- 1Programa de Oncovirologia, Instituto Nacional de Cancer, Rio de Janeiro, Brazil
- 2Department of Tropical Medicine, Hawaii Center for AIDS, John A. Burns School of Medicine, University of Hawaii, Honolulu, HI, USA
- 3Blood Systems Research Institute, San Francisco, CA, USA
- 4University of California, San Francisco, CA, USA
Now in its fourth decade, the burden of HIV disease still persists, despite significant milestone achievements in HIV prevention, diagnosis, treatment, care, and support. Even with long-term use of currently available antiretroviral therapies (ARTs), eradication of HIV remains elusive and now poses a unique set of challenges for the HIV-infected individual. The occurrence of HIV-associated non-AIDS-related comorbidities outside the scope of AIDS-defining illnesses, in particular non-AIDS-defining cancers, is much greater than the age-matched uninfected population. The underlying mechanism is now recognized in part to be related to the immune dysregulated and inflammatory status characteristic of HIV infection that persists despite ART. Natural killer (NK) cells are multifunctional effector immune cells that play a critical role in shaping the innate immune responses to viral infections and cancer. NK cells can modulate the adaptive immune response via their role in dendritic cell (DC) maturation, removal of immature tolerogenic DCs, and their ability to produce immunoregulatory cytokines. NK cells are therefore poised as attractive therapeutic targets that can be harnessed to control or clear both HIV and HIV-associated malignancies. To date, features of the tumor microenvironment and the evolution of NK-cell function among individuals with HIV-related malignancies remain unclear and may be distinct from malignancies observed in uninfected persons. This review intends to uncouple anti-HIV and antitumor NK-cell features that can be manipulated to halt the evolution of HIV disease and HIV-associated malignancies and serve as potential preventative and curative immunotherapeutic options.
Introduction
Since the introduction of antiretroviral therapy (ART), life expectancy of people living with HIV (PLHIV) has notably improved, and the gap between the uninfected population ranging from 60 to 90%, of normal life expectancy is narrowing in regions of the world among those with access to ART (1). Before the availability of ART, immune suppression-related complications represented the predominant cause of mortality among HIV-infected individuals. Incidence rates of non-Hodgkin lymphoma (NHL) and Kaposi sarcoma (KS) were more than 100 times higher in the pre-ART era and were classified, together with cervical cancer, as AIDS-defining cancers (ADCs) (2). Overtime, as ART became the standard of care, prolonged use has lead to a remarkable improvement in immune status, dramatically reducing ADC rates (ratio of ART to no-ART) by 0.61 per year (3).
In the United States, despite the sharp decline in the incidence of ADCs, increased risk of developing specific types of NHL such as Burkitt lymphoma and classical Hodgkin lymphoma (HL) has evolved (4, 5). A meta-analysis of standardized incidence ratios from 18 studies showed that infection-related cancers such as anal, liver and HL, as well as smoking-related cancers such as lung, kidney, and oral cancers, had an increased incidence among the HIV population despite being on ART, with lymphomas being the most frequent type of cancer observed (6). A separate comprehensive epidemiological study showed that the PLHIV also had significantly higher rates of these now termed “non-AIDS defining cancers” (NADCs) when compared to matched HIV-negative individuals, suggesting the likelihood of other etiologies besides an increase in life expectancy as predisposing the HIV population to develop these types of cancers more frequently (7). In fact, NADCs have now become one of the leading causes of mortality among HIV-infected individuals (8, 9). Surprisingly, however, the incidences of breast and prostate cancers have significantly declined in HIV-infected persons, suggesting that HIV infection, ART, or other viral–host interactions have differential impacts on cancer risk in this population (10, 11). The increased incidence of NADC despite viral suppression and CD4 T-cell recovery in the era of ART raises important key mechanistic questions in the oncogenesis of NADC. The direct oncogenic effect of HIV, HIV-induced immunodeficiency, and chronic inflammation, as well as ART toxicities are some of the plausible mechanisms that are being investigated (12). Complete immune recovery after prolonged ART is variable and underscores that harnessing specific components of the host immune response may play a vital role in preventing NADC.
Chronic immune activation and immune senescence contribute to immune dysfunction in chronic HIV infection and partially persist even after CD4 T-cell count recovery and viral suppression by ART (13). Such processes lead to immune exhaustion/senescence, thereby facilitating reactivation of other latent viral infections, such as Epstein–Barr virus (EBV). Besides HIV, all ADCs and the majority of NADCs appear to be associated with several chronic viral infections (12), justifying a new way to categorize HIV malignancies into infection-related and non-infectious-related cancers. KS is intimately associated with HHV-8 infection (14), and cervical cancer is almost always caused by HPV infection (15). B-cell lymphoproliferative disorders are frequently associated with EBV infection, and such association is even more common in HIV-infected individuals, ranging from 60 to 100% (5, 16). Viral co-infections are present in NADC. The incidence of hepatocellular carcinoma has progressively increased among HIV-infected persons in the last decade (17). Merkel cell carcinoma, recently reported to be associated with Merkel cell virus, has a 20-fold increased risk among HIV-infected individuals (18). The potential direct effects of HIV in modulating oncogenes are under investigation, but how HIV impacts the oncogenic potential of other chronic viral infections is unclear (19–21). Persistent immune alterations may play a critical role in the oncogenic process in this population and deserve special attention, particularly in the context of co-infections.
Natural Killer (NK) Cells: A Critical Immune Player in Antitumor and Anti-HIV Immunity
Since the discovery of NK cells 40 years ago, a plethora of research has uncovered their phenotypic and functional capacity against virally infected and tumor cells (22–25). NK cells are CD3− multifunctional effector lymphocytes defined based on levels of CD56 and CD16 expression (26), the majority (>90% of NK cells) in the peripheral blood being CD56dim and predominantly cytotoxic upon activation, thereby releasing pro-apoptotic cytoplasmic granules composed of granzymes and perforins. CD56dim NK cells can also induce cytolysis via induction of Fas/FasL-dependent or TRAIL-dependent apoptotic signals. In addition, a minority of NK cells express the FcγRIIIA receptor (CD16) that binds to the constant (Fc) domain of IgG antibodies that can bind to viral antigens expressed on the surface of infected cells. This antibody conjugation of NK-cell and antibody-coated target cell, strongly mediating NK-cell activation, is known as antibody-dependent cell-mediated cytotoxicity (ADCC) (27). A distinct subset of CD56bright cytokine-producing NK cells with a limited cytotoxic capacity is more abundantly present in lymph nodes (28). By producing IFN-γ, TNF-α, IL-10, and chemokines, this NK subset predominantly modulates other subsets of lymphocytes, thereby regulating dendritic cell maturation, differentiation of helper T cells, and B- and T-cell-specific immune responses (29, 30).
