J Cell Sci (2002) 115:2750–70.
84. Rodriguez M, Cabal-Hierro L, Carcedo MT, Iglesias JM, Artime N, Darnay BG, et al. NF-kappaB signal triggering and termination by tumor necrosis factor receptor 2. J Biol Chem (2011) 286(26):22814–24. doi:10.1074/jbc.M111.225631
85. Cabal-Hierro L, Artime N, Iglesias J, Prado MA, Ugarte-Gil L, Casado P, et al. A TRAF2 binding independent region of TNFR2 is responsible for TRAF2 depletion and enhancement of cytotoxicity driven by TNFR1. Oncotarget (2014) 5(1):224–36. doi:10.18632/oncotarget.1492
86. Cabal-Hierro L, Rodriguez M, Artime N, Iglesias J, Ugarte L, Prado MA, et al. TRAF-mediated modulation of NF-kB AND JNK activation by TNFR2. Cell Signal (2014) 26(12):2658–66. doi:10.1016/j.cellsig.2014.08.011
87. Micheau O, Tschopp J. Induction of TNF receptor I-mediated apoptosis via two sequential signaling complexes. Cell (2003) 114(2):181–90. doi:10.1016/S0092-8674(03)00521-X
88. Rothe M, Pan MG, Henzel WJ, Ayres TM, Goeddel DV. The TNFR2-TRAF signaling complex contains two novel proteins related to baculoviral inhibitor of apoptosis proteins. Cell (1995) 83(7):1243–52. doi:10.1016/0092-8674(95)90149-3
89. Reinhard C, Shamoon B, Shyamala V, Williams LT. Tumor necrosis factor alpha-induced activation of c-jun N-terminal kinase is mediated by TRAF2. EMBO J (1997) 16(5):1080–92. doi:10.1093/emboj/16.5.1080
90. Liu ZG, Hsu H, Goeddel DV, Karin M. Dissection of TNF receptor 1 effector functions: JNK activation is not linked to apoptosis while NF-kappaB activation prevents cell death. Cell (1996) 87(3):565–76. doi:10.1016/S0092-8674(00)81375-6
91. Eleouet JF, Romeo PH. CCACC-binding or simian-virus-40-protein-1-binding proteins cooperate with human GATA-1 to direct erythroid-specific transcription and to mediate 5’ hypersensitive site 2 sensitivity of a TATA-less promoter. Eur J Biochem (1993) 212(3):763–70. doi:10.1111/j.1432-1033.1993.tb17716.x
Keywords: tumor necrosis factor, tumor necrosis factor receptor type II, CD8+ regulatory T cells, CD8+ effector T cells, CD4+ regulatory T cells
Citation: Ye L-L, Wei X-S, Zhang M, Niu Y-R and Zhou Q (2018) The Significance of Tumor Necrosis Factor Receptor Type II in CD8+ Regulatory T Cells and CD8+ Effector T Cells. Front. Immunol. 9:583. doi: 10.3389/fimmu.2018.00583
Received: 31 December 2017; Accepted: 08 March 2018;
Published: 22 March 2018
Edited by:
Xin Chen, University of Macau, ChinaReviewed by:
Haruhiko Suzuki, Nagoya University Graduate School of Medicine, JapanMuriel Moser, Université libre de Bruxelles, Belgium
Copyright: © 2018 Ye, Wei, Zhang, Niu and Zhou. This is an open-access article distributed under the terms of the Creative Commons Attribution License (CC BY). The use, distribution or reproduction in other forums is permitted, provided the original author(s) and the copyright owner are credited and that the original publication in this journal is cited, in accordance with accepted academic practice. No use, distribution or reproduction is permitted which does not comply with these terms.
*Correspondence: Qiong Zhou, zhouqiongtj@126.com