- 1Universitätsklinikum Erlangen, Medizinische Klinik 1, Erlangen, Germany
- 2Charité Universitätsmedizin Berlin, Campus Benjamin Franklin Medizinische Klinik für Gastroenterologie, Infektiologie und Rheumatologie, Berlin, Germany
Transient receptor potential (TRP) ion channels are widely expressed in several tissues throughout the mammalian organism. Originally, TRP channel physiology was focusing on its fundamental meaning in sensory neuronal function. Today, it is known that activation of several TRP ion channels in peptidergic neurons does not only result in neuropeptide release and consecutive neurogenic inflammation. Growing evidence demonstrates functional extra-neuronal TRP channel expression in immune and epithelial cells with important implications for mucosal immunology. TRP channels maintain intracellular calcium homeostasis to regulate various functions in the respective cells such as nociception, production and release of inflammatory mediators, phagocytosis, and cell migration. In this review, we provide an overview about TRP-mediated effects in immune and epithelial cells with an emphasis on mucosal immunology of the gut. Crosstalk between neurons, epithelial cells, and immune cells induced by activation of TRP channels orchestrates the immunologic response. Understanding of its molecular mechanisms paves the way to novel clinical approaches for the treatment of various inflammatory disorders including IBD.
Introduction
The transient receptor potential (TRP) ion channel family consists of 28 members, which are divided into six subsets: TRPC (“canonical”), TRPM (“melastatin”), TRPV (“vanilloid”), TRPA (“ankyrin”), TRPML (“mucolipin”), and TRPP (or PKD) (“polycystin”) (1). TRP channels are membrane proteins with substantial cation permeability, preferentially high calcium ion permeability, and calcium signaling plays a central role in many physiological processes. TRP receptors are polymodal ion channels with an exceptional role in the integration of various environmental stimuli including mechanical, thermal, or chemical stimuli. Inhering this function they are likely to be sensors for monitoring specific responses to different exogenous and endogenous chemical noxious and physical stimuli. As such, various TRP channels play an essential role in somatic and visceral nociception (2, 3). Upon activation, TRP channels also control the release of immunomodulatory neuropeptides such as substance P (SP) and calcitonin gene-related peptide (CGRP), the so-called neurogenic inflammation. During recent years, increasing evidence has demonstrated an important role of many TRP channels outside the nervous system in the context of inflammation; findings that extend the role of TRP channels in the regulation of inflammation beyond neuropeptide release. To date, only little is known about the functional role of TRP channels in the immune system. Moreover, recent reports describe a fundamental role of TRP channels in epithelial cells in mediating cytokine/chemokine release as well (4). In summary, TRP channel activation induces immunomodulatory effects on multiple levels. This review will focus on the role of TRP channels in immune cells (with a focus on macrophages and T cells) and epithelial cells in general, with an additional special focus on TRPs in intestinal inflammation. Recognizing the large family of TRP channels, this mini-review focuses on TRPA1, TRPM8, TRPV1, and TRPV4 (alphabetical order), which are the most relevant TRP channels in the present context based on published literature until today. Table S1 (available in supplementary material) gives an overview of receptor expression and resulting biological effects in the different cellular compartments.
TRP Channel Function—General Role in Immune Cells and Epithelia
TRPA1
TRPA1 is an irritant receptor that belongs to the ankyrin subfamily and is highly co-expressed with TRPV1 in a subset of sensory neurons (5). Only very little is known to date about TRPA1 expression in immune cells. The role of TRPA1 in macrophages was recently investigated in the context of the pathogenesis of atherosclerosis. Oxidized low-density lipoprotein (oxLDL) and the prototypical TRPA1 agonist allyl isothiocyanate (pungent ingredient in garlic) induced calcium transients in bone marrow-derived macrophages via TRPA1. TRPA1 expression was found to be upregulated in macrophage foam cells in atherosclerotic aortas of apolipoprotein E-deficient (apoE−/−) mice. Treatment with a selective TRPA1 antagonist HC030031 (HC) led to aggravation of oxLDL-induced lipid accumulation and subsequently exacerbated atherosclerotic lesions in apoE−/− mice. In addition, HC-treated apoE−/− mice showed increased levels of serum HDL, triglycerides, total cholesterol, and the pro-inflammatory cytokines IL-1β, TNF-α, MCP-1, IL-6, and macrophage inflammatory protein-2 (MIP-2), which suggested a crucial anti-inflammatory role of TRPA1 in the pathogenesis of atherosclerosis and cholesterol metabolism of macrophage foam cells (6). Previously, another group provided evidence about the functional expression of TRPA1 in peritoneal macrophages. LPS-stimulated cannabichromene-treated (CBC, cannabinoid TRPA1 agonist) peritoneal macrophages showed a significantly decreased level of nitrite (stable metabolite of nitric oxide) compared to LPS-stimulated peritoneal macrophages without CBC pretreatment. Nitric oxide acts as an abundant pro-inflammatory mediator, which indicates anti-inflammatory effects of TRPA1 activation by CBC in peritoneal macrophages. Interestingly, the TRPA1 antagonists AP-18 and HC had almost the same inhibitory effect on the nitrite production as TRPA1 activation, which indicated that the effect of TRPA1 agonists was due to receptor activation and subsequent desensitization (7). The TRPA1 agonists acrolein and crotonaldehyde were able to excite the release of TNF-α and IL-8 (CXCL8, a potent neutrophil chemoattractant) from the human macrophage cell line U937, whereas acrolein induced release of IL-8 from the THP-1 macrophage cell line and from human alveolar macrophages. In addition, the lipid peroxidation product 4-hydroxy-2-nonenal (4-HNE), a mediator of oxidative stress and a TRPA1 agonist was found to be upregulated in lungs of patients with chronic obstructive pulmonary disease (COPD), and induced release of IL-8 from U937 cells. Conversely, saturated aldehydes had no effect. This indicated that alpha, beta-unsaturated aldehydes such as 4-HNE (an ingredient of cigarette smoke) are likely to be pivotal in activating macrophages that may ultimately result in the destructive inflammatory reaction involved in the course of disease in COPD (8).