To understand the NK-cell effector functions, it is paramount to take into consideration the balance between activating and inhibitory signals (31) that drive NK-cell cytotoxicity. NK-cell activation relies on stimulatory signals capable of overcoming the steady inhibitory state that is maintained by signaling through inhibitory receptors. Self-recognition of MHC-I proteins through C-type lectin receptor NKG2A and inhibitory killer cell immunoglobulin-like receptors (KIRs) represent the physiological interaction between NK and target cells. The absence of recognition of “self” by inhibitory receptors characterizes the “missing-self” phenomenon and lowers the activating threshold. NK cells become more susceptible to activation, especially if activating molecules are expressed in infected or transformed target cells and recognized by activating receptors, characterizing the altered-self phenomenon. Activating C-type lectin receptor NKG2D recognizes the altered self-state of infected or transformed cells and triggers NK-cell cytolytic activity. Other surface molecules, such as natural cytotoxic receptors Nkp30, Nkp44, and Nkp46, and activating KIRs also contribute to NK-cell activation process and are critical to determine whether NK cells will be activated to target infected or transformed cells (27, 31).
Both HIV infection and oncogenesis lead to a downregulation of surface MHC-I expression as a way to avoid T-cell recognition but in turn renders target cells more susceptible to NK-cell-mediated cytolysis. However, HIV has developed immune evasion mechanisms via the viral protein Nef, thereby leading to preferential downregulation of HLA-A and -B, and preserving expression of HLA-C and -E (32). Therefore, HIV prevents NK activation as well as CTL recognition of infected cells. Besides interfering with self-recognition, HIV infection and cancer can induce expression of stress signaling molecules, in particular MHC class I polypeptide-related sequence A/B (MICA/MICB). More importantly, HIV leads to persistent activation and consequently T cell and NK-cell immune exhaustion. Despite viral suppression and normal CD4 T-cell counts in the majority of HIV-infected persons on ART, NK-cell phenotype and functionality are not fully restored, suggesting that these individuals may be more susceptible to long-term comorbidities associated with immune dysfunction, such as HIV-related malignancies (33).
The Interplay between the Tumor Microenvironment and NK-Cell Immunity
The process by which the immune system can promote or suppress tumor growth and development is based on animal models and data from cancer patients and has evolved to define the concept of cancer immunoediting (34). Tumor immunoediting is comprised of three phases: elimination, equilibrium, and escape. The elimination phase is when immune cells target cancer cells that succeeded in overcoming intrinsic tumor suppressor mechanisms. If tumor elimination is only partially achieved, a state of equilibrium between malignant cells and the immune system ensues. Tumor cells can become dormant or accumulate mutations, while the immune system continues to exert selective pressure, thereby controlling tumor progress temporarily or eventually eliminating the cancer cells. If elimination does not occur, tumor cell variants resistant to the existent immune response eventually give rise to tumor progression, thereby initiating the escape phase and characterizing failure of tumor immune control. The contribution of NK cells in cancer immunoediting and clinical outcomes is now being appreciated (35).
Natural killer cells have proved to be critical for the eradication and inhibition of metastasis of cancer cells in vivo (36). Perforin protein (pfp) and/or IFN-γ knockout (KO) mice predominately develop B-cell lymphomas, especially after 1 year of age (older animals) with a combination of pfp and IFN-γ KO inducing an early onset of lymphoma, suggesting a synergistic immunosurveillance effect (37). Late age development of B-cell lymphoma and lung adenocarcinoma were also observed in TRAIL KO mice (38) and pfp KO mice (37), respectively. These findings support a role for NK-cell immunosurveillance of B-cell lymphomas as well as epithelial malignancies, through a combination of NK-cell-mediated cytotoxic activity, IFN-γ secretion, and TRAIL killing pathways. In humans, NK cells are particularly relevant in the prevention of tumor development. An 11-year follow-up of the general population for cancer incidence showed an association between reduced NK-cell cytotoxicity and increased risk of cancer (39). It has been postulated that NK cells are critical to the prevention of cancer development (elimination and equilibrium); however, once the tumor microenvironment is established (escape), suppressor factors such as TGF-β and IL-10 are induced and negative inhibitory receptors, such as the T-cell immunoglobulin-and-mucin-domain-containing molecule-3 receptor (TIM-3) on NK cells, that maintain an NK-cell anergic state (40) are upregulated. The induced aberrant expression of HLA-G (membrane-bound and soluble) and increased shedding of MICA (sMICA) seen in tumor cells (41, 42) can further suppress NK-cell antitumor immune responses. HLA-G interaction with ILT2 and CD94/NKG2A results in the inhibition of NK-cell cytotoxicity, IFN-γ secretion, and chemotaxis (43), while sMICA–NKG2D binding impairs NK-cell tumor-specific cytotoxicity, NKG2D expression, and homeostatic maintenance (42).
As a result of these immune deregulated events, NK-cell-associated suppressor factors are currently being considered as immunotherapeutic targets. TIM-3, for instance, is an immunoregulatory checkpoint expressed by most lymphocyte subtypes with critical and complex implications in cytotoxic NK cells (44, 45). Increased TIM-3 expression on NK cells has been shown as a marker of poorer prognosis in lung adenocarcinoma and other types of cancer and correlates with reduced NK-cell cytotoxicity. Blockade of TIM-3 is capable of restoring IFN-γ secretion and cytotoxicity of NK cells in lung cancer (46). Recent studies by Fowke et al. have shown that low expression of various inhibitory molecules on NK cells were associated with HIV viral control (47). Despite the complexity of the immune suppressive strategy of the tumor microenvironment, targeting these inhibitory checkpoint receptors shows potential to restore NK-cell functionality in the control or clearance of solid tumors (48). Currently, several trials are underway assessing the impact of Tim-3 blockade in cancer patients (http://ClinicalTrials.gov: NCT02817633; NCT02608268). However, such therapeutic tools are still in their infancy in the context of HIV-associated ADC or NADCs. Given the success of immunotherapy targeting the inhibitory receptors PD-1 and CTLA-4 against several malignancies (49), evaluation of the impact of NK-cell function following immune checkpoint blockade may have relevance in the setting of HIV and may serve a dual purpose in both HIV eradication and tumor clearance. Combining immunotherapy and NK-cell-based therapies is another potential targeted strategy and warrants further investigation in individuals with HIV-associated malignancies (50–53).
