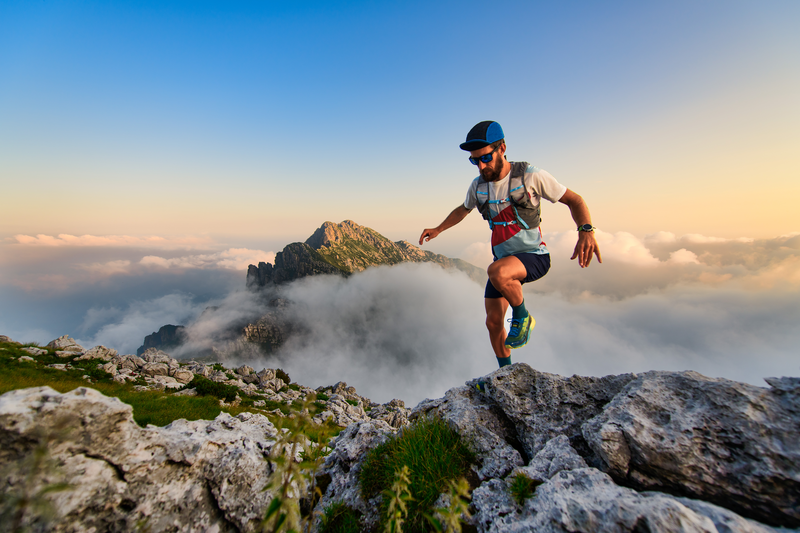
94% of researchers rate our articles as excellent or good
Learn more about the work of our research integrity team to safeguard the quality of each article we publish.
Find out more
MINI REVIEW article
Front. Immunol. , 01 March 2018
Sec. Alloimmunity and Transplantation
Volume 9 - 2018 | https://doi.org/10.3389/fimmu.2018.00389
Cytomegalovirus (CMV) infection can cause significant complications after transplantation, but recent emerging data suggest that CMV may paradoxically also exert beneficial effects in two specific allogeneic transplant settings. These potential benefits have been underappreciated and are therefore highlighted in this review. First, after allogeneic hematopoietic stem cell transplantation (HSCT) for acute myeloid leukemia (AML) using T-cell and natural killer (NK) cell-replete grafts, CMV reactivation is associated with protection from leukemic relapse. This association was not observed for other hematologic malignancies. This anti-leukemic effect might be mediated by CMV-driven expansion of donor-derived memory-like NKG2C+ NK and Vδ2negγδ T-cells. Donor-derived NK cells probably recognize recipient leukemic blasts by engagement of NKG2C with HLA-E and/or by the lack of donor (self) HLA molecules. Vδ2negγδ T cells probably recognize as yet unidentified antigens on leukemic blasts via their TCR. Second, immunological imprints of CMV infection, such as expanded numbers of Vδ2negγδ T cells and terminally differentiated TCRαβ+ T cells, as well as enhanced NKG2C gene expression in peripheral blood of operationally tolerant liver transplant patients, suggest that CMV infection or reactivation may be associated with liver graft acceptance. Mechanistically, poor alloreactivity of CMV-induced terminally differentiated TCRαβ+ T cells and CMV-induced IFN-driven adaptive immune resistance mechanisms in liver grafts may be involved. In conclusion, direct associations indicate that CMV reactivation may protect against AML relapse after allogeneic HSCT, and indirect associations suggest that CMV infection may promote allograft acceptance after liver transplantation. The causative mechanisms need further investigations, but are probably related to the profound and sustained imprint of CMV infection on the immune system.
While the positive impact of host–microbiota interaction on human health is being extensively studied in recent years, possible beneficial effects of life-long persistent viruses on human health remain a whole new world to explore. One of the most prevalent viruses among humans is cytomegalovirus (CMV). The sero-prevalence of CMV ranges from 30 to 100% depending on socioeconomic and ethnic background. CMV generally remains quiescent in healthy individuals, but can cause severe disorders in immunocompromised individuals, such as patients after hematopoietic stem cell transplantation (HSCT) or solid organ transplantation (SOT). Paradoxically, accumulating recent evidence suggests that CMV infection after transplantation may also have beneficial effects, particularly in protection against leukemic relapse following HSCT for acute myeloid leukemia (AML) and in promoting graft acceptance after liver transplantation (LTx). Recent research has shed first light on potential immunological mechanisms behind these surprising beneficial effects. Here, we discuss recent evidence for these two potential benefits of CMV infection after transplantation and emerging insights into the immunological mechanisms that may be involved.
Cytomegalovirus reactivation is a frequent and major complication after HSCT, causing a variety of organ-specific diseases, including pneumonia, encephalitis, and gastrointestinal disease. Prior to the age of prophylactic and pre-emptive treatment of CMV reactivation, CMV pneumonia was the most common infectious cause of death after HSCT. Despite, advances in diagnostic techniques and treatment strategies, CMV seropositivity remains to be associated with inferior outcome, especially after myeloablative HSCT (1–3). However, paradoxical observations show that CMV reactivation may protect against leukemic relapse after allogeneic HSCT for AML go back to the mid-1980s (4), and have been confirmed in a series of recent studies which we summarize in Table 1.
Table 1. Summary of recent studies on the association of post-transplant Cytomegalovirus (CMV) replication and relapse of hematological malignancies after HSCT.
In a homogeneous cohort of adult AML patients monitored by the pp65 antigenemia assay and treated with preemptive anti-CMV therapy, patients with early CMV replication after allo-HSCT had a significantly reduced risk to develop relapse within 10 years after transplantation (5). In a large cohort of allo-HSCT patients treated for different hematologic malignancies, Green et al. confirmed the anti-leukemia effect of early CMV replication detected by pp65 in AML patients, but did not observe such effect in acute lymphoblastic leukemia (ALL), chronic myeloid leukemia (CML), lymphoma, and myelodysplastic syndrome (MDS) patients (6). Interestingly, pre-transplant CMV seropositivity was associated with an increased risk of relapse, which was confirmed in another study (1). Therefore, not pre-transplant CMV serostatus, but actual CMV reactivation seems to contribute to the observed beneficial effect.