TRPA1 is also expressed in cultured human airway cells including epithelial cells, smooth muscle cells, and fibroblasts. In vitro, acrolein and cigarette smoke aqueous extract (CSE) (both TRPA1 agonists) induced the release of IL-8 TRPA1 dependently which was reduced by pharmacological TRPA1 blockade. TRPA1 expression was highly co-localized with TRPV1 expression in airway sensory nerves and the activation of both TRPA1 and TRPV1 channels induced the release of the pro-inflammatory neuropeptide SP. Interestingly however, the pro-inflammatory effects of acrolein and CSE were independent of sensory neuronal activation. After 24 h of intra-tracheal instillation with both compounds, neutrophil chemoattractant chemokine (KC) was increased in bronchoalveolar lavage (BAL) independent of pretreatment with a SP receptor/NK1 antagonist. In contrast, intra-tracheal instillation of capsaicin or SP had no effect on KC levels. Furthermore, pretreatment with a TRPA1 antagonist (HC) decreased KC release. Moreover, BAL from TRPA1-deficient mice did not show any release of acrolein- and CSE-induced KC. Thus, KC accumulation-derived inflammation was independent of neurogenic factors, and non-neuronal TRPA1 was shown to be essential in this model of inflammatory airway disorder (9).
In line with these observations of TRPA1 acting as an immune modulator, TRPA1 expression was detected by northern blot, western blot, and immunohistochemical methods in Jurkat T cells as well as in human splenocytes (10). Bertin et al. also confirmed the expression of mouse and human TRPA1 at mRNA and protein level in murine T cells (11).
TRPM8
TRPM8 is characterized in peripheral sensory neurons as a cold sensor (12, 13). Increasing evidence is accumulating that TRPM8 might also be implicated in inflammatory disorders. Previously, direct evidence for TRPM8 expression in macrophages was reported. TRPM8-like channels could be activated by the TRPM8 agonist icilin measured by the patch-clamp technique in RAW 264.7 macrophages (14). Recently, we observed evidence for TRPM8 expression in several populations of murine macrophages, which modulated inflammatory responses. In vitro, TRPM8 activation by menthol induced an anti-inflammatory cytokine profile in murine peritoneal macrophages (increased IL-10 and decreased TNF-α release) (15). Consistently, 1,8-cineol (eucalyptol) and L-menthol (both TRPM8 agonist) were able to inhibit the production of pro-inflammatory cytokines in human monocytes and lymphocytes in vitro (16, 17). In our own studies, activation of TRPM8 in wild-type (WT) but not in TRPM8-deficient peritoneal macrophages enhanced phagocytosis of zymosan beads. In vivo, phagocytic activity of peritoneal macrophages was impaired in TRPM8-deficient mice compared to WT controls (15).
TRPM8 was also found in human lung epithelial cells. Activation of this channel increased the expression of several cytokines and chemokines, including TNF-α, IL-4, IL-13, IL-1α, and IL-1ß, that modulate the response of other resident cell types in the lung such as immune cells, smooth muscle cells, and sensory neurons (18).
