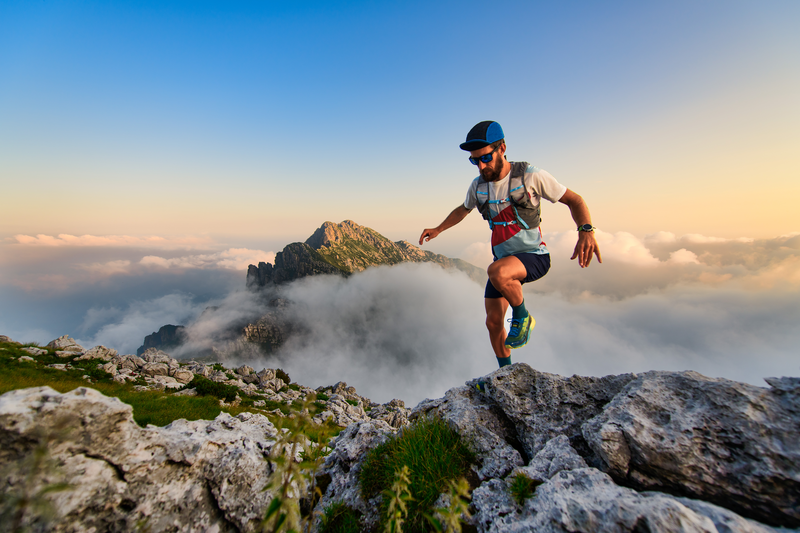
95% of researchers rate our articles as excellent or good
Learn more about the work of our research integrity team to safeguard the quality of each article we publish.
Find out more
OPINION article
Front. Immunol. , 27 March 2017
Sec. Immunological Tolerance and Regulation
Volume 8 - 2017 | https://doi.org/10.3389/fimmu.2017.00342
This article is part of the Research Topic Ying and Yang of members of TNF superfamily: Friends or Foes in immune-mediated diseases and cancer View all 9 articles
Receptor/ligand interactions of the tumor necrosis factor (TNF) superfamily are associated with versatile apoptotic and regulatory signaling pathways in parenchymal tissues and the immunohematopoietic system. Apoptotic signaling mediated by Fas and TNF receptor-1 (TNF-R1) is one of the major cytotoxic mechanisms used by immune cells to kill endogenous cells and exogenous pathogens. In this capacity, these ubiquitous effector mechanisms of cell death, along with perforin/granzyme, are direct mediators of β-cell injury that is inflicted in autoimmune insulitis in type 1 diabetes (T1D). At the same time, the TNF family receptor/ligand interactions are prime constituents of immune homeostasis that enforce negative regulation of sensitized immunocytes (1–3). All immune cells upregulate TNF family receptors upon activation and are therefore submitted to negative regulation by apoptosis within the process of activation-induced cell death (AICD), which is also essential to termination of inflammation (4).
Tumor necrosis factor family receptor/ligand interactions participate as universal cytotoxic mechanisms in physiological turnover of cells, removal of dysfunctional cellular elements, and clearance of debris following injury of parenchymal tissues. As ubiquitous injury factors, the TNF family ligands concomitantly activate precursors and synergize with other growth factors to induce generation of renewed functional parenchymal cells to replace the injured tissue. For example, the Fas receptor and the cognate Fas-ligand (FasL) are constitutively expressed and transduce trophic regulatory signals in tissues such as glia, neurons, hepatocytes, and endothelium (5–7). Likewise, trophic signals are transduced by TNF superfamily receptors in hematopoietic stem and progenitor cells, which synergize with other growth factors to foster hematopoiesis under stress conditions (8). Therefore, common injury factors such as TNFα and interferons (IFN) couple hematopoietic activity to inflammation and injury in order to fuel immune reactions and support initial resolution of injury (9).
The Fas/FasL interaction is a common effector mechanism of β-cell apoptosis and islet injury under inflammatory conditions, and physiological modulation of immune homeostasis, including control of aberrant autoimmune reactions. In the absence of specific characteristics of the toxic and anti-inflammatory pathways, it is questioned whether neutralization or reinforcement of the Fas/FasL interaction is of therapeutic value in autoimmune disorders. We examine a wide array of evidence arguing in favor of and against therapeutic neutralization of Fas and/or FasL and conversely, consider the feasibility of implementation of this molecular interaction as approaches to abrogate autoimmune diabetes. Despite focus on a particular signaling pathway, the Fas/FasL interaction, in a particular autoimmune disorder, T1D, the debate is rather relevant to multiple receptor/ligand interactions of the TNF superfamily and to the entire range of inflammatory and autoimmune disorders.
The Fas/FasL interaction attracts attention as the common executioner of apoptosis in the TNF superfamily endowed with distinct characteristics (10). Like perforin/granzyme (11, 12), apoptotic signaling by Fas/FasL is localized by the requirement of direct contact between the effector and target cells, a unique feature caused by essential Fas receptor trimerization through engagement of the membrane-bound or oligomers of the ligand. The physiological significance of this receptor/ligand interaction is emphasized by lymphoproliferative disorders resulting from disruption of homeostatic negative regulation in Fas-deficient (lpr) and FasL-defective (gld) mice (13). Despite these particular characteristics of the Fas/FasL interaction, common physiological trophic and apoptotic activities are shared by soluble ligands of the TNF superfamily, including TNFα and TNF-related apoptosis-inducing ligand receptor-1 (8).