The association between early CMV replication after allo-HSCT and reduction of AML relapse risk was further confirmed by four independent recent studies from different countries (7–10). However, this association was not observed in patients who did not receive a myeloablative conditioning regimen (7) or were treated with an in vivo T-cell depleting therapy, such as ATG or alemtuzumab (10, 11). In three of these studies, CMV reactivation was determined by PCR. In contrast, one recent registry study, included 5,310 AML patients, showed no benefit of CMV reactivation for AML relapse risk after allo-HSCT (12). However, in this study 28% of AML patients did not receive myeloablative therapy and 27% of AML patients were treated by in vivo T/NK-cell depleting therapy. In addition, the methods for evaluation of CMV reactivation were unknown, which may have resulted in different definitions of CMV reactivation. A recent meta-analysis of 6 studies, including the recent registry study (12), with 8,511 AML patients who received mainly T-cell replete grafts and were not treated with T-cell depleting therapy, confirmed that CMV reactivation after allo-HSCT results in a substantial reduction of the risk of relapse (HR = 0.6, 95% CI = 0.43–0.84, P = 0.003) (13).
Thus, the evidence of a protective association between CMV replication and leukemic relapse in AML patients appears compelling, but only under specific transplantation conditions (7, 10, 11). However, it should be emphasized that only three studies reported an improved overall survival in AML patients with CMV replication after HSCT (5, 8, 10), while the majority of studies found that the anti-leukemic effect did not translate into improved survival. Indeed, other studies found either no difference in survival between patients with and without CMV replication (6, 7) or reported that CMV replication was associated with worse survival due to increased non-relapse mortality (9, 12).
The only evidence available in the cord blood transplantation setting is a recent registry study (14), which showed a trend to reduced AML relapse in patients with CMV reactivation. However, like in the registry study of Teira et al. (12), the methods used to detect CMV reactivation are unknown, and part of the patients received T-cell depleting therapy.
Whether CMV reactivation can protect against relapse after allo-HSCT for other hematological malignancies is controversial. While Ito et al. found a decreased risk of relapse in CML patients after CMV reactivation within 100 days after allo-HSCT (15), two other studies did not confirm this finding (6, 9). Most studies did not observe any beneficial effect of CMV reactivation after allo-HSCT for acute lymphoblastic leukemia (ALL), myelodysplastic syndrome (MDS), or lymphomas (6, 9, 14, 16), but a recent study by Koldehoff et al. reports a reduced relapse incidence in non-Hodgkin lymphoma (NHL) patients (17). Because of contradictory results reported for other hematological malignancies, results on associations between CMV reactivation and relapse after allo-HSCT derived from mixed populations of patients with different hematological malignancies are difficult to interpret (3, 18).
In addition, apart from one study where more severe (grade II–IV) acute GVHD was observed in patients with CMV reactivation (10), it was not associated with acute or chronic GVHD and remained an independent risk factor for AML relapse in multivariate analyses in which GVHD was included (Table S1 in Supplementary Material), however, this was not always reported. Available data, therefore, suggest that protection of AML relapse cannot be merely explained by an increased CMV-induced allogeneic immune response.
Cytomegalovirus reactivation does not protect against AML relapse when T- and/or NK cells are depleted in vivo or in vitro, suggesting that CMV reactivation requires a reconstitution of donor-derived T cells and/or NK cells to reduce leukemic relapse (7, 10, 11). Although recently a direct pro-apoptotic effect of CMV on acute leukemia cell lines has been shown (19), CMV is generally thought to be non-cytolytic, but instead to protect infected cells from apoptosis in order to delay cell death and maintain viral replication. Therefore, the anti-leukemic effects of CMV infection after allo-HSCT for AML are probably mainly caused by cross-reactivity of CMV-stimulated innate and adaptive immune responses with cancer cells. CMV infection leaves a deep and life-long imprint on the human immune system. Two types of immune cells that are expanded during CMV infection have been postulated to be involved in CMV-induced protection against AML. These are natural killer (NK) cell and Vδ2negγδ T cells (Figure 1).
Figure 1. Schematic overview of (potential) beneficial effects of cytomegalovirus (CMV) infection after transplantation. This schematic overview illustrates the (potential) beneficial effects exhibited by different CMV-induced immune cell subsets and intra-graft IFN-signaling pathways after transplantation. Compelling evidence exists for anti-leukemic effects of CMV-induced donor-derived memory-like natural killer (NK) cells after hematopoietic stem cell transplantation (HSCT) in acute myeloid leukemia (AML) patients, and two mechanisms have been described. One involves enhanced expression of the activating NK-receptor NKG2C, at the expense of the inhibitory NKG2A, interacting with HLA-E expressed by AML blasts. The other mechanism involves the “missing self” principle, as recipient tumor cells do not express donor HLA class I and are, therefore, a target for killing by donor-derived NK cells as a result of lack of inhibition via donor HLA class I-recognizing inhibitory killer cell immunoglobulin-like receptors. CMV-induced TCRδ2− γδ T cells have also been associated with anti-leukemic effects after HSCT, probably via recognition of an as yet unknown ligand by their TCR. Evidence implicating CMV-specific TCR αβ T cells in preventing AML relapse after HSCT is lacking. In addition, CMV-induced immune cell subsets have been associated with graft acceptance and liver-transplant tolerance. Evidence merely consists of associations and no detailed mechanistic insights are available yet. Induction of terminally differentiated TCRαβ T cells with low alloreactivity by CMV infection in various types of solid organ transplantations may be involved in development of graft acceptance. CMV-induced circulating TCRδ2− γδ T cells are associated with liver transplant tolerance, but probably not functionally involved. Overexpression of NKG2C in peripheral blood is associated with both CMV infection and graft acceptance after liver transplantation, but whether a causal exists between NKG2C+ NK cells and graft acceptance is unknown. Apart from CMV-induced immune cell subsets, intra-graft IFN-α, β, and γ production, which can be induced by CMV, has been associated with liver transplant tolerance by induction of PD-L1 expression in the graft, thereby counteracting the host immune response.
A CMV reactivation after allogeneic HSCT induces a long-lasting expansion of, mainly donor-derived, memory-like NK cells, or CMV-adapted NK cells, with enhanced functional properties compared to conventional NK cells. This CMV-induced memory-like NK-cell population is characterized by low expressionof CD56, expression of CD57, lack of the inhibitory NKG2a receptor, and expression of the activating heterodimeric receptor CD94-NKG2C (20, 21). The memory-associated features of these CMV-induced NK include secondary expansion and enhanced capacity to produce IFN-γ upon CMV reactivation (20, 22, 23). Once induced, their expansion is not limited to CMV reactivation, as stimulation via the low affinity Fc receptor IIIa (CD16) by IgG, as well as pro-inflammatory cytokines, can contribute to the expansion, persistence, and functional properties of CMV-induced memory-like NK cells (21, 24, 25). The enhanced functional properties of CMV-induced donor-derived NKG2C+ memory NK cells compared to conventional NK cells are caused by epigenetic remodeling resulting in increased proliferative responses as well as cytokine production (21, 24, 26).Interestingly, expansion of these cells after HSCT is not only associated with protection from CMV reactivation (27), but also trended to be associated with a reduced rate of AML relapse (28).