TRPV1
TRPV1 is expressed in sensory neurons of dorsal root ganglia (DRG), trigeminal, and vagal ganglia (19). Due to its high abundance in nociceptive neurons, TRPV1 acts as a nociceptor marker (20). Most of the TRPV1 expressing DRG neurons co-express peptidergic markers such as SP and CGRP (20). TRPV1 is activated by noxious stimuli such as capsaicin, extracellular acidification, or heat and is sensitized or activated by inflammatory mediators in vitro (21–23). Zhao and colleagues found that oxLDL-stimulated bone marrow-derived macrophages showed an increased level of TRPV1 expression and oxLDL activated TRPV1 which led to intracellular Ca2+-transients that were abolished by superfusion with the TRPV1 antagonist capsazepine. Capsazepine aggravated the oxLDL-induced lipid accumulation and induced the production of MCP-1 and IL-6 in macrophages. In contrast, pretreatment of bone marrow-derived macrophages with evodiamine or capsaicin (TRPV1 agonists) alleviated lipid accumulation and impaired the production of MCP-1, MIP-2, and IL-6 (24). Capsaicin application to LPS- and IFN-γ-stimulated RAW 264.7 macrophages exhibited inhibitory effects on iNOS and the production of NO, COX-2, and PGE2 in a concentration-dependent manner. Capsazepine failed to abolish the effect of capsaicin but rather showed similar inhibitory effects even synergistic with capsaicin on PGE2 released from LPS-stimulated peritoneal macrophages (25). In another report, capsaicin failed to modulate the protein/mRNA levels of COX-2, whereas capsazepine exhibited an inhibitory effect on the COX-2 levels produced from LPS-stimulated peritoneal macrophages (25). Both capsaicin and capsazepine decreased iNOS mRNA levels in LPS/IFN-γ-stimulated peritoneal macrophages in a concentration-dependent manner (25). Since iNOS and COX-2 are regulated by transcription factors like nuclear transcription factor kB (NF-kB) (26), it was examined, whether capsaicin or capsazepine regulated the activation of NF-kB. Both, capsaicin and capsazepine, blocked the degradation of IkB-a induced by LPS-stimulated peritoneal macrophages, reflecting that both capsaicin and capsazepine inhibit the activation of NF-kB. Due to the inability of capsazepine to block the effect of capsaicin, the authors suggested that peritoneal macrophages do not express TRPV1 but rather a TRPV1-like protein (25). Intriguingly, these results become more complicated in their interpretation since we were recently able to show that capsazepine is also a potent TRPA1 agonist (2).
In a sepsis model of cecal ligation and puncture, LPS-stimulated TRPV1-deficient peritoneal macrophages showed impaired phagocytosis compared to unstimulated controls (27). LPS/SP co-stimulated TRPV1−/− macrophages were shown to restore phagocytic activity, an effect that was abolished by pretreatment with a selective antagonist of the SP/NK1 receptor. In contrast, CGRP-stimulated TRPV1-deficient macrophages did not show a significant difference in response to LPS (27). Furthermore, a TRPV1 antagonist decreased phagocytosis of LPS-stimulated WT macrophages compared to control cells (27). Recently, a previously unknown role of the endocannabinoid system in regulating immune homeostasis TRPV1 dependently was reported. Activation of TRPV1 by capsaicin induced production of the endocannabinoid anandamide in myeloid cells and promoted the presence of immunosuppressive CXCR1hi macrophages via anandamide acting on CB2 receptors expressed in the enteric nervous system. Moreover, this mechanism also provided protection from experimental autoimmune diabetes (28).
Amantini et al. could demonstrate that distinct thymocyte subsets express TRPV1, which is required for capsaicin-induced apoptosis (29). Functional expression of TRPV1 was furthermore shown by Bertin et al. in primary murine CD4+ T cells (30). The authors could show that the co-stimulatory molecules CD4 and TRPV1 were co-localized within the plasma cell membrane of CD4+ T cells and stimulation with capsaicin triggered calcium ion influx.
In bronchial epithelial cells, TRPV1 was able to control the expression of pro-inflammatory cytokines such as IL-6 and IL-8 due to its modulation of calcium traffic between the intra- and extracellular compartment (31). IL-8 is an essential chemotactic protein produced by neutrophils in the lung (32), which provides the molecular basis for a vital epithelial–immune interaction.
TRPV4
TRPV4 was originally identified to be involved in the regulation of osmotic homeostasis (33). Furthermore, TRPV4 is activated by mechanical stress and non-noxious heat (34). The prominent role of TRPV4 in visceral nociception was highlighted by recent work (35, 36). Beyond TRPV4 function in neurons, several reports suggest an important physiological function of non-neuronal TRPV4. TRPV4 is expressed in lung and gut epithelial cells and immune cells including macrophages, monocytes, neutrophils, and T cells (4, 37). Functional TRPV4 expression was recently observed in bone marrow-derived macrophages. The selective TRPV4 agonist GSK1016790A increased the intracellular calcium ion concentration in a dose-dependent manner, an effect that was inhibited by TRPV4 siRNA or a pharmacological blocker and completely abolished in TRPV4-deficient bone marrow-derived macrophages. Using fluorometry in vitro and quantifying phagocyted particles in vivo, the authors also observed impaired phagocytosis after downregulation of TRPV4 with siRNA in LPS-treated bone marrow-derived macrophages (38).
