- 1Clinical Research, Miltenyi Biotec Inc., Gaithersburg, MD, USA
- 2Division for Stem Cell Transplantation and Immunology, Department for Children and Adolescents Medicine, Hospital of the Goethe University, Frankfurt, Germany
- 3LOEWE Center for Cell and Gene Therapy, Cellular Immunology, Goethe University, Frankfurt, Germany
- 4Institute of Cellular Therapeutics, Integrated Research and Treatment Center Transplantation, Hannover Medical School, Hannover, Germany
- 5Innate Immunity Group, German Cancer Research Center, Heidelberg, Germany
- 6Division of Immunbiochemistry, Medical Faculty Mannheim, Heidelberg University, Heidelberg, Germany
- 7R&D Reagents, Miltenyi Biotec GmbH, Bergisch Gladbach, Germany
Natural killer (NK) cells are a promising tool for the use in adoptive immunotherapy, since they efficiently recognize and kill tumor cells. In this context, ex vivo cultivation is an attractive option to increase NK cells in numbers and to improve their antitumor potential prior to clinical applications. Consequently, various strategies to generate NK cells for adoptive immunotherapy have been developed. Here, we give an overview of different NK cell cultivation approaches and their impact on shaping the NK cell antitumor activity. So far, the cytokines interleukin (IL)-2, IL-12, IL-15, IL-18, and IL-21 are used to culture and expand NK cells. The selection of the respective cytokine combination is an important factor that directly affects NK cell maturation, proliferation, survival, distribution of NK cell subpopulations, activation, and function in terms of cytokine production and cytotoxic potential. Importantly, cytokines can upregulate the expression of certain activating receptors on NK cells, thereby increasing their responsiveness against tumor cells that express the corresponding ligands. Apart from using cytokines, cocultivation with autologous accessory non-NK cells or addition of growth-inactivated feeder cells are approaches for NK cell cultivation with pronounced effects on NK cell activation and expansion. Furthermore, ex vivo cultivation was reported to prime NK cells for the killing of tumor cells that were previously resistant to NK cell attack. In general, NK cells become frequently dysfunctional in cancer patients, for instance, by downregulation of NK cell activating receptors, disabling them in their antitumor response. In such scenario, ex vivo cultivation can be helpful to arm NK cells with enhanced antitumor properties to overcome immunosuppression. In this review, we summarize the current knowledge on NK cell modulation by different ex vivo cultivation strategies focused on increasing NK cytotoxicity for clinical application in malignant diseases. Moreover, we critically discuss the technical and regulatory aspects and challenges underlying NK cell based therapeutic approaches in the clinics.
Introduction
As an important part of the innate immune system, natural killer (NK) cells are deployed as first line of defense against aberrant cells caused by viral infections or malignancies. Human NK cells can be identified via their morphology as large granular lymphocytes, and via their surface marker profile, as they express by definition CD56, but not CD3. The NK cell compartment can be further divided into subpopulations. There are two main NK cell subsets that can be distinguished, the CD56highCD16neg subpopulation, which has mostly immune modulatory function, mainly accomplished by interferon (IFN)-γ secretion, and the CD56lowCD16pos fraction with direct cytotoxic capacity (1–3). NK cell activation is based on a balanced system integrating signals from activating and inhibitory receptors. Inhibitory signals derive mainly from germ-line encoded inhibitory killer cell immunoglobulin-like receptors (KIRs). Ligands for inhibitory KIRs, in humans major histocompatibility complex (MHC) class I molecules, are highly expressed by healthy cells and thereby prevent NK cell activation. Malignant cells often downregulate MHC class I molecules on their surface to evade T cell attack (4). However, these so-called “missing-self” cells are recognized by NK cells through inhibitory receptors, and as signals from activating receptors prevail, NK cells become active and react against the encountered targets. Alternatively, NK cells can be activated by overexpression of stress-induced surface ligands on infected or abnormal cells, which are recognized by activating receptors, such as the natural cytotoxicity receptors (NCRs) NKp30, NKp44, and NKp46, and the so-called C-type lectin-like receptors, such as NKG2D (1, 5–9). In this case, activating signals outbalance inhibitory self-signals and lead to NK cell activation. Furthermore, NK cells become activated upon encounter of antibody-coated targets by CD16, which binds to the Fc portion of the antibody and mediates a strong activating signal. By means of activating and inhibitory receptors, NK cells, unlike T and B-lymphocytes, can react immediately without prior priming or antigen presentation.
Activated NK cells execute effector functions through different mechanisms. NK cells mediate direct cytotoxicity via the exocytosis pathway with release of cytotoxic granules, which contain granzymes and perforin, resulting in lysis of the target cell (10). In addition, NK cells induce apoptosis of target cells by expression of death receptor ligands, such as Fas ligand or tumor necrosis factor-related apoptosis-inducing ligand (TRAIL) (11). Production and release of IFN-γ by NK cells after activation also has multiple functional consequences, with particular relevance in tumor surveillance, as IFN-γ inhibits tumor angiogenesis, has antimetastatic activity, and acts pro-apoptotic (12, 13).
The ability of tumor cells to bypass the immune response is a basic prerequisite for cancer formation and progression. Within immune editing, tumors undergo genetic, epigenetic, and phenotypic changes, thereby becoming a heterogeneous cell population that is hardly visible to or assailable by immune cells due to downregulation of tumor antigens and NCR ligands (14). Additionally, malignant cells suppress NK cells by blocking the NKG2D receptor via shedding of NKG2D ligands (15–17) or upregulation of inhibitory MHC class I molecules (18, 19). Immunosuppressive cytokines such as transforming growth factor-β, interleukin (IL)-10, or immunosuppressive enzymes, such as indoleamin 2,3-dioxigenase, further impair antitumor NK cell responses of cancer patients (20–22).
Ex vivo modulation of NK cell receptor expression is therefore an important tool to overcome immune response inhibition. A number of studies reported an upregulation of DNAM-1, NKG2D, and other NK cell-activating receptors under certain culture conditions, mostly involving stimulation by IL-2 (23–26). In addition, other ILs such as IL-12, IL15, IL-18, or IL-21 and Type I IFNs shape the NK cell receptor expression profile (27–31).
Natural killer cells can play an important role for cellular immunotherapy and the adoptive transfer of NK cells represents an attractive strategy to treat cancer patients (32, 33). In this context, ex vivo expansion of NK cells prior to their clinical application is not only required to increase the applicable cell doses but it is also reasonable to pre-activate and modify their antitumor features. For ex vivo cultivation, NK cells from different sources can be stimulated with different cytokines, and, to reach efficient expansion rates, NK cells are cultured among autologous accessory cells or together with different types of growth-inactivated autologous or allogeneic feeder cells (Figure 1). Of note, it is possible to genetically engineer NK cells ex vivo to further augment their antitumor activity, for example, to integrate chimeric antigen receptors against distinct tumor antigens (34, 35). In this review, we focus on the cultivation of NK cells without genetic modifications. Many different protocols exist for ex vivo expansion of NK cells, all with different features and capacities. Here, we give a comprehensive overview of strategies to obtain appropriate amounts of functional NK cells. We will discuss starting material and culture systems as well as the use of cytokines, feeder cells, and other additives.
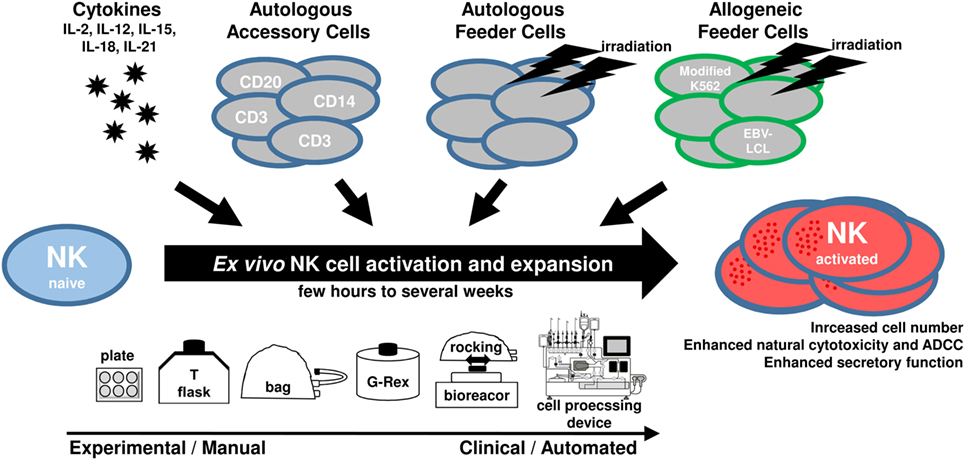
Figure 1. Scheme showing main components utilized for ex vivo natural killer (NK) cell activation and expansion procedures.
Starting Material for NK Cell Expansion and Role of NK Cell Purity
Until recent, 92% of clinical studies used NK cells from peripheral blood, either donor (79% of recruiting trials) or patient derived (13% of recruiting trials) (36). Alternatives are the use of NK cell lines, or the differentiation of NK cells from umbilical cord blood or pluripotent stem cells (37–39). NK cell lines, such as NK-92, avoid the need for donor selection and enable the production of large cell doses to treat patients on a flexible schedule (40). Nevertheless, NK cell lines require growth inactivation mainly achieved by irradiation, possibly reducing their antitumor potential due to short in vivo persistence. Differentiation of NK cells from cord blood CD34+ cells is attractive because of the “off-the-shelf” availability from a cord blood bank. Similarly, NK cells from pluripotent stem cells are a promising concept for the future but still in early development (39, 41). In this overview, we focus on peripheral blood-derived NK cells, currently the main source for NK cells for clinical use.
The NK cell purity, meaning the frequency of NK cells among other cells, is an important factor for the intended therapeutic application. For ex vivo expansion, NK cells are often cultured within a mixture of cells, such as PBMC, thereby avoiding further purification. Whereas the cultivation of NK cells among other accessory cells is a practical strategy for autologous therapeutic settings, it may be critical for allogeneic applications, since non-NK cells may induce unwanted side effects. Alloreactive T cells are a major risk factor for the patient, as they mediate “graft-versus-host disease” (GvHD), a severe complication following allogeneic hematopoietic stem cell transplantation (HSCT) (42). Furthermore, donor-derived B cells can lead to B cell lymphoproliferative disorder after reactivation of an Epstein–Barr virus (EBV) infection (43, 44), and they can cause the passenger lymphocyte syndrome (45), both critical side effects for the patient. Therefore, purification of NK cells might be required and is realized so far in most clinical settings by magnetic cell separation, for instance, by depletion of CD3-expressing cells and subsequent enrichment for CD56-expressing cells (26, 46–49). In addition, a first proof of concept is shown for good manufacturing practice (GMP)-compliant fluorescence-activated cell sorting to purify for NK cell subsets, such as NK cells expressing a single KIR (50).
Cytokine-Induced NK Cell Expansion
Aims of adoptive transfer of ex vivo expanded NK cells are the enhancement of natural cytotoxicity and homing to tumor sites under maintenance of “self” protection. Studies performed with cytokine-stimulated NK cells or PBMC have shown the safety of this approach and indicated some clinical responses upon adoptive NK cell transfer following HSCT. In the next paragraph, we summarize ex vivo NK cell expansion protocols starting with purified NK cells (Table 1) or PBMC (Table 2). Concepts administering cytokines in the presence of growth-inactivated feeder cells will be discussed in later sections of this article.