The mechanism by which this NK-cell subset recognizes leukemic blasts may be related to the switch in expression from the inhibitory NKG2A to the activating NKG2C, both receptors for HLA-E, which is expressed on leukemic blasts. Alternatively, in partially HLA-mismatched HSCT, the anti-leukemic effect may be related to the general mechanism of NK-cell self-tolerance, which is mediated by inhibitory receptors, such as killer cell immunoglobulin-like receptors (KIR), that recognize self-MHC class I molecules. According to the “missing-self hypothesis,” recipient AML cells can be the target for cytotoxicity of donor-derived NKG2C+ NK cells, induced upon CMV infection, as they lack donor HLA molecules (23).
γδ T cells are involved in the first line of host immune defense to microbial pathogens and expanded in the circulation during CMV infection. They also show some adaptive features, such as accelerated expansion upon CMV reactivation (29, 30). The main γδ T-cell subset in peripheral blood expresses T-cell receptors encoded by the Vδ2 and Vδ9 gene segments and is referred to as Vδ2posγδ T-cells. γδ T cells that express Vδ1, Vδ3, or Vδ5, but not Vδ2, TCR are collectively designated as Vδ2negγδ T-cells. The latter reside mainly in intestinal and skin epithelia, spleen, and liver (30). Interestingly, a strong and durable expansion of circulating Vδ2negγδ T cells occurs upon CMV reactivation after allo-HSCT (31, 32). This phenomenon is unique for CMV infection and does not occur after infections with other viruses after HSCT (31). Involvement of CMV-induced Vδ2negγδ T cells in protection from leukemic relapse has been suggested by studies showing that expansion of circulating Vδ2negγδ T cells after HSCT is associated with improved leukemia-free survival (33) and that CMV-reactive Vδ2negγδ T cells isolated from CMV-infected HSCT recipients cross-react to primary AML cells (32).
Vδ2negγδ T cells recognize leukemic blasts via their TCR, while CD8αα probably serves as a co-receptor in antigen recognition (29). They probably recognize novel antigens in similar manner as Vδ2posγδ T-cells. Quite different from αβ-TCR, Vδ2posγδ TCR recognizes conformational changes in proteins in an antibody-like way (34–36). In addition, natural cytotoxicity receptors, especially NKp30, may play a role in tumor cell recognition by Vδ2negγδ T cells (37).
In experimental animal SOT models, both acute CMV infection and CMV reactivation have been shown to prevent or disrupt graft acceptance, which is thought to result from cross-reactivity of virus-specific T cells to allo-antigens (38). In addition, in the clinical setting, CMV infection after SOT is generally associated with an increased risk of acute and chronic allograft rejection and like for HSCT, CMV infection is a major cause of morbidity and mortality. These data have been summarized in excellent reviews (39–41). Therefore, sophisticated strategies have been implemented to detect CMV early and treat pre-emptively.
However, a main issue in the interpretation of the observed associations between CMV infection and graft rejection is that rejections often occur before CMV infection, and, therefore, it is difficult to prove cause and effect (39, 42). Two recent studies suggest that the link between CMV infection and acute as well as chronic rejection after kidney transplantation are far less significant than previously thought (42, 43). A CMV infection significantly impacts the immune system leaving a clear fingerprint of memory inflation in the T-cell compartment, resembling features of immune aging or senescence (44, 45). This memory inflation is accelerated during CMV reactivation under immunosuppressive medication after SOT (46). Nevertheless, CMV-specific T cells that cross-react to donor cells are only transiently present in the circulation of CMV-infected kidney transplant recipients, and their presence is not associated with inferior graft function (47). Several recent studies even suggest that under certain conditions there may even be an opposite association between CMV infection and graft rejection. First, in elderly kidney transplant recipients, CMV seropositivity was associated with CD4+ T-cell immune senescence and freedom of acute rejection (48). Second, donor-specific T-cell hypo-responsiveness, i.e., reduced frequencies of donor-specific, but not of third party-specific T-cells, and reduced immunological graft damage were observed in patients with strong CMV-specific T-cell responses after kidney and heart transplantation (49, 50). Third, increased numbers of terminally differentiated CD8+ T-cells, as well as CD4+ T cells lacking CD28, both T-cell subsets associated with CMV latency, in the circulation prior to kidney transplantation have been associated with a lower risk for acute rejection (51, 52). Finally, primary CMV infection following LTx is associated with accumulation of terminally differentiated CD8+ T cells in the circulation as well as with donor-specific CD8+ T-cell hypo-responsiveness and a reduced incidence of acute rejection episodes late after transplantation (53).
As compared with other solid organ grafts, liver grafts display unique immunological features, and LTx is the only setting in which a significant proportion of patients can eventually discontinue immunosuppressive medication without undergoing rejection, a phenomenon known as spontaneous operational tolerance (54). Recent prospective immunosuppression withdrawal studies have shown that operational tolerance can be achieved in about 40% of stable adult LTx patients and 60% of stable pediatric LTX patients (55–57). Interestingly, studies from two different centers found expanded numbers of peripheral Vδ1+γδ T cells and an increased peripheral Vδ1/Vδ2 γδ T-cell ratio in tolerant compared to non-tolerant LTx patients (58–60). In addition, tolerant pediatric LTx recipients exhibit an increased intra-graft Vδ1/Vδ2 ratio (61). A high peripheral Vδ1/Vδ2 γδ T-cell ratio has even successfully been used as a biomarker to select liver transplant (LT) patients for immunosuppression withdrawal (56). Since, durable expansion of circulating Vδ1+γδ T cells and an increased peripheral Vδ1/Vδ2 ratio after CMV infection has been observed in all types of SOT, including LTx (53, 62–65), these observations suggest an association between CMV infection and tolerance after LTx. Such relationship is further supported by a recent study which showed that primary CMV infection after LTx is associated with both expansion of circulating Vδ1+γδ T cells and donor-specific CD8+ T cell hypo-responsiveness (53). CMV-responsive Vδ2− γδ T cells have been implicated in antibody mediated rejection after kidney transplant recipients (66), suggesting a different role for these cells after liver transplantation, which may be related to the lower impact of antibodies in liver graft rejection compared to kidney graft rejection.