Several components of the signaling pathways associated with the Fas receptor are involved in insulin secretion (14, 15) and physiological adjustment of β-cell mass. For example, the antiapoptotic factor FADD-like interleukin-1β-converting enzyme-inhibitory protein acting as a competitive caspase-8 antagonist promotes β-cell growth (16) under the inductive influence of interleukin-1β (IL-1β) (17). The Fas signaling pathway is also involved in regulation of insulin secretion (14, 15).
Physiological immune privilege uses FasL to defend organs from excessive inflammation that is often more harmful than pathogens, such as the anterior eye chamber and reproductive organs (10, 18). The pancreas is not one of the first line immune privileged tissues. However, both islet cells and vascular endothelium constitutively express FasL, while the Fas receptor is prevalently detected in resident macrophages (19, 20) and in inflamed islets (21, 22). The histological pattern of expression is suggestive of a defensive rim of FasL-expressing α- and β-cells in the islets of Langerhans (23). In fact, the frontier battleground between reactive T cells and tissues is islet vasculature (24), which constitutively expresses both Fas and FasL (25) and is therefore inherently insensitive to Fas-mediated apoptosis (26, 27). Protection of the islets by relative immune privilege represents a wider network of defense of the pancreas from incidental inflammation and immune attack (28) due to the extensive digestive capacity of pancreatic enzymes responsible for intestinal degradation of substrates, which might cause autolytic pancreatitis associated with severe morbidity and mortality.
Naïve islets express low levels of Fas (21, 22) and are intrinsically resistant to apoptosis triggered by this receptor (29, 30). Islets become gradually sensitive to apoptosis along the course of inflammation (31–33) due to increased Fas expression in β-cells (34, 35) and concomitant sensitization to Fas-mediated apoptosis (36, 37). Upregulated expression of the Fas receptor is one of the many features of the transcriptional profiles of inflamed islets (38, 39) caused by activation of the nuclear factor-κB (NFκB) pathways (40). Induced Fas transcription and modulation of expression is caused by a number of proinflammatory cytokines including IL-1α, IL-1β, IFNγ, nitric oxide (NO), and TNF-α that synergize with Fas as effector mechanisms of β-cell destruction (37, 41). Furthermore, both Fas expression induced by IL-1β (42) independent of NFκB activation (43, 44) and islet sensitization to apoptosis (45, 46) also evolve as direct consequences of hyperglycemia. The vast changes in transcriptomes and expression profiles of the inflamed islets may be viewed as an effort of the tissue to sustain insulin production and increase β-cell mass. However, cytokines secreted by the islets themselves paradoxally enhance immune activation and islet injury under inflammatory conditions (47–49).
There is extensive evidence emphasizing a pivotal role of the Fas/FasL interaction in destructive insulitis in T1D, including experiments performed in transgenes deficient in the receptor and/or the ligand. For example, homozygous transgenes of non-obese diabetic (NOD) mice deficient in the Fas receptor (lpr) are protected from spontaneous evolution of diabetes, and heterozygous NOD. lpr transgenes display severe mononuclear infiltration in the islets without hyperglycemia (50, 51). However, NOD. lpr with and without superposed SCID mutations that display reduced incidence of spontaneous diabetes are susceptible to islet injury inflicted by adoptive transfer of diabetogenic cells (52). Likewise, disease incidence is reduced in FasL-deficient transgenes (gld) crossed onto the NOD background (52, 53). It is logical and tempting to approach inflammatory insulitis by neutralization of the Fas/FasL interaction as one of the pivotal cytotoxic mechanisms used by diabetogenic effectors to attack β-cells (54–57), similar to the potential therapeutic benefit of TNF-α neutralization (58). However, it should be noted that FasL neutralization in the early postnatal period (53, 59) and in very early stages of inflammation slowed the pace but failed to prevent evolution of insulitis (60).
The insights into effective disease diversion by interruption of functional Fas/FasL signaling led to identification of a number of indirect immunogenic factors that modulate the course of inflammation in transgenic mice. The variable patterns of disease expression in lpr transgenes are explained by two mechanisms beyond relative insensitivity of Fas-deficient islets to apoptosis. First, slow pace of spontaneous inflammatory insulitis is attributed to reduced aggressiveness and slow proliferation of effector lymphocytes of the lpr transgenes (61). Second, T cells of lpr transgenes overexpress FasL, which inhibits the activity of endogenous and adoptively transferred diabetogenic cells (62). In variance, the pace of disease is slowed in gld transgenes by the activity of B lymphocytes that attenuate the course of inflammation by enhanced secretion of IL-10 (63). It is therefore evident that disruption of a pivotal immune homeostatic mechanism such as the Fas/FasL interaction has quite significant consequences that affect autoimmunity beyond direct participation as a cytotoxic mechanism of islet injury.
The difficulty in designation of an exact role of Fas-mediated apoptosis stands in the multiple, redundant, and interrelated mechanisms of β-cell death in T1D. The progressive involvement of Fas as a mediator of apoptosis along the course of inflammation has been challenged by a series of studies showing that this mechanism is neither obligatory nor essential in the process of destructive insulitis (64–68). Although Fas expression correlates with β-cell inflammation and unequivocally contributes to destructive insulitis, a causal relationship is rather complex because apoptosis also correlates with upregulation of granzyme and TNF-R1 (69–72). In addition to the canonical mechanisms of apoptosis, islet injury is inflicted by a number of cytotoxic cytokines such as IL-1β, IFNγ, and NO (73, 74). It is quite difficult to attribute distinct activities to these interrelated mechanisms in the process of inflammatory insulitis, because most cytokines as well as TNFα induce Fas expression (21, 22, 29, 75).