The Role of IL-2
Interleukin-2 plays an important role in activation of NK cells via binding to the IL-2 receptor (IL-2R), a heterotrimeric protein expressed on NK cells and other immune cells. This led to the interest in both (i) using IL-2 for stimulation of autologous NK cells in cancer patients and (ii) ex vivo activation and expansion of allogeneic donor NK cells for adaptive immunotherapy. At the beginning of the 1980s, researchers around Rosenberg and colleagues showed that IL-2 exposed lymphokine-activated killer (LAK) cells were able to attack autologous fresh tumor cells and that this effect could mainly be ascribed to NK cells (71, 72). Nevertheless, in first clinical trials using adoptive transfer of LAK cells and IL-2 therapy, the clinical response did not exceed the efficacy of IL-2 monotherapy (73).
Importantly, during the last 20 years, it has been elaborated that NK cells play a major role in the regulation of the balance between GvL and GvHD after allogeneic HSCT, especially haploidentical HSCT (33, 74–78), demonstrating improved anticancer activity while avoiding GvHD. In order to make haploidentical NK cells available for clinical use, large-scale GMP-conform manufacturing protocols were established. After starting with a leukapheresis product that was depleted for CD3+ cells and enriched for CD56+ cells, cultivation in medium containing IL-2 (1,000 U/mL) for up to 2 weeks yielded 0.1–3 × 109 CD56+CD3− NK cells (Table 1), sometimes sufficient for multiple infusions to patients with hematological malignancies (26). Median NK cell expansion was fivefold and median NK cell purity was >94 with <0.1% T cell contamination (26). Ex vivo stimulation with IL-2 induced elevated cytokine secretion by NK cells, enhanced intracellular STAT3/AKT signaling, and upregulation of various NCRs and NKG2D receptors (52). Depletion of CD3 cells from leukapheresis products without subsequent CD56 enrichment and short-term activation with IL-2 overnight led to a final product containing 40% NK cells (Table 2). In all cases, IL-2-activated NK cells demonstrated a much higher cytotoxic activity against K562 target cells compared to unstimulated NK cells (26, 43, 52, 53, 60). In addition, after cryopreservation and thawing, NK cells showed a moderate to high viability when activated with IL-2, whereas the viability of unstimulated NK cells was low (26).
Transfer into the clinic in 2004 and 2005 with first patient studies using those IL-2-activated donor NK cells were performed in parallel in Europe and the US, for both, haploidentical HSCT (53), and in the non-transplant setting (43). In the latter one, Miller and coworkers used IL-2 expanded haploidentical NK to treat 43 patients with advanced cancer (43), with 19 of them suffering from acute myeloid leukemia, followed by studies in patient with ovarian and breast cancer and B-cell non-Hodgkin lymphoma (60, 79). Importantly, the authors reported in vivo persistence and even expansion of the alloreactive donor NK cells in patients pretreated with high dose preparative regimen, consisting of 5 days of 60 mg/kg intravenous cyclophosphamide and 25 mg/m2 intravenous fludarabine (43). Of note, successful NK cell engraftment was dependent on the patients’ pretreatment regimen, which was also responsible for the patients’ elevated IL-15 plasma concentrations (43). In addition, it was demonstrated that in vivo persistence of donor NK cells at day 7 after infusion and successful in vivo expansion (more than 100 donor-derived NK cells per microliter of patient blood 14 days after transfer) correlated with leukemia clearance (60). Expansion of host regulatory T cells was associated with low numbers of NK cells (60). In parallel, Koehl et al. reported on three pediatric patients with multiply relapsed leukemia (still in blast persistence at HSCT) treated with repeated transfusions of IL-2-activated donor NK cells post-haploidentical HSCT (53), which led to complete remission remaining for several weeks up to some months. In the following clinical study, they also demonstrated a small clinical benefit in patients with various malignancies receiving IL-2-activated compared to patients receiving resting NK cells only (80). Interestingly, IL-2-stimulated NK cells but not unstimulated NK cells promoted NK cell trafficking and changes in the distribution of leukocyte subpopulations in the peripheral blood. In the meanwhile, safety and feasibility using IL-2-activated and -expanded NK cells for adaptive immunotherapy has been demonstrated in various clinical studies as summarized in a recent review by Koehl and others (33).
Impact of IL-15 on NK Cell Expansion
Carson et al. postulated that NK cells might be dependent on other cytokines than IL-2 such as IL-15 (81, 82). The trimeric IL-15 receptor on NK cells shares two subunits with the IL-2R, but not CD25 forming the high affinity IL-2R. Therefore, they also share some functions, e.g., maintenance of NK cell survival (82). Similarities and differences between IL-2 and IL-15 effects on NK cells have been extensively reviewed elsewhere, and IL-15 might be the preferable cytokine for cancer therapy as it inhibits activation-induced cell death and it is considered safe (83–85). In addition, compared to IL-2, IL-15 leads to more sustained antitumor capacity of NK cells via signaling through mammalian target of rapamycin and stress-activated gene expression (86). However, recent data revealed that continuous IL-15 signaling causes functional exhaustion of NK cells by decreased fatty acid oxidation, resulting in lower cytotoxicity in vitro and decreased tumor control in vivo (87). Thus, optimal dosing and timing of IL-15 is critical for ex vivo NK cell activation. Purified NK cells expanded using IL-15 exhibit upregulation of NCRs and CD69 and cytolysis of leukemia and primary ALL blasts (51). Enhanced cytotoxicity of IL-15-stimulated NK cells against leukemia and rhabdomyosarcoma cell lines could be attributed to NCRs, DNAM-1 and NKG2D (54). Using IL-15 to expand NK cells from CD56-enriched PBMC for 20 days resulted in a 23-fold expansion of CD3−CD56+ NK cells with a final purity of about 98% (63). NK cells generated with the latter protocol were transferred to 15 non-small lung cancer patients in a phase I clinical trial in two to four doses of 0.2–29 × 106 NK cells/kg, showing the safety of the approach (63).
IL-21 Enhances NK Cell Effector Functions
The cytokine IL-21, in combination with IL-2 or IL-15, is utilized in some protocols for NK cell stimulation (55, 64). IL-21 belongs to the IL-2 family and signals through a heterodimer consisting of the common γ-chain and the IL-21 receptor α-chain. Activated CD4+ T cells are the main producers of IL-21 and IL-21 affects many different cell types expressing the IL-21 receptor, including NK cells (88). IL-21 plays a role in the development of NK cells from bone marrow progenitors (89), and, in mice, it dampens the expansion of NK cells but is required for functional NK cell maturation (90, 91). Recently, expansion of “memory-like” NK cells has been shown to be IL-21 dependent in the context of tuberculosis infection (92). Wendt et al. observed increased proliferation of CD56bright human NK cells (55), but another group reported no effect of IL-21 on the proliferation of NK cells from healthy human donors and from HIV patients (93). Moreover, IL-21 is known to trigger apoptosis, resulting in a shorter lifespan of NK cells in vitro (90, 94). Thus, the time span NK cells are exposed to IL-21 appears critical (95, 96). Besides its effect on NK cell proliferation, IL-21 enhances the effector functions of NK cells, including secretory and cytotoxic functions as well as enhanced ADCC responses (93, 97, 98). Culturing CD3-depleted PBMC for 13–20 days with IL-21 and IL-15 without additional feeder cells yields activated NK cells with a purity of >90%, which were applied in a clinical trial with 41 leukemia patients receiving infusions of donor-derived NK cells 2–3 weeks after HSCT (64). Although the NK cells expanded weakly under this condition (3.7-fold), they possessed potent cytotoxic activity against primary bone marrow blasts prior to transplantation, and infusions with a median dose of 2 × 108 NK cells/kg were well tolerated and correlated with a reduction in leukemia progression compared to historical controls (64).
IL-12/15/18 Induced Memory NK Cells
Interleukin-12 was originally discovered as NK cell-stimulating factor, inducing proliferation, enhanced cytotoxicity, and production of IFN-γ by NK cells when added to PBMC (99, 100). IL-12 is produced by DCs, macrophages, and B cells, and its receptor consists of two subunits (α and β), which mediate signaling through members of the JAK–STAT family (101). IL-2 enhances the response of NK cells to IL-12 by increasing the expression of the IL-12 receptor and STAT4, a relevant factor for IL-12 signaling (102). Furthermore, it was revealed that IL-12-mediated IFN-γ production of NK cells requires priming with IL-18, a cytokine also known to enhance IL-15-induced NK cell proliferation (103, 104). Due to the synergistic effects, it seems reasonable to combine the different cytokines for ex vivo stimulation of NK cells. In this context, the combination of IL-12, IL-15, and IL-18 raised special interest, as it leads to the so-called “cytokine-induced memory-like” (CIML) NK cells in mice and humans, which exhibit an increased capacity to produce IFN-γ upon re-stimulation at later time points (56, 105). Importantly, this memory response is a cell intrinsic effect that is passed on to offspring cells and is maintained up to several months (56). In mice, the intrinsic ability for mediated IFN-γ production coincided with demethylation of the conserved non-coding sequence 1 in the IFN-γ locus (106). Furthermore, adoptive transfer of CIML NK cells had a clear antitumor activity against established melanoma or lymphoma in vivo, which required IL-2 from CD4+ T cells (57, 106). For both, murine and human NK cells, IL-12, IL-15, and IL-18 together induce an increased expression of CD25, making CIML NK cells responsive to low concentrations of IL-2 in vitro and in vivo (57, 58). Thus, there is a clear rationale to apply adoptive transfer of ex vivo-generated CIML NK cells together with IL-2 injections as a combination therapy. Recently, CIML NK cells together with low dose IL-2 therapy were evaluated in a first-in-human phase I clinical trial with promising results, as clinical response was observed in five of nine treated patients (107).
Autologous Accessory Cells and Autologous Feeder Cells for NK Cell Expansion
Although cytokines efficiently activate NK cells and result in cell products with advanced effector functions, cytokines alone do not allow pronounced ex vivo expansion (Table 1). Consequently, in addition to the activation with cytokines, stimuli from autologous accessory cells can be used to further enhance the expansion of NK cells to overcome the hurdle of limited NK cell doses for adoptive NK cell therapy (Table 2). Outgrowth of NK cells from the whole PBMC fraction is more effective than cultivation of pure NK cells, because other cell types provide additional factors for NK cell proliferation. CD14+ cells, for instance, enhance the ex vivo NK cell proliferation via direct cell contact and soluble factors (108, 109). After activation, for instance by concanavalin A, T cells also trigger NK cell proliferation (110).
Stimulation of PBMC with IL-2 and the clinically approved anti-CD3 antibody OKT-3 leads to a profound outgrowth of NK cells (25, 65–67, 111), probably by activation of T cells and this is utilized by several clinical protocols for NK cell cultivation. Nevertheless, starting the culture from PBMC goes along with extensive coexpansion of unwanted CD3+/CD56− T cells and CD3+/CD56+ NK-like T (NKT) cells, accounting for the majority of cells in the final cellular product. Surprisingly, infusion of this heterogeneous cell product without removal of potentially alloreactive T cells did not cause side effects, such as GvHD, in a safety trial with five cancer patients, evaluating the cultivated cellular product in an allogeneic setting (66). This can be explained by the fact that T cells may lose their alloreactivity during extended ex vivo expansion (112). Thus, low NK cell purities may be less critical for long-term cultivated cellular products compared to NK cells directly obtained from a donor, but more clinical data are required to prove this hypothesis. Of note, the approach also allows efficient expansion of functional patient-derived NK cells, as shown for B cell chronic lymphocytic leukemia and multiple myeloma patients, enabling therapy with autologous NK cells and further circumventing possible safety risks of therapy with donor-derived cells (25, 111).