TRPV4 expression was shown in human airway epithelial cell lines (A549, Beas 2B, and NCI-H292) and primary airway epithelial cells. Selective TRPV4 agonists were shown to trigger calcium influx in NCI-H292 and increased the release and secretion of IL-8 and PGE2 in a time- and concentration-dependent manner (37). In vivo, intranasal administration of the TRPV4 agonist 4α-PDD enhanced the concentration of KC, which subsequently led to the recruitment of neutrophils in BAL fluids in WT but not TRPV4-deficient mice (37), indicating an indirect epithelial–immunological interaction. However, a direct role for TRPV4 expression in the function of murine alveolar macrophages was also demonstrated. 4α-PDD triggered calcium influx and subsequently production of superoxide and nitric oxide in alveolar macrophages of WT but not TRPV4-deficient mice. Likewise, the adoptive transfer of TRPV4-expressing alveolar macrophages into lungs of TRPV4−/− mice restored hypersusceptibility in a model of ventilator-induced mechanical injury (39).
In line with these pro-inflammatory functions of TRPV4, Majhi and colleagues found endogenous expression of different TRPV isoforms in Jurkat, primary human T cells, and mouse T cells isolated from spleens. Moreover, they observed calcium influx in T cells upon treatment with different TRPV4 agonists. Furthermore, in vitro activation of TRPV4 in T cells resulted in upregulation of TRPV1 and TRPV4 channels, T cell proliferation, and production of the pro-inflammatory cytokines IFN-γ, TNF-α, and IL-2. This effect was blocked pharmacologically suggesting that TRPV4-mediated calcium influx might play a crucial role in T cell-mediated immune responses (40).
TRP Channels in Immune Cells and Epithelia—Role in Models of Intestinal Inflammation
The majority of published literature ascribes the role of TRP channels in modulation of experimental colitis to its capacity to attenuate neuropeptide release and subsequently neurogenic inflammation. In accordance with this, we have found that TRPA1 and the pro-inflammatory neuropeptide SP in extrinsic primary afferent neurons are fundamental for the development of TNBS colitis. Pharmacological blocking of TRPA1 attenuated chronic colitis through inhibition of neuropeptide release (41). However, the role of extra-neuronally expressed TRP channels in the pathogenesis of intestinal inflammation is emerging and results in a complex crosstalk of different cellular compartments (see Figure 1). A recent study stressed the important role of extra-neuronal TRPA1 and TRPV1 receptor expression in this context. TRPA1 and TRPV1 were expressed in macrophages and epithelial cells in both healthy and inflamed human and murine colons (42). Since macrophages are major producers of TNF-α and activation of TRPA1 was able to impair the expression of TNF-α in distal colon homogenates during colitis, it is likely that TRPA1 in macrophages mediated the anti-colitogenic effect. In addition, employing different mouse models of colitis including IL-10 knockout mice as well as adoptive T cell transfer models of colitis, it was shown that the genetic deletion of TRPA1 in CD4+ T cells caused intestinal inflammation via induction of the transcription factor Tbet which subsequently increased production of the pro-inflammatory cytokines IFN-γ and IL-2 (11) and resulted in a higher capacity to differentiate into TH1 effector cells. Additionally, CD4+ T cells isolated from TRPV1 knockout mice showed impaired calcium influx upon T cell receptor stimulation, resulting in the inactivation of the transcription factors NFAT and NFkB. Likewise, TRPV1-deficient CD4+ T cells failed to induce colitis in transfer models of colitis as TRPV1-deficient CD4+ T cells showed decreased production of the pro-inflammatory cytokines IFN-γ, TNF-α, and IL-17 (30). An important extra-neuronal role of TRPV4 was recently demonstrated with regard to colonic inflammation. TRPV4 mRNA was found to be upregulated in colonic intestinal epithelial cells from mice with dextran sulfate sodium (DSS)-induced colitis. TRPV4 activation led to chemokine and cytokine release such as IL-8, IP-10, MIG, and MCP-1, which indicated a potential role of TRPV4 in activating pro-inflammatory signaling pathways that might induce the recruitment of macrophages and other immune cells. In addition, intrarectal enemas with the TRPV4 agonist 4α-PDD induced acute and chronic colonic inflammation in mice (4). Finally, we could recently show that mice that were reconstituted with TRPM8-deficient macrophages exhibited increased susceptibility to DSS, demonstrating a fundamental role of constitutive TRPM8 expression in macrophages in the context of colitis (15). Two recent reports, however, favor a dominant role for TRPM8 expression in controlling neuropeptide release and, thus, colitis development (43, 44). TRPM8 was shown to modulate the release of CGRP in the colonic microenvironment from peptidergic sensory neurons, which might have directed the protective effects of CGRP on CD11+ dendritic cells (44).