Each one of the canonical cytotoxic mechanisms, including Fas, TNF-R1, and perforin/granzyme, is dispensable in autoimmune β-cell destruction (68, 75–80), as well as islet allograft rejection (27, 81). Outstanding is the compensation of dysfunctional Fas/FasL interactions by other effector mechanisms of β-cell death (11, 35, 69, 82–84). Likewise, redundant activity of TGF-β, IL-1β, IFNγ, and NO is a common characteristic, as each individual cytotoxic mechanism is largely dispensable in β-cell lysis (36, 41, 47, 85).
Extending the mechanism of immune privilege, negative regulation of immune cells by TNF family receptor/ligand interactions has significant homeostatic impact on the intensity of inflammatory reactions. The common mechanism of tissue defense involves induction of apoptosis in autoreactive effectors sensitive to AICD at the level of pancreatic islets in situ (30, 62, 86). Physical elimination of diabetogenic cells has been attained by targeted expression of TNFα (87–90) and TGFβ (91, 92) under control of the insulin promoter, systemic administration of TNF-α (93–95), and overexpression of FasL protein in regulatory T cells (30, 96).
The analysis presented here suggests that inhibition of the Fas/FasL interaction has little potential efficacy in prevention of β-cell destruction by inflammatory insulitis, while targeted reinforcement of this mechanisms of immune homeostasis holds the potential to abrogate diabetic autoimmunity. Signaling through Fas receptor is one of many redundant and dispensable mechanisms of β-cell lysis by autoimmune attack; thus, neutralization provides transient symptomatic relief with little impact on alternative cytotoxic mechanisms (64–67). Implementation of FasL neutralization for treatment of T1D might even result in increased incidence of malignancies as seen with TNF-α inhibitors (97–99). On the contrary, the role of FasL along TNF-α and perforin/granzyme is much more significant and non-redundant in immune homeostasis than in induction of β-cell death (10, 61, 62, 88, 100). These homeostatic immune mechanisms counteract inflammation as mediators of effector cell death (27, 30, 86, 89–96) are mandatory to reinstitution of suppressor mechanisms (101) and are indispensable in termination of inflammatory reactions (4).
All authors contributed equally to this manuscript.
HS and EY are inventors of several patents related to Fas-ligand. NA discloses no potential conflict of interest.
No dedicated funding to be declared.
1. Cohen JJ, Duke RC. Apoptosis and programmed cell death in immunity. Ann Rev Immunol (1992) 10:267–93. doi:10.1146/annurev.immunol.10.1.267
2. Kabelitz D, Pohl T, Pechhold K. Activation-induced cell death (apoptosis) of mature peripheral T lymphocytes. Immunol Today (1993) 14:338–9. doi:10.1016/0167-5699(93)90231-9
3. Nagata S, Golstein P. The Fas death factor. Science (1995) 267:1449–56. doi:10.1126/science.7533326
4. Yolcu ES, Ash S, Kaminitz A, Sagiv Y, Askenasy N, Yarkoni S. Apoptosis as a mechanism of T-regulatory cell homeostasis and suppression. Immunol Cell Biol (2008) 86:650–8. doi:10.1038/icb.2008.62
5. Desbarats J, Newell MK. Fas engagement accelerates liver regeneration after partial hepatectomy. Nat Med (2000) 6:920–3. doi:10.1038/78688
6. Lambert C, Landau AM, Desbarats J. Fas-beyond death: a regenerative role for Fas in the nervous system. Apoptosis (2003) 8:551–62. doi:10.1023/A:1026113222478
7. Song JH, Bellail A, Tse MC, Yong VW, Hao C. Human astrocytes are resistant to Fas ligand and tumor necrosis factor-related apoptosis-inducing ligand-induced apoptosis. J Neurosci (2006) 26:3299–308. doi:10.1523/JNEUROSCI.5572-05.2006
8. Mizrahi K, Askenasy N. Physiological functions of TNF family receptor/ligand interactions in hematopoiesis and transplantation. Blood (2014) 124:176–83. doi:10.1182/blood-2014-03-559641
9. Askenasy N. Interferon and tumor necrosis factor as humoral mechanisms coupling hematopoietic activity to inflammation and injury. Blood Rev (2015) 29:11–5. doi:10.1016/j.blre.2014.09.002
10. Askenasy N, Yolcu ES, Yaniv I, Shirwan H. Induction of tolerance using Fas ligand: a double-edged immunomodulator. Blood (2005) 105:1396–404. doi:10.1182/blood-2004-06-2364
11. Kreuwel HT, Morgan DJ, Krahl T, Ko A, Sarvetnick N, Sherman LA. Comparing the relative role of perforin/granzyme versus Fas/Fas ligand cytotoxic pathways in CD8+ T cell-mediated insulin-dependent diabetes mellitus. J Immunol (1999) 163:4335–41.