Starting with PBMC enriched for CD56 cells together with the corresponding non-CD56 PBMC in a 1:1 mixture favors a 89-fold NK cell expansion with a final product consisting of 92% NK cells after 21 days (69). Alternatively, adding irradiated autologous PBMC to the culture is a strategy to benefit from these “feeder cells” for NK cell activation and expansion but to avoid their coexpansion (Table 3). Of note, to make a clear difference, we use the term “feeder cells” for all inactivated cells that are added to the culture, whereas cocultured non-NK cells that are not inactivated are defined as “accessory cells.” Besides its growth inactivating function, irradiation can induce upregulation of stress-regulated surface molecules on PBMC, such as ULBP1–3, that further trigger NK cell activation, e.g., through NKG2D (113). Still, irradiated autologous PBMC induce only weak NK cell proliferation without additional activation of the feeder cells (e.g., only 16-fold expansion within 2 weeks) (24). Whereas irradiated autologous PBMC previously activated with IL-2, OKT-3 and RetroNectin allow a median 4,720-fold NK cell expansion after 3 weeks with a NK cell purity of 91% starting from PBMC (114). To obtain a more pure final product with 98% NK cells, it is possible to start the culture with already CD3-depleted PBMC and add irradiated autologous PBMC as feeder cells together with IL-2 and OKT-3 (23). The highest purity can be achieved by cell sorting, representing also the method of choice to expand defined NK cell subpopulations. As demonstrated by Siegler et al., GMP-sorted and highly pure single KIR+ NK cells can be expanded 160- to 390-fold in 19 days with IL-2, IL-15, OKT-3, and irradiated autologous PBMC (50).
NK Cell Expansion with Allogeneic Feeder Cells
Using irradiated allogeneic cells as feeder cells is another option to stimulate NK cell expansion ex vivo (118) (Table 4). Compared to autologous PBMC, allogeneic PBMC may be even more efficient as feeder cells for NK stimulation. Accordingly, in a study testing the expansion of NK cells from patients with advanced lymphomas or terminal solid tumors, 300-fold NK expansion was obtained with irradiated allogeneic PBMC feeder cells from healthy donors, whereas only 169-fold expansion was achieved with irradiated autologous PBMC feeder cells from the patients (115). Furthermore, whereas the availability of autologous feeder cells is limited, as they have to be obtained directly from the patient, for allogeneic feeder cells it is possible to utilize established cell lines. Cell lines can be grown easily to sufficient numbers and different cell lines in fact trigger NK cell proliferation, such as HFWT, K562, RPMI 1866, Daudi, KL-1, MM-170, and different EBV-transformed lymphoblastoid cell lines (EBV-LCL) (99, 119–122).
Culturing PBMC together with the Wilms tumor cell line HFWT and IL-2 leads to significant NK cell expansion (124, 145), and interestingly under this condition NK cells not only arise from mature CD3−CD56+ NK cells but also from CD3−CD14−CD19−CD56− NK cell precursors expressing CD122 (146). In 2004, early clinical data showed that adoptive transfer of autologous NK cells generated by coculture with irradiated HFWT is safe and patients with recurrent malignant glioma partially responded to the treatment (125).
Another advantage of cell lines is that it is relatively easy to genetically modify them and to integrate additional factors for NK cell stimulation. In recent years, modified K562 cells have been utilized, such as K562 expressing membrane-bound IL-15 and 41BBL (K562-mb15-41BBL) (126). While unmodified K562 only induce a weak NK cell proliferation (2.5-fold NK cell expansion in 1 week), with K562-mb15-41BBL the NK cell number can be significantly increased by 20- or 1,000-fold in 1 or 3 weeks (126). In addition, stimulation of NK cells with K562-mb15-41BBL demonstrated that NK cells actually have a substantial proliferative potential ex vivo, with up to 30 population doublings and 5.9 × 104-fold NK cell expansion (147). NK cells expanded with K562-mb15-41BBL exhibit enhanced natural cytotoxicity against several allogeneic and autologous tumors in vitro, efficiently mediate ADCC and showed antitumor efficacy in mouse xenograft models for the treatment of sarcoma and myeloma (128, 148, 149). Of note, in a clinical trial assessing adoptive transfer of K562-mb15-41BBL following HSCT, acute GvHD occurred in five of nine patients, although the donors were completely HLA matched and the doses of injected NK cells and cotransferred T cells were low (1–10 × 105 and ≤2 × 104/kg) (131). These observations suggested that the acute GvHD was T cell mediated, but NK cells apparently may promote this severe side effect indirectly (150). Importantly, another group utilized NK cells expanded with a similar K562 variant expressing 41BBL and IL-15 in another treatment setting and did not observe GvHD, although up to 1 × 108 NK cells/kg were administered (129).
Furthermore, Denman and colleagues revealed that K562 expressing 41BBL and membrane-bound IL-21 instead of IL-15 are even more effective for ex vivo expansion of NK cells, and weekly restimulation with this cell line supports a sustained NK cell proliferation over several weeks (134). In coculture with K562 expressing membrane-bound IL-21 and 41BBL, NK cells show an increased telomere length and enhanced activation of the STAT-3 signaling pathway, explaining the positive effect for sustained expansion of NK cells over long time (134, 151). Adoptive transfer of NK cells expanded with K562 expressing membrane-bound IL-21 and 41BBL into tumor-bearing mice improved the survival of the animals, indicating a therapeutic effect of these NK cells (135).
The stimulatory effect of EBV-LCL on NK cell proliferation was discovered more than 30 years ago (152). In 1994, an early clinical trial already evaluated the adoptive transfer of autologous NK cells expanded with the LAZ 388 cell line to treat 10 patients with metastatic renal cell adenocarcinoma (137). More recently, the cell lines TM-LCL and SMI-LCL were reported for NK cell expansion, allowing around 800-fold expansion of highly pure NK cells within 2 weeks (139–142). NK cells generated with these EBV-LCL feeder cells are currently applied in a study testing them for adoptive transfer in an autologous setting with intended doses up to 1 × 109 NK cells/kg (141). Recently, it was reported that repeated stimulation with SMI-LCL in IL-2-containing medium and adding IL-21 only at start of cultivation enables 1011-fold NK cell expansion after 6 weeks, to our knowledge representing the most efficient protocol to expand NK cells at the moment (96). NK cells generated with the latter method are highly cytotoxic in vitro, show a sustained high productivity of IFN-γ and TNF-α, similar to CIML NK cells, and they efficiently controlled melanoma in a xenograft mouse model (96).
Although feeder cells, and allogeneic feeder cell lines in particular, make it possible to generate substantial numbers of NK cells for adoptive therapy, from a regulatory point of view this strategy has drawbacks as feeder cell lines must be qualified as safe for human use. The cell line qualification of modified K562 cells, for instance, includes costly viral testing and assays to prove absence of bacterial and Mycoplasma contamination (153). In this context, lysates from cell lines containing the NK cell-stimulating factors could be an alternative to the intact feeder cells to minimize regulatory concerns. It was demonstrated that short cultivation of NK cells with lysate of the leukemia cell line CTV-1 primes NK cells to specifically lyse cell lines that are resistant to resting NK cells (143). Interestingly, the priming effect of CTV-1 on NK cells is KIR independent and does not require supplementation of cytokines, such as IL-2 or IL-15, making this an unique approach for NK cell activation (154). NK cells primed with CTV-1 were evaluated in the first UK clinical trial of a cell therapy regulated as a medicine, with an anti-leukemia effect in four of seven treated patients and no evidence of NK cell infusion-related toxicities (144). Another step forward from a regulatory standpoint could be to add only specific fragments of feeder cells to the culture that are responsible for the desired NK cell activation, instead of using intact feeder cells or their lysates. Of note, NK cells can be expanded ex vivo with IL-2 and plasma membrane particles prepared from K562-expressing membrane-bound IL-15 and 41BBL with a rate of expansion that is comparable to stimulation with intact feeder cells and far better than stimulation with soluble IL-15, 41BBL, and IL-2 (133). Plasma membrane particles from K562 expressing membrane-bound IL-21 and 41BBL work for ex vivo NK cell expansion as well and may be an option for in vivo NK cell expansion, as demonstrated in a first proof of concept using a mouse model (136).
Technical Aspects of NK Cell Expansion
In general, one encounters technical challenges and opportunities when manufacturing NK cells as medicinal products, as reviewed recently (155). In this section, we focus on technical options for NK cell culture, ranging from simple cell culture plates for small scale experiments to highly standardized and automated systems for clinical scale. The selection of the adequate culture system is based on the intended application of the cells. Most preclinical experimental studies grow NK cells in cell culture plates or tissue culture (T) flasks. These are commonly used and very convenient to test and compare different culture additives in parallel, e.g., different cytokine concentrations. However, for clinical applications in large scale, cultivation in plates and flasks is rather inappropriate for different reasons. First, due to the small volume of T flasks, numerous T flasks have to be handled at the same time, with for instance 51 T flasks for the treatment of a single patient (116). In addition, T flasks have to be opened from time to time for medium exchange or harvesting of cells, bearing the risk of contaminating the cellular product. Although the likelihood of contamination for each T flask is reduced to a minimum by sterile workflows in safety cabinets, the remaining risk potentates by the number of flasks.
To overcome the drawbacks of small cell culture vessels, clinical NK cell cultivation is often done in cell culture bags, which make it possible to culture high volumes in a closed system, as all required steps can be done by sterile welding of tubing connections for the transfer of media, harvesting of cells, etc. Unfortunately, different reports describe that the NK cell expansion performance is reduced after transition of a protocol from T flasks to larger scale in cell culture bags (50, 67). In addition, bag systems still require several labor-intensive interventions during the culture, especially when different cultures are set up in parallel.
The G-Rex vessel is another system avoiding frequent processing steps for exchange of medium during the culture. In contrast to normal cell culture flasks, the bottom of the G-Rex is highly gas permeable, ensuring optimal CO2 exchange and O2 supply for the cells. Thus, by its design, G-Rex flasks can be filled directly with a high level of cell culture medium and exchange of medium is not necessary for long time. For NK cell culture, G-Rex were used for example for 10 days of culture without any cell manipulation or feeding, and resulted in higher fold expansion of NK cells compared to cell culture bags (130). Unfortunately, although G-Rex are scalable in general, multiple G-Rex flasks are still required to achieve high cell numbers for clinical trials, which can be cumbersome and costly, and G-Rex flasks are still an open system and may require adaption to a closed system (156).
Automated systems combine the need for reduced interventions during the culture with a closed system. Automation of the cell manufacturing ensures constant product quality without the need for highly skilled experts, is finally cost saving, and may be required for cellular therapy to become available beyond specialized academic centers (157). Although early integration of automation is associated with higher capital costs in the development phase, it allows a smooth transition at later stages of clinical development (158). A first feasibility study of automated NK cell cultivation with a stirred bioreactor was already published in 1996, showing advantages of the bioreactor culture over manually handled controls (159). More recently, different investigators report automated NK cell expansion procedures with a rocking motion bioreactor (67, 69, 156, 160), yielding 2–10 × 109 NK cells under GMP-compliant conditions. However, the latter system still needs preceding manual cultivation, because relatively high cell numbers are required as inoculum for the automated culture (67, 69, 156, 160). Alternatively, fully automated NK cell expansion with an automated cell processing device can be performed for clinical use, with as little as 106 NK cells being sufficient to initiate the automated culture that can yield up to 2.7 × 109 NK cells after stimulation with clinical grade feeder cells (142). Of note, in addition to the culture process, the cell processing device is designed for GMP-compliant cell separation, concentration, and washing applications, so that combined NK cell purification, cultivation, and final formulation of the cellular product is possible fully automated (161). Thus, the whole processing, from the starting material, such as a leukapheresis product, to the finally expanded NK cells, readily prepared for infusion, can be covered by a single instrument.