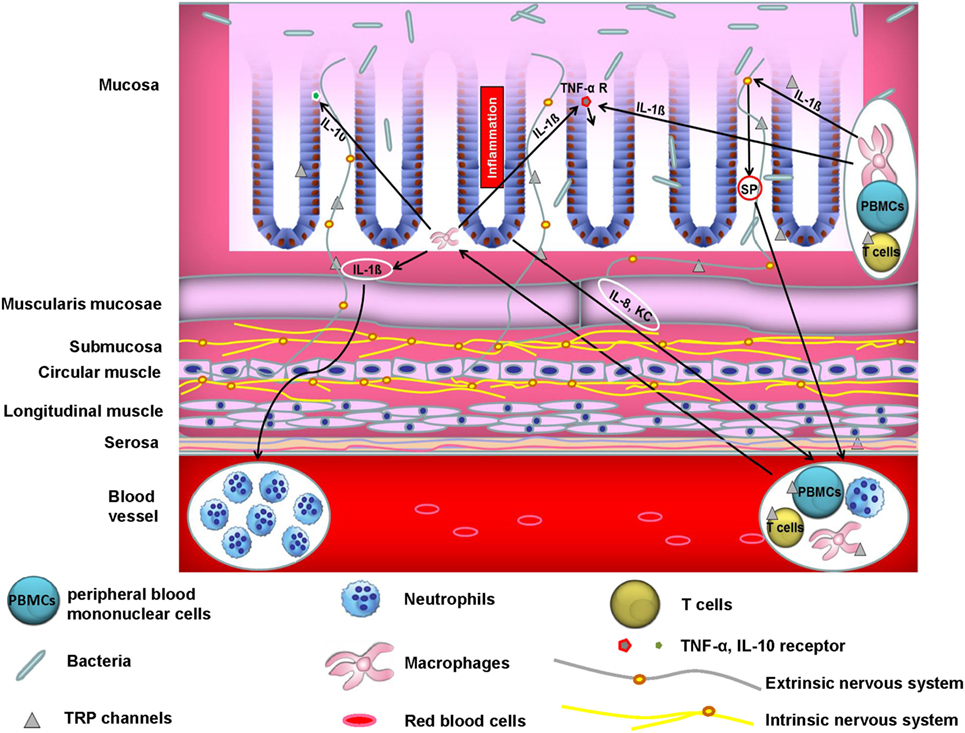
Figure 1. Crosstalk between neurons, immune cells, and epithelial cells controls colonic homeostasis. A vital interplay between neurons, immune cells, and epithelial cells is crucial for colonic homeostasis. Aberrant function of one or more of these players may cause inflammation. For instance, activated macrophages release IL-1ß which among other cytokines acts as a chemoattractant to human peripheral blood mononuclear cells (PBMCs) (45). On the other hand, IL-1ß increases TNF-α receptor expression in epithelial cells which may promote TNF-α-mediated inflammatory responses and subsequently colitis. Moreover, IL-1ß acts on peptidergic sensory neurons through induction of pro-inflammatory substance P (SP) release. SP in turn induces migration of polymorphonuclear leukocytes in vivo (46). In addition, epithelial IL-8 and KC induce immune cell infiltration into the colonic wall. IL-10 is an essential immunoregulatory cytokine (47). Epithelial cells from murine small intestine and colon express the IL-10 receptor and its stimulation blocks IFN-γ-mediated pro-inflammatory effects (48). Moreover, not only epithelial cells but also enteric neurons express cytokines and chemokines such as IL-8, whose neuronal production is promoted by IL-1ß via MAPK signaling pathways (49).
Conclusion
The role of TRP channels reaches beyond the control of immunomodulatory neuropeptide release from sensory nerve endings. Many TRP channels are expressed in various immune cells, especially in macrophages and T cells. Here, they modulate many functions such as cytokine expression and release, migration, or phagocytic activity. Moreover, in a third compartment, the epithelial layer, TRP channel expression was also found to be relevant in the pathogenesis of many inflammatory disorders mainly through controlling chemokine/cytokine expression and release. Thus, a vital interplay between neurons, epithelia, and mucosal immune cells seems to maintain homeostasis in different organs, for example, the gut, the lung, and the vascular system and disruption of one or more of these players may induce disease (see Figure 1). Thus, targeting TRP channels and neuropeptide receptors might represent a promising new therapeutic approach in various inflammatory disorders such as inflammatory bowel disease, asthma, COPD, and atherosclerosis.
Author Contributions
MK: conception and writing of the manuscript; KA: conception and writing of the manuscript; CW and CY: conception and writing of the manuscript; SW and CB: conception of the manuscript, revising it critically for important intellectual content, and final approval of the version to be published; ME: conception and writing of the manuscript, revising it critically for important intellectual content and final approval of the version to be published.
Conflict of Interest Statement
The authors declare that the research was conducted in the absence of any commercial or financial relationships that could be construed as a potential conflict of interest.
Acknowledgments
Thank you to Rachel Rouse (MD, Medical Department 1) for linguistic revision of the manuscript.
Funding
MK and ME received support from the Deutsche Forschungsgemeinschaft (DFG EN 1060/2-1, CRU/KFO 257), Marohn-Stiftung, ELAN, IZKF, and Universitaetsbund of the Friedrich-Alexander-Universitat Erlangen-Nuernberg.
Supplementary Material
The Supplementary Material for this article can be found online at http://www.frontiersin.org/articles/10.3389/fimmu.2018.00174/full#supplementary-material.
Table S1. Expression and biological effects of the different TRP channels in immune cells and epithelia.