12. Thomas HE, Trapani JA, Kay TW. The role of perforin and granzymes in diabetes. Cell Death Differ (2010) 17:577–85. doi:10.1038/cdd.2009.165
13. Nagata S, Suda T. Fas and Fas ligand: lpr and gld mutations. Immunol Today (1995) 16:39–43. doi:10.1016/0167-5699(95)80069-7
14. Schumann DM, Maedler K, Franklin I, Konrad D, Størling J, Böni-Schnetzler M, et al. The Fas pathway is involved in pancreatic beta cell secretory function. Proc Natl Acad Sci U S A (2007) 104:2861–6. doi:10.1073/pnas.0611487104
15. Choi D, Radziszewska A, Schroer SA, Liadis N, Liu Y, Zhang Y, et al. Deletion of Fas in the pancreatic beta-cells leads to enhanced insulin secretion. Am J Physiol Endocrinol Metab (2009) 297:E1304–12. doi:10.1152/ajpendo.00217.2009
16. Maedler K, Fontana A, Ris F, Sergeev P, Toso C, Oberholzer J, et al. FLIP switches Fas-mediated glucose signaling in human pancreatic beta cells from apoptosis to cell replication. Proc Natl Acad Sci U S A (2002) 99:8236–41. doi:10.1073/pnas.122686299
17. Maedler K, Schumann DM, Sauter N, Ellingsgaard H, Bosco D, Baertschiger R, et al. Low concentration of interleukin-1beta induces FLICE-inhibitory protein-mediated beta-cell proliferation in human pancreatic islets. Diabetes (2006) 55:2713–22. doi:10.2337/db05-1430
18. Green DR, Ferguson TA. The role of Fas ligand in immune privilege. Nat Rev Mol Cell Biol (2001) 2:917–24. doi:10.1038/35103104
19. Signore A, Annovazzi A, Procaccini E, Beales PE, Spencer J, Testi R, et al. CD95 and CD95-ligand expression in endocrine pancreas of NOD, NOR and BALB/c mice. Diabetologia (1997) 40:1476–9. doi:10.1007/s001250050852
20. Reddy S, Ross JM. Fas and Fas ligand immunoexpression in pancreatic islets of NOD mice during spontaneous and cyclophosphamide-accelerated diabetes. Ann N Y Acad Sci (2003) 1005:166–9. doi:10.1196/annals.1288.019
21. Stassi G, De Maria R, Trucco G, Rudert W, Testi R, Galluzzo A, et al. Nitric oxide primes pancreatic beta cells for Fas-mediated destruction in insulin-dependent diabetes mellitus. J Exp Med (1997) 186:1193–200. doi:10.1084/jem.186.8.1193
22. Loweth AC, Williams GT, James RF, Scarpello JH, Morgan NG. Human islets of Langerhans express Fas ligand and undergo apoptosis in response to interleukin-1beta and Fas ligation. Diabetes (1998) 47:727–32. doi:10.2337/diabetes.47.5.727
23. Signore A, Annovazzi A, Gradini R, Liddi R, Ruberti G. Fas and Fas ligand-mediated apoptosis and its role in autoimmune diabetes. Diabetes Metab Rev (1998) 14:197–206. doi:10.1002/(SICI)1099-0895(1998090)14:3<197::AID-DMR213>3.0.CO;2-G
24. Savinov AY, Wong FS, Stonebraker AC, Chervonsky AV. Presentation of antigen by endothelial cells and chemoattraction are required for homing of insulin-specific CD8+ T cells. J Exp Med (2003) 197:643–56. doi:10.1084/jem.20021378
25. Sata M, Suhara T, Walsh K. Vascular endothelial cells and smooth muscle cells differ in expression of Fas and Fas ligand and in sensitivity to Fas ligand-induced cell death: implications for vascular disease and therapy. Arterioscler Thromb Vasc Biol (2000) 20:309–16. doi:10.1161/01.ATV.20.2.309
26. Tran TH, Grey S, Anrather J, Steinhauslin F, Bach FH, Winkler H. Regulated and endothelial cell-specific expression of Fas ligand: an in vitro model for a strategy aiming at inhibiting xenograft rejection. Transplantation (1998) 66:1126–31. doi:10.1097/00007890-199811150-00002
27. Pearl-Yafe M, Yolcu ES, Yaniv I, Stein J, Shirwan H, Askenasy N. The dual role of Fas-ligand as an injury effector and defense strategy in diabetes and islet transplantation. Bioessays (2006) 28:211–22. doi:10.1002/bies.20356
28. Sainio-Pollanen S, Liukas A, Pollanen P, Simell O. The role of CD8+ cells, cell degeneration, and Fas ligand in insulitis after intraperitoneal transfer of NOD splenocytes. Pancreas (1999) 18:282–93. doi:10.1097/00006676-199904000-00010
29. Yamada K, Takane-Gyotoku N, Yuan X, Ichikawa F, Inada C, Nonaka K. Mouse islet cell lysis mediated by interleukin-1-induced Fas. Diabetologia (1996) 39:1306–12. doi:10.1007/s001250050574
30. Kaminitz A, Yolcu ES, Stein J, Yaniv I, Shirwan H, Askenasy N. Killer Treg restore immune homeostasis and suppress autoimmune diabetes in prediabetic NOD mice. J Autoimmun (2011) 37:39–47. doi:10.1016/j.jaut.2011.03.003
31. Zumsteg U, Frigerio S, Hollander GA. Nitric oxide production and Fas surface expression mediate two independent pathways of cytokine-induced murine beta-cell damage. Diabetes (2000) 49:39–47. doi:10.2337/diabetes.49.1.39