Centralized processing of NK cell products probably will be carried out mainly in specialized centers for manufacturing of cellular products. Consequently, after ex vivo cultivation, storage of the NK cell product and shipment to the location of use will be needed. Compared to naive NK cells, IL-2-activated NK cells are less sensitive to freezing, as they show higher recovery and viability after thawing (26). Still, different groups state that cryopreservation of cultivated NK cells goes along with a drop in cell viability and cytotoxicity, whereas the latter can be restored by a short re-stimulation, e.g., by a short resting in IL-2-containing medium (139, 156). Poor survival of the NK cells can be an issue during further in vitro culture post thawing, so that shipping of freshly formulated cells for direct infusion may be advantageous (129). Interestingly, some groups recently claim that freezing and thawing does not influence the cytotoxicity or the proliferative ability of cultivated NK cells in their hands (24, 68). These divergent observations possibly result from different cultivation methods and different protocols for freezing and thawing, which should be investigated further. Without freezing, transport of the readily prepared cells in an appropriate time frame is challenging, and any delay during the shipment affects the quality of the cellular product with critical consequences for the patient. Alternatively, automated and closed systems for cell processing open the way for scale out strategies and de-centralized NK cell manufacturing directly at the location of intended use, avoiding the freezing and shipment process (142). But, although de-centralized manufacturing in the clinics seems promising, cellular therapeutics are very complex and still in early development, so that manufacturing by well-trained specialists in specific facilities is reasonable at that state.
Regulatory Aspects of NK Cell Cultivation for Clinical Use
Apart from technical difficulties, one has to consider regulatory aspects for the use of ex vivo-generated NK cells with regulations varying in time and geographical policies (153). In Europe, for instance, cytokine-activated and -expanded NK cells are currently classified as advanced therapy medicinal products and will be regulated accordingly either centralized or under the hospital exemption by the member states [Regulation (EC) No 1394/2007; Directive 2001/83/EC and Regulation (EC) No 726/2004]. Quality aspects related to somatic cell therapy medicinal product as defined in guidelines (CPMP/BWP/3088/99; EMEA/CHMP/410869/2006; Ph. Eur. 0784: Ph. Eur. 5.14) will apply to the identity, potency, and activity. The establishment of correspondingly adequate in process and quality controls as well as of process target values and product specifications will have to take into account the variability of the primary effector cell as the starting material (162).
Conclusion and Outlook
Comparing different protocols for NK cell cultivation in detail is challenging as these are extremely heterogeneous. The duration of ex vivo NK cell cultivation ranges from a few hours for short NK cell activation up to several weeks for long-term expansion, different starting materials are in use with varying NK cell purities, different cytokines are combined at different doses, and NK cells often are cocultured with different feeder cells at different NK-to-feeder ratios. Nevertheless, overall differently ex vivo expanded NK cells exhibit some common characteristics.
In general, ex vivo cultivated NK cells show an increased cytotoxicity and may become even responsive against tumor targets previously appearing resistant to NK cell lysis. This explains the use of IL-2 or IL-15 in virtually every protocol, as it is known since a long time that both cytokines amplify NK cell activity (81, 163). However, upon NK cell activation with different stimuli, including IL-2 and IL-15, downregulation of CD16 surface levels occurs by metalloproteases-mediated shedding of CD16 (164–166). The Fc receptor CD16 is crucial for NK cells to perform ADCC and would be of particular importance for potential combination therapies using NK cells together with therapeutic antibodies. Of note, although reduced levels of CD16 on NK cells are observed for several NK cell cultivation protocols the NK cells still mediate ADCC (70, 129, 142). Nevertheless, inhibition of the relevant metalloproteases to maintain CD16 on NK cells could be an option to further increase the ADCC function of ex vivo activated NK cells (164, 167).
Another clinically highly relevant aspect is the tumor-induced immunosuppression as important challenge for all cell therapeutic strategies. Remarkably, it ruled out from most preclinical and clinical NK cell studies that NK cells may gain the capability to overcome tumor immunosuppression. Different research groups have reported signs of NK cell suppression in cancer patients such as a lower expression of NK cell receptors, e.g., NCRs, NKG2D, DNAM-1, and 2B4 (22, 25, 168–170), the shedding of tumor cell ligands, such as NKp30 and NKG2D (171–174), or the release of blocking NKG2D ligands, such as MICA and ULBP3, via tumor-derived exosomes (175, 176). Notably, ex vivo cultivation of patient-derived NK cells is often possible with same efficacy as for donor-derived NK cells (25, 111) and can normalize the NK cell phenotype and activation (25). Additionally, elevated levels of NKG2D on ex vivo-activated NK cells can scavenge shed NKG2D ligands and counter their inhibitory effect (177). Furthermore, the high cytotoxicity of ex vivo expanded NK cells has been shown to be independent of KIR inhibition for some protocols (107, 132).
In comparison to other cell therapeutic approaches using, e.g., T cells, donor-derived allogeneic NK cells mediate GVL without an elevated risk for GVHD or even with a GVHD-reducing effect, as reported in mice and men (74, 75, 77, 78). However, contradictory results regarding GVHD induction have been reported in clinical trials assessing adoptive transfer of NK cells expanded with K562 feeder cell variants expressing 41BBL and IL-15 (129, 131). These reports show that there are still open questions that have to be unraveled to better understand the complex role of NK cells and their specific subsets in the bidirectional regulation of GVL and GVHD.
In conclusion, many different protocols are in use to expand NK cells in vitro, each with its specific advantages and disadvantages in regard of cell numbers, function, and handling efforts. The data summarized in this review underline the complexity related to the design of an optimal NK cell therapeutic protocol that should be not only reliable and safe in use but also highly efficient in targeting different forms of malignancies. With this in mind, additional studies need to be envisioned that not only further address ex vivo NK cell purification, expansion, and activation strategies but also the final clinical setting including pre-conditioning, dosing, and timing of the NK cell application. Efforts for harmonization of protocols at the European and worldwide level should be undertaken to ensure highest quality and efficacy of the NK cell product for clinical application. Finally, with regard to the possible tumor-mediated immunosuppression, therapeutic concepts have to be developed that either directly strengthen NK cells to deal with the hostile tumor environment and/or specifically counteract tumor-induced immunosuppressive mechanisms.
Author Contributions
MG, JW, and EU extensively reviewed the current literature on ex vivo NK cell cultivation and expansion and prepared a comprehensive overview that is listed in the tables. MG, JW, UK, AC, VH, and EU wrote and critically reviewed the manuscript.
Disclaimer
MG and VH were employed by company Miltenyi Biotec.
Conflict of Interest Statement
All authors, including MG and VH, declare that they have no commercial, proprietary, or financial conflict of interest.
Acknowledgments
The authors apologize to all investigators whose works were not cited in this article due to space limitations. EU and JW have been supported by the LOEWE Center for Cell and Gene Therapy, Frankfurt, funded by the Hessian Ministry of Higher Education, Research and the Arts, Germany (III L 4-518/17.004). AC’s work on cytokine-induced NK cells is supported by the Grant of German Cancer Aid 111455 and the GRK 2099.
References
1. Moretta A, Bottino C, Vitale M, Pende D, Cantoni C, Mingari MC, et al. Activating receptors and coreceptors involved in human natural killer cell-mediated cytolysis. Annu Rev Immunol (2001) 19:197–223. doi: 10.1146/annurev.immunol.19.1.197
2. Voskoboinik I, Smyth MJ, Trapani JA. Perforin-mediated target-cell death and immune homeostasis. Nat Rev Immunol (2006) 6:940–52. doi:10.1038/nri1983
3. Vivier E, Tomasello E, Baratin M, Walzer T, Ugolini S. Functions of natural killer cells. Nat Immunol (2008) 9:503–10. doi:10.1038/ni1582
4. Garrido F, Ruiz-Cabello F, Cabrera T, Pérez-Villar JJ, López-Botet M, Duggan-Keen M, et al. Implications for immunosurveillance of altered HLA class I phenotypes in human tumours. Immunol Today (1997) 18:89–95. doi:10.1016/S0167-5699(96)10075-X
5. Bottino C, Castriconi R, Moretta L, Moretta A. Cellular ligands of activating NK receptors. Trends Immunol (2005) 26:221–6. doi:10.1016/j.it.2005.02.007
6. Pende D, Cantoni C, Rivera P, Vitale M, Castriconi R, Marcenaro S, et al. Role of NKG2D in tumor cell lysis mediated by human NK cells: cooperation with natural cytotoxicity receptors and capability of recognizing tumors of nonepithelial origin. Eur J Immunol (2001) 31:1076–86. doi:10.1002/1521-4141(200104)31:4<1076:AID-IMMU1076>3.0.CO;2-Y
7. Pende D, Spaggiari GM, Marcenaro S, Martini S, Rivera P, Capobianco A, et al. Analysis of the receptor-ligand interactions in the natural killer-mediated lysis of freshly isolated myeloid or lymphoblastic leukemias: evidence for the involvement of the Poliovirus receptor (CD155) and Nectin-2 (CD112). Blood (2005) 105:2066–73. doi:10.1182/blood-2004-09-3548
8. Bauer S, Groh V, Wu J, Steinle A, Phillips JH, Lanier LL, et al. Activation of NK cells and T cells by NKG2D, a receptor for stress-inducible MICA. Science (1999) 285:727–9. doi:10.1126/science.285.5428.727
9. Ullrich E, Koch J, Cerwenka A, Steinle A. New prospects on the NKG2D/NKG2DL system for oncology. Oncoimmunology (2013) 2:e26097. doi:10.4161/onci.26097
10. Smyth MJ, Cretney E, Kelly JM, Westwood JA, Street SEA, Yagita H, et al. Activation of NK cell cytotoxicity. Mol Immunol (2005) 42:501–10. doi:10.1016/j.molimm.2004.07.034
11. Smyth MJ, Cretney E, Takeda K, Wiltrout RH, Sedger LM, Kayagaki N, et al. Tumor necrosis factor-related apoptosis-inducing ligand (TRAIL) contributes to interferon gamma-dependent natural killer cell protection from tumor metastasis. J Exp Med (2001) 193:661–70. doi:10.1084/jem.193.6.661
12. Smyth MJ, Crowe NY, Pellicci DG, Kyparissoudis K, Kelly JM, Takeda K, et al. Sequential production of interferon-gamma by NK1.1(+) T cells and natural killer cells is essential for the antimetastatic effect of alpha-galactosylceramide. Blood (2002) 99:1259–66. doi:10.1182/blood.V99.4.1259
13. Chawla-Sarkar M, Lindner DJ, Liu Y-F, Williams BR, Sen GC, Silverman RH, et al. Apoptosis and interferons: role of interferon-stimulated genes as mediators of apoptosis. Apoptosis (2003) 8:237–49. doi:10.1023/A:1023668705040
14. Costello RT, Sivori S, Marcenaro E, Lafage-Pochitaloff M, Mozziconacci M-J, Reviron D, et al. Defective expression and function of natural killer cell-triggering receptors in patients with acute myeloid leukemia. Blood (2002) 99:3661–7. doi:10.1182/blood.V99.10.3661
15. Salih HR, Rammensee H-G, Steinle A. Cutting edge: down-regulation of MICA on human tumors by proteolytic shedding. J Immunol (2002) 169:4098–102. doi:10.4049/jimmunol.169.8.4098
16. Salih HR, Goehlsdorf D, Steinle A. Release of MICB molecules by tumor cells: mechanism and soluble MICB in sera of cancer patients. Hum Immunol (2006) 67:188–95. doi:10.1016/j.humimm.2006.02.008
17. Kaiser BK, Yim D, Chow I-T, Gonzalez S, Dai Z, Mann HH, et al. Disulphide-isomerase-enabled shedding of tumour-associated NKG2D ligands. Nature (2007) 447:482–6. doi:10.1038/nature05768
18. Rouas-Freiss N, Moreau P, Ferrone S, Carosella ED. HLA-G proteins in cancer: do they provide tumor cells with an escape mechanism? Cancer Res (2005) 65:10139–44. doi:10.1158/0008-5472.CAN-05-0097
