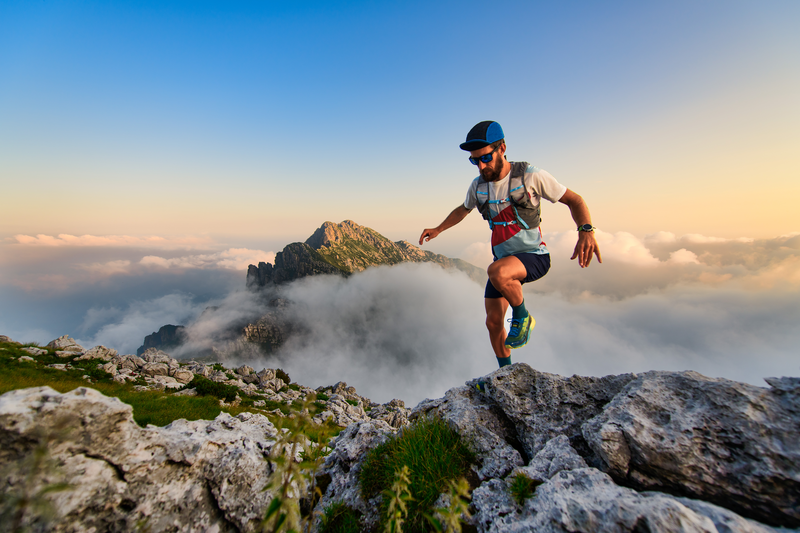
94% of researchers rate our articles as excellent or good
Learn more about the work of our research integrity team to safeguard the quality of each article we publish.
Find out more
MINI REVIEW article
Front. Immunol. , 12 January 2018
Sec. Inflammation
Volume 8 - 2017 | https://doi.org/10.3389/fimmu.2017.01981
This article is part of the Research Topic Regulation of Inflammation in Chronic Disease View all 48 articles
CCR5 and its interaction with chemokine ligands have been crucial for understanding and tackling HIV-1 entry into target cells. However, over time, CCR5 has witnessed an impressive transition from being considered rather unimportant in physiology and pathology to becoming central in a growing number of pathophysiological conditions. It now turns out that the massive efforts devoted to combat HIV-1 entry by interfering with CCR5, and the subsequent production of chemokine ligand variants, small chemical compounds, and other molecular entities and strategies, may set the therapeutic standards for a wealth of different pathologies. Expressed on various cell types, CCR5 plays a vital role in the inflammatory response by directing cells to sites of inflammation. Aside HIV-1, CCR5 has been implicated in other infectious diseases and non-infectious diseases such as cancer, atherosclerosis, and inflammatory bowel disease. Individuals carrying the CCR5Δ32 mutation live a normal life and are warranted a natural barrier to HIV-1 infection. Therefore, CCR5 antagonism and gene-edited knockout of the receptor gained growing interest for the therapeutic role that CCR5 blockade may play in the attenuation of the severity or progression of numerous diseases.
From its discovery, CCR5 has been a key player in HIV-1 entry into target cells and, together with its chemokine ligands, helped in understanding and tackling HIV-1 infection (1, 2). CCR5 predominates among the chemokine co-receptors used by HIV-1 for cell entry, and R5-tropic HIV-1 strains are those most commonly transmitted in the early stages of infection. A 32 base pair deletion within the CCR5 gene leads to a non-functional gene product that does not reach the cell surface, and subjects with a homozygous CCR5Δ32 deletion are protected from HIV-1 infection (3).
The discovery and implication of CCR5, CXCR4, and their chemokine ligands in HIV-1 pathogenesis triggered massive research efforts that cross-fertilized many biomedical fields related to the chemokine system and regulation. In recent years, evidence has accumulated that CCR5 and its ligands may play a role in various inflammatory diseases, as cellular activation of CCR5 normally happens through chemokine binding, which then regulate intracellular trafficking and protective cellular and humoral responses. Indeed, the migration of lymphocytes to inflammatory areas is controlled by chemokine gradients (4). CCR5 may also be relevant in the development of various types of cancer, as tumor cells directly secrete or induce fibroblasts to secrete CCL5, which maintain proliferation of CCR5-positive cancer cells. Finally, CCR5 may play a role in autoimmune diseases such as rheumatoid arthritis and multiple sclerosis (MS).
In this mini-review, we describe several aspects related to the pathophysiology of CCR5, discuss its possible dispensability, and analyze its blockade as a comprehensive therapeutic perspective.
Soon after its discovery and implication for HIV-1 entry, CCR5 has been the subject of extensive research as a possible new player in the search for preventative and therapeutic solutions to the HIV-1 infection pandemic (2). A radical approach to CCR5 targeting could be the elimination of the receptor by gene editing, in an attempt to resemble the naturally occurring Δ32 deletion (5) (Figure 1). This approach has its foundation on the fact that individuals homozygous for the CCR5Δ32 deletion are seemingly healthy. The proof of concept for CCR5 elimination has been provided by the so-called Berlin patient, an HIV-1 infected person who, after a double CCR5Δ32 stem cell transplant, has remained HIV free (6, 7). However, the CCR5Δ32 mutation dates thousands of years and individuals that carry it naturally may have adapted their chemokine system to physiologically balance the absence of a functional CCR5 (8). Therefore, the effect of CCR5Δ32 stem cell transplants and artificially induced CCR5 knockouts should be considered carefully, and individuals subjected to these treatments should be followed up for a long time (9). Similar caution needs to be taken when acting on CCR5 with more conventional approaches (e.g., using CCR5 antagonists), although drug discontinuation is likely to restore normal CCR5 expression and function.