32. Suarez-Pinzon W, Sorensen O, Bleackley RC, Elliott JF, Rajotte RV, Rabinovitch A. Beta-cell destruction in NOD mice correlates with Fas (CD95) expression on beta-cells and proinflammatory cytokine expression in islets. Diabetes (1999) 48:21–8. doi:10.2337/diabetes.48.1.21
33. Amrani A, Verdaguer J, Thiessen S, Bou S, Santamaria P. IL-1alpha, IL-1beta, and IFN-gamma mark beta cells for Fas-dependent destruction by diabetogenic CD4(+) T lymphocytes. J Clin Invest (2000) 105:459–68. doi:10.1172/JCI8185
34. Moriwaki M, Itoh N, Miyagawa J, Yamamoto K, Imagawa A, Yamagata K, et al. Fas and Fas ligand expression in inflamed islets in pancreas sections of patients with recent-onset Type I diabetes mellitus. Diabetologia (1999) 42:1332–40. doi:10.1007/s001250051446
35. Darwiche R, Chong MM, Santamaria P, Thomas HE, Kay TW. Fas is detectable on beta cells in accelerated, but not spontaneous, diabetes in nonobese diabetic mice. J Immunol (2003) 170:6292–7. doi:10.4049/jimmunol.170.12.6292
36. Darville MI, Eizirik DL. Cytokine induction of Fas gene expression in insulin-producing cells requires the transcription factors NF-kappaB and C/EBP. Diabetes (2001) 50:1741–8. doi:10.2337/diabetes.50.8.1741
37. Rabinovitch A. Immunoregulation by cytokines in autoimmune diabetes. Adv Exp Med Biol (2003) 520:159–93.
38. von Herrath M, Holz A. Pathological changes in the islet milieu precede infiltration of islets and destruction of beta-cells by autoreactive lymphocytes in a transgenic model of virus-induced IDDM. J Autoimmun (1997) 10:231–8. doi:10.1006/jaut.1997.0131
39. Aspord C, Rome S, Thivolet C. Early events in islets and pancreatic lymph nodes in autoimmune diabetes. J Autoimmun (2004) 23:27–35. doi:10.1016/j.jaut.2004.03.007
40. Kwon G, Corbett JA, Rodi CP, Sullivan P, McDaniel ML. Interleukin-1 beta-induced nitric oxide synthase expression by rat pancreatic beta-cells: evidence for the involvement of nuclear factor kappa B in the signaling mechanism. Endocrinology (1995) 136:4790–5. doi:10.1210/endo.136.11.7588208
41. Kay TW, Darwiche R, Irawaty W, Chong MM, Pennington HL, Thomas HE. The role of cytokines as effectors of tissue destruction in autoimmunity. Adv Exp Med Biol (2003) 520:73–86. doi:10.1007/978-1-4615-0171-8_5
42. Maedler K, Spinas GA, Lehmann R, Sergeev P, Weber M, Fontana A, et al. Glucose induces beta-cell apoptosis via upregulation of the Fas receptor in human islets. Diabetes (2001) 50:1683–90. doi:10.2337/diabetes.50.8.1683
43. Elouil H, Cardozo AK, Eizirik DL, Henquin JC, Jonas JC. High glucose and hydrogen peroxide increase c-Myc and haeme-oxygenase 1 mRNA levels in rat pancreatic islets without activating NFkB. Diabetologia (2005) 48:496–505. doi:10.1007/s00125-005-0043-0
44. Wen L, Green EA, Stratmann T, Panosa A, Gomis R, Eynon EE, et al. In vivo diabetogenic action of CD4+ T lymphocytes requires Fas expression and is independent of IL-1 and IL-18. Eur J Immunol (2011) 41:1344–51. doi:10.1002/eji.201041216
45. Hoorens A, Van de Casteele M, Klöppel G, Pipeleers D. Glucose promotes survival of rat pancreatic beta cells by activating synthesis of proteins which suppress a constitutive apoptotic program. J Clin Invest (1996) 98:1568–74. doi:10.1172/JCI118950
46. Laybutt DR, Glandt M, Xu G, Ahn YB, Trivedi N, Bonner-Weir S, et al. Critical reduction in beta-cell mass results in two distinct outcomes over time. Adaptation with impaired glucose tolerance or decompensated diabetes. J Biol Chem (2003) 278:2997–3005. doi:10.1074/jbc.M210581200
47. Kaminitz A, Stein J, Yaniv I, Askenasy N. The vicious cycle of apoptotic beta-cell death in type 1 diabetes. Immunol Cell Biol (2007) 85:582–9. doi:10.1038/sj.icb.7100093
48. Calderon B, Carrero JA, Miller MJ, Unanue ER. Entry of diabetogenic T cells into islets induces changes that lead to amplification of the cellular response. Proc Natl Acad Sci U S A (2011) 108:1567–72. doi:10.1073/pnas.1018975108
49. Kornete M, Beauchemin H, Polychronakos C, Piccirillo CA. Pancreatic islet cell phenotype and endocrine function throughout diabetes development in non-obese diabetic mice. Autoimmunity (2013) 46:259–68. doi:10.3109/08916934.2012.752462
50. Chervonsky AV, Wang Y, Wong FS, Visintin I, Flavell RA, Janeway CA, et al. The role of Fas in autoimmune diabetes. Cell (1997) 89:17–24. doi:10.1016/S0092-8674(00)80178-6
51. Itoh N, Imagawa A, Hanafusa T, Waguri M, Yamamoto K, Iwahashi H, et al. Requirement of Fas for the development of autoimmune diabetes in nonobese diabetic mice. J Exp Med (1997) 186:613–8. doi:10.1084/jem.186.4.613