19. Urosevic M, Dummer R. Human leukocyte antigen-G and cancer immunoediting. Cancer Res (2008) 68:627–30. doi:10.1158/0008-5472.CAN-07-2704
20. Castriconi R, Dondero A, Bellora F, Moretta L, Castellano A, Locatelli F, et al. Neuroblastoma-derived TGF-β1 modulates the chemokine receptor repertoire of human resting NK cells. J Immunol (2013) 190:5321–8. doi:10.4049/jimmunol.1202693
21. Stringaris K, Sekine T, Khoder A, Alsuliman A, Razzaghi B, Sargeant R, et al. Leukemia-induced phenotypic and functional defects in natural killer cells predict failure to achieve remission in acute myeloid leukemia. Haematologica (2014) 99:836–47. doi:10.3324/haematol.2013.087536
22. Pietra G, Manzini C, Rivara S, Vitale M, Cantoni C, Petretto A, et al. Melanoma cells inhibit natural killer cell function by modulating the expression of activating receptors and cytolytic activity. Cancer Res (2012) 72:1407–15. doi:10.1158/0008-5472.CAN-11-2544
23. Lim O, Lee Y, Chung H, Her JH, Kang SM, Jung M-Y, et al. GMP-compliant, large-scale expanded allogeneic natural killer cells have potent cytolytic activity against cancer cells in vitro and in vivo. PLoS One (2013) 8:e53611. doi:10.1371/journal.pone.0053611
24. Torelli GF, Rozera C, Santodonato L, Peragine N, D’Agostino G, Montefiore E, et al. A good manufacturing practice method to ex vivo expand natural killer cells for clinical use. Blood Transfus (2015) 13:464–71. doi:10.2450/2015.0231-14
25. Alici E, Sutlu T, Björkstrand B, Gilljam M, Stellan B, Nahi H, et al. Autologous antitumor activity by NK cells expanded from myeloma patients using GMP-compliant components. Blood (2008) 111:3155–62. doi:10.1182/blood-2007-09-110312
26. Koehl U, Brehm C, Huenecke S, Zimmermann S-Y, Kloess S, Bremm M, et al. Clinical grade purification and expansion of NK cell products for an optimized manufacturing protocol. Front Oncol (2013) 3:118. doi:10.3389/fonc.2013.00118
27. Colombo MP, Trinchieri G. Interleukin-12 in anti-tumor immunity and immunotherapy. Cytokine Growth Factor Rev (2002) 13:155–68. doi:10.1016/S1359-6101(01)00032-6
28. Becknell B, Caligiuri MA. Interleukin-2, interleukin-15, and their roles in human natural killer cells. Adv Immunol (2005) 86:209–39. doi:10.1016/S0065-2776(04)86006-1
29. Theofilopoulos AN, Baccala R, Beutler B, Kono DH. Type I interferons (alpha/beta) in immunity and autoimmunity. Annu Rev Immunol (2005) 23:307–36. doi:10.1146/annurev.immunol.23.021704.115843
30. Burgess SJ, Marusina AI, Pathmanathan I, Borrego F, Coligan JE. IL-21 down-regulates NKG2D/DAP10 expression on human NK and CD8+ T cells. J Immunol (2006) 176:1490–7. doi:10.4049/jimmunol.176.3.1490
31. Ortaldo JR, Winkler-Pickett R, Wigginton J, Horner M, Bere EW, Mason AT, et al. Regulation of ITAM-positive receptors: role of IL-12 and IL-18. Blood (2006) 107:1468–75. doi:10.1182/blood-2005-04-1579
32. Terme M, Ullrich E, Delahaye NF, Chaput N, Zitvogel L. Natural killer cell-directed therapies: moving from unexpected results to successful strategies. Nat Immunol (2008) 9:486–94. doi:10.1038/ni1580
33. Koehl U, Kalberer C, Spanholtz J, Lee D, Miller J, Cooley S, et al. Advances in clinical NK cell studies: donor selection, manufacturing and quality control. Oncoimmunology (2015) 5(4):e1115178. doi:10.1080/2162402X.2015.1115178
34. Glienke W, Esser R, Priesner C, Suerth JD, Schambach A, Wels WS, et al. Advantages and applications of CAR-expressing natural killer cells. Front Pharmacol (2015) 6:21. doi:10.3389/fphar.2015.00021
35. Klingemann H. Challenges of cancer therapy with natural killer cells. Cytotherapy (2015) 17:245–9. doi:10.1016/j.jcyt.2014.09.007
36. Cany J, Dolstra H, Shah N. Umbilical cord blood-derived cellular products for cancer immunotherapy. Cytotherapy (2015) 17:739–48. doi:10.1016/j.jcyt.2015.03.005
37. Spanholtz J, Tordoir M, Eissens D, Preijers F, van der Meer A, Joosten I, et al. High log-scale expansion of functional human natural killer cells from umbilical cord blood CD34-positive cells for adoptive cancer immunotherapy. PLoS One (2010) 5:e9221. doi:10.1371/journal.pone.0009221
38. Suck G, Odendahl M, Nowakowska P, Seidl C, Wels WS, Klingemann HG, et al. NK-92: an “off-the-shelf therapeutic” for adoptive natural killer cell-based cancer immunotherapy. Cancer Immunol Immunother (2015) 65:485–92. doi:10.1007/s00262-015-1761-x
39. Knorr DA, Kaufman DS. Pluripotent stem cell-derived natural killer cells for cancer therapy. Transl Res (2010) 156:147–54. doi:10.1016/j.trsl.2010.07.008
40. Klingemann H, Boissel L, Toneguzzo F. Natural killer cells for immunotherapy – advantages of the NK-92 cell line over blood NK cells. Front Immunol (2016) 7:91. doi:10.3389/fimmu.2016.00091
41. Eguizabal C, Zenarruzabeitia O, Monge J, Santos S, Vesga MA, Maruri N, et al. Natural killer cells for cancer immunotherapy: pluripotent stem cells-derived NK cells as an immunotherapeutic perspective. Front Immunol (2014) 5:439. doi:10.3389/fimmu.2014.00439
42. Ferrara JLM, Levine JE, Reddy P, Holler E. Graft-versus-host disease. Lancet (2009) 373:1550–61. doi:10.1016/S0140-6736(09)60237-3
43. Miller JS, Soignier Y, Panoskaltsis-Mortari A, McNearney SA, Yun GH, Fautsch SK, et al. Successful adoptive transfer and in vivo expansion of human haploidentical NK cells in patients with cancer. Blood (2005) 105:3051–7. doi:10.1182/blood-2004-07-2974
44. Ho VT, Soiffer RJ. The history and future of T-cell depletion as graft-versus-host disease prophylaxis for allogeneic hematopoietic stem cell transplantation. Blood (2001) 98:3192–204. doi:10.1182/blood.V98.12.3192
45. Skeate R, Singh C, Cooley S, Geller M, Northouse J, Welbig J, et al. Hemolytic anemia due to passenger lymphocyte syndrome in solid malignancy patients treated with allogeneic natural killer cell products. Transfusion (2013) 53:419–23. doi:10.1111/j.1537-2995.2012.03942.x
46. McKenna DH, Sumstad D, Bostrom N, Kadidlo DM, Fautsch S, McNearney S, et al. Good manufacturing practices production of natural killer cells for immunotherapy: a six-year single-institution experience. Transfusion (2007) 47:520–8. doi:10.1111/j.1537-2995.2006.01145.x
47. Iyengar R, Handgretinger R, Babarin-Dorner A, Leimig T, Otto M, Geiger TL, et al. Purification of human natural killer cells using a clinical-scale immunomagnetic method. Cytotherapy (2003) 5:479–84. doi:10.1080/14653240310003558
48. Passweg JR, Tichelli A, Meyer-Monard S, Heim D, Stern M, Kuhne T, et al. Purified donor NK-lymphocyte infusion to consolidate engraftment after haploidentical stem cell transplantation. Leukemia (2004) 18:1835–8. doi:10.1038/sj.leu.2403524
49. Meyer-Monard S, Passweg J, Siegler U, Kalberer C, Koehl U, Rovó A, et al. Clinical-grade purification of natural killer cells in haploidentical hematopoietic stem cell transplantation. Transfusion (2009) 49:362–71. doi:10.1111/j.1537-2995.2008.01969.x
50. Siegler U, Meyer-Monard S, Jorger S, Stern M, Tichelli A, Gratwohl A, et al. Good manufacturing practice-compliant cell sorting and large-scale expansion of single KIR-positive alloreactive human natural killer cells for multiple infusions to leukemia patients. Cytotherapy (2010) 12:750–63. doi:10.3109/14653241003786155
51. van Ostaijen-ten Dam MM, Prins H-J, Boerman GH, Vervat C, Pende D, Putter H, et al. Preparation of cytokine-activated NK cells for use in adoptive cell therapy in cancer patients: protocol optimization and therapeutic potential. J Immunother (2016) 39:90–100. doi:10.1097/CJI.0000000000000110
52. Huenecke S, Zimmermann SY, Kloess S, Esser R, Brinkmann A, Tramsen L, et al. IL-2-driven regulation of NK cell receptors with regard to the distribution of CD16+ and CD16- subpopulations and in vivo influence after haploidentical NK cell infusion. J Immunother (2010) 33:200–10. doi:10.1097/CJI.0b013e3181bb46f7
53. Koehl U, Sörensen J, Esser R, Zimmermann S, Grüttner HP, Tonn T, et al. IL-2 activated NK cell immunotherapy of three children after haploidentical stem cell transplantation. Blood Cells Mol Dis (2004) 33:261–6. doi:10.1016/j.bcmd.2004.08.013
54. Boerman GH, van Ostaijen-ten Dam MM, Kraal KCJM, Santos SJ, Ball LM, Lankester AC, et al. Role of NKG2D, DNAM-1 and natural cytotoxicity receptors in cytotoxicity toward rhabdomyosarcoma cell lines mediated by resting and IL-15-activated human natural killer cells. Cancer Immunol Immunother (2015) 64:573–83. doi:10.1007/s00262-015-1657-9
55. Wendt K, Wilk E, Buyny S, Schmidt RE, Jacobs R. Interleukin-21 differentially affects human natural killer cell subsets. Immunology (2007) 122:486–95. doi:10.1111/j.1365-2567.2007.02675.x
56. Romee R, Schneider SE, Leong JW, Chase JM, Keppel CR, Sullivan RP, et al. Cytokine activation induces human memory-like NK cells. Blood (2012) 120:4751–60. doi:10.1182/blood-2012-04-419283
57. Ni J, Miller M, Stojanovic A, Garbi N, Cerwenka A. Sustained effector function of IL-12/15/18-preactivated NK cells against established tumors. J Exp Med (2012) 209:2351–65. doi:10.1084/jem.20120944
58. Leong JW, Chase JM, Romee R, Schneider SE, Sullivan RP, Cooper MA, et al. Preactivation with IL-12, IL-15, and IL-18 induces CD25 and a functional high-affinity IL-2 receptor on human cytokine-induced memory-like natural killer cells. Biol Blood Marrow Transplant (2014) 20:463–73. doi:10.1016/j.bbmt.2014.01.006
59. Berrien-Elliott MM, Wagner JA, Fehniger TA. Human cytokine-induced memory-like natural killer cells. J Innate Immun (2015) 7:563–71. doi:10.1159/000382019
60. Geller MA, Cooley S, Judson PL, Ghebre R, Carson LF, Argenta PA, et al. A phase II study of allogeneic natural killer cell therapy to treat patients with recurrent ovarian and breast cancer. Cytotherapy (2011) 13:98–107. doi:10.3109/14653249.2010.515582.A
61. Klingemann H, Grodman C, Cutler E, Duque M, Kadidlo D, Klein AK, et al. Autologous stem cell transplant recipients tolerate haploidentical related-donor natural killer cell-enriched infusions. Transfusion (2013) 53:412–8. doi:10.1111/j.1537-2995.2012.03764.x
62. Koepsell SA, Kadidlo DM, Fautsch S, McCullough J, Klingemann H, Wagner JE, et al. Successful “in-flight” activation of natural killer cells during long-distance shipping. Transfusion (2013) 53:398–403. doi:10.1111/j.1537-2995.2012.03695.x
63. Iliopoulou EG, Kountourakis P, Karamouzis MV, Doufexis D, Ardavanis A, Baxevanis CN, et al. A phase I trial of adoptive transfer of allogeneic natural killer cells in patients with advanced non-small cell lung cancer. Cancer Immunol Immunother (2010) 59:1781–9. doi:10.1007/s00262-010-0904-3
64. Choi I, Yoon SR, Park S-Y, Kim H, Jung S-J, Jang YJ, et al. Donor-derived natural killer cells infused after human leukocyte antigen-haploidentical hematopoietic cell transplantation: a dose-escalation study. Biol Blood Marrow Transplant (2014) 20:696–704. doi:10.1016/j.bbmt.2014.01.031
65. Carlens S, Gilljam M, Chambers BJ, Aschan J, Guven H, Ljunggren HG, et al. A new method for in vitro expansion of cytotoxic human CD3-CD56+ natural killer cells. Hum Immunol (2001) 62:1092–8. doi:10.1016/S0198-8859(01)00313-5
66. Barkholt L, Alici E, Conrad R, Sutlu T, Gilljam M, Stellan B, et al. Safety analysis of ex vivo-expanded NK and NK-like T cells administered to cancer patients: a phase I clinical study. Immunotherapy (2009) 1:753–64. doi:10.2217/imt.09.47
67. Sutlu T, Stellan B, Gilljam M, Quezada HC, Nahi H, Gahrton G, et al. Clinical-grade, large-scale, feeder-free expansion of highly active human natural killer cells for adoptive immunotherapy using an automated bioreactor. Cytotherapy (2010) 12:1044–55. doi:10.3109/14653249.2010.504770
68. Masuyama J, Murakami T, Iwamoto S, Fujita S. Ex vivo expansion of natural killer cells from human peripheral blood mononuclear cells co-stimulated with anti-CD3 and anti-CD52 monoclonal antibodies. Cytotherapy (2015) 18:1–6. doi:10.1016/j.jcyt.2015.09.011
69. Rujkijyanont P, Chan WK, Eldridge PW, Lockey T, Holladay M, Rooney B, et al. Ex vivo activation of CD56+ immune cells that eradicate neuroblastoma. Cancer Res (2013) 73:2608–18. doi:10.1158/0008-5472.CAN-12-3322
70. Deng X, Terunuma H, Nieda M, Xiao W, Nicol A. Synergistic cytotoxicity of ex vivo expanded natural killer cells in combination with monoclonal antibody drugs against cancer cells. Int Immunopharmacol (2012) 14:593–605. doi:10.1016/j.intimp.2012.09.014
71. Lotze MT, Grimm EA, Mazumder A, Strausser JL, Rosenberg SA. Lysis of fresh and cultured autologous tumor by human lymphocytes cultured in T-cell growth factor. Cancer Res (1981) 41:4420–5.