Figure 1. CCR5 blockade. CCR5 blockade may occur (i) naturally (triangle on the bottom), by the CCR5Δ32 deletion that prevents the receptor to be transported to the cell surface; (ii) via gene editing strategies (triangle on the left) that ablate the CCR5 gene; or (iii) by receptor antagonism (triangle on the right) using different molecular entities. The cell membrane is represented with a green rectangle. Ribbon representation of CCR5 and 5p7-CCL5 three-dimensional structures were generated using PyMOL from PDB entry 5UIW (10), MVC from PDB entry 4MBS (11), and the FAB fragment of RoAb13 from PDB entry 4S2S (12).
Therefore, CCR5 blockade is still an open question, as well as the genetic mechanism and environmental pressure that generated the CCR5Δ32 mutation. While HIV-1 cannot be accounted for the origins of the CCR5Δ32 mutation, these have been initially attributed to selective pressure by pathogens such as Yersinia pestis or variola virus. However, these hypotheses have been dismissed in favor of an older selection event connected to a different pathogen (13). Indeed, the CCR5Δ32 gene has been detected in Bronze Age skeletons (14) and is estimated to have emerged ~5,000 years ago, predating the time during which smallpox and plague became widespread human pathogens (13).
A role for CCR5 has been suggested in numerous diseases, many involving the nervous system. CCR5 ligands are produced in the central nervous system (CNS) by microglia, astrocytes, endothelial cells, and even neurons (15, 16). The cerebrospinal fluid (CSF) of patients with relapsing-remitting MS has CCR2+CCR5+ TH1 cells during a relapse; CCR5+CD8+ T cells and CCR5+ monocytes are higher in the CSF than in the blood of patients with the disease, and CCR5 is expressed in inflammatory cells infiltrating the CNS in vivo (17, 18). CCR5 is also expressed on immune cells within inflammatory lesions in MS and may contribute to recruitment of these cells to the inflamed tissue or to their activation. Finally, the expression of CCR5 ligands has been shown at sites of inflammation in MS (19). Interestingly, MS can develop in people who are homozygous for the CCR5Δ32 mutation. The CCR5Δ32 allele is not associated with MS risk (20, 21), but the disease seems to be less severe in carriers of the allele (22), suggesting that CCR5 antagonists might diminish disease activity.
In contrast, homozygosity for the CCR5Δ32 allele is overrepresented in patients with symptomatic West Nile virus infection (23, 24) and is associated with severe meningoencephalitis in tick-borne encephalitis virus infections (25). Most likely, CCR5 facilitates clearance of these infections by promoting leukocyte trafficking to the CNS, a proof of its beneficial effects for human health (23). CCR5 may instead be detrimental in patients with cerebral malaria, in brain samples of whom it was found to be upregulated (26). The CCR5Δ32 allele seems to be associated with resistance to Crimean-Congo hemorrhagic fever (CCHF) virus infection, at least in the Turkish population (27). Indeed, CCL3, CCL4, and CCL5, natural ligands of CCR5, are associated with CCHF, and their levels are increased in adult patients with the infection (28).
In an emerging infectious disease, dengue virus infection, an association has been found with CCR5 expression, and the infection induces the expression of CCR5 ligands (29).
In its pathogenesis, Toxoplasma gondii produces a chemokine mimic that triggers CCR5, a subtle mechanism likely used to warrant T. gondii survival in the host (30). However, in the absence of CCR5, mice succumb to infection with uncontrolled parasite growth, altered lipid metabolism, hepatic steatosis, and widespread intestinal damage with ileum necrosis and prominent neutrophils infiltrate (31). Whether CCR5 is essential for T. gondii infection control in humans is unknown.
Poxviruses use chemokine receptors, including CCR5, to infect target cells; however, their molecular mechanism of receptor usage is distinct from that of HIV-1 (32). In a mouse model based on intranasal vaccinia virus infection, CCR5 expression in T cells contributes to the dissemination of the virus to the lungs and beyond; the data suggest that the role of CCR5 in vaccinia virus infection is not redundant and that CCR5 may be necessary for systemic infection in vivo (33).
Staphylococcus aureus is the cause of a large number of deadly infections worldwide, and the emergence of antibiotic-resistant S. aureus strains represents a steadily increasing global threat. The bicomponent pore-forming leukotoxin ED (LukED) is used by S. aureus to compromise the host immune system and cause deadly infectivity, and the gene for LukED is present in numerous clinically relevant S. aureus strains (34). LukE binds to human (and mouse) CCR5 on T cells, macrophages, and dendritic cells (35); subsequently, a bicomponent octamer formed by alternate LukE and LukD monomers assembles on the surface of target cells. The pores formed by LukED ultimately lead to cell death. LukED kills CCR5+ cells in vivo in mice, and animals lacking CCR5 are protected from mortality due to S. aureus infection (35). Even though both LukE and gp120 target CCR5, they use different determinants on the receptor (36). Interestingly, CCR5 antagonism by maraviroc (a small chemical HIV-1 entry inhibitor) confers mice with resistance to lethal S. aureus infection. Maraviroc completely blocks LukED pore formation in vitro and therefore toxicity toward CCR5+ cells (35). Therefore, the use of CCR5 antagonists to counteract S. aureus infection is an interesting example of antibacterial intervention, alternative or even complementary to antibiotics. In light of the debate on the emergence of the CCR5Δ32 mutation, the deadly effects of S. aureus infections on humankind and LukE tropism for CCR5 might have generated the ancient selection of the CCR5Δ32 allele (35).
CCR5 may also have a role in autoimmune diseases. In rheumatoid arthritis, increased levels of CCR5 ligands CCL3, CCL4, and CCL5 are found in the synovial fluid (37, 38), and the CCR5Δ32 variant seems to protect from the disease (39). However, maraviroc does not efficiently control inflammation in this setting (40).