52. Su X, Hu Q, Kristan JM, Costa C, Shen Y, Gero D, et al. Significant role for Fas in the pathogenesis of autoimmune diabetes. J Immunol (2000) 164:2523–32. doi:10.4049/jimmunol.164.5.2523
53. Mohamood AS, Guler ML, Xiao Z, Zheng D, Hess A, Wang Y, et al. Protection from autoimmune diabetes and T-cell lymphoproliferation induced by FasL mutation are differentially regulated and can be uncoupled pharmacologically. Am J Pathol (2007) 171:97–106. doi:10.2353/ajpath.2007.070148
54. Sia C, Hänninen A. Apoptosis in autoimmune diabetes: the fate of beta-cells in the cleft between life and death. Rev Diabet Stud (2006) 3:39–46. doi:10.1900/RDS.2006.3.39
55. Baumann B, Salem HH, Boehm BO. Anti-inflammatory therapy in type 1 diabetes. Curr Diab Rep (2012) 12:499–509. doi:10.1007/s11892-012-0299-y
56. Nepom GT, Ehlers M, Mandrup-Poulsen T. Anti-cytokine therapies in T1D: concepts and strategies. Clin Immunol (2013) 149:279–85. doi:10.1016/j.clim.2013.02.003
57. Sedger LM, McDermott MF. TNF and TNF-receptors: from mediators of cell death and inflammation to therapeutic giants – past, present and future. Cytokine Growth Factor Rev (2014) 25:453–72. doi:10.1016/j.cytogfr.2014.07.016
58. Yang X, Tisch R, Singer SM, Cao ZA, Liblau RS, Schreiber RD, et al. Effect of tumor necrosis factor {alpha} on insulin-dependent diabetes mellitus in NOD mice. I. The early development of autoimmunity and the diabetogenic process. J Exp Med (2004) 180:995–1004. doi:10.1084/jem.180.3.995
59. Nakayama M, Nagata M, Yasuda H, Arisawa K, Kotani R, Yamada K, et al. Fas/Fas ligand interactions play an essential role in the initiation of murine autoimmune diabetes. Diabetes (2002) 51:1391–7. doi:10.2337/diabetes.51.5.1391
60. Hamad AR, Arcara K, Uddin S, Donner T. The potential of Fas ligand (apoptosis-inducing molecule) as an unconventional therapeutic target in type 1 diabetes. Front Immunol (2012) 3:196. doi:10.3389/fimmu.2012.00196
61. Vence L, Benoist C, Mathis D. Fas deficiency prevents type 1 diabetes by inducing hyporesponsiveness in islet β-cell reactive T-cells. Diabetes (2004) 53:2797–803. doi:10.2337/diabetes.53.11.2797
62. Kim S, Kim KA, Hwang DY, Lee TH, Kayagaki N, Yagita H, et al. Inhibition of autoimmune diabetes by Fas ligand: the paradox is solved. J Immunol (2000) 164:2931–6. doi:10.4049/jimmunol.164.6.2931
63. Xiao Z, Mohamood AS, Uddin S, Gutfreund R, Nakata C, Marshall A, et al. Inhibition of Fas ligand in NOD mice unmasks a protective role for IL-10 against insulitis development. Am J Pathol (2011) 179:725–32. doi:10.1016/j.ajpath.2011.04.016
64. Mathis D, Vence L, Benoist C. Cell death during progression to diabetes. Nature (2001) 414:792–8. doi:10.1038/414792a
65. Lee MS, Chang I, Kim S. Death effectors of beta-cell apoptosis in type 1 diabetes. Mol Genet Metab (2004) 83:82–92. doi:10.1016/j.ymgme.2004.08.002
66. Pearl-Yafe M, Kaminitz A, Yolcu ES, Yaniv I, Stein J, Askenasy N. Pancreatic islets under attack: cellular and molecular effectors. Curr Pharm Des (2007) 13:749–60. doi:10.2174/138161207780249155
67. Thomas HE, McKenzie MD, Angstetra E, Campbell PD, Kay TW. Beta cell apoptosis in diabetes. Apoptosis (2009) 14:1389–404. doi:10.1007/s10495-009-0339-5
68. Kaminitz A, Ash S, Askenasy N. Neutralization versus reinforcement of proinflammatory cytokines to arrest autoimmunity in type 1 diabetes. Clinic Rev Allerg Immunol (2016). doi:10.1007/s12016-016-8587-y
69. Mueller C, Held W, Imboden MA, Carnaud C. Accelerated-cell destruction in adoptively transferred autoimmune diabetes correlates with an increased expression of the genes coding for TNF-a and granzyme A in the intra-islet infiltrates. Diabetes (1995) 44:112–7. doi:10.2337/diabetes.44.1.112
70. Pakala SV, Chivetta M, Kelly CB, Katz JD. In autoimmune diabetes the transition from benign to pernicious insulitis requires an islet cell response to tumor necrosis factor a. J Exp Med (1999) 189:1053–62. doi:10.1084/jem.189.7.1053
71. Walter U, Franzke A, Sarukhan A, Zober C, von Boehmer H, Buer J, et al. Monitoring gene expression of TNFR family members by beta-cells during development of autoimmune diabetes. Eur J Immunol (2000) 30:1224–32. doi:10.1002/1521-4141(200004)30:4<1224::AID-IMMU1224>3.0.CO;2-B
72. Kagi D, Ho A, Odermatt B, Zakarian A, Ohashi PS, Mak TW. TNF receptor 1-dependent beta cell toxicity as an effector pathway in autoimmune diabetes. J Immunol (1999) 162:4598–605.