72. Grimm EA, Mazumder A, Zhang HZ, Rosenberg SA. Lymphokine-activated killer cell phenomenon. Lysis of natural killer-resistant fresh solid tumor cells by interleukin 2-activated autologous human peripheral blood lymphocytes. J Exp Med (1982) 155:1823–41. doi:10.1084/jem.155.6.1823
73. Burns LJ, Weisdorf DJ, DeFor TE, Vesole DH, Repka TL, Blazar BR, et al. IL-2-based immunotherapy after autologous transplantation for lymphoma and breast cancer induces immune activation and cytokine release: a phase I/II trial. Bone Marrow Transplant (2003) 32:177–86. doi:10.1038/sj.bmt.1704086
74. Asai O, Longo DL, Tian ZG, Hornung RL, Taub DD, Ruscetti FW, et al. Suppression of graft-versus-host disease and amplification of graft-versus-tumor effects by activated natural killer cells after allogeneic bone marrow transplantation. J Clin Invest (1998) 101:1835–42. doi:10.1172/JCI1268
75. Olson JA, Leveson-Gower DB, Gill S, Baker J, Beilhack A, Negrin RS. NK cells mediate reduction of GVHD by inhibiting activated, alloreactive T cells while retaining GVT effects. Blood (2010) 115:4293–301. doi:10.1182/blood-2009-05-222190
76. Ruggeri L, Capanni M, Urbani E, Perruccio K, Shlomchik WD, Tosti A, et al. Effectiveness of donor natural killer cell alloreactivity in mismatched hematopoietic transplants. Science (2002) 295:2097–100. doi:10.1126/science.1068440
77. Velardi A, Ruggeri L, Mancusi A, Aversa F, Christiansen FT. Natural killer cell allorecognition of missing self in allogeneic hematopoietic transplantation: a tool for immunotherapy of leukemia. Curr Opin Immunol (2009) 21:525–30. doi:10.1016/j.coi.2009.07.015
78. Meinhardt K, Kroeger I, Bauer R, Ganss F, Ovsiy I, Rothamer J, et al. Identification and characterization of the specific murine NK cell subset supporting graft-versus-leukemia- and reducing graft-versus-host-effects. Oncoimmunology (2015) 4:e981483. doi:10.4161/2162402X.2014.981483
79. Bachanova V, Burns LJ, McKenna DH, Curtsinger J, Panoskaltsis-Mortari A, Lindgren BR, et al. Allogeneic natural killer cells for refractory lymphoma. Cancer Immunol Immunother (2010) 59:1739–44. doi:10.1007/s00262-010-0896-z
80. Brehm C, Huenecke S, Quaiser A, Esser R, Bremm M, Kloess S, et al. IL-2 stimulated but not unstimulated NK cells induce selective disappearance of peripheral blood cells: concomitant results to a phase I/II study. PLoS One (2011) 6:e27351. doi:10.1371/journal.pone.0027351
81. Carson WE, Giri JG, Lindemann MJ, Linett ML, Ahdieh M, Paxton R, et al. Interleukin (IL) 15 is a novel cytokine that activates human natural killer cells via components of the IL-2 receptor. J Exp Med (1994) 180:1395–403. doi:10.1084/jem.180.4.1395
82. Carson WE, Fehniger TA, Haldar S, Eckhert K, Lindemann MJ, Lai CF, et al. A potential role for interleukin-15 in the regulation of human natural killer cell survival. J Clin Invest (1997) 99:937–43. doi:10.1172/JCI119258
83. Waldmann TA. The biology of interleukin-2 and interleukin-15: implications for cancer therapy and vaccine design. Nat Rev Immunol (2006) 6:595–601. doi:10.1038/nri1901
84. Fehniger TA, Cooper MA, Caligiuri MA. Interleukin-2 and interleukin-15: immunotherapy for cancer. Cytokine Growth Factor Rev (2002) 13:169–83. doi:10.1016/S1359-6101(01)00021-1
85. Waldmann TA, Lugli E, Roederer M, Perera LP, Smedley JV, Macallister RP, et al. Safety (toxicity), pharmacokinetics, immunogenicity, and impact on elements of the normal immune system of recombinant human IL-15 in rhesus macaques. Blood (2011) 117:4787–95. doi:10.1182/blood-2010-10-311456
86. Mao Y, van Hoef V, Zhang X, Wennerberg E, Lorent J, Witt K, et al. IL-15 activates mTOR and primes stress-activated gene expression leading to prolonged antitumor capacity of NK cells. Blood (2016) 128:1475–89. doi:10.1182/blood-2016-02-698027
87. Felices M, Lenvik A, Chu S, McElmurry R, Cooley S, Tolar J, et al. Continuous IL-15 signaling leads to functional exhaustion of human natural killer cells through metabolic changes that alters their in vivo anti-tumor activity. Blood (2016) 128:551.
88. Davis MR, Zhu Z, Hansen DM, Bai Q, Fang Y. The role of IL-21 in immunity and cancer. Cancer Lett (2015) 358:107–14. doi:10.1016/j.canlet.2014.12.047
89. Parrish-novak J, Dillon SR, Nelson A, Hammond A, Sprecher C, Gross JA, et al. Interleukin 21 and its receptor are involved in NK cell expansion and regulation of lymphocyte function. Nature (2000) 408:57–63. doi:10.1038/35040504
90. Kasaian MT, Whitters MJ, Carter LL, Lowe LD, Jussif JM, Deng B, et al. IL-21 limits NK cell responses and promotes antigen-specific T cell activation: a mediator of the transition from innate to adaptive immunity. Immunity (2002) 16:559–69. doi:10.1016/S1074-7613(02)00295-9
91. Brady J, Hayakawa Y, Smyth MJ, Nutt SL. IL-21 induces the functional maturation of murine NK cells. J Immunol (2004) 172:2048–58. doi:10.4049/jimmunol.172.4.2048
92. Venkatasubramanian S, Cheekatla S, Paidipally P, Tripathi D, Welch E, Tvinnereim AR, et al. IL-21-dependent expansion of memory-like NK cells enhances protective immune responses against Mycobacterium tuberculosis. Mucosal Immunol (2016):1–12. doi:10.1038/mi.2016.105
93. Strbo N, de Armas L, Liu H, Kolber MA, Lichtenheld M, Pahwa S. IL-21 augments natural killer effector functions in chronically HIV-infected individuals. AIDS (2008) 22:1551–60. doi:10.1097/QAD.0b013e3283089367
94. Li Q, Ye L-J, Ren H-L, Huyan T, Li J, Shi J-L, et al. Multiple effects of IL-21 on human NK cells in ex vivo expansion. Immunobiology (2015) 220:876–88. doi:10.1016/j.imbio.2015.01.009
95. Lim D-P, Jang Y-Y, Kim S, Koh SS, Lee J-J, Kim J-S, et al. Effect of exposure to interleukin-21 at various time points on human natural killer cell culture. Cytotherapy (2014) 16:1419–30. doi:10.1016/j.jcyt.2014.04.008
96. Granzin M, Stojanovic A, Miller M, Childs R, Huppert V, Cerwenka A. Highly efficient IL-21 and feeder cell-driven ex vivo expansion of human NK cells with therapeutic activity in a xenograft mouse model of melanoma. Oncoimmunology (2016) 5:e1219007. doi:10.1080/2162402X.2016.1219007
97. McMichael EL, Jaime-Ramirez AC, Guenterberg KD, Luedke E, Atwal LS, Campbell AR, et al. IL-21 enhances natural killer cell response to cetuximab-coated pancreatic tumor cells. Clin Cancer Res (2017) 23:489–502. doi:10.1158/1078-0432.CCR-16-0004
98. Iannello A, Boulassel M-R, Samarani S, Tremblay C, Toma E, Routy J-P, et al. IL-21 enhances NK cell functions and survival in healthy and HIV-infected patients with minimal stimulation of viral replication. J Leukoc Biol (2010) 87:857–67. doi:10.1189/jlb.1009701
99. Kobayashi M, Fitz L, Ryan M, Hewick RM, Clark SC, Chan S, et al. Identification and purification of natural killer cell stimulatory factor (NKSF), a cytokine with multiple biologic effects on human lymphocytes. J Exp Med (1989) 170:827–45. doi:10.1084/jem.170.3.827
100. D’Andrea A, Rengaraju M, Valiante NM, Chehimi J, Kubin M, Aste M, et al. Production of natural killer cell stimulatory factor (interleukin 12) by peripheral blood mononuclear cells. J Exp Med (1992) 176:1387–98. doi:10.1084/jem.176.5.1387
101. Vignali DAA, Kuchroo VK. IL-12 family cytokines: immunological playmakers. Nat Immunol (2012) 13:722–8. doi:10.1038/ni.2366
102. Wang KS, Frank DA, Ritz J. Interleukin-2 enhances the response of natural killer cells to interleukin-12 through up-regulation of the interleukin-12 receptor and STAT4. Blood (2000) 95:3183–90.