CCR5 appears to be relevant in atherosclerosis and the development of related diseases (41). A meta-analysis of 13 studies assessed whether individuals carrying the CCR5Δ32 variant could be either protected or at risk for atherosclerosis-related cardiovascular diseases and indicated that the CCR5 Δ32-positive genotype (Δ32/Δ32 or wt/Δ32) increases the risk of atherosclerotic disease only in Asian populations (42). In a recent report, CCR5 has been described as a non-redundant, essential receptor for the homing of CD4 T cells that exacerbate atherosclerosis (43).
An increased expression of CCL5 has been detected as early as 8 days postpartum in a mouse model of tubulointerstitial kidney disease, an inflammatory disorder that causes progressive kidney damage and renal failure (44). It might be possible that CCL5 participates in the early cascade of event bridging the unfolded protein response (caused by an uromodulin mutation) to inflammation, although further investigations are needed (44).
CCL5 expression is increased in inflammatory bowel disease (IBD), likely pointing to a contribution by CCL5 in the progressive tissue destruction during the inflammatory processes (45). A recent investigation provided evidence that blocking CCR5 either by genetic ablation or by pharmacological inhibition with maraviroc rescued mice from colitis in both acute and chronic models (46). The latter is particularly interesting since the live microbicide strategy developed to provide vaginal in vivo delivery of CCL5-based HIV-1 entry inhibitors by engineered lactobacilli (47) could indeed be applied in the context of IBD, where lactobacilli are naturally resident commensal bacteria.
CCR5 has been implicated in the development of various types of cancer, including breast cancer, ovarian and cervical cancer, prostate cancer, colon cancer, melanoma, Hodgkin lymphoma, and multiple myeloma (48). Cancer cells secrete CCL5 or induce fibroblasts to secrete CCL5, which sustain the proliferation of CCR5-positive tumor cells (48); recruit T-regulatory cells and monocytes with suppressive functions; cause osteoclast activation; and favor bone metastasis, neo-angiogenesis, and dissemination of cancer cells to distant organs (49). CCL5 has been reported to provide antitumor adjuvanticity or, conversely, to promote carcinogenesis, depending on the tumor environment (50). These opposite effects appear to be justified by the type of cancer, CCR5 expression by cancer cells, and localization of CCL5 expression. Hence, CCR5 antagonism or activation may be circumstantially tailored to provide an antitumor effect (50–53).
Finally, a multivariate analysis of unrelated HLA-matched bone marrow transplantation for hematologic malignancies conducted in Japan showed that the recipient CCR5-2086A/A genotype was significantly associated with a lower relapse rate, resulting in better disease-free and overall survival rates than other variations (54). Therefore, the recipient CCR5-2086A/A genotype affects the induction of the graft-versus-tumor effect without augmenting the development of graft-versus-host-disease (GVHD), and CCR5 genotyping in transplant recipients may be useful in determining pretransplantation risks.
In a recently published comparison of a cohort of patients enrolled in a trial of reduced-intensity allo-hematopoietic stem cell transplantation with standard GVHD prophylaxis plus maraviroc and a contemporary control cohort receiving standard GVHD prophylaxis alone, maraviroc treatment was associated with a lower incidence of acute GVHD without increased risk of disease relapse and with reduced levels of gut-specific markers (55). Maraviroc treatment increased CCR5 expression on T cells and reduced T cell activation in peripheral blood without increasing the risk of infections. These data suggest that maraviroc protects against GVHD through modulation of allo-reactive donor T cell responses.
As discussed earlier, CCR5 knockout induced by gene therapy techniques is a strategy to reproduce the naturally occurring CCR5Δ32 deletion (56) (Figure 1). However, CCR5 abrogation by gene editing has been so far considered exclusively for the cure of HIV-1 (5). Zinc finger nucleases (57) have been used on CCR5 (58) and recently reviewed for their therapeutic potential and clinical trial implications (59). Other CCR5-targeted gene editing techniques include the CRISPR/Cas9 nuclease system and the transcription activator-like effector nuclease (60), as well as short hairpin RNAs (61) and ribozymes (62).
In 2007, maraviroc, a negative allosteric modulator of the CCR5 receptor and therefore competitive CCR5 inhibitor, was approved for clinical use as an HIV-1 entry inhibitor that showed additional efficiency in antiretroviral-pretreated patients (63). Thus, maraviroc is far the single success story emerged from the massive pharmaceutical effort spent in the development of small chemical compounds acting as chemokine antagonists (64); many hurdles were associated with the lack of receptor specificity and the toxicity derived from it. The effect of CCR5 antagonism by maraviroc in HIV-1-infected individuals has been reported to lead to transient early treatment increase in the CD4 count and a late treatment increase in the CD8 count, which may imply a recovery of the cell-mediated immunity (65). Overall, maraviroc treatment did not seem to interfere with normal homeostasis, rather to improve it (66, 67), and ameliorate inflammatory processes in HIV-1 and beyond (68).
In the effort to attain HIV-1 entry inhibition by CCR5 blockade, CCR5 must be engaged by antagonist ligands, to avoid sustained receptor activation that could generate unwanted pro-inflammatory conditions. As described above, the participation of CCR5 in a large array of chronic inflammatory diseases makes CCR5 antagonism (or, more drastically, gene-edited CCR5 knock out) an elective therapeutic option.
Two other CCR5 antagonists have been evaluated in clinical trials in HIV-infected individuals and have failed to progress. In phase II trials in treatment-naive patients of vicriviroc, a noncompetitive allosteric CCR5 antagonist (69, 70), viral rebound with continued treatment was observed (71), and in treatment-experienced patients, there was an increase in malignancies (72). Aplaviroc, a spirodiketopiperazine derivative, caused severe hepatotoxicity in infected patients in phase II clinical trials (73).