73. Campbell IL, Iscaro A, Harrison LC. IFN-gamma and tumor necrosis factor-alpha. Cytotoxicity to murine islets of Langerhans. J Immunol (1988) 141:2325–9.
74. Kaneto H, Fujii J, Seo HG, Suzuki K, Matsuoka T, Nakamura M, et al. Apoptotic cell death triggered by nitric oxide in pancreatic beta-cells. Diabetes (1995) 44:733–8. doi:10.2337/diabetes.44.7.733
75. Angstetra E, Graham KL, Emmett S, Dudek NL, Darwiche R, Ayala-Perez R, et al. In vivo effects of cytokines on pancreatic beta-cells in models of type I diabetes dependent on CD4(+) T lymphocytes. Immunol Cell Biol (2009) 87:178–85. doi:10.1038/icb.2008.81
76. Kagi D, Odermatt B, Seiler P, Zinkernagel RM, Mak TW, Hengartner H. Reduced incidence and delayed onset of diabetes in perforin-deficient nonobese diabetic mice. J Exp Med (1997) 186:989–97. doi:10.1084/jem.186.7.989
77. Amrani A, Verdaguer J, Anderson B, Utsugi T, Bou S, Santamaria P. Perforin-independent beta-cell destruction by diabetogenic CD8(+) T lymphocytes in transgenic nonobese diabetic mice. J Clin Invest (1999) 103:1201–9. doi:10.1172/JCI6266
78. Apostolou I, Hao Z, Rajewsky K, von Boehmer H. Effective destruction of Fas-deficient insulin-producing beta cells in type 1 diabetes. J Exp Med (2003) 198:1103–6. doi:10.1084/jem.20030698
79. McKenzie MD, Dudek NL, Mariana L, Chong MM, Trapani JA, Kay TW, et al. Perforin and Fas induced by IFNgamma and TNFalpha mediate beta cell death by OT-I CTL. Int Immunol (2006) 18:837–46. doi:10.1093/intimm/dxl020
80. Kobayashi M, Kaneko-Koike C, Abiru N, Uchida T, Akazawa S, Nakamura K, et al. Genetic deletion of granzyme B does not confer resistance to the development of spontaneous diabetes in non-obese diabetic mice. Clin Exp Immunol (2013) 173:411–8. doi:10.1111/cei.12134
81. Sleater M, Diamond AS, Gill RG. Islet allograft rejection by contact-dependent CD8+ T cells: perforin and FasL play alternate but obligatory roles. Am J Transplant (2007) 7:1927–33. doi:10.1111/j.1600-6143.2007.01889.x
82. Kim YH, Kim S, Kim KA, Yagita H, Kayagaki N, Kim KW, et al. Apoptosis of pancreatic beta-cells detected in accelerated diabetes of NOD mice: no role of Fas-Fas ligand interaction in autoimmune diabetes. Eur J Immunol (1999) 29:455–65. doi:10.1002/(SICI)1521-4141(199902)29:02<455::AID-IMMU455>3.0.CO;2-A
83. Thomas HE, Darwiche R, Corbett JA, Kay TW. Evidence that beta cell death in the nonobese diabetic mouse is Fas independent. J Immunol (1999) 163:1562–9.
84. Varanasi V, Avanesyan L, Schumann DM, Chervonsky AV. Cytotoxic mechanisms employed by mouse T cells to destroy pancreatic β-cells. Diabetes (2012) 61:2862–70. doi:10.2337/db11-1784
85. Rabinovitch A, Suarez-Pinzon WL. Role of cytokines in the pathogenesis of autoimmune diabetes mellitus. Rev Endocr Metab Disord (2003) 4:291–9. doi:10.1023/A:1025160614313
86. Dharnidharka VR, Van Patten Y, Bahjat FR, Clare-Salzler M. Fas stimulation results in selective islet infiltrate apoptosis in situ and reversal of diabetes. Ann N Y Acad Sci (2002) 958:160–2. doi:10.1111/j.1749-6632.2002.tb02960.x
87. Higuchi Y, Herrera P, Muniesa P, Huarte J, Belin D, Ohashi P, et al. Expression of a tumor necrosis factor alpha transgene in murine pancreatic beta cells results in severe and permanent insulitis without evolution towards diabetes. J Exp Med (1992) 176:1719–31. doi:10.1084/jem.176.6.1719
88. Picarella DE, Kratz A, Li CB, Ruddle NH, Flavell RA. Transgenic tumor necrosis factor (TNF)-alpha production in pancreatic islets leads to insulitis, not diabetes. Distinct patterns of inflammation in TNF-alpha and TNF-beta transgenic mice. J Immunol (1993) 150:4136–50.