NK-Cell Immune Control of HIV Infection during ART
HIV infection induces significant phenotypic changes and negatively impacts NK-cell cytotoxicity (54). Cytotoxic NK cells in aviremic HIV donors have impaired ADCC that is associated with a reduced expression of FcRIIIA, an activated phenotype represented by increased expression of CD38 and HLA-DR. Furthermore, NK cells are rarely found in lymph nodes, an important site of both HIV replication and B-cell transformation (55). Critical activating receptors important in cancer immunosurveillance such as NKp30 and NKp46 are downregulated (56) in HIV infection, and expansion of dysfunctional CD56− NK-cell subsets (57) persists even after cART.
Non-neutralizing anti-HIV-1 antibodies can mediate protection through ADCC in assays of HIV candidate vaccines in non-human primate models of HIV infection. Several studies have suggested that HIV-specific ADCC responses may be contributing to partial control of HIV viremia during acute infection. The early initial interest in the utility of NK-mediated ADCC via HIV-specific antibodies was to enhance the development of an HIV vaccine and novel therapies to suppress HIV replication. Interestingly, in the RV144 HIV vaccine Thai trial, the robust ADCC responses observed were associated with modest protective efficacy (58). It would be intriguing to determine the effects of NK-mediated ADCC in the recently launched HVTN 702 study that builds on the success of the RV144 trial in support of this correlate. In addition, recent studies suggest that cytokine stimulation can enhance direct NK cytotoxicity and NK-mediated ADCC of autologous HIV-infected CD4+ T cells (59–61). Therefore, modulating NK activity is a potential strategy to develop novel immunotherapies to prevent and possibly lead to the eradication of HIV.
Influence of NK-Cell Immunity in HIV-Associated Malignancies
It is becoming apparent that NK cells may also contribute to tumorigenesis. The potential impact of such alterations in HIV malignancies is illustrated in Figure 1. Overexpression of activation markers on NK cells and spontaneous degranulation occurring during HIV infection may directly contribute to tumor development (33). Analysis of NK cells from patients with lymphoma demonstrated decreased levels of activating receptors in those with HIV compared to uninfected patients, suggesting that these cells might be less efficient to target cancer cells (62). NK cells can also present proangiogenic activity in the tumor microenvironment in a similar way to decidual NK (dNK) cells early on in pregnancy. In fact, in non-small cell lung cancer, the majority of tumor-infiltrating NK cells have a dNK-cell phenotype: CD56bright, CD9+, perforin low, and high production of vascular endothelial growth factor (VEGF) (63). Hypoxia and TGF-β secreted by tumor cells has a known immunomodulatory impact in the tumor microenvironment and induces VEGF secretion (64). In vitro exposure to TGF-β and hypoxia led to conversion of CD56dim NK cells into dNK-like cells (65). HIV infection leads to increased levels of TGF-β by monocytes (66) and T cells (67), suggesting that TGF-β may play a more prominent role in tumorigenesis during HIV infection.
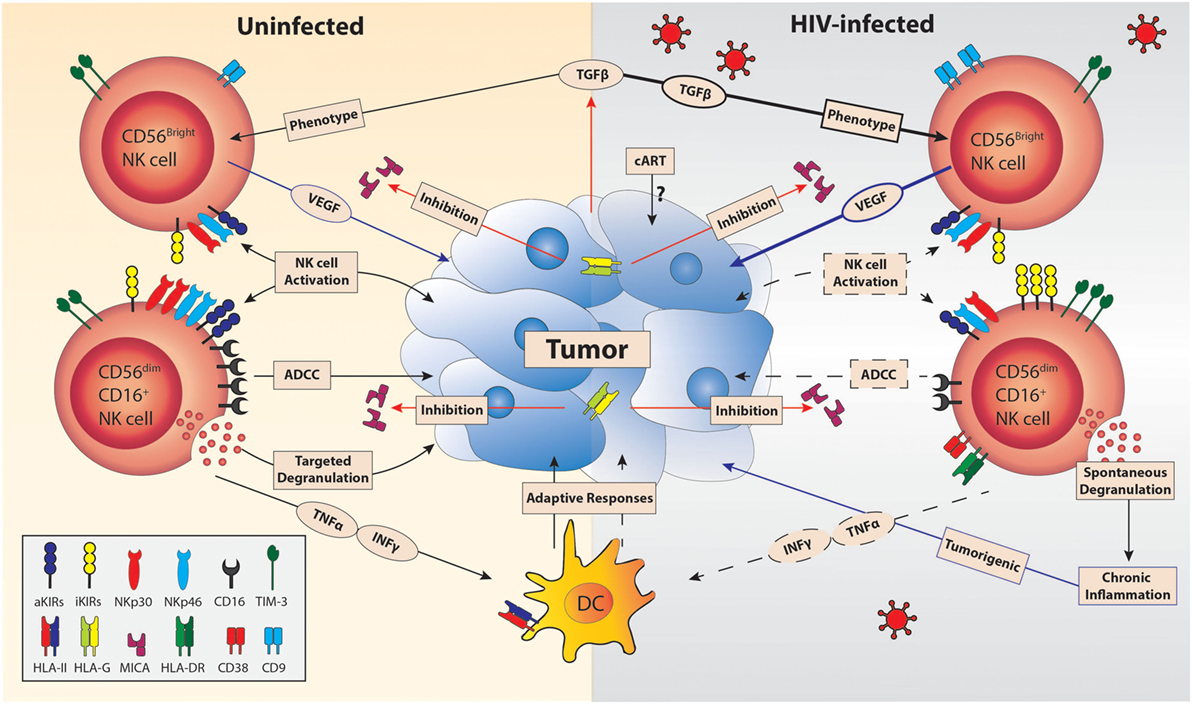
Figure 1. Compounded effect of HIV infection on natural killer (NK) cell antitumor responses. The tumor microenvironment constrains NK-cell functionality through the expression of tumor-derived transforming growth factor β (TGF-β) (68), shedding of MICA, and HLA-G (69). The limitations of antitumor mechanisms by NK cells are exacerbated in HIV infection. HIV infection reduces the surface expression of activation receptors (aKIRs, NKp30, and NKp46) and CD16 (56) while upregulating the expression of inhibitory receptors (iKIRs). The net result of the influence of HIV on NK-cell receptor expression further impairs NK-cell activation by cancer cell interaction and decreases tumor-directed antibody-dependent cell-mediated cytotoxicity (ADCC) responses (70). HIV infection decreases INF-γ and TNF-α production by NK cells despite HIV viral suppression by cART (71, 72), which will limit dendritic cell (DC) maturation and thus prevent efficient tumor-directed adaptive responses (73). The increased plasma TGF-β and loss of cell-specific degranulation of NK cells seen in HIV infection could lead to tumorigenicity via contributing to increased frequency of vascular endothelial growth factor (VEGF)-producing intratumoral NK cells (63) and occurrence of chronic inflammation, respectively (74). Furthermore, the affect of cART on tumor activity is yet to be explored. Blue lines represent responses that promote tumor growth, while responses that inhibit NK-cell function are indicated in red. Decreases are indicated by dashed lines and increases by bolded lines.