Cenicriviroc is a relatively new CCR5 antagonist presently assessed in clinical trials; it inhibits both CCR2 and CCR5 receptors and has good oral absorption (74). Cenicriviroc may offer other benefits in addition to its anti-HIV activity and is also currently in clinical trials testing its ability to reduce fibrosis in patients with non-alcoholic steatohepatitis and primary sclerosing cholangitis (75).
CCL3, CCL4, and CCL5, natural agonist ligands of CCR5, represent obvious templates for the development of protein-based CCR5 antagonists. However, CCR5 activation by these chemokines required them to be molecularly switched into antagonists. A long-lasting success story is represented by the CCL5 derivatives saga (76). Populated by several different approaches targeting the chemokine N-terminus, it ultimately led to highly potent variants that interact with CCR5 as antagonists (77) and are ~200-fold more potent than maraviroc in blocking HIV-1 in vitro.
Another protein-based approach to CCR5 antagonism is the development of monoclonal antibodies (mAbs) against CCR5 (78). PRO 140, a humanized IgG4 mAb derived from the murine mAb PA14 (79, 80), is currently in a phase III clinical trial (81). PRO 140 efficiently inhibits HIV-1 gp120 binding to CCR5 and, with lower potency, chemokines interaction with the receptor (78). Another promising anti-CCR5 mAb is CCR5mAb004, a fully human IgG4 also being tested in clinical trials (82). RoAb13 is also capable of blocking HIV-1 infection (83), and the three-dimensional structure of its Fab has been recently solved (12). Interestingly, naturally occurring anti-CCR5 antibodies have been suggested to contribute to the maintenance of homeostasis (84).
Blockade of CCR5 with antagonists is increasingly adopted to counteract inflammatory diseases and infections where this receptor plays a relevant role. Being FDA approved and a small chemical compound, maraviroc is the CCR5 antagonist of election; however, protein-based CCR5 antagonists could be equally or even more effective. The three-dimensional structure of the complex between CCR5 and maraviroc (11) helped significantly in understanding GPCR conformational modularity and visualized the deep insertion of maraviroc in the CCR5 ligand cavity. Small chemical compounds have a relatively lower production cost and might be easier to administer, compared to protein drugs. However, last generation CCL5-based antagonists (77) provided in vitro anti-HIV-1 potency far superior than that of maraviroc and grant a virtually absent development of HIV-1-resistant strains (85), which is not the case for maraviroc. In a recent breakthrough in structural biology (10), the three-dimensional structure of the complex between CCR5 and 5p7-CCL5 (a potent CCR5 antagonist) (77) has been solved, revealing the extensive and deep area of CCR5 occupancy by 5p7-CCL5. This intimate molecular interaction largely justifies the impossibility for HIV-1 to generate escape mutants since gp120 occupies a similar cavity on CCR5 [modeled in Ref. (10)]; also the virus cannot generate a gp120 molecule able to circumvent the presence of 5p7-CCL5 or similar CCL5 variants. Ultimately, the high CCR5 affinity of these CCL5 variants could be exploited in the different pathological conditions where CCR5 plays a potentially crucial role.
Biomedical investigations are elucidating a growing role played by CCR5 in several inflammatory diseases, and a number of microorganisms hijack CCR5 to exert their tropism. In this scenario, CCR5 blockade is conceived as a relatively harmless therapeutic option (Figure 1). This option is implemented either by biochemical blockade of the receptor using CCR5 antagonists or by excision of the receptor by gene editing strategies. Which of the two strategies is preferable may depend on the disease dynamics and the actual CCR5 dispensability suggested by the CCR5Δ32 allele present in individuals living a seemingly healthy life.
Both authors have made a substantial, direct, and intellectual contribution to the work and approved it for publication.
The authors declare the absence of any commercial or financial relationships that could be construed as a potential conflict of interest.
1. Berger EA, Murphy PM, Farber JM. Chemokine receptors as HIV-1 coreceptors: roles in viral entry, tropism, and disease. Annu Rev Immunol (1999) 17:657–700. doi:10.1146/annurev.immunol.17.1.657