Increased numbers of circulating terminally differentiated CD8+ T cells expressing co-inhibitory receptors is another feature shared by CMV infection (53, 67) and operational tolerance (56) after LTx, again suggesting a possible association. Finally, comparison of gene expression patterns in circulating leukocytes between tolerant and non-tolerant LTx recipients revealed over-expression of NK-cell-related genes in tolerant patients. Interestingly, KLRC4, one of genes encoding NKG2C, which is induced on circulating NK cells by CMV infection and reactivation both after HSCT (20–22, 27) and SOT, including LTx (68–70), was found to be over-expressed in tolerant LTx patients in two different cohorts (59, 71).
Thus, although a direct association between CMV infection and graft acceptance after LTx has not been demonstrated, the presence of sustained immunological imprints of CMV infection in operationally tolerant, but not in non-tolerant, LT patients is strongly suggestive for such association.
How CMV restrains alloreactivity after LTx remains elusive, and whether CMV-induced peripheral immune cell signatures play a causative role in promoting LT tolerance is as yet unknown. Although Vδ1+γδ T cells under certain conditions may have immune-regulatory properties (72, 73), it is as yet unknown whether they can contribute to liver graft acceptance. However, the massive peripheral expansion of terminally differentiated CD8+ T cells expressing co-inhibitory receptors upon CMV infection after LTx may contribute to reduced T-cell alloreactivity to organ grafts, since these cells show impaired functional responses to allo-antigens (53, 67) (Figure 1). In addition, as terminally differentiated CD8+ T cells poorly infiltrate in organ grafts, they might not contribute to graft rejection even when functionally competent to respond to allo-antigens (53, 74).
Cytomegalovirus infection of the liver graft may also promote resistance of liver grafts to allogeneic attack by triggering type I interferon production (75) and recruitment of T-helper 1 cells that produce IFN-γ (76), which is strikingly absolutely needed for LT tolerance in animal models (77). IFN-γ produced by graft-infiltrating T cells critically contributes to immunological tolerance of liver grafts in experimental animals by induction of intra-graft PD-L1 expression that leads to T-cell apoptosis (78). Indeed, interaction of PD-L1 expressed in the liver graft with the co-inhibitory receptor PD-1 on graft-infiltrating T cells also counter-regulates rejection activity against liver grafts in humans (79) (Figure 1). Although this mechanism of CMV-driven graft anti-host resistance is speculative, a role for intra-graft interferon signaling, in the development of operational tolerance after LTX has been suggested by an immunosuppression withdrawal trial in HCV-infected LT patients in which operationally tolerant patients were observed to overexpress interferon-stimulated genes as well as PD-L1 and PD-1 in their liver graft (56). Such so-called “adaptive immune resistance” mechanisms, in which expression of immunosuppressive molecules, such as PD-L1, is induced in response to IFN type I and/or IFN-γ produced by infiltrating immune cells, are also utilized by tumors to escape immune attack (80). In addition, recent data show that IFN-type I signaling during chronic viral infections may promote CD8+ T cell exhaustion and impair memory T-cell responses against unrelated antigens (81, 82), connecting IFN-signaling to terminal differentiation and exhaustion of CD8+ T cells.
Finally, one or more of the well-established immune evasion strategies of CMV to establish latency may be involved, such as production of viral IL-10, which may exert systemic immunosuppressive effects (83).
Cytomegalovirus infection, although generally disadvantageous in immunosuppressed subjects, paradoxically has beneficial effects in HSCT and probably also in LTx recipients. More research is definitely needed to substantiate and better understand these enigmatic observations. Whereas, the anti-leukemic effect of CMV infection after HSCT in AML patients is firmly established, prospective studies are required to investigate whether there is a direct association between CMV infection after LTx and development of allogeneic liver graft acceptance. Such studies should be accompanied by extensive peripheral and intra-graft immune profiling to begin to understand the putative immunological mechanisms linking CMV infection with tolerance after LTX. Similarly, studies aiming to further unravel the immunological mechanisms linking CMV infection and prevention of AML recurrence after HSCT are required. Once those benefits and the underlying mechanisms have been firmly established, development of therapeutic approaches to mimic the beneficial effects of CMV infection after HSCT for AML and after LTx becomes an interesting, although challenging aim. Development of a CMV vaccine that induces similar immunological imprints as CMV infections might be a way to achieve this goal.
NL, LW, JKu, and JKw all participated in writing the manuscript. NL and JKw co-edited the final version of the manuscript. All authors have read and approved the final manuscript.
NL, LW, and JKw have nothing to disclose. JKu reports grants from Novartis and Miltenyi Biotech. He is scientific co-founder and CSO of gadeta (www.gadeta.nl) and inventor on multiple patents on γδ TCR receptors and isolation strategies for engineered immune cells.
The Supplementary Material for this article can be found online at http://www.frontiersin.org/articles/10.3389/fimmu.2018.00389/full#supplementary-material.
CMV, cytomegalovirus; HSCT, hematopoietic stem cell transplantation; AML, acute myeloid leukemia; SOT, solid organ transplantation; LT patient, liver transplant patient; LTx, liver transplantation; ALL, acute lymphoblastic leukemia (ALL); CML, chronic myeloid leukemia; MDS, myelo-dysplastic syndrome (MDS); a/cGVHD, acute/chronic graft versus host disease; KIR, killer cell immunoglobulin-like receptors.