103. Chaix J, Tessmer MS, Hoebe K, Fuseri N, Ryffel B, Dalod M, et al. Cutting edge: priming of NK cells by IL-18. J Immunol (2008) 181:1627–31. doi:10.4049/jimmunol.181.3.1627
104. French AR, Holroyd EB, Yang L, Kim S, Yokoyama WM. IL-18 acts synergistically with IL-15 in stimulating natural killer cell proliferation. Cytokine (2006) 35:229–34. doi:10.1016/j.cyto.2006.08.006
105. Cooper MA, Elliott JM, Keyel PA, Yang L, Carrero JA, Yokoyama WM. Cytokine-induced memory-like natural killer cells. Proc Natl Acad Sci U S A (2009) 106:1915–9. doi:10.1073/pnas.0813192106
106. Ni J, Hölsken O, Miller M, Hammer Q, Luetke-Eversloh M, Romagnani C, et al. Adoptively transferred natural killer cells maintain long-term antitumor activity by epigenetic imprinting and CD4(+) T cell help. Oncoimmunology (2016) 5:e1219009. doi:10.1080/2162402X.2016.1219009
107. Romee R, Rosario M, Berrien-Elliott MM, Wagner JA, Jewell BA, Schappe T, et al. Cytokine-induced memory-like natural killer cells exhibit enhanced responses against myeloid leukemia. Sci Transl Med (2016) 8:357ra123. doi:10.1126/scitranslmed.aaf2341
108. Miller JS, Oelkers S, Verfaillie C, McGlave P. Role of monocytes in the expansion of human activated natural killer cells. Blood (1992) 80:2221–9.
109. Rölle A, Pollmann J, Ewen E, Le VTK, Halenius A, Hengel H, et al. IL-12-producing monocytes and HLA-E control HCMV-driven NKG2C+ NK cell expansion. J Clin Invest (2014) 124:5305–16. doi:10.1172/JCI77440
110. Rabinowich H, Sedlmayr P, Herberman RB, Whiteside TL. Increased proliferation, lytic activity, and purity of human natural killer cells cocultured with mitogen-activated feeder cells. Cell Immunol (1991) 135:454–70. doi:10.1016/0008-8749(91)90290-R
111. Guven H, Gilljam M, Chambers BJ, Ljunggren HG, Christensson B, Kimby E, et al. Expansion of natural killer (NK) and natural killer-like T (NKT)-cell populations derived from patients with B-chronic lymphocytic leukemia (B-CLL): a potential source for cellular immunotherapy. Leukemia (2003) 17:1973–80. doi:10.1038/sj.leu.2403083
112. Sutlu T, Alici E. Ex vivo expansion of natural killer cells: a question of function. Cytotherapy (2011) 13:767–8. doi:10.3109/14653249.2011.563295
113. Ahn Y-O, Kim S, Kim TM, Song EY, Park MH, Heo DS. Irradiated and activated autologous PBMCs induce expansion of highly cytotoxic human NK cells in vitro. J Immunother (2013) 36:373–81. doi:10.1097/CJI.0b013e3182a3430f
114. Sakamoto N, Ishikawa T, Kokura S, Okayama T, Oka K, Ideno M, et al. Phase I clinical trial of autologous NK cell therapy using novel expansion method in patients with advanced digestive cancer. J Transl Med (2015) 13:277. doi:10.1186/s12967-015-0632-8
115. Kim E-K, Ahn Y-O, Kim S, Kim TM, Keam B, Heo DS. Ex vivo activation and expansion of natural killer cells from patients with advanced cancer with feeder cells from healthy volunteers. Cytotherapy (2013) 15:231–41.e1. doi:10.1016/j.jcyt.2012.10.019
116. Parkhurst MR, Riley JP, Dudley ME, Rosenberg SA. Adoptive transfer of autologous natural killer cells leads to high levels of circulating natural killer cells but does not mediate tumor regression. Clin Cancer Res (2011) 17:6287–97. doi:10.1158/1078-0432.CCR-11-1347
117. Yang Y, Lim O, Kim TM, Ahn Y-O, Choi H, Chung H, et al. Phase I study of random healthy donor-derived allogeneic natural killer cell therapy in patients with malignant lymphoma or advanced solid tumors. Cancer Immunol Res (2016) 4:215–24. doi:10.1158/2326-6066.CIR-15-0118
118. Luhm J, Brand JM, Koritke P, Hoppner M, Kirchner H, Frohn C. Large-scale generation of natural killer lymphocytes for clinical application. J Hematother Stem Cell Res (2002) 11:651–7. doi:10.1089/15258160260194794
119. Bae DS, Lee JK. Development of NK cell expansion methods using feeder cells from human myelogenous leukemia cell line. Blood Res (2014) 49:154. doi:10.5045/br.2014.49.3.154
120. Fuchshuber PR, Lotzova E. Feeder cells enhance oncolytic and proliferative activity of long-term human bone marrow interleukin-2 cultures and induce different lymphocyte subsets. Cancer Immunol Immunother (1991) 33:15–20. doi:10.1007/BF01742522
121. Lim SA, Kim T-J, Lee JE, Sonn CH, Kim K, Kim J, et al. Ex vivo expansion of highly cytotoxic human nk cells by cocultivation with irradiated tumor cells for adoptive immunotherapy. Cancer Res (2013) 73(8):2598–607. doi:10.1158/0008-5472.CAN-12-2893
122. Warren HS, Kinnear BF, Skipsey LJ. Human natural killer (NK) cells: requirements for cell proliferation and expansion of phenotypically novel subpopulations. Immunol Cell Biol (1993) 71(Pt 2):87–97. doi:10.1038/icb.1993.9
123. Lister J, Rybka WB, Donnenberg AD, DeMagalhaes-Silverman M, Pincus SM, Bloom EJ, et al. Autologous peripheral blood stem cell transplantation and adoptive immunotherapy with activated natural killer cells in the immediate posttransplant period. Clin Cancer Res (1995) 1:607–14.
124. Harada H, Saijo K, Watanabe S, Tsuboi K, Nose T, Ishiwata I, et al. Selective expansion of human natural killer cells from peripheral blood mononuclear cells by the cell line, HFWT. Jpn J Cancer Res (2002) 93:313–9. doi:10.1111/j.1349-7006.2002.tb02174.x
125. Ishikawa E, Tsuboi K, Saijo K, Harada H, Takano S, Nose T, et al. Autologous natural killer cell therapy for human recurrent malignant glioma. Anticancer Res (2004) 24:1861–71.
126. Imai C, Iwamoto S, Campana D. Genetic modification of primary natural killer cells overcomes inhibitory signals and induces specific killing of leukemic cells. Blood (2005) 106:376–83. doi:10.1182/blood-2004-12-4797
127. Fujisaki H, Kakuda H, Shimasaki N, Imai C, Ma J, Lockey T, et al. Expansion of highly cytotoxic human natural killer cells for cancer cell therapy. Cancer Res (2009) 69:4010–7. doi:10.1158/0008-5472.CAN-08-3712
128. Garg TK, Szmania SM, Khan JA, Hoering A, Malbrough PA, Moreno-Bost A, et al. Highly activated and expanded natural killer cells for multiple myeloma immunotherapy. Haematologica (2012) 97:1348–56. doi:10.3324/haematol.2011.056747
129. Szmania S, Lapteva N, Garg T, Greenway A, Lingo J, Nair B, et al. Ex vivo-expanded natural killer cells demonstrate robust proliferation in vivo in high-risk relapsed multiple myeloma patients. J Immunother (2015) 38:24–36. doi:10.1097/CJI.0000000000000059
130. Lapteva N, Durett AG, Sun J, Rollins LA, Huye LL, Fang J, et al. Large-scale ex vivo expansion and characterization of natural killer cells for clinical applications. Cytotherapy (2012) 14:1131–43. doi:10.3109/14653249.2012.700767
131. Shah NN, Baird K, Delbrook CP, Fleisher TA, Kohler ME, Rampertaap S, et al. Acute GVHD in patients receiving IL-15/4-1BBL activated NK cells following T cell depleted stem cell transplantation. Blood (2014) 125:784–92. doi:10.1182/blood-2014-07-592881
132. Zhang H, Cui Y, Voong N, Sabatino M, Stroncek DF, Morisot S, et al. Activating signals dominate inhibitory signals in CD137L/IL-15 activated natural killer cells. J Immunother (2011) 34:187–95. doi:10.1097/CJI.0b013e31820d2a21
133. Oyer JL, Igarashi RY, Kulikowski AR, Colosimo DA, Solh MM, Zakari A, et al. Generation of highly cytotoxic natural killer cells for treatment of AML using feeder-free, particle based approach. Biol Blood Marrow Transplant (2015) 21:632–39. doi:10.1016/j.bbmt.2014.12.037
134. Denman CJ, Senyukov VV, Somanchi SS, Phatarpekar PV, Kopp LM, Johnson JL, et al. Membrane-bound IL-21 promotes sustained ex vivo proliferation of human natural killer cells. PLoS One (2012) 7:e30264. doi:10.1371/journal.pone.0030264
135. Liu Y, Wu H-W, Sheard MA, Sposto R, Somanchi SS, Cooper LJN, et al. Growth and activation of natural killer cells ex vivo from children with neuroblastoma for adoptive cell therapy. Clin Cancer Res (2013) 19:2132–43. doi:10.1158/1078-0432.CCR-12-1243
136. Oyer JL, Pandey V, Igarashi RY, Somanchi SS, Zakari A, Solh M, et al. Natural killer cells stimulated with PM21 particles expand and biodistribute in vivo: clinical implications for cancer treatment. Cytotherapy (2016) 18:653–63. doi:10.1016/j.jcyt.2016.02.006
137. Escudier B, Farace F, Angevin E, Charpentier F, Nitenberg G, Triebel F, et al. Immunotherapy with interleukin-2 (IL2) and lymphokine-activated natural killer cells: improvement of clinical responses in metastatic renal cell carcinoma patients previously treated with IL2. Eur J Cancer (1994) 30A:1078–83. doi:10.1016/0959-8049(94)90460-X
138. Hercend T, Farace F, Baume D, Charpentier F, Droz JP, Triebel F, et al. Immunotherapy with lymphokine-activated natural killer cells and recombinant interleukin-2: a feasibility trial in metastatic renal cell carcinoma. J Biol Response Mod (1990) 9:546–55.