2. Lusso P. HIV and the chemokine system: 10 years later. EMBO J (2006) 25:447–56. doi:10.1038/sj.emboj.7600947
3. Samson M, Libert F, Doranz BJ, Rucker J, Liesnard C, Farber CM, et al. Resistance to HIV-1 infection in Caucasian individuals bearing mutant alleles of the CCR-5 chemokine receptor gene. Nature (1996) 382:722–5. doi:10.1038/382722a0
4. Jin T, Xu X, Hereld D. Chemotaxis, chemokine receptors and human disease. Cytokine (2008) 44:1–8. doi:10.1016/j.cyto.2008.06.017
5. Haworth KG, Peterson CW, Kiem HP. CCR5-edited gene therapies for HIV cure: closing the door to viral entry. Cytotherapy (2017) 19(11):1325–38. doi:10.1016/j.jcyt.2017.05.013
6. Hütter G, Nowak D, Mossner M, Ganepola S, Müssig A, Allers K, et al. Long-term control of HIV by CCR5 Delta32/Delta32 stem-cell transplantation. N Engl J Med (2009) 360:692–8. doi:10.1056/NEJMoa0802905
7. Brown TR. I am the Berlin patient: a personal reflection. AIDS Res Hum Retroviruses (2015) 31:2–3. doi:10.1089/AID.2014.0224
8. Barmania F, Pepper MS. C-C chemokine receptor type five (CCR5): an emerging target for the control of HIV infection. Appl Transl Genom (2013) 2:3–16. doi:10.1016/j.atg.2013.05.004
9. Telenti A. Safety concerns about CCR5 as an antiviral target. Curr Opin HIV AIDS (2009) 4:131–5. doi:10.1097/COH.0b013e3283223d76
10. Zheng Y, Han GW, Abagyan R, Wu B, Stevens RC, Cherezov V, et al. Structure of CC chemokine receptor 5 with a potent chemokine antagonist reveals mechanisms of chemokine recognition and molecular mimicry by HIV. Immunity (2017) 46:1005.e–17.e. doi:10.1016/j.immuni.2017.05.002
11. Tan Q, Zhu Y, Li J, Chen Z, Han GW, Kufareva I, et al. Structure of the CCR5 chemokine receptor-HIV entry inhibitor maraviroc complex. Science (2013) 341:1387–90. doi:10.1126/science.1241475
12. Chain B, Arnold J, Akthar S, Brandt M, Davis D, Noursadeghi M, et al. A linear epitope in the N-terminal domain of CCR5 and its interaction with antibody. PLoS One (2015) 10:e0128381. doi:10.1371/journal.pone.0128381
13. Hedrick PW, Verrelli BC. “Ground truth” for selection on CCR5-Delta32. Trends Genet (2006) 22:293–6. doi:10.1016/j.tig.2006.04.007
14. Hummel S, Schmidt D, Kremeyer B, Herrmann B, Oppermann M. Detection of the CCR5-Delta32 HIV resistance gene in Bronze Age skeletons. Genes Immun (2005) 6:371–4. doi:10.1038/sj.gene.6364172
15. Shukaliak JA, Dorovini-Zis K. Expression of the β chemokines RANTES and MIP 1β by human brain microvessel endothelial cells in primary culture. J Neuropathol Exp Neurol (2000) 59:339–52. doi:10.1093/jnen/59.5.339
16. Subileau EA, Rezaie P, Davies HA, Colyer FM, Greenwood J, Male DK, et al. Expression of chemokines and their receptors by human brain endothelium: implications for multiple sclerosis. J Neuropathol Exp Neurol (2009) 68:227–40. doi:10.1097/NEN.0b013e318197eca7
17. Balashov KE, Rottman JB, Weiner HL, Hancock WW. CCR5+ and CXCR3+ T cells are increased in multiple sclerosis and their ligands MIP 1α and IP 10 are expressed in demyelinating brain lesions. Proc Natl Acad Sci U S A (1999) 96:6873–8. doi:10.1073/pnas.96.12.6873
18. Sørensen TL, Tani M, Jensen J, Pierce V, Lucchinetti C, Folcik VA, et al. Expression of specific chemokines and chemokine receptors in the central nervous system of multiple sclerosis patients. J Clin Invest (1999) 103:807–15. doi:10.1172/JCI5150
19. Boven LA, Montagne L, Nottet HS, De Groot CJ. Macrophage inflammatory protein 1α (MIP 1α), MIP 1β, and RANTES mRNA semiquantification and protein expression in active demyelinating multiple sclerosis (MS) lesions. Clin Exp Immunol (2000) 122:257–63. doi:10.1046/j.1365-2249.2000.01334.x
20. Silversides JA, Heggarty SV, McDonnell GV, Hawkins SA, Graham CA. Influence of CCR5 δ32 polymorphism on multiple sclerosis susceptibility and disease course. Mult Scler (2004) 10:149–52. doi:10.1191/1352458504ms994oa
21. Kantarci OH, Morales Y, Ziemer PA, Hebrink DD, Mahad DJ, Atkinson EJ, et al. CCR5Δ32 polymorphism effects on CCR5 expression, patterns of immunopathology and disease course in multiple sclerosis. J Neuroimmunol (2005) 169:137–43. doi:10.1016/j.jneuroim.2005.07.025
22. van Veen T, Nielsen J, Berkhof J, Barkhof F, Kamphorst W, Bö L, et al. CCL5 and CCR5 genotypes modify clinical, radiological and pathological features of multiple sclerosis. J Neuroimmunol (2007) 190:157–64. doi:10.1016/j.jneuroim.2007.08.005