1. Schmidt-Hieber M, Labopin M, Beelen D, Volin L, Ehninger G, Finke J, et al. CMV serostatus still has an important prognostic impact in de novo acute leukemia patients after allogeneic stem cell transplantation: a report from the acute leukemia working party of EBMT. Blood (2013) 122(19):3359–64. doi:10.1182/blood-2013-05-499830
2. Ljungman P. The role of cytomegalovirus serostatus on outcome of hematopoietic stem cell transplantation. Curr Opin Hematol (2014) 21(6):466–9. doi:10.1097/MOH.0000000000000085
3. Verduyn Lunel FM, Raymakers R, van Dijk A, van der Wagen L, Minnema MC, Kuball J. Cytomegalovirus status and the outcome of T cell-replete reduced-intensity allogeneic hematopoietic stem cell transplantation. Biol Blood Marrow Transplant (2016) 22(10):1883–7. doi:10.1016/j.bbmt.2016.07.009
4. Lonnqvist B, Ringden O, Ljungman P, Wahren B, Gahrton G. Reduced risk of recurrent leukaemia in bone marrow transplant recipients after cytomegalovirus infection. Br J Haematol (1986) 63(4):671–9. doi:10.1111/j.1365-2141.1986.tb07551.x
5. Elmaagacli AH, Steckel NK, Koldehoff M, Hegerfeldt Y, Trenschel R, Ditschkowski M, et al. Early human cytomegalovirus replication after transplantation is associated with a decreased relapse risk: evidence for a putative virus-versus-leukemia effect in acute myeloid leukemia patients. Blood (2011) 118(5):1402–12. doi:10.1182/blood-2010-08-304121
6. Green ML, Leisenring WM, Xie H, Walter RB, Mielcarek M, Sandmaier BM, et al. CMV reactivation after allogeneic HCT and relapse risk: evidence for early protection in acute myeloid leukemia. Blood (2013) 122(7):1316–24. doi:10.1182/blood-2013-02-487074
7. Manjappa S, Bhamidipati PK, Stokerl-Goldstein KE, DiPersio JF, Uy GL, Westervelt P, et al. Protective effect of cytomegalovirus reactivation on relapse after allogeneic hematopoietic cell transplantation in acute myeloid leukemia patients is influenced by conditioning regimen. Biol Blood Marrow Transplant (2014) 20(1):46–52. doi:10.1016/j.bbmt.2013.10.003
8. Jang JE, Kim SJ, Cheong JW, Hyun SY, Kim YD, Kim YR, et al. Early CMV replication and subsequent chronic GVHD have a significant anti-leukemic effect after allogeneic HSCT in acute myeloid leukemia. Ann Hematol (2015) 94(2):275–82. doi:10.1007/s00277-014-2190-1
9. Takenaka K, Nishida T, Asano-Mori Y, Oshima K, Ohashi K, Mori T, et al. Cytomegalovirus reactivation after allogeneic hematopoietic stem cell transplantation is associated with a reduced risk of relapse in patients with acute myeloid leukemia who survived to day 100 after transplantation: the Japan society for hematopoietic cell transplantation transplantation-related complication working group. Biol Blood Marrow Transplant (2015) 21(11):2008–16. doi:10.1016/j.bbmt.2015.07.019
10. Bao X, Zhu Q, Xue S, Hu X, Ma X, Chen F, et al. Cytomegalovirus induces strong antileukemic effect in acute myeloid leukemia patients following sibling HSCT without ATG-containing regimen. Am J Transl Res (2016) 8(2):653–61.
11. Elmaagacli A, Koldehoff M, Lindemann M, Sonius M, Ditschkowski M, Steckel N, et al. Response: T cells are required for the CMV-induced antileukemia effect after transplant. Blood (2012) 119(4):1090–1. doi:10.1182/blood-2011-11-386821
12. Teira P, Battiwalla M, Ramanathan M, Barrett AJ, Ahn KW, Chen M, et al. Early cytomegalovirus reactivation remains associated with increased transplant-related mortality in the current era: a CIBMTR analysis. Blood (2016) 127(20):2427–38. doi:10.1182/blood-2015-11-679639
13. Elmaagacli AH, Koldehoff M. Cytomegalovirus replication reduces the relapse incidence in patients with acute myeloid leukemia. Blood (2016) 128(3):456–9. doi:10.1182/blood-2016-04-713644
14. Ramanathan M, Teira P, Battiwalla M, Barrett J, Ahn KW, Chen M, et al. Impact of early CMV reactivation in cord blood stem cell recipients in the current era. Bone Marrow Transplant (2016) 51(8):1113–20. doi:10.1038/bmt.2016.89
15. Ito S, Pophali P, Co W, Koklanaris EK, Superata J, Fahle GA, et al. CMV reactivation is associated with a lower incidence of relapse after allo-SCT for CML. Bone Marrow Transplant (2013) 48(10):1313–6. doi:10.1038/bmt.2013.49
16. Mariotti J, Maura F, Spina F, Roncari L, Dodero A, Farina L, et al. Impact of cytomegalovirus replication and cytomegalovirus serostatus on the outcome of patients with B cell lymphoma after allogeneic stem cell transplantation. Biol Blood Marrow Transplant (2014) 20(6):885–90. doi:10.1016/j.bbmt.2014.02.015
17. Koldehoff M, Ross SR, Duhrsen U, Beelen DW, Elmaagacli AH. Early CMV-replication after allogeneic stem cell transplantation is associated with a reduced relapse risk in lymphoma. Leuk Lymphoma (2017) 58(4):822–33. doi:10.1080/10428194.2016.1217524
18. Inagaki J, Noguchi M, Kurauchi K, Tanioka S, Fukano R, Okamura J. Effect of cytomegalovirus reactivation on relapse after allogeneic hematopoietic stem cell transplantation in pediatric acute leukemia. Biol Blood Marrow Transplant (2016) 22(2):300–6. doi:10.1016/j.bbmt.2015.09.006
19. Koldehoff M, Lindemann M, Opalka B, Bauer S, Ross RS, Elmaagacli AH. Cytomegalovirus induces apoptosis in acute leukemia cells as a virus-versus-leukemia function. Leuk Lymphoma (2015) 56(11):3189–97. doi:10.3109/10428194.2015.1032968
20. Foley B, Cooley S, Verneris MR, Pitt M, Curtsinger J, Luo X, et al. Cytomegalovirus reactivation after allogeneic transplantation promotes a lasting increase in educated NKG2C+ natural killer cells with potent function. Blood (2012) 119(11):2665–74. doi:10.1182/blood-2011-10-386995
21. Fehniger TA, Cooper MA. Harnessing NK cell memory for cancer immunotherapy. Trends Immunol (2016) 37(12):877–88. doi:10.1016/j.it.2016.09.005
22. Foley B, Cooley S, Verneris MR, Curtsinger J, Luo X, Waller EK, et al. Human cytomegalovirus (CMV)-induced memory-like NKG2C(+) NK cells are transplantable and expand in vivo in response to recipient CMV antigen. J Immunol (2012) 189(10):5082–8. doi:10.4049/jimmunol.1201964
23. Jin F, Lin H, Gao S, Wang H, Yan H, Guo J, et al. Characterization of IFNgamma-producing natural killer cells induced by cytomegalovirus reacti-vation after haploidentical hematopoietic stem cell transplantation. Oncotarget (2017) 8(1):51–63. doi:10.18632/oncotarget.13916