The combination of (1) reduced expression of activating receptors and increased inhibitory receptors (e.g., TIM-3), (2) reduced ADCC, (3) reduced secretion of TNF-α and IFN-γ, and (4) development of pro-cancer features such as persistent activation, spontaneous degranulation, and production of VEGF suggests that NK cells may directly be associated with the increased cancer risk in the setting of ART-treated HIV infection. It is fair to speculate that targeted immunotherapies reversing specific NK cells deficits may be relevant for many HIV-related malignancies.
NK-Cell-Based Immunotherapies Targeting HIV and HIV-Associated Malignancies
With the exception of the Berlin patient (75), and given the continued resurgence of virus in HIV-infected persons in various eradication approaches (76–78), it is clear that elimination of all latent HIV reservoirs is going to be critical to successfully achieve ART-free sustained HIV control or remission. Innovative approaches that are extrapolated from these studies and cases have lead to renewed interest in determining ways to bolster the host immune response and/or manipulate HIV target cells to render them refractory to infection. Since HIV and cancers have evolved sophisticated modalities to escape the host immune defense mechanisms, enhancing NK-cell function may serve as a promising tool as part of a multifaceted approach in the elimination of HIV as well as HIV-associated malignancies.
Recently, there has been renewed interest in harnessing HIV-specific ADCC responses as an HIV cure strategy. A monoclonal antibody (mAb) targeting the CD4-binding site on the HIV envelope spike (3BNC117) may have the potential to guide host immune effector cells to accelerate the clearance of HIV-1-infected cells by an FcyR-dependent mechanism (79, 80). In addition, Byrareddy et al. recently reported that a rhesusized mAb against α4β7 mediated sustained control of SIV infection in the absence of ART in non-human primates. This remarkable response was associated with increased cytokine-synthesizing NK cells (81). These studies, and others, highlight the potential of using mAbs through modulation of NK-cell-mediated activity as an exciting therapeutic tool to achieve sustained HIV remission and be beneficial in the context of HIV-associated tumors.
Natural killer-cell-based antitumor immunotherapeutic strategies targeting NK-cell activity have shown some promise in the oncology field (Table 1). Infusion of allogeneic or autologous NK cells has, to some degree, been successful in tumor clearance. Recently, single-chain variable fragment recombinant reagents, such as bispecific and trispecific killer cell engagers, are being considered as novel immunomodulators to enhance NK-cell function, antigen specificity, and in vivo expansion of these infused cells; however, these reagents need to be fully evaluated for clinical use (82–91). The efficiency of ADCC-mediated NK-cell responses is dependent on several factors from the mAbs themselves, NK-cell, and target cell status and also to the glycosylation state and the expression of glycosylation-specific ligands of both the NK-cell and target cells (92, 93). These features can be modified to enhance antiviral and immunomodulatory functions and/or the ability of the target cell to trigger or evade immunological recognition.
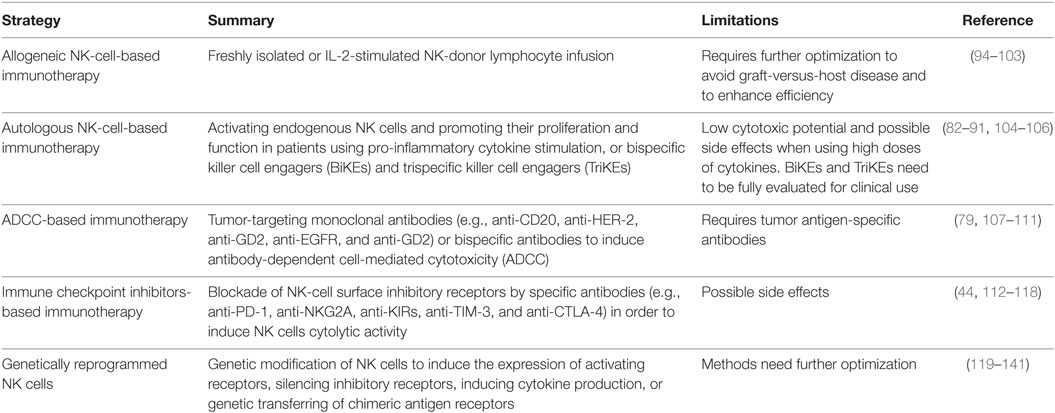
Table 1. Therapeutic strategies utilizing natural killer (NK) cells in cancer immunotherapy that should be evaluated in the eradication of both HIV and HIV-associated malignancies.
Several gene-editing technologies have been explored to genetically reprogram NK cells to optimize their persistence, expansion, migration, and cytotoxic capacity to improve the antitumor efficacy of primary human NK cells in vivo. With many challenges associated with most of these technologies, CRISPR/Cas9 nuclease system offers a new promising tool to gene-edit NK cells to improve their utility as a novel cell-based cancer immunotherapy strategy (142). Finally, latency reversal agents (LRAs) are being explored as a part of a “shock” approach to reverse cellular HIV latency and expose HIV reservoirs to immune-mediated clearance. Garrido et al. recently studied the impact of LRAs on the function of primary NK cells ex vivo and showed a heterogeneous mixed effect of different LRAs on antiviral activity, cytotoxicity, cytokine secretion, phenotype, and viability (143). Therefore, it will be important to comprehensively evaluate the impact of potential HIV curative strategies and elucidate the possible effect on NK cells against HIV-associated cancers.
Conclusion
To achieve ART-free sustained HIV remission, it is likely that a multidisciplinary approach that includes a combination of immunotherapies and novel targeted approaches that will boost both the adaptive and innate immunity will be necessary. From this review, we posit that NK cells remain a very attractive therapeutic target to develop curative interventions for both HIV and HIV-associated malignancies.
Author Contributions
LN and MA-M conceived and designed the review; LN, MA-M, and FL wrote the paper; and TP contributed to editing the review and designed and developed the figure.