23. Lim JK, Glass WG, McDermott DH, Murphy PM. CCR5: no longer a “good for nothing” gene – chemokine control of West Nile virus infection. Trends Immunol (2006) 27:308–12. doi:10.1016/j.it.2006.05.007
24. Lim JK, Louie CY, Glaser C, Jean C, Johnson B, Johnson H, et al. Genetic deficiency of chemokine receptor CCR5 is a strong risk factor for symptomatic West Nile virus infection: a meta-analysis of 4 cohorts in the US epidemic. J Infect Dis (2008) 197:262–5. doi:10.1086/524691
25. Kindberg E, Mickiene A, Ax C, Akerlind B, Vene S, Lindquist L, et al. A deletion in the chemokine receptor 5 (CCR5) gene is associated with tickborne encephalitis. J Infect Dis (2008) 197:266–9. doi:10.1086/524709
26. Sarfo BY, Singh S, Lillard JW Jr, Quarshie A, Gyasi RK, Armah H, et al. The cerebral-malaria-associated expression of RANTES, CCR3 and CCR5 in postmortem tissue samples. Ann Trop Med Parasitol (2004) 98:297–303. doi:10.1179/000349804225003271
27. Rustemoglu A, Ekinci D, Nursal AF, Barut S, Duygu F, Günal Ö. The possible role of CCR5Δ32 mutation in Crimean-Congo hemorrhagic fever infection. J Med Virol (2017) 89(10):1714–9. doi:10.1002/jmv.24865
28. Arasli M, Ozsurekci Y, Elaldi N, McAuley AJ, Karadag Oncel E, Tekin IO, et al. Elevated chemokine levels during adult but not pediatric Crimean-Congo hemorrhagic fever. J Clin Virol (2015) 66:76–82. doi:10.1016/j.jcv.2015.03.010
29. Marques RE, Guabiraba R, Del Sarto JL, Rocha RF, Queiroz AL, Cisalpino D, et al. Dengue virus requires the CC-chemokine receptor CCR5 for replication and infection development. Immunology (2015) 145:583–96. doi:10.1111/imm.12476
30. Aliberti J, Valenzuela JG, Carruthers VB, Hieny S, Andersen J, Charest H, et al. Molecular mimicry of a CCR5 binding-domain in the microbial activation of dendritic cells. Nat Immunol (2003) 4:485–90. doi:10.1038/ni915
31. Bonfá G, Benevides L, Souza Mdo C, Fonseca DM, Mineo TW, Rossi MA, et al. CCR5 controls immune and metabolic functions during Toxoplasma gondii infection. PLoS One (2014) 9:e104736. doi:10.1371/journal.pone.0104736
32. Lalani AS, Masters J, Zeng W, Barrett J, Pannu R, Everett H, et al. Use of chemokine receptors by poxviruses. Science (1999) 286:1968–71. doi:10.1126/science.286.5446.1968
33. Rahbar R, Murooka TT, Fish EN. Role for CCR5 in dissemination of vaccinia virus in vivo. J Virol (2009) 83:2226–36. doi:10.1128/JVI.01655-08
34. Vandenesch F, Naimi T, Enright MC, Lina G, Nimmo GR, Heffernan H, et al. Community-acquired methicillin-resistant Staphylococcus aureus carrying Panton-Valentine leukocidin genes: worldwide emergence. Emerg Infect Dis (2003) 9:978–84. doi:10.3201/eid0908.030089
35. Alonzo F III, Kozhaya L, Rawlings SA, Reyes-Robles T, DuMont AL, Myszka DG, et al. CCR5 is a receptor for Staphylococcus aureus leukotoxin ED. Nature (2013) 493:51–5. doi:10.1038/nature11724
36. Tam K, Schultz M, Reyes-Robles T, Vanwalscappel B, Horton J, Alonzo F III, et al. Staphylococcus aureus leukocidin LukED and HIV-1 gp120 target different sequence determinants on CCR5. MBio (2016) 7:e2024–2016. doi:10.1128/mBio.02024-16
37. Mack M, Bruhl H, Gruber R, Jaeger C, Cihak J, Eiter V, et al. Predominance of mononuclear cells expressing the chemokine receptor CCR5 in synovial effusions of patients with different forms of arthritis. Arthritis Rheum (1999) 42:981–8. doi:10.1002/1529-0131(199905)42:5<981::AID-ANR17>3.0.CO;2-4
38. Patel DD, Zachariah JP, Whichard LP. CXCR3 and CCR5 ligands in rheumatoid arthritis synovium. Clin Immun (2001) 98:39–45. doi:10.1006/clim.2000.4957
39. Pokorny V, McQueen F, Yeoman S, Merriman M, Merriman A, Harrison A, et al. Evidence for negative association of the chemokine receptor CCR5 d32 polymorphism with rheumatoid arthritis. Ann Rheum Dis (2005) 64:487–90. doi:10.1136/ard.2004.023333
40. Fleishaker DL, Garcia Meijide JA, Petrov A, Kohen MD, Wang X, Menon S, et al. Maraviroc, a chemokine receptor 5 antagonist, fails to demonstrate efficacy in the treatment of patients with rheumatoid arthritis in a randomized, double-blind placebo-controlled trial. Arthritis Res Ther (2012) 14:R11. doi:10.1186/ar3685
41. Jones KL, Maguire JJ, Davenport AP. Chemokine receptor CCR5: from AIDS to atherosclerosis. Br J Pharmacol (2011) 162:1453–69. doi:10.1111/j.1476-5381.2010.01147.x
42. Zhang Z, Liu J, Wang H, Wu H, Wu X, Dong J, et al. Association between chemokine receptor 5 (CCR5) delta32 gene variant and atherosclerosis: a meta-analysis of 13 studies. Int J Clin Exp Med (2015) 8:658–65.