24. Lee J, Zhang T, Hwang I, Kim A, Nitschke L, Kim M, et al. Epigenetic modification and antibody-dependent expansion of memory-like NK cells in human cytomegalovirus-infected individuals. Immunity (2015) 42(3):431–42. doi:10.1016/j.immuni.2015.02.013
25. Rolle A, Pollmann J, Ewen EM, Le VT, Halenius A, Hengel H, et al. IL-12-producing monocytes and HLA-E control HCMV-driven NKG2C+ NK cell expansion. J Clin Invest (2014) 124(12):5305–16. doi:10.1172/JCI77440
26. Schlums H, Cichocki F, Tesi B, Theorell J, Beziat V, Holmes TD, et al. Cytomegalovirus infection drives adaptive epigenetic diversification of NK cells with altered signaling and effector function. Immunity (2015) 42(3):443–56. doi:10.1016/j.immuni.2015.02.008
27. Davis ZB, Cooley SA, Cichocki F, Felices M, Wangen R, Luo X, et al. Adaptive natural killer cell and killer cell immunoglobulin-like receptor-expressing T cell responses are induced by cytomegalovirus and are associated with protection against cytomegalovirus reactivation after allogeneic donor hematopoietic cell transplantation. Biol Blood Marrow Transplant (2015) 21(9):1653–62. doi:10.1016/j.bbmt.2015.05.025
28. Cichocki F, Cooley S, Davis Z, DeFor TE, Schlums H, Zhang B, et al. CD56dimCD57+NKG2C+ NK cell expansion is associated with reduced leukemia relapse after reduced intensity HCT. Leukemia (2016) 30(2):456–63. doi:10.1038/leu.2015.260
29. Scheper W, Grunder C, Straetemans T, Sebestyen Z, Kuball J. Hunting for clinical translation with innate-like immune cells and their receptors. Leukemia (2014) 28(6):1181–90. doi:10.1038/leu.2013.378
30. Couzi L, Pitard V, Moreau JF, Merville P, Dechanet-Merville J. Direct and indirect effects of cytomegalovirus-induced gammadelta T cells after kidney transplantation. Front Immunol (2015) 6:3. doi:10.3389/fimmu.2015.00003
31. Knight A, Madrigal AJ, Grace S, Sivakumaran J, Kottaridis P, Mackinnon S, et al. The role of Vdelta2-negative gammadelta T cells during cytomega-lovirus reactivation in recipients of allogeneic stem cell transplantation. Blood (2010) 116(12):2164–72. doi:10.1182/blood-2010-01-255166
32. Scheper W, van Dorp S, Kersting S, Pietersma F, Lindemans C, Hol S, et al. GammadeltaT cells elicited by CMV reactivation after allo-SCT cross-recognize CMV and leukemia. Leukemia (2013) 27(6):1328–38. doi:10.1038/leu.2012.374
33. Godder KT, Henslee-Downey PJ, Mehta J, Park BS, Chiang KY, Abhyankar S, et al. Long term disease-free survival in acute leukemia patients recovering with increased gammadelta T cells after partially mismatched related donor bone marrow transplantation. Bone Marrow Transplant (2007) 39(12):751–7. doi:10.1038/sj.bmt.1705650
34. Vavassori S, Kumar A, Wan GS, Ramanjaneyulu GS, Cavallari M, El Daker S, et al. Butyrophilin 3A1 binds phosphorylated antigens and stimulates human gammadelta T cells. Nat Immunol (2013) 14(9):908–16. doi:10.1038/ni.2665
35. Sandstrom A, Peigne CM, Leger A, Crooks JE, Konczak F, Gesnel MC, et al. The intracellular B30.2 domain of butyrophilin 3A1 binds phosphoantigens to mediate activation of human Vgamma9Vdelta2 T cells. Immunity (2014) 40(4):490–500. doi:10.1016/j.immuni.2014.03.003
36. Sebestyen Z, Scheper W, Vyborova A, Gu S, Rychnavska Z, Schiffler M, et al. RhoB mediates phosphoantigen recognition by Vgamma9Vdelta2 T cell receptor. Cell Rep (2016) 15(9):1973–85. doi:10.1016/j.celrep.2016.04.081
37. Correia DV, Fogli M, Hudspeth K, da Silva MG, Mavilio D, Silva-Santos B. Differentiation of human peripheral blood Vdelta1+ T cells expressing the natural cytotoxicity receptor NKp30 for recognition of lymphoid leukemia cells. Blood (2011) 118(4):992–1001. doi:10.1182/blood-2011-02-339135
38. Krummey SM, Ford ML. Heterogeneity within T cell memory: implications for transplant tolerance. Front Immunol (2012) 3:36. doi:10.3389/fimmu.2012.00036
39. Cainelli F, Vento S. Infections and solid organ transplant rejection: a cause-and-effect relationship? Lancet Infect Dis (2002) 2(9):539–49. doi:10.1016/S1473-3099(02)00370-5
40. Fishman JA. Infection in solid-organ transplant recipients. N Engl J Med (2007) 357(25):2601–14. doi:10.1056/NEJMra064928
41. Eid AJ, Razonable RR. New developments in the management of cytomegalovirus infection after solid organ transplantation. Drugs (2010) 70(8):965–81. doi:10.2165/10898540-000000000-00000
42. Erdbrugger U, Scheffner I, Mengel M, Schwarz A, Haller H, Gwinner W.Long-term impact of CMV infection on allografts and on patient survival in renal transplant patients with protocol biopsies. Am J Physiol Renal Physiol (2015) 309(11):F925–32. doi:10.1152/ajprenal.00317.2015
43. Erdbruegger U, Scheffner I, Mengel M, Schwarz A, Verhagen W, Haller H, et al. Impact of CMV infection on acute rejection and long-term renal allograft function: a systematic analysis in patients with protocol biopsies and indicated biopsies. Nephrol Dial Transplant (2012) 27(1):435–43. doi:10.1093/ndt/gfr306
44. O’Hara GA, Welten SP, Klenerman P, Arens R. Memory T cell inflation: understanding cause and effect. Trends Immunol (2012) 33(2):84–90. doi:10.1016/j.it.2011.11.005
45. Solana R, Tarazona R, Aiello AE, Akbar AN, Appay V, Beswick M, et al. CMV and immunosenescence: from basics to clinics. Immun Ageing (2012) 9(1):23. doi:10.1186/1742-4933-9-23
46. van Leeuwen EM, Koning JJ, Remmerswaal EB, van Baarle D, van Lier RA, ten Berge IJ. Differential usage of cellular niches by cytomegalovirus versus EBV- and influenza virus-specific CD8+ T cells. J Immunol (2006) 177(8):4998–5005. doi:10.4049/jimmunol.177.8.4998
47. Heutinck KM, Yong SL, Tonneijck L, van den Heuvel H, van der Weerd NC, van der Pant KA, et al. Virus-specific CD8(+) T cells cross-reactive to donor-alloantigen are transiently present in the circulation of kidney transplant recipients infected with CMV and/or EBV. Am J Transplant (2016) 16(5):1480–91. doi:10.1111/ajt.13618
48. Trzonkowski P, Debska-Slizien A, Jankowska M, Wardowska A, Carvalho-Gaspar M, Hak L, et al. Immunosenescence increases the rate of accep-tance of kidney allotransplants in elderly recipients through exhaustion of CD4+ T-cells. Mech Ageing Dev (2010) 131(2):96–104. doi:10.1016/j.mad.2009.12.006