139. Berg M, Lundqvist A, McCoy P Jr, Samsel L, Fan Y, Tawab A, et al. Clinical-grade ex vivo-expanded human natural killer cells up-regulate activating receptors and death receptor ligands and have enhanced cytolytic activity against tumor cells. Cytotherapy (2009) 11:341–55. doi:10.1080/14653240902807034
140. Park KU, Jin P, Sabatino M, Feng J, Civini S, Khuu H, et al. Gene expression analysis of ex vivo expanded and freshly isolated NK cells from cancer patients. J Immunother (2010) 33:945–55. doi:10.1097/CJI.0b013e3181f71b81
141. Childs RW, Berg M. Bringing natural killer cells to the clinic: ex vivo manipulation. Hematology Am Soc Hematol Educ Program (2013) 2013:234–46. doi:10.1182/asheducation-2013.1.234
142. Granzin M, Soltenborn S, Müller S, Kollet J, Berg M, Cerwenka A, et al. Fully automated expansion and activation of clinical-grade natural killer cells for adoptive immunotherapy. Cytotherapy (2015) 17:621–32. doi:10.1016/j.jcyt.2015.03.611
143. North J, Bakhsh I, Marden C, Pittman H, Addison E, Navarrete C, et al. Tumor-primed human natural killer cells lyse NK-resistant tumor targets: evidence of a two-stage process in resting NK cell activation. J Immunol (2007) 178:85–94. doi:10.4049/jimmunol.178.1.85
144. Kottaridis PD, North J, Tsirogianni M, Marden C, Samuel ER, Jide-Banwo S, et al. Two-stage priming of allogeneic natural killer cells for the treatment of patients with acute myeloid leukemia: a phase I trial. PLoS One (2015) 10:e0123416. doi:10.1371/journal.pone.0123416
145. Peng B-G, Liang L-J, He Q, Huang J-F, Lu M-D. Expansion and activation of natural killer cells from PBMC for immunotherapy of hepatocellular carcinoma. World J Gastroenterol (2004) 10:2119–23. doi:10.3748/wjg.v10.i14.2119
146. Harada H, Watanabe S, Saijo K, Ishiwata I, Ohno T. A Wilms tumor cell line, HFWT, can greatly stimulate proliferation of CD56+ human natural killer cells and their novel precursors in blood mononuclear cells. Exp Hematol (2004) 32:614–21. doi:10.1016/j.exphem.2004.03.011
147. Fujisaki H, Kakuda H, Imai C, Mullighan CG, Campana D. Replicative potential of human natural killer cells. Br J Haematol (2009) 145:606–13. doi:10.1111/j.1365-2141.2009.07667.x
148. Cho D, Shook DR, Shimasaki N, Chang Y-H, Fujisaki H, Campana D. Cytotoxicity of activated natural killer cells against pediatric solid tumors. Clin Cancer Res (2010) 16:3901–9. doi:10.1158/1078-0432.CCR-10-0735
149. Voskens CJ, Watanabe R, Rollins S, Campana D, Hasumi K, Mann DL. Ex-vivo expanded human NK cells express activating receptors that mediate cytotoxicity of allogeneic and autologous cancer cell lines by direct recognition and antibody directed cellular cytotoxicity. J Exp Clin Cancer Res (2010) 29:134. doi:10.1186/1756-9966-29-134
150. Lee DA. The off-target effects of nonspecific NK cells. Blood (2015) 125:744–5. doi:10.1182/blood-2014-12-616359
151. Wang X, Lee DA, Wang Y, Wang L, Yao Y, Lin Z, et al. Membrane-bound interleukin-21 and CD137 ligand induce functional human natural killer cells from peripheral blood mononuclear cells through STAT-3 activation. Clin Exp Immunol (2013) 172:104–12. doi:10.1111/cei.12034
152. Hercend T, Meuer S, Reinherz EL, Schlossman SF, Ritz J. Generation of a cloned NK cell line derived from the “null cell” fraction of human peripheral blood. J Immunol (1982) 129:1299–305.
153. Lee DA. Regulatory considerations for NK cells used in human immunotherapy applications. Methods Mol Biol (2016) 1441:347–61. doi:10.1007/978-1-4939-3684-7_29
154. Sabry M, Tsirogianni M, Bakhsh IA, North J, Sivakumaran J, Giannopoulos K, et al. Leukemic priming of resting NK cells is killer Ig-like receptor independent but requires CD15-mediated CD2 ligation and natural cytotoxicity receptors. J Immunol (2011) 187:6227–34. doi:10.4049/jimmunol.1101640
155. Chabannon C, Mfarrej B, Guia S, Ugolini S, Devillier R, Blaise D, et al. Manufacturing natural killer cells as medicinal products. Front Immunol (2016) 7:504. doi:10.3389/fimmu.2016.00504
156. Lapteva N, Szmania SM, van Rhee F, Rooney CM. Clinical grade purification and expansion of natural killer cells. Crit Rev Oncog (2014) 19:121–32. doi:10.1615/CritRevOncog.2014010931
157. Kalos M, June CH. Adoptive T cell transfer for cancer immunotherapy in the era of synthetic biology. Immunity (2013) 39:49–60. doi:10.1016/j.immuni.2013.07.002
158. Rafiq QA, Thomas RJ. The evolving role of automation in process development and manufacture of cell and gene-based therapies. Cell Gene Ther Insights (2016) 2:473–9. doi:10.18609/cgti.2016.058
159. Pierson BA, Europa AF, Hu WS, Miller JS. Production of human natural killer cells for adoptive immunotherapy using a computer-controlled stirred-tank bioreactor. J Hematother (1996) 5:475–83. doi:10.1089/scd.1.1996.5.475
160. Spanholtz J, Preijers F, Tordoir M, Trilsbeek C, Paardekooper J, de Witte T, et al. Clinical-grade generation of active NK cells from cord blood hematopoietic progenitor cells for immunotherapy using a closed-system culture process. PLoS One (2011) 6:e20740. doi:10.1371/journal.pone.0020740
161. Apel M, Brüning M, Granzin M, Essl M, Stuth J, Blaschke J, et al. Integrated clinical scale manufacturing system for cellular products derived by magnetic cell separation, centrifugation and cell culture. Chemie-Ingenieur-Technik (2013) 85:103–10. doi:10.1002/cite.201200175
162. Schüle S, Renner M, Longhurst S, Narayanan G. Regulatory requirements for clinical trial and marketing authorisation application for gene therapy medicinal products. Bundesgesundheitsblatt Gesundheitsforschung Gesundheitsschutz (2010) 53:30–7. doi:10.1007/s00103-009-0988-0
163. Caligiuri MA, Zmuidzinas A, Manley TJ, Levine H, Smith KA, Ritz J. Functional consequences of interleukin 2 receptor expression on resting human lymphocytes. Identification of a novel natural killer cell subset with high affinity receptors. J Exp Med (1990) 171:1509–26. doi:10.1084/jem.171.5.1509
164. Romee R, Foley B, Lenvik T, Wang Y, Zhang B, Ankarlo D, et al. NK cell CD16 surface expression and function is regulated by a disintegrin and metalloprotease-17 (ADAM17). Blood (2013) 121:3599–608. doi:10.1182/blood-2012-04-425397
165. Peruzzi G, Femnou L, Gil-Krzewska A, Borrego F, Weck J, Krzewski K, et al. Membrane-type 6 matrix metalloproteinase regulates the activation-induced downmodulation of CD16 in human primary NK cells. J Immunol (2013) 191:1883–94. doi:10.4049/jimmunol.1300313
166. Lajoie L, Congy-Jolivet N, Bolzec A, Gouilleux-Gruart V, Sicard E, Sung HC, et al. ADAM17-mediated shedding of FcγRIIIA on human NK cells: identification of the cleavage site and relationship with activation. J Immunol (2014) 192:741–51. doi:10.4049/jimmunol.1301024
167. Wiernik A, Foley B, Zhang B, Verneris MR, Warlick E, Gleason MK, et al. Targeting natural killer cells to acute myeloid leukemia in vitro with a CD16 x 33 bispecific killer cell engager and ADAM17 inhibition. Clin Cancer Res (2013) 19:3844–55. doi:10.1158/1078-0432.CCR-13-0505
168. Fauriat C, Just-Landi S, Mallet F, Arnoulet C, Sainty D, Olive D, et al. Deficient expression of NCR in NK cells from acute myeloid leukemia: evolution during leukemia treatment and impact of leukemia cells in NCRdull phenotype induction. Blood (2007) 109:323–30. doi:10.1182/blood-2005-08-027979
169. Le Maux Chansac B, Moretta A, Vergnon I, Opolon P, Lécluse Y, Grunenwald D, et al. NK cells infiltrating a MHC class I-deficient lung adenocarcinoma display impaired cytotoxic activity toward autologous tumor cells associated with altered NK cell-triggering receptors. J Immunol (2005) 175:5790–8. doi:10.1182/blood-2005-08-027979
170. Carlsten M, Norell H, Bryceson YT, Poschke I, Schedvins K, Ljunggren H-G, et al. Primary human tumor cells expressing CD155 impair tumor targeting by down-regulating DNAM-1 on NK cells. J Immunol (2009) 183:4921–30. doi:10.4049/jimmunol.0901226
171. Doubrovina ES, Doubrovin MM, Vider E, Sisson RB, O’Reilly RJ, Dupont B, et al. Evasion from NK cell immunity by MHC class I chain-related molecules expressing colon adenocarcinoma. J Immunol (2003) 171:6891–9. doi:10.4049/jimmunol.171.12.6891
172. Hilpert J, Grosse-Hovest L, Grünebach F, Buechele C, Nuebling T, Raum T, et al. Comprehensive analysis of NKG2D ligand expression and release in leukemia: implications for NKG2D-mediated NK cell responses. J Immunol (2012) 189:1360–71. doi:10.4049/jimmunol.1200796
173. Reiners KS, Topolar D, Henke A, Simhadri VR, Kessler J, Sauer M, et al. Soluble ligands for NK cell receptors promote evasion of chronic lymphocytic leukemia cells from NK cell anti-tumor activity. Blood (2013) 121:3658–65. doi:10.1182/blood-2013-01-476606
174. Schlecker E, Fiegler N, Arnold A, Altevogt P, Rose-John S, Moldenhauer G, et al. Metalloprotease-mediated tumor cell shedding of B7-H6, the ligand of the natural killer cell-activating receptor NKp30. Cancer Res (2014) 74:3429–40. doi:10.1158/0008-5472.CAN-13-3017
175. Ashiru O, Boutet P, Fernández-Messina L, Agüera-González S, Skepper JN, Valés-Gómez M, et al. Natural killer cell cytotoxicity is suppressed by exposure to the human NKG2D ligand MICA*008 that is shed by tumor cells in exosomes. Cancer Res (2010) 70:481–9. doi:10.1158/0008-5472.CAN-09-1688
176. Fernández-Messina L, Ashiru O, Boutet P, Agüera-González S, Skepper JN, Reyburn HT, et al. Differential mechanisms of shedding of the glycosylphosphatidylinositol (GPI)-anchored NKG2D ligands. J Biol Chem (2010) 285:8543–51. doi:10.1074/jbc.M109.045906
Keywords: natural killer cells, natural killer cell cultivation, natural killer cell expansion, natural killer cell therapy, natural killer cell cytotoxicity, ex vivo stimulation
Citation: Granzin M, Wagner J, Köhl U, Cerwenka A, Huppert V and Ullrich E (2017) Shaping of Natural Killer Cell Antitumor Activity by Ex Vivo Cultivation. Front. Immunol. 8:458. doi: 10.3389/fimmu.2017.00458
Received: 28 January 2017; Accepted: 04 April 2017;
Published: 26 April 2017
Edited by:
Hermann Einsele, University of Würzburg, GermanyReviewed by:
Reem Al-Daccak, Institut national de la santé et de la recherche médicale (INSERM), FranceStanislaw Stepkowski, University of Toledo, USA
Copyright: © 2017 Granzin, Wagner, Köhl, Cerwenka, Huppert and Ullrich. This is an open-access article distributed under the terms of the Creative Commons Attribution License (CC BY). The use, distribution or reproduction in other forums is permitted, provided the original author(s) or licensor are credited and that the original publication in this journal is cited, in accordance with accepted academic practice. No use, distribution or reproduction is permitted which does not comply with these terms.
*Correspondence: Markus Granzin, bWFya3VzLmdyYW56aW5AbWlsdGVueWliaW90ZWMuY29t;
Evelyn Ullrich, ZXZlbHluLnVsbHJpY2hAa2d1LmRl