References
1. Clapham DE, Julius D, Montell C, Schultz G. International Union of Pharmacology. XLIX. Nomenclature and structure-function relationships of transient receptor potential channels. Pharmacol Rev (2005) 57:427–50. doi:10.1124/pr.57.4.6
2. Kistner K, Siklosi N, Babes A, Khalil M, Selescu T, Zimmermann K, et al. Systemic desensitization through TRPA1 channels by capsazepine and mustard oil – a novel strategy against inflammation and pain. Sci Rep (2016) 6:28621. doi:10.1038/srep28621
3. Caterina MJ, Leffler A, Malmberg AB, Martin WJ, Trafton J, Petersen-Zeitz KR, et al. Impaired nociception and pain sensation in mice lacking the capsaicin receptor. Science (2000) 288:306–13. doi:10.1126/science.288.5464.306
4. D’Aldebert E, Cenac N, Rousset P, Martin L, Rolland C, Chapman K, et al. Transient receptor potential vanilloid 4 activated inflammatory signals by intestinal epithelial cells and colitis in mice. Gastroenterology (2011) 140:275–85. doi:10.1053/j.gastro.2010.09.045
5. Story GM, Peier AM, Reeve AJ, Eid SR, Mosbacher J, Hricik TR, et al. ANKTM1, a TRP-like channel expressed in nociceptive neurons, is activated by cold temperatures. Cell (2003) 112:819–29. doi:10.1016/S0092-8674(03)00158-2
6. Zhao JF, Shyue SK, Kou YR, Lu TM, Lee TS. Transient receptor potential ankyrin 1 channel involved in atherosclerosis and macrophage-foam cell formation. Int J Biol Sci (2016) 12:812–23. doi:10.7150/ijbs.15229
7. Romano B, Borrelli F, Fasolino I, Capasso R, Piscitelli F, Cascio M, et al. The cannabinoid TRPA1 agonist cannabichromene inhibits nitric oxide production in macrophages and ameliorates murine colitis. Br J Pharmacol (2013) 169:213–29. doi:10.1111/bph.12120
8. Facchinetti F, Amadei F, Geppetti P, Tarantini F, Di Serio C, Dragotto A, et al. α,β-unsaturated aldehydes in cigarette smoke release inflammatory mediators from human macrophages. Am J Respir Cell Mol Biol (2007) 37:617–23. doi:10.1165/rcmb.2007-0130OC
9. Nassini R, Pedretti P, Moretto N, Fusi C, Carnini C, Facchinetti F, et al. Transient receptor potential ankyrin 1 channel localized to non-neuronal airway cells promotes non-neurogenic inflammation. PLoS One (2012) 7:e42454. doi:10.1371/journal.pone.0042454
10. Stokes A, Wakano C, Koblan-Huberson M, Adra CN, Fleig A, Turner H. TRPA1 is a substrate for de-ubiquitination by the tumor suppressor CYLD. Cell Signal (2006) 18:1584–94. doi:10.1016/j.cellsig.2005.12.009
11. Bertin S, Aoki-Nonaka Y, Lee J, de Jong PR, Kim P, Han T, et al. The TRPA1 ion channel is expressed in CD4+ T cells and restrains T-cell-mediated colitis through inhibition of TRPV1. Gut (2016) 66:1584–96. doi:10.1136/gutjnl-2015-310710
12. Peier AM, Moqrich A, Hergarden AC, Reeve AJ, Andersson DA, Story GM, et al. A TRP channel that senses cold stimuli and menthol. Cell (2002) 108:705–15. doi:10.1016/S0092-8674(02)00652-9
13. McKemy DD, Neuhausser WM, Julius D. Identification of a cold receptor reveals a general role for TRP channels in thermosensation. Nature (2002) 416:52–8. doi:10.1038/nature719
14. Wu SN, Wu PY, Tsai ML. Characterization of TRPM8-like channels activated by the Cooling Agent Icilin in the macrophage cell line RAW 264.7. J Membr Biol (2011) 241:11. doi:10.1007/s00232-011-9358-6
15. Khalil M, Babes A, Lakra R, Forsch S, Reeh PW, Wirtz S, et al. Transient receptor potential melastatin 8 ion channel in macrophages modulates colitis through a balance-shift in TNF-alpha and interleukin-10 production. Mucosal Immunol (2016) 9:1500–13. doi:10.1038/mi.2016.16
16. Juergens UR, Engelen T, Racké K, Stöber M, Gillissen A, Vetter H. Inhibitory activity of 1,8-cineol (eucalyptol) on cytokine production in cultured human lymphocytes and monocytes. Pulm Pharmacol Ther (2004) 17:281–7. doi:10.1016/j.pupt.2004.06.002
17. Juergens UR, Stöber M, Vetter H. The anti-inflammatory activity of L-menthol compared to mint oil in human monocytes in vitro: a novel perspective for its therapeutic use in inflammatory diseases. Eur J Med Res (1998) 3:539–45.