49. Nickel P, Bold G, Presber F, Biti D, Babel N, Kreutzer S, et al. High levels of CMV-IE-1-specific memory T cells are associated with less alloimmu-nity and improved renal allograft function. Transpl Immunol (2009) 20(4):238–42. doi:10.1016/j.trim.2008.11.002
50. Tu W, Potena L, Stepick-Biek P, Liu L, Dionis KY, Luikart H, et al. T-cell immunity to subclinical cytomegalovirus infection reduces cardiac allo-graft disease. Circulation (2006) 114(15):1608–15. doi:10.1161/CIRCULA-TIONAHA.105.607549
51. Betjes MG, Meijers RW, de Wit EA, Weimar W, Litjens NH. Terminally differentiated CD8+ temra cells are associated with the risk for acute kidney allograft rejection. Transplantation (2012) 94(1):63–9. doi:10.1097/TP.0b013e31825306ff
52. Dedeoglu B, Meijers RW, Klepper M, Hesselink DA, Baan CC, Litjens NH, et al. Loss of CD28 on peripheral T cells decreases the risk for early acute rejection after kidney transplantation. PLoS One (2016) 11(3):e0150826. doi:10.1371/journal.pone.0150826
53. Shi XL, de Mare-Bredemeijer EL, Tapirdamaz O, Hansen BE, van Gent R, van Campenhout MJ, et al. CMV Primary infection is associated with donor-specific T cell hyporesponsiveness and fewer late acute rejections after liver transplantation. Am J Transplant (2015) 15(9):2431–42. doi:10.1111/ajt.13288
54. Orlando G, Soker S, Wood K. Operational tolerance after liver transplan-tation. J Hepatol (2009) 50(6):1247–57. doi:10.1016/j.jhep.2009.03.006
55. Benitez C, Londono MC, Miquel R, Manzia TM, Abraldes JG, Lozano JJ, et al. Prospective multicenter clinical trial of immunosuppressive drug withdrawal in stable adult liver transplant recipients. Hepatology (2013) 58(5):1824–35. doi:10.1002/hep.26426
56. Bohne F, Londono MC, Benitez C, Miquel R, Martinez-Llordella M, Russo C, et al. HCV-induced immune responses influence the development of operational tolerance after liver transplantation in humans. Sci Transl Med (2014) 6(242):242ra81. doi:10.1126/scitranslmed.3008793
57. Feng S, Ekong UD, Lobritto SJ, Demetris AJ, Roberts JP, Rosenthal P, et al. Complete immunosuppression withdrawal and subsequent allograft function among pediatric recipients of parental living donor liver trans-plants. JAMA (2012) 307(3):283–93. doi:10.1001/jama.2011.2014
58. Li Y, Koshiba T, Yoshizawa A, Yonekawa Y, Masuda K, Ito A, et al. Analyses of peripheral blood mononuclear cells in operational tolerance after pediatric living donor liver transplantation. Am J Transplant (2004) 4(12):2118–25. doi:10.1111/j.1600-6143.2004.00611.x
59. Martinez-Llordella M, Lozano JJ, Puig-Pey I, Orlando G, Tisone G, Lerut J, et al. Using transcriptional profiling to develop a diagnostic test of operational tolerance in liver transplant recipients. J Clin Invest (2008) 118(8):2845–57. doi:10.1172/JCI35342
60. Martinez-Llordella M, Puig-Pey I, Orlando G, Ramoni M, Tisone G, Rimola A, et al. Multiparameter immune profiling of operational tolerancein liver transplantation. Am J Transplant (2007) 7(2):309–19. doi:10.1111/j.1600-6143.2006.01621.x
61. Zhao X, Li Y, Ohe H, Nafady-Hego H, Uemoto S, Bishop GA, et al. Intra-graft Vdelta1 gammadelta T cells with a unique T-cell receptor are closely associated with pediatric semiallogeneic liver transplant tolerance. Transplantation (2013) 95(1):192–202. doi:10.1097/TP.0b013e3182782f9f
62. Couzi L, Lafarge X, Pitard V, Neau-Cransac M, Dromer C, Billes MA, et al. Gamma-delta T cell expansion is closely associated with cytomegalovirus infection in all solid organ transplant recipients. Transpl Int (2011) 24(5):e40–2. doi:10.1111/j.1432-2277.2010.01181.x
63. Puig-Pey I, Bohne F, Benitez C, Lopez M, Martinez-Llordella M, Oppenheimer F, et al. Characterization of gammadelta T cell subsets in organ transplantation. Transpl Int (2010) 23(10):1045–55. doi:10.1111/j.1432-2277.2010.01095.x
64. Roux A, Mourin G, Larsen M, Fastenackels S, Urrutia A, Gorochov G, et al. Differential impact of age and cytomegalovirus infection on the gammadelta T cell compartment. J Immunol (2013) 191(3):1300–6. doi:10.4049/jimmunol.1202940
65. Kaminski H, Garrigue I, Couzi L, Taton B, Bachelet T, Moreau JF, et al. Surveillance of gammadelta T cells predicts cytomegalovirus infection resolution in kidney transplants. J Am Soc Nephrol (2016) 27(2):637–45. doi:10.1681/ASN.2014100985
66. Bachelet T, Couzi L, Pitard V, Sicard X, Rigothier C, Lepreux S, et al. Cytomegalovirus-responsive gammadelta T cells: novel effector cells in antibody-mediated kidney allograft microcirculation lesions. J Am Soc Nephrol (2014) 25(11):2471–82. doi:10.1681/ASN.2013101052
67. de Mare-Bredemeijer EL, Shi XL, Mancham S, van Gent R, van der Heide-Mulder M, de Boer R, et al. Cytomegalovirus-induced expression of CD244 after liver transplantation is associated with CD8+ T cell hyporesponsiveness to alloantigen. J Immunol (2015) 195(4):1838–48. doi:10.4049/jimmunol.1500440
68. Redondo-Pachon D, Crespo M, Yelamos J, Muntasell A, Perez-Saez MJ, Perez-Fernandez S, et al. Adaptive NKG2C+ NK cell response and the risk of cytomegalovirus infection in kidney transplant recipients. J Immunol (2017) 198(1):94–101. doi:10.4049/jimmunol.1601236
69. Achour A, Baychelier F, Besson C, Arnoux A, Marty M, Hannoun L, et al. Expansion of CMV-mediated NKG2C+ NK cells associates with the development of specific de novo malignancies in liver-transplanted patients. J Immunol (2014) 192(1):503–11. doi:10.4049/jimmunol.1301951
70. Lopez-Verges S, Milush JM, Schwartz BS, Pando MJ, Jarjoura J, York VA, et al. Expansion of a unique CD57(+)NKG2Chi natural killer cell subset during acute human cytomegalovirus infection. Proc Natl Acad Sci U S A (2011) 108(36):14725–32. doi:10.1073/pnas.1110900108
71. Bohne F, Martinez-Llordella M, Lozano JJ, Miquel R, Benitez C, Londono MC, et al. Intra-graft expression of genes involved in iron homeostasis predicts the development of operational tolerance in human liver transplantation. J Clin Invest (2012) 122(1):368–82. doi:10.1172/JCI59411
72. Barakonyi A, Polgar B, Szekeres-Bartho J. The role of gamma/delta T-cell receptor-positive cells in pregnancy: part II. Am J Reprod Immunol (1999) 42(2):83–7.