Conflict of Interest Statement
The authors declare that the research was conducted in the absence of any commercial or financial relationships that could be construed as a potential conflict of interest.
Funding
MA-M receives support from the National Institutes of Health, National Institute of Allergy and Infectious Diseases (NIH/NIAID) grant number R21AI129636 and LN receives support from NIH/NIAHD grant numbers P30 AI027757 and R21 AI122393.
References
1. Wandeler G, Johnson LF, Egger M. Trends in life expectancy of HIV-positive adults on antiretroviral therapy across the globe: comparisons with general population. Curr Opin HIV AIDS (2016) 11(5):492–500. doi: 10.1097/COH.0000000000000298
2. Goedert JJ, Cote TR, Virgo P, Scoppa SM, Kingma DW, Gail MH, et al. Spectrum of AIDS-associated malignant disorders. Lancet (1998) 351(9119):1833–9. doi:10.1016/S0140-6736(97)09028-4
3. Chao C, Leyden WA, Xu L, Horberg MA, Klein D, Towner WJ, et al. Exposure to antiretroviral therapy and risk of cancer in HIV-infected persons. AIDS (2012) 26(17):2223–31. doi:10.1097/QAD.0b013e32835935b3
4. Biggar RJ, Chaturvedi AK, Goedert JJ, Engels EA; HIV/AIDS Cancer Match Study. AIDS-related cancer and severity of immunosuppression in persons with AIDS. J Natl Cancer Inst (2007) 99(12):962–72. doi:10.1093/jnci/djm010
5. Carbone A, Volpi CC, Gualeni AV, Gloghini A. Epstein-Barr virus associated lymphomas in people with HIV. Curr Opin HIV AIDS (2017) 12(1):39–46. doi:10.1097/COH.0000000000000333
6. Shiels MS, Cole SR, Kirk GD, Poole C. A meta-analysis of the incidence of non-AIDS cancers in HIV-infected individuals. J Acquir Immune Defic Syndr (2009) 52(5):611–22. doi:10.1097/QAI.0b013e3181b327ca
7. Robbins HA, Pfeiffer RM, Shiels MS, Li J, Hall HI, Engels EA. Excess cancers among HIV-infected people in the United States. J Natl Cancer Inst (2015) 107(4):dju503. doi:10.1093/jnci/dju503
8. Zucchetto A, Virdone S, Taborelli M, Grande E, Camoni L, Pappagallo M, et al. Non-AIDS-defining cancer mortality: emerging patterns in the late HAART era. J Acquir Immune Defic Syndr (2016) 73(2):190–6. doi:10.1097/QAI.0000000000001033
9. Rodger AJ, Lodwick R, Schechter M, Deeks S, Amin J, Gilson R, et al. Mortality in well controlled HIV in the continuous antiretroviral therapy arms of the SMART and ESPRIT trials compared with the general population. AIDS (2013) 27(6):973–9. doi:10.1097/QAD.0b013e32835cae9c
10. Engels EA, Biggar RJ, Hall HI, Cross H, Crutchfield A, Finch JL, et al. Cancer risk in people infected with human immunodeficiency virus in the United States. Int J Cancer (2008) 123(1):187–94. doi:10.1002/ijc.23487
11. Grulich AE, van Leeuwen MT, Falster MO, Vajdic CM. Incidence of cancers in people with HIV/AIDS compared with immunosuppressed transplant recipients: a meta-analysis. Lancet (2007) 370(9581):59–67. doi:10.1016/S0140-6736(07)61050-2
12. Borges AH, Dubrow R, Silverberg MJ. Factors contributing to risk for cancer among HIV-infected individuals, and evidence that earlier combination antiretroviral therapy will alter this risk. Curr Opin HIV AIDS (2014) 9(1):34–40. doi:10.1097/COH.0000000000000025
13. Nasi M, De Biasi S, Gibellini L, Bianchini E, Pecorini S, Bacca V, et al. Ageing and inflammation in patients with HIV infection. Clin Exp Immunol (2016) 187(1):44–52. doi:10.1111/cei.12814
14. Dittmer DP, Damania B. Kaposi sarcoma-associated herpesvirus: immunobiology, oncogenesis, and therapy. J Clin Invest (2016) 126(9):3165–75. doi:10.1172/JCI84418
15. Senapati R, Senapati NN, Dwibedi B. Molecular mechanisms of HPV mediated neoplastic progression. Infect Agent Cancer (2016) 11:59. doi:10.1186/s13027-016-0107-4
16. Carbone A, Cesarman E, Spina M, Gloghini A, Schulz TF. HIV-associated lymphomas and gamma-herpesviruses. Blood (2009) 113(6):1213–24. doi:10.1182/blood-2008-09-180315
17. Merchante N, Merino E, Lopez-Aldeguer J, Jover F, Delgado-Fernandez M, Galindo MJ, et al. Increasing incidence of hepatocellular carcinoma in HIV-infected patients in Spain. Clin Infect Dis (2013) 56(1):143–50. doi:10.1093/cid/cis777
18. Wieland U, Kreuter A. Merkel cell polyomavirus infection and Merkel cell carcinoma in HIV-positive individuals. Curr Opin Oncol (2011) 23(5):488–93. doi:10.1097/CCO.0b013e3283495a5b
19. De Paoli P, Carbone A. Microenvironmental abnormalities induced by viral cooperation: impact on lymphomagenesis. Semin Cancer Biol (2015) 34:70–80. doi:10.1016/j.semcancer.2015.03.009