43. Li J, McArdle S, Gholami A, Kimura T, Wolf D, Gerhardt T, et al. CCR5+T-bet+FoxP3+ effector CD4 T cells drive atherosclerosis. Circ Res (2016) 118:1540–52. doi:10.1161/CIRCRESAHA.116.308648
44. Trudu M, Schaeffer C, Riba M, Ikehata M, Brambilla P, Messa P, et al. Early involvement of cellular stress and inflammatory signals in the pathogenesis of tubulointerstitial kidney disease due to UMOD mutations. Sci Rep (2017) 7:7383. doi:10.1038/s41598-017-07804-6
45. McCormack G, Moriarty D, O’Donoghue DP, McCormick PA, Sheahan K, Baird AW. Tissue cytokine and chemokine expression in inflammatory bowel disease. Inflamm Res (2001) 50:491–5. doi:10.1007/PL00000223
46. Mencarelli A, Cipriani S, Francisci D, Santucci L, Baldelli F, Distrutti E, et al. Highly specific blockade of CCR5 inhibits leukocyte trafficking and reduces mucosal inflammation in murine colitis. Sci Rep (2016) 6:30802. doi:10.1038/srep30802
47. Vangelista L, Secchi M, Liu X, Bachi A, Jia L, Xu Q, et al. Engineering of Lactobacillus jensenii to secrete RANTES and a CCR5 antagonist analogue as live HIV-1 blockers. Antimicrob Agents Chemother (2010) 54:2994–3001. doi:10.1128/AAC.01492-09
48. Aldinucci D, Colombatti A. The inflammatory chemokine CCL5 and cancer progression. Mediators Inflamm (2014) 2014:292376. doi:10.1155/2014/292376
49. de Oliveira CE, Oda JM, Losi Guembarovski R, de Oliveira KB, Ariza CB, Neto JS, et al. CC chemokine receptor 5: the interface of host immunity and cancer. Dis Markers (2014) 2014:126954. doi:10.1155/2014/126954
50. Lapteva N, Huang XF. CCL5 as an adjuvant for cancer immunotherapy. Expert Opin Biol Ther (2010) 10:725–33. doi:10.1517/14712591003657128
51. Velasco-Velázquez M, Xolalpa W, Pestell RG. The potential to target CCL5/CCR5 in breast cancer. Expert Opin Ther Targets (2014) 18:1265–75. doi:10.1517/14728222.2014.949238
52. Bronte V, Bria E. Interfering with CCL5/CCR5 at the tumor-stroma interface. Cancer Cell (2016) 29:437–9. doi:10.1016/j.ccell.2016.03.019
53. González-Martin A, Mira E, Mañes S. CCR5 as a potential target in cancer therapy: inhibition or stimulation? Anticancer Agents Med Chem (2012) 12:1045–57. doi:10.2174/187152012803529637
54. Horio T, Mizuno S, Uchino K, Mizutani M, Hanamura I, Espinoza JL, et al. The recipient CCR5 variation predicts survival outcomes after bone marrow transplantation. Transpl Immunol (2017) 42:34–9. doi:10.1016/j.trim.2017.05.003
55. Moy RH, Huffman AP, Richman LP, Crisalli L, Wang XK, Hoxie JA, et al. Clinical and immunologic impact of CCR5 blockade in graft-versus-host disease prophylaxis. Blood (2017) 129:906–16. doi:10.1182/blood-2016-08-735076
56. Hütter G, Bodor J, Ledger S, Boyd M, Millington M, Tsie M, et al. CCR5 targeted cell therapy for HIV and prevention of viral escape. Viruses (2015) 7:4186–203. doi:10.3390/v7082816
57. Jamieson AC, Miller JC, Pabo CO. Drug discovery with engineered zinc-finger proteins. Nat Rev Drug Discov (2003) 2:361–8. doi:10.1038/nrd1087
58. Perez EE, Wang J, Miller JC, Jouvenot Y, Kim KA, Liu O, et al. Establishment of HIV-1 resistance in CD4+ T cells by genome editing using zinc-finger nucleases. Nat Biotechnol (2008) 26:808–16. doi:10.1038/nbt1410
59. Ando D, Meyer K. Gene editing: regulatory and translation to clinic. Hematol Oncol Clin North Am (2017) 31:797–808. doi:10.1016/j.hoc.2017.06.002
60. Cornu TI, Mussolino C, Cathomen T. Refining strategies to translate genome editing to the clinic. Nat Med (2017) 23:415–23. doi:10.1038/nm.4313
61. Swamy MN, Wu H, Shankar P. Recent advances in RNAi-based strategies for therapy and prevention of HIV-1/AIDS. Adv Drug Deliv Rev (2016) 103:174–86. doi:10.1016/j.addr.2016.03.005
62. Scarborough RJ, Gatignol A. HIV and ribozymes. Adv Exp Med Biol (2015) 848:97–116. doi:10.1007/978-1-4939-2432-5_5
63. Fatkenheuer G, Nelson M, Lazzarin A, Konourina I, Hoepelman AI, Lampiris H, et al. Subgroup analyses of maraviroc in previously treated R5 HIV-1 infection. N Engl J Med (2008) 359:1442–55. doi:10.1056/NEJMoa0803154
64. Horuk R. Chemokine receptor antagonists: overcoming developmental hurdles. Nat Rev Drug Discov (2009) 8:23–33. doi:10.1038/nrd2734
65. Rusconi S, Vitiello P, Adorni F, Colella E, Focà E, Capetti A, et al. Maraviroc as intensification strategy in HIV-1 positive patients with deficient immunological response: an Italian randomized clinical trial. PLoS One (2013) 8:e80157. doi:10.1371/journal.pone.0080157
66. Arberas H, Guardo AC, Bargalló ME, Maleno MJ, Calvo M, Blanco JL, et al. In vitro effects of the CCR5 inhibitor maraviroc on human T cell function. J Antimicrob Chemother (2013) 68:577–86. doi:10.1093/jac/dks432
67. Pozo-Balado MM, Rosado-Sánchez I, Méndez-Lagares G, Rodríguez-Méndez MM, Ruiz-Mateos E, Benhnia MR, et al. Maraviroc contributes to the restoration of the homeostasis of regulatory T-cell subsets in antiretroviral-naive HIV-infected subjects. Clin Microbiol Infect (2016) 22:.e1–5. doi:10.1016/j.cmi.2015.12.025
68. Woollard SM, Kanmogne GD. Maraviroc: a review of its use in HIV infection and beyond. Drug Des Devel Ther (2015) 9:5447–68. doi:10.2147/DDDT.S90580