18. Sabnis AS, Reilly CA, Veranth JM, Yost GS. Increased transcription of cytokine genes in human lung epithelial cells through activation of a TRPM8 variant by cold temperatures. Am J Physiol Lung Cell Mol Physiol (2008) 295:L194–200. doi:10.1152/ajplung.00072.2008
19. Clapham DE, Runnels LW, Strubing C. The trp ion channel family. Nat Rev Neurosci (2001) 2:387–96. doi:10.1038/35077544
20. Hwang SJ, Min Oh J, Valtschanoff JG. Expression of the vanilloid receptor TRPV1 in rat dorsal root ganglion neurons supports different roles of the receptor in visceral and cutaneous afferents. Brain Res (2005) 1047(2):261–6. doi:10.1016/j.brainres.2005.04.036
21. Kichko TI, Reeh PW. TRPV1 controls acid- and heat-induced calcitonin gene-related peptide release and sensitization by bradykinin in the isolated mouse trachea. Eur J Neurosci (2009) 29:1896–904. doi:10.1111/j.1460-9568.2009.06747.x
22. Ferreira J, da Silva GL, Calixto JB. Contribution of vanilloid receptors to the overt nociception induced by B(2) kinin receptor activation in mice. Br J Pharmacol (2004) 141:787–94. doi:10.1038/sj.bjp.0705546
23. Davis JB, Gray J, Gunthorpe MJ, Hatcher JP, Davey PT, Overend P, et al. Vanilloid receptor-1 is essential for inflammatory thermal hyperalgesia. Nature (2000) 405:183–7. doi:10.1038/35012076
24. Zhao JF, Ching LC, Kou YR, Lin SJ, Wei J, Shyue SK, et al. Activation of TRPV1 prevents OxLDL-induced lipid accumulation and TNF-α-induced inflammation in macrophages: role of liver X receptor α. Mediators Inflamm (2013) 2013:925171. doi:10.1155/2013/925171
25. Kim CS, Kawada T, Kim BS, Han IS, Choe SY, Kurata T, et al. Capsaicin exhibits anti-inflammatory property by inhibiting IkB-a degradation in LPS-stimulated peritoneal macrophages. Cell Signal (2003) 15:299–306. doi:10.1016/S0898-6568(02)00086-4
26. Surh YJ, Chun KS, Cha HH, Han SS, Keum YS, Park KK, et al. Molecular mechanisms underlying chemopreventive activities of anti-inflammatory phytochemicals: down-regulation of COX-2 and iNOS through suppression of NF-κB activation. Mutat Res (2001) 480–481:243–68. doi:10.1016/S0027-5107(01)00183-X
27. Fernandes ES, Liang L, Smillie SJ, Kaiser F, Purcell R, Rivett DW, et al. TRPV1 deletion enhances local inflammation and accelerates the onset of systemic inflammatory response syndrome. J Immunol (2012) 188:5741–51. doi:10.4049/jimmunol.1102147
28. Acharya N, Penukonda S, Shcheglova T, Hagymasi AT, Basu S, Srivastava PK. Endocannabinoid system acts as a regulator of immune homeostasis in the gut. Proc Natl Acad Sci U S A (2017) 114:5005–10. doi:10.1073/pnas.1612177114
29. Amantini C, Mosca M, Lucciarini R, Perfumi M, Morrone S, Piccoli M, et al. Distinct thymocyte subsets express the vanilloid receptor VR1 that mediates capsaicin-induced apoptotic cell death. Cell Death Differ (2004) 11:1342–56. doi:10.1038/sj.cdd.4401506
30. Bertin S, Aoki-Nonaka Y, de Jong PR, Nohara LL, Xu H, Stanwood SR, et al. The ion channel TRPV1 regulates the activation and proinflammatory properties of CD4+ T cells. Nat Immunol (2014) 15:1055–63. doi:10.1038/ni.3009
31. Reilly CA, Johansen ME, Lanza DL, Lee J, Lim JO, Yost GS. Calcium-dependent and independent mechanisms of capsaicin receptor (TRPV1)-mediated cytokine production and cell death in human bronchial epithelial cells. J Biochem Mol Toxicol (2005) 19:266–75. doi:10.1002/jbt.20084
32. Kunkel SL, Standiford T, Kasahara K, Strieter RM. Interleukin-8 (IL-8): the major neutrophil chemotactic factor in the lung. Exp Lung Res (1991) 17:17–23. doi:10.3109/01902149109063278
33. Liedtke W, Friedman JM. Abnormal osmotic regulation in trpv4-/- mice. Proc Natl Acad Sci U S A (2003) 100:13698–703. doi:10.1073/pnas.1735416100
34. Jin M, Wu Z, Chen L, Jaimes J, Collins D, Walters ET, et al. Determinants of TRPV4 activity following selective activation by small molecule agonist GSK1016790A. PLoS One (2011) 6:e16713. doi:10.1371/journal.pone.0016713
35. Mueller-Tribbensee SM, Karna M, Khalil M, Neurath MF, Reeh PW, Engel MA. Differential contribution of TRPA1, TRPV4 and TRPM8 to colonic nociception in mice. PLoS One (2015) 10:e0128242. doi:10.1371/journal.pone.0128242