89. Grewal IS, Grewal KD, Wong FS, Picarella DE, Janeway CA, Flavell RA. Local expression of transgene encoded TNF alpha in islets prevents autoimmune diabetes in nonobese diabetic (NOD) mice by preventing the development of auto-reactive islet-specific T cells. J Exp Med (1996) 184:1963–74. doi:10.1084/jem.184.5.1963
90. Christen U, Von Herrath MG. Apoptosis of autoreactive CD8 lymphocytes as a potential mechanism for the abrogation of type 1 diabetes by islet-specific TNF-alpha expression at a time when the autoimmune process is already ongoing. Ann N Y Acad Sci (2002) 958:166–9. doi:10.1111/j.1749-6632.2002.tb02962.x
91. Moritani M, Yoshimoto K, Wong SF, Tanaka C, Yamaoka T, Sano T, et al. Abrogation of autoimmune diabetes in nonobese diabetic mice and protection against effector lymphocytes by transgenic paracrine TGF-beta1. J Clin Invest (1998) 102:499–506. doi:10.1172/JCI2992
92. Grewal IS, Grewal KD, Wong FS, Wang H, Picarella DE, Janeway CA, et al. Expression of transgene encoded TGF-beta in islets prevents autoimmune diabetes in NOD mice by a local mechanism. J Autoimmun (2002) 19:9–22. doi:10.1006/jaut.2002.0599
93. Satoh J, Seino H, Abo T, Tanaka S, Shintani S, Ohta S, et al. Recombinant human tumor necrosis factor alpha suppresses autoimmune diabetes in nonobese diabetic mice. J Clin Invest (1989) 84:1345–8. doi:10.1172/JCI114304
94. Jacob CO, Aiso S, Michie SA, McDevitt HO, Acha-Orbea H. Prevention of diabetes in nonobese diabetic mice by tumor necrosis factor (TNF): similarities between TNF-alpha and interleukin 1. Proc Natl Acad Sci U S A (1990) 87:968–72. doi:10.1073/pnas.87.3.968
95. Campbell IL, Oxbrow L, Harrison LC. Reduction in insulitis following administration of IFN-gamma and TNF-alpha in the NOD mouse. J Autoimmun (1991) 4:249–62. doi:10.1016/0896-8411(91)90022-5
96. Kaminitz A, Yolcu ES, Mizrahi K, Shirwan H, Askenasy N. Killer Treg cells ameliorate inflammatory insulitis in non-obese diabetic mice through local and systemic immunomodulation. Int Immunol (2013) 25:485–94. doi:10.1093/intimm/dxt016
97. Bongartz T, Sutton AJ, Sweeting MJ, Buchan I, Matteson EL, Montori V. Anti-TNF antibody therapy in rheumatoid arthritis and the risk of serious infections and malignancies: systematic review and meta-analysis of rare harmful effects in randomized controlled trials. JAMA (2006) 295:2275–85. doi:10.1001/jama.295.19.2275
98. Wong AK, Kerkoutian S, Said J, Rashidi H, Pullarkat ST. Risk of lymphoma in patients receiving antitumor necrosis factor therapy: a meta-analysis of published randomized controlled studies. Clin Rheumatol (2012) 31:631–6. doi:10.1007/s10067-011-1895-y
99. Liu Y, Fan W, Chen H, Yu MX. Risk of breast cancer and total malignancies in rheumatoid arthritis patients undergoing TNF-α antagonist therapy: a meta-analysis of randomized control trials. Asian Pac J Cancer Prev (2014) 15:3403–10. doi:10.7314/APJCP.2014.15.8.3403
100. Herrera PL, Harlan DM, Vassalli P. A mouse CD8 T cell-mediated acute autoimmune diabetes independent of the perforin and Fas cytotoxic pathways: possible role of membrane TNF. Proc Natl Acad Sci U S A (2000) 97:279–84. doi:10.1073/pnas.97.1.279
Keywords: type 1 diabetes, β-cell inflammation, activation-induced cell death, Fas, Fas-ligand
Citation: Yolcu ES, Shirwan H and Askenasy N (2017) Fas/Fas-Ligand Interaction As a Mechanism of Immune Homeostasis and β-Cell Cytotoxicity: Enforcement Rather Than Neutralization for Treatment of Type 1 Diabetes. Front. Immunol. 8:342. doi: 10.3389/fimmu.2017.00342
Received: 12 May 2016; Accepted: 09 March 2017;
Published: 27 March 2017
Edited by:
Lucienne Chatenoud, Université Paris Descartes, FranceReviewed by:
Alf Hamann, Deutsches Rheuma-Forschungszentrum (DRFZ), GermanyCopyright: © 2017 Yolcu, Shirwan and Askenasy. This is an open-access article distributed under the terms of the Creative Commons Attribution License (CC BY). The use, distribution or reproduction in other forums is permitted, provided the original author(s) or licensor are credited and that the original publication in this journal is cited, in accordance with accepted academic practice. No use, distribution or reproduction is permitted which does not comply with these terms.
*Correspondence: Nadir Askenasy, YW5hZGlyQDAxMi5uZXQuaWw=
Disclaimer: All claims expressed in this article are solely those of the authors and do not necessarily represent those of their affiliated organizations, or those of the publisher, the editors and the reviewers. Any product that may be evaluated in this article or claim that may be made by its manufacturer is not guaranteed or endorsed by the publisher.
Research integrity at Frontiers
Learn more about the work of our research integrity team to safeguard the quality of each article we publish.