20. Dolcetti R, Gloghini A, Caruso A, Carbone A. A lymphomagenic role for HIV beyond immune suppression? Blood (2016) 127(11):1403–9. doi:10.1182/blood-2015-11-681411
21. Wang L, Li G, Yao ZQ, Moorman JP, Ning S. microRNA regulation of viral immunity, latency, and carcinogenesis of selected tumor viruses and HIV. Rev Med Virol (2015) 25(5):320–41. doi:10.1002/rmv.1850
22. Herberman RB, Nunn ME, Holden HT, Lavrin DH. Natural cytotoxic reactivity of mouse lymphoid cells against syngeneic and allogeneic tumors. II. Characterization of effector cells. Int J Cancer (1975) 16(2):230–9. doi:10.1002/ijc.2910160205
23. Herberman RB, Nunn ME, Lavrin DH. Natural cytotoxic reactivity of mouse lymphoid cells against syngeneic acid allogeneic tumors. I. Distribution of reactivity and specificity. Int J Cancer (1975) 16(2):216–29. doi:10.1002/ijc.2910160205
24. Kiessling R, Klein E, Pross H, Wigzell H. “Natural” killer cells in the mouse. II. Cytotoxic cells with specificity for mouse Moloney leukemia cells. Characteristics of the killer cell. Eur J Immunol (1975) 5(2):117–21. doi:10.1002/eji.1830050208
25. Kiessling R, Klein E, Wigzell H. “Natural” killer cells in the mouse. I. Cytotoxic cells with specificity for mouse Moloney leukemia cells. Specificity and distribution according to genotype. Eur J Immunol (1975) 5(2):112–7. doi:10.1002/eji.1830050208
26. Cooper MA, Fehniger TA, Caligiuri MA. The biology of human natural killer-cell subsets. Trends Immunol (2001) 22(11):633–40. doi:10.1016/S1471-4906(01)02060-9
27. Wu J, Lanier LL. Natural killer cells and cancer. Adv Cancer Res (2003) 90:127–56. doi:10.1016/S0065-230X(03)90004-2
28. Ferlazzo G, Thomas D, Lin SL, Goodman K, Morandi B, Muller WA, et al. The abundant NK cells in human secondary lymphoid tissues require activation to express killer cell Ig-like receptors and become cytolytic. J Immunol (2004) 172(3):1455–62. doi:10.4049/jimmunol.172.3.1455
29. Martin-Fontecha A, Thomsen LL, Brett S, Gerard C, Lipp M, Lanzavecchia A, et al. Induced recruitment of NK cells to lymph nodes provides IFN-gamma for T(H)1 priming. Nat Immunol (2004) 5(12):1260–5. doi:10.1038/ni1138
30. Mocikat R, Braumuller H, Gumy A, Egeter O, Ziegler H, Reusch U, et al. Natural killer cells activated by MHC class I(low) targets prime dendritic cells to induce protective CD8 T cell responses. Immunity (2003) 19(4):561–9. doi:10.1016/S1074-7613(03)00264-4
31. Cerwenka A, Lanier LL. Natural killer cells, viruses and cancer. Nat Rev Immunol (2001) 1(1):41–9. doi:10.1038/35095564
32. Specht A, DeGottardi MQ, Schindler M, Hahn B, Evans DT, Kirchhoff F. Selective downmodulation of HLA-A and -B by Nef alleles from different groups of primate lentiviruses. Virology (2008) 373(1):229–37. doi:10.1016/j.virol.2007.11.019
33. Lichtfuss GF, Cheng WJ, Farsakoglu Y, Paukovics G, Rajasuriar R, Velayudham P, et al. Virologically suppressed HIV patients show activation of NK cells and persistent innate immune activation. J Immunol (2012) 189(3):1491–9. doi:10.4049/jimmunol.1200458
34. Dunn GP, Old LJ, Schreiber RD. The three Es of cancer immunoediting. Annu Rev Immunol (2004) 22:329–60. doi:10.1146/annurev.immunol.22.012703.104803
35. Tu MM, Mahmoud AB, Makrigiannis AP. Licensed and unlicensed NK cells: differential roles in cancer and viral control. Front Immunol (2016) 7:166. doi:10.3389/fimmu.2016.00166
36. Kim S, Iizuka K, Aguila HL, Weissman IL, Yokoyama WM. In vivo natural killer cell activities revealed by natural killer cell-deficient mice. Proc Natl Acad Sci U S A (2000) 97(6):2731–6. doi:10.1073/pnas.050588297
37. Street SE, Trapani JA, MacGregor D, Smyth MJ. Suppression of lymphoma and epithelial malignancies effected by interferon gamma. J Exp Med (2002) 196(1):129–34. doi:10.1084/jem.20020063
38. Zerafa N, Westwood JA, Cretney E, Mitchell S, Waring P, Iezzi M, et al. Cutting edge: TRAIL deficiency accelerates hematological malignancies. J Immunol (2005) 175(9):5586–90. doi:10.4049/jimmunol.175.9.5586
39. Imai K, Matsuyama S, Miyake S, Suga K, Nakachi K. Natural cytotoxic activity of peripheral-blood lymphocytes and cancer incidence: an 11-year follow-up study of a general population. Lancet (2000) 356(9244):1795–9. doi:10.1016/S0140-6736(00)03231-1
40. Fridman WH, Pages F, Sautes-Fridman C, Galon J. The immune contexture in human tumours: impact on clinical outcome. Nat Rev Cancer (2012) 12(4):298–306. doi:10.1038/nrc3245
41. Curigliano G, Criscitiello C, Gelao L, Goldhirsch A. Molecular pathways: human leukocyte antigen G (HLA-G). Clin Cancer Res (2013) 19(20):5564–71. doi:10.1158/1078-0432.CCR-12-3697