73. Peng G, Wang HY, Peng W, Kiniwa Y, Seo KH, Wang RF. Tumor-infiltrating gammadelta T cells suppress T and dendritic cell function via mechanisms controlled by a unique toll-like receptor signaling pathway. Immunity (2007) 27(2):334–48. doi:10.1016/j.immuni.2007.05.020
74. Macedo C, Walters JT, Orkis EA, Isse K, Elinoff BD, Fedorek SP, et al. Long-term effects of alemtuzumab on regulatory and memory T-cell subsets in kidney transplantation. Transplantation (2012) 93(8):813–21. doi:10.1097/TP.0b013e318247a717
75. Hokeness-Antonelli KL, Crane MJ, Dragoi AM, Chu WM, Salazar-Mather TP. IFN-alphabeta-mediated inflammatory responses and antiviral defense in liver is TLR9-independent but MyD88-dependent during murine cytomegalovirus infection. J Immunol (2007) 179(9):6176–83. doi:10.4049/jimmunol.179.9.6176
76. Bruns T, Zimmermann HW, Pachnio A, Li KK, Trivedi PJ, Reynolds G, et al. CMV infection of human sinusoidal endothelium regulates hepatic T cell recruitment and activation. J Hepatol (2015) 63(1):38–49. doi:10.1016/j.jhep.2015.02.046
77. Mele TS, Kneteman NM, Zhu LF, Ramassar V, Urmson J, Halloran B, et al. IFN-gamma is an absolute requirement for spontaneous acceptance of liver allografts. Am J Transplant (2003) 3(8):942–51. doi:10.1034/j.1600-6143.2003.00153.x
78. Morita M, Joyce D, Miller C, Fung JJ, Lu L, Qian S. Rejection triggers liver transplant tolerance: involvement of mesenchyme-mediated immune control mechanisms in mice. Hepatology (2015) 62(3):915–31. doi:10.1002/hep.27909
79. Shi XL, Mancham S, Hansen BE, de Knegt RJ, de Jonge J, van der Laan LJ, et al. Counter-regulation of rejection activity against human liver grafts by donor PD-L1 and recipient PD-1 interaction. J Hepatol (2016) 64(6):1274–82. doi:10.1016/j.jhep.2016.02.034
80. Spranger S, Spaapen RM, Zha Y, Williams J, Meng Y, Ha TT, et al. Up-regulation of PD-L1, IDO, and T(regs) in the melanoma tumor microenvironment is driven by CD8(+) T cells. Sci Transl Med (2013) 5(200):200ra116. doi:10.1126/scitranslmed.3006504
81. Stelekati E, Shin H, Doering TA, Dolfi DV, Ziegler CG, Beiting DP, et al. Bystander chronic infection negatively impacts development of CD8(+) T cell memory. Immunity (2014) 40(5):801–13. doi:10.1016/j.immuni.2014.04.010
82. Zhen A, Rezek V, Youn C, Lam B, Chang N, Rick J, et al. Targeting type I interferon-mediated activation restores immune function in chronic HIV infection. J Clin Invest (2017) 127(1):260–8. doi:10.1172/JCI89488
Keywords: cytomegalovirus infections, hematopoietic stem cell transplantation, solid organ transplantation, leukemia, tolerance
Citation: Litjens NHR, van der Wagen L, Kuball J and Kwekkeboom J (2018) Potential Beneficial Effects of Cytomegalovirus Infection after Transplantation. Front. Immunol. 9:389. doi: 10.3389/fimmu.2018.00389
Received: 01 November 2017; Accepted: 12 February 2018;
Published: 01 March 2018
Edited by:
Ulrike Koehl, Hannover Medical School, GermanyReviewed by:
Anne Mary Dickinson, Newcastle University, United KingdomCopyright: © 2018 Litjens, van der Wagen, Kuball and Kwekkeboom. This is an open-access article distributed under the terms of the Creative Commons Attribution License (CC BY). The use, distribution or reproduction in other forums is permitted, provided the original author(s) and the copyright owner are credited and that the original publication in this journal is cited, in accordance with accepted academic practice. No use, distribution or reproduction is permitted which does not comply with these terms.
*Correspondence: Jaap Kwekkeboom, ai5rd2Vra2Vib29tQGVyYXNtdXNtYy5ubA==
Disclaimer: All claims expressed in this article are solely those of the authors and do not necessarily represent those of their affiliated organizations, or those of the publisher, the editors and the reviewers. Any product that may be evaluated in this article or claim that may be made by its manufacturer is not guaranteed or endorsed by the publisher.
Research integrity at Frontiers
Learn more about the work of our research integrity team to safeguard the quality of each article we publish.