36. Brierley SM, Page AJ, Hughes PA, Adam B, Liebregts T, Cooper NJ, et al. Selective role for TRPV4 ion channels in visceral sensory pathways. Gastroenterology (2008) 134:2059–69. doi:10.1053/j.gastro.2008.01.074
37. Henry CO, Dalloneau E, Pérez-Berezo MT, Plata C, Wu Y, Guillon A, et al. In VITRO and in vivo evidence for an inflammatory role of the calcium channel TRPV4 in lung epithelium: potential involvement in cystic fibrosis. Am J Physiol Lung Cell Mol Physiol (2016) 311:664–75. doi:10.1152/ajplung.00442.2015
38. Scheraga RG, Abraham S, Niese KA, Southern BD, Grove LM, Hite RD, et al. TRPV4 mechanosensitive ion channel regulates lipopolysaccharide-stimulated macrophage phagocytosis. J Immunol (2016) 196:428–36. doi:10.4049/jimmunol.1501688
39. Hamanaka K, Jian MY, Townsley MI, King JA, Liedtke W, Weber DS, et al. TRPV4 channels augment macrophage activation and ventilator-induced lung injury. Am J Physiol Lung Cell Mol Physiol (2010) 299:353–62. doi:10.1152/ajplung.00315.2009
40. Majhi RK, Sahoo SS, Yadav M, Pratheek BM, Chattopadhyay S, Goswami C. Functional expression of TRPV channels in T cells and their implications in immune regulation. FEBS J (2015) 282:2661–81. doi:10.1111/febs.13306
41. Engel MA, Leffler A, Niedermirtl F, Babes A, Zimmermann K, Filipovic MR, et al. TRPA1 and substance P mediate colitis in mice. Gastroenterology (2011) 141:1346–58. doi:10.1053/j.gastro.2011.07.002
42. Kun J, Szitter I, Kemény Á, Perkecz A, Kereskai L, Pohóczky K, et al. Upregulation of the transient receptor potential ankyrin 1 ion channel in the inflamed human and mouse colon and its protective roles. PLoS One (2014) 9:e108164. doi:10.1371/journal.pone.0108164
43. Ramachandran R, Hyun E, Zhao L, Lapointe TK, Chapman K, Hirota CL, et al. TRPM8 activation attenuates inflammatory responses in mouse models of colitis. Proc Natl Acad Sci U S A (2013) 110:7476–81. doi:10.1073/pnas.1217431110
44. de Jong PR, Takahashi N, Peiris M, Bertin S, Lee J, Gareau MG, et al. TRPM8 on mucosal sensory nerves regulates colitogenic responses by innate immune cells via CGRP. Mucosal Immunol (2015) 8:491–504. doi:10.1038/mi.2014.82
45. Tixier E, Galmiche JP, Neunlist M. Intestinal neuro-epithelial interactions modulate neuronal chemokines production. Biochem Biophys Res Commun (2006) 344:554–61. doi:10.1016/j.bbrc.2006.03.159
46. Perretti M, Ahluwalia A, Flower RJ, Manzini S. Endogenous tachykinins play a role in IL-1-induced neutrophil accumulation: involvement of NK-1 receptors. Immunology (1993) 80:73–7.
47. Chung EY, Liu J, Homma Y, Zhang Y, Brendolan A, Saggese M, et al. Interleukin-10 expression in macrophages during phagocytosis of apoptotic cells is mediated by homeodomain proteins Pbx1 and Prep-1. Immunity (2007) 27:952–64. doi:10.1016/j.immuni.2007.11.014
48. Denning TL, Campbell NA, Song F, Garofalo RP, Klimpel GR, Reyes VE, et al. Expression of IL-10 receptors on epithelial cells from the murine small and large intestine. Int Immunol (2000) 12:133–9. doi:10.1093/intimm/12.2.133
Keywords: colitis, macrophages, cytokines, neuropeptides, neurogenic inflammation, nociception
Citation: Khalil M, Alliger K, Weidinger C, Yerinde C, Wirtz S, Becker C and Engel MA (2018) Functional Role of Transient Receptor Potential Channels in Immune Cells and Epithelia. Front. Immunol. 9:174. doi: 10.3389/fimmu.2018.00174
Received: 20 July 2017; Accepted: 19 January 2018;
Published: 07 February 2018
Edited by:
Mats Bemark, University of Gothenburg, SwedenReviewed by:
Milena Bogunovic, Pennsylvania State University, United StatesClaudio Nicoletti, University of Florence, Italy
Copyright: © 2018 Khalil, Alliger, Weidinger, Yerinde, Wirtz, Becker and Engel. This is an open-access article distributed under the terms of the Creative Commons Attribution License (CC BY). The use, distribution or reproduction in other forums is permitted, provided the original author(s) and the copyright owner are credited and that the original publication in this journal is cited, in accordance with accepted academic practice. No use, distribution or reproduction is permitted which does not comply with these terms.
*Correspondence: Matthias Agop Engel, bWF0dGhpYXMuZW5nZWxAdWstZXJsYW5nZW4uZGU=