42. Wu J. NKG2D ligands in cancer immunotherapy: target or not? Austin J Clin Immunol (2014) 1(1):2.
43. Lin A, Yan WH. HLA-G expression in cancers: roles in immune evasion, metastasis and target for therapy. Mol Med (2015) 21(1):782–91. doi:10.2119/molmed.2015.00083
44. Ndhlovu LC, Lopez-Verges S, Barbour JD, Jones RB, Jha AR, Long BR, et al. Tim-3 marks human natural killer cell maturation and suppresses cell-mediated cytotoxicity. Blood (2012) 119(16):3734–43. doi:10.1182/blood-2011-11-392951
45. Han G, Chen G, Shen B, Li Y. Tim-3: an activation marker and activation limiter of innate immune cells. Front Immunol (2013) 4:449. doi:10.3389/fimmu.2013.00449
46. Xu L, Huang Y, Tan L, Yu W, Chen D, Lu C, et al. Increased Tim-3 expression in peripheral NK cells predicts a poorer prognosis and Tim-3 blockade improves NK-cell-mediated cytotoxicity in human lung adenocarcinoma. Int Immunopharmacol (2015) 29(2):635–41. doi:10.1016/j.intimp.2015.09.017
47. Taborda NA, Hernandez JC, Lajoie J, Juno JA, Kimani J, Rugeles MT, et al. Short communication: low expression of activation and inhibitory molecules on NK cells and CD4(+) T cells is associated with viral control. AIDS Res Hum Retroviruses (2015) 31(6):636–40. doi:10.1089/AID.2014.0325
48. da Silva IP, Gallois A, Jimenez-Baranda S, Khan S, Anderson AC, Kuchroo VK, et al. Reversal of NK-cell exhaustion in advanced melanoma by Tim-3 blockade. Cancer Immunol Res (2014) 2(5):410–22. doi:10.1158/2326-6066.CIR-13-0171
49. Swart M, Verbrugge I, Beltman JB. Combination approaches with immune-checkpoint blockade in cancer therapy. Front Oncol (2016) 6:233. doi:10.3389/fonc.2016.00233
50. Dahlberg CI, Sarhan D, Chrobok M, Duru AD, Alici E. Natural killer cell-based therapies targeting cancer: possible strategies to gain and sustain anti-tumor activity. Front Immunol (2015) 6:605. doi:10.3389/fimmu.2015.00605
51. Jones RB, Mueller S, O’Connor R, Rimpel K, Sloan DD, Karel D, et al. A subset of latency-reversing agents expose HIV-infected resting CD4+ T-cells to recognition by cytotoxic T-lymphocytes. PLoS Pathog (2016) 12(4):e1005545. doi:10.1371/journal.ppat.1005545
52. Rosario M, Liu B, Kong L, Collins LI, Schneider SE, Chen X, et al. The IL-15-based ALT-803 complex enhances FcgammaRIIIa-triggered NK-cell responses and in vivo clearance of B cell lymphomas. Clin Cancer Res (2016) 22(3):596–608. doi:10.1158/1078-0432.CCR-15-1419
53. Seay K, Church C, Zheng JH, Deneroff K, Ochsenbauer C, Kappes JC, et al. In vivo activation of human NK cells by treatment with an interleukin-15 superagonist potently inhibits acute in vivo HIV-1 infection in humanized mice. J Virol (2015) 89(12):6264–74. doi:10.1128/JVI.00563-15
54. Scully E, Alter G. NK cells in HIV disease. Curr HIV/AIDS Rep (2016) 13(2):85–94. doi:10.1007/s11904-016-0310-3
55. Luteijn R, Sciaranghella G, van Lunzen J, Nolting A, Dugast AS, Ghebremichael MS, et al. Early viral replication in lymph nodes provides HIV with a means by which to escape NK-cell-mediated control. Eur J Immunol (2011) 41(9):2729–40. doi:10.1002/eji.201040886
56. Zhou J, Amran FS, Kramski M, Angelovich TA, Elliott J, Hearps AC, et al. An NK-cell population lacking FcRgamma is expanded in chronically infected HIV patients. J Immunol (2015) 194(10):4688–97. doi:10.4049/jimmunol.1402448
57. Mavilio D, Lombardo G, Benjamin J, Kim D, Follman D, Marcenaro E, et al. Characterization of CD56-/CD16+ natural killer (NK) cells: a highly dysfunctional NK subset expanded in HIV-infected viremic individuals. Proc Natl Acad Sci U S A (2005) 102(8):2886–91. doi:10.1073/pnas.0409872102
58. Haynes BF, Gilbert PB, McElrath MJ, Zolla-Pazner S, Tomaras GD, Alam SM, et al. Immune-correlates analysis of an HIV-1 vaccine efficacy trial. N Engl J Med (2012) 366(14):1275–86. doi:10.1056/NEJMoa1113425
59. Tomescu C, Chehimi J, Maino VC, Montaner LJ. NK-cell lysis of HIV-1-infected autologous CD4 primary T cells: requirement for IFN-mediated NK activation by plasmacytoid dendritic cells. J Immunol (2007) 179(4):2097–104. doi:10.4049/jimmunol.179.4.2097
60. Tomescu C, Mavilio D, Montaner LJ. Lysis of HIV-1-infected autologous CD4+ primary T cells by interferon-alpha-activated NK cells requires NKp46 and NKG2D. AIDS (2015) 29(14):1767–73. doi:10.1097/QAD.0000000000000777
61. Tomescu C, Tebas P, Montaner LJ. IFN-alpha augments NK-mediated antibody-dependent cellular cytotoxicity (ADCC) of HIV-1 infected autologous CD4+ T cells regardless of MHC-I downregulation. AIDS (2017) 31(5):613–22. doi:10.1097/QAD.0000000000001380
62. Mercier-Bataille D, Sanchez C, Baier C, Le Treut T, Mounier N, Mokhtari S, et al. Expression of activating receptors on natural killer cells from AIDS-related lymphoma patients. AIDS Res Ther (2014) 11:38. doi:10.1186/1742-6405-11-38
63. Bruno A, Focaccetti C, Pagani A, Imperatori AS, Spagnoletti M, Rotolo N, et al. The proangiogenic phenotype of natural killer cells in patients with non-small cell lung cancer. Neoplasia (2013) 15(2):133–42. doi:10.1593/neo.121758
64. Li Z, Zhang LJ, Zhang HR, Tian GF, Tian J, Mao XL, et al. Tumor-derived transforming growth factor-beta is critical for tumor progression and evasion from immune surveillance. Asian Pac J Cancer Prev (2014) 15(13):5181–6. doi:10.7314/APJCP.2014.15.13.5181
65. Cerdeira AS, Rajakumar A, Royle CM, Lo A, Husain Z, Thadhani RI, et al. Conversion of peripheral blood NK cells to a decidual NK-like phenotype by a cocktail of defined factors. J Immunol (2013) 190(8):3939–48. doi:10.4049/jimmunol.1202582
66. Gibellini D, Zauli G, Re MC, Milani D, Furlini G, Caramelli E, et al. Recombinant human immunodeficiency virus type-1 (HIV-1) Tat protein sequentially up-regulates IL-6 and TGF-beta 1 mRNA expression and protein synthesis in peripheral blood monocytes. Br J Haematol (1994) 88(2):261–7. doi:10.1111/j.1365-2141.1994.tb05016.x