69. McCombie SW, Tagat JR, Vice SF, Lin SI, Steensma R, Palani A, et al. Piperazine-based CCR5 antagonists as HIV-1 inhibitors. III: synthesis, antiviral and pharmacokinetic profiles of symmetrical heteroaryl carboxamides. Bioorg Med Chem Lett (2003) 13:567–71. doi:10.1016/S0960-894X(02)00918-6
70. Tagat JR, McCombie SW, Nazareno D, Labroli MA, Xiao Y, Steensma RW, et al. Piperazine-based CCR5 antagonists as HIV-1 inhibitors. IV. Discovery of 1-[(4,6-dimethyl-5-pyrimidinyl)carbonyl]-4-[4-[2-methoxy-1(R)-4-(trifluoromethyl)phenyl] ethyl-3(S)-methyl-1-piperazinyl]-4-methylpiperidine (Sch-417690/Sch-D), a potent, highly selective, and orally bioavailable CCR5 antagonist. J Med Chem (2004) 47:2405–8. doi:10.1021/jm0304515
71. Alcom K. Second company halts CCR5 inhibitor study in another blow to new drug class. NAM AidsMap. (2005). Available from: www.aidsmap.com/Second-company-halts-CCR5-inhibitorstudy-in-another-blow-to-new-drug-class/page/1422234/
72. Gulick RM, Su Z, Flexner C, Hughes MD, Skolnik PR, Wilkin TJ, et al. Phase 2 study of the safety and efficacy of vicriviroc, a CCR5 inhibitor, in HIV-1-infected, treatment-experienced patients: AIDS clinical trials group 5211. J Infect Dis (2007) 196:304–12. doi:10.1086/518797
73. Nichols WG, Steel HM, Bonny T, Adkison K, Curtis L, Millard J, et al. Hepatotoxicity observed in clinical trials of aplaviroc (GW873140). Antimicrob Agents Chemother (2008) 52:858–65. doi:10.1128/AAC.00821-07
74. Seto M, Aikawa K, Miyamoto N, Aramaki Y, Kanzaki N, Takashima K, et al. Highly potent and orally active CCR5 antagonists as anti-HIV-1 agents: synthesis and biological activities of 1-benzazocine derivatives containing a sulfoxide moiety. J Med Chem (2006) 49:2037–48. doi:10.1021/jm0509703
75. Friedman S, Sanyal A, Goodman Z, Lefebvre E, Gottwald M, Fischer L, et al. Efficacy and safety study of cenicriviroc for the treatment of non-alcoholic steatohepatitis in adult subjects with liver fibrosis: CENTAUR phase 2b study design. Contemp Clin Trials (2016) 47:356–65. doi:10.1016/j.cct.2016.02.012
76. Vangelista L, Secchi M, Lusso P. Rational design of novel HIV-1 entry inhibitors by RANTES engineering. Vaccine (2008) 26:3008–15. doi:10.1016/j.vaccine.2007.12.023
77. Gaertner H, Cerini F, Escola JM, Kuenzi G, Melotti A, Offord R, et al. Highly potent, fully recombinant anti-HIV chemokines: reengineering a low-cost microbicide. Proc Natl Acad Sci U S A (2008) 105:17706–11. doi:10.1073/pnas.0805098105
78. Hutchings CJ, Koglin M, Olson WC, Marshall FH. Opportunities for therapeutic antibodies directed at G-protein-coupled receptors. Nat Rev Drug Discov (2017) 16(9):661. doi:10.1038/nrd.2017.91
79. Olson WC, Rabut GE, Nagashima KA, Tran DN, Anselma DJ, Monard SP, et al. Differential inhibition of human immunodeficiency virus type 1 fusion, gp120 binding, and CC-chemokine activity by monoclonal antibodies to CCR5. J Virol (1999) 73:4145–55.
80. Trkola A, Ketas TJ, Nagashima KA, Zhao L, Cilliers T, Morris L, et al. Potent, broad-spectrum inhibition of human immunodeficiency virus type 1 by the CCR5 monoclonal antibody PRO 140. J Virol (2001) 75:579–88. doi:10.1128/JVI.75.2.579-588.2001
81. Reichert JM. Antibodies to watch in 2017. MAbs (2017) 9:167–81. doi:10.1080/19420862.2016.1269580
82. Flego M, Ascione A, Cianfriglia M, Vella S. Clinical development of monoclonal antibody-based drugs in HIV and HCV diseases. BMC Med (2013) 11:4. doi:10.1186/1741-7015-11-4
83. Ji C, Brandt M, Dioszegi M, Jekle A, Schwoerer S, Challand S, et al. Novel CCR5 monoclonal antibodies with potent and broad-spectrum anti-HIV activities. Antiviral Res (2007) 74:125–37. doi:10.1016/j.antiviral.2006.11.003
84. Venuti A, Pastori C, Lopalco L. The role of natural antibodies to CC chemokine receptor 5 in HIV infection. Front Immunol (2017) 8:1358. doi:10.3389/fimmu.2017.01358
Keywords: CCR5, CCL5, inflammation, HIV-1, cancer
Citation: Vangelista L and Vento S (2018) The Expanding Therapeutic Perspective of CCR5 Blockade. Front. Immunol. 8:1981. doi: 10.3389/fimmu.2017.01981
Received: 09 October 2017; Accepted: 20 December 2017;
Published: 12 January 2018
Edited by:
Jixin Zhong, Case Western Reserve University, United StatesReviewed by:
Ankit Saxena, National Institutes of Health (NIH), United StatesCopyright: © 2018 Vangelista and Vento. This is an open-access article distributed under the terms of the Creative Commons Attribution License (CC BY). The use, distribution or reproduction in other forums is permitted, provided the original author(s) or licensor are credited and that the original publication in this journal is cited, in accordance with accepted academic practice. No use, distribution or reproduction is permitted which does not comply with these terms.
*Correspondence: Luca Vangelista, bHVjYS52YW5nZWxpc3RhQG51LmVkdS5reg==
Disclaimer: All claims expressed in this article are solely those of the authors and do not necessarily represent those of their affiliated organizations, or those of the publisher, the editors and the reviewers. Any product that may be evaluated in this article or claim that may be made by its manufacturer is not guaranteed or endorsed by the publisher.
Research integrity at Frontiers
Learn more about the work of our research integrity team to safeguard the quality of each article we publish.