67. Sawaya BE, Thatikunta P, Denisova L, Brady J, Khalili K, Amini S. Regulation of TNFalpha and TGFbeta-1 gene transcription by HIV-1 Tat in CNS cells. J Neuroimmunol (1998) 87(1–2):33–42. doi:10.1016/S0165-5728(98)00044-7
68. Gigante M, Gesualdo L, Ranieri E. TGF-beta: a master switch in tumor immunity. Curr Pharm Des (2012) 18(27):4126–34. doi:10.2174/138161212802430378
69. Bruno A, Ferlazzo G, Albini A, Noonan DM. A think tank of TINK/TANKs: tumor-infiltrating/tumor-associated natural killer cells in tumor progression and angiogenesis. J Natl Cancer Inst (2014) 106(8):dju200. doi:10.1093/jnci/dju200
70. Liu Q, Sun Y, Rihn S, Nolting A, Tsoukas PN, Jost S, et al. Matrix metalloprotease inhibitors restore impaired NK-cell-mediated antibody-dependent cellular cytotoxicity in human immunodeficiency virus type 1 infection. J Virol (2009) 83(17):8705–12. doi:10.1128/JVI.02666-08
71. Azzoni L, Papasavvas E, Chehimi J, Kostman JR, Mounzer K, Ondercin J, et al. Sustained impairment of IFN-gamma secretion in suppressed HIV-infected patients despite mature NK-cell recovery: evidence for a defective reconstitution of innate immunity. J Immunol (2002) 168(11):5764–70. doi:10.4049/jimmunol.168.11.5764
72. Goodier MR, Imami N, Moyle G, Gazzard B, Gotch F. Loss of the CD56hiCD16- NK-cell subset and NK-cell interferon-gamma production during antiretroviral therapy for HIV-1: partial recovery by human growth hormone. Clin Exp Immunol (2003) 134(3):470–6. doi:10.1111/j.1365-2249.2003.02329.x
73. Chehimi J, Campbell DE, Azzoni L, Bacheller D, Papasavvas E, Jerandi G, et al. Persistent decreases in blood plasmacytoid dendritic cell number and function despite effective highly active antiretroviral therapy and increased blood myeloid dendritic cells in HIV-infected individuals. J Immunol (2002) 168(9):4796–801. doi:10.4049/jimmunol.168.9.4796
74. Appay V, Sauce D. Immune activation and inflammation in HIV-1 infection: causes and consequences. J Pathol (2008) 214(2):231–41. doi:10.1002/path.2276
75. Hutter G, Nowak D, Mossner M, Ganepola S, Mussig A, Allers K, et al. Long-term control of HIV by CCR5 Delta32/Delta32 stem-cell transplantation. N Engl J Med (2009) 360(7):692–8. doi:10.1056/NEJMoa0802905
76. Ananworanich J, Robb ML. The transient HIV remission in the Mississippi baby: why is this good news? J Int AIDS Soc (2014) 17(1):19859. doi:10.7448/IAS.17.1.19859
77. Butler KM, Gavin P, Coughlan S, Rochford A, Donagh SM, Cunningham O, et al. Rapid viral rebound after 4 years of suppressive therapy in a seronegative HIV-1 infected infant treated from birth. Pediatr Infect Dis J (2015) 34:235–40. doi:10.1097/INF.0000000000000570
78. Henrich TJ, Hanhauser E, Marty FM, Sirignano MN, Keating S, Lee TH, et al. Antiretroviral-free HIV-1 remission and viral rebound after allogeneic stem cell transplantation: report of 2 cases. Ann Intern Med (2014) 161(5):319–27. doi:10.7326/M14-1027
79. Veluchamy JP, Spanholtz J, Tordoir M, Thijssen VL, Heideman DA, Verheul HM, et al. Combination of NK cells and cetuximab to enhance anti-tumor responses in RAS mutant metastatic colorectal cancer. PLoS One (2016) 11(6):e0157830. doi:10.1371/journal.pone.0157830
80. Schoofs T, Klein F, Braunschweig M, Kreider EF, Feldmann A, Nogueira L, et al. HIV-1 therapy with monoclonal antibody 3BNC117 elicits host immune responses against HIV-1. Science (2016) 352(6288):997–1001. doi:10.1126/science.aaf0972
81. Byrareddy SN, Arthos J, Cicala C, Villinger F, Ortiz KT, Little D, et al. Sustained virologic control in SIV+ macaques after antiretroviral and alpha4beta7 antibody therapy. Science (2016) 354(6309):197–202. doi:10.1126/science.aag1276
82. Felices M, Lenvik TR, Davis ZB, Miller JS, Vallera DA. Generation of BiKEs and TriKEs to improve NK-cell-mediated targeting of tumor cells. Methods Mol Biol (2016) 1441:333–46. doi:10.1007/978-1-4939-3684-7_28
83. Gleason MK, Ross JA, Warlick ED, Lund TC, Verneris MR, Wiernik A, et al. CD16xCD33 bispecific killer cell engager (BiKE) activates NK cells against primary MDS and MDSC CD33+ targets. Blood (2014) 123(19):3016–26. doi:10.1182/blood-2013-10-533398
84. Gleason MK, Verneris MR, Todhunter DA, Zhang B, McCullar V, Zhou SX, et al. Bispecific and trispecific killer cell engagers directly activate human NK cells through CD16 signaling and induce cytotoxicity and cytokine production. Mol Cancer Ther (2012) 11(12):2674–84. doi:10.1158/1535-7163.MCT-12-0692
85. Schmohl JU, Gleason MK, Dougherty PR, Miller JS, Vallera DA. Heterodimeric bispecific single chain variable fragments (scFv) killer engagers (BiKEs) enhance NK-cell activity against CD133+ colorectal cancer cells. Target Oncol (2016) 11(3):353–61. doi:10.1007/s11523-015-0391-8
86. Tay SS, Carol H, Biro M. TriKEs and BiKEs join CARs on the cancer immunotherapy highway. Hum Vaccin Immunother (2016) 12(11):2790–6. doi:10.1080/21645515.2016.1198455
87. Vallera DA, Felices M, McElmurry R, McCullar V, Zhou X, Schmohl JU, et al. IL15 trispecific killer engagers (TriKE) make natural killer cells specific to CD33+ targets while also inducing persistence, in vivo expansion, and enhanced function. Clin Cancer Res (2016) 22(14):3440–50. doi:10.1158/1078-0432.CCR-15-2710
88. Vallera DA, Zhang B, Gleason MK, Oh S, Weiner LM, Kaufman DS, et al. Heterodimeric bispecific single-chain variable-fragment antibodies against EpCAM and CD16 induce effective antibody-dependent cellular cytotoxicity against human carcinoma cells. Cancer Biother Radiopharm (2013) 28(4):274–82. doi:10.1089/cbr.2012.1329
89. Reiners KS, Kessler J, Sauer M, Rothe A, Hansen HP, Reusch U, et al. Rescue of impaired NK-cell activity in Hodgkin lymphoma with bispecific antibodies in vitro and in patients. Mol Ther (2013) 21(4):895–903. doi:10.1038/mt.2013.14
90. Singer H, Kellner C, Lanig H, Aigner M, Stockmeyer B, Oduncu F, et al. Effective elimination of acute myeloid leukemic cells by recombinant bispecific antibody derivatives directed against CD33 and CD16. J Immunother (2010) 33(6):599–608. doi:10.1097/CJI.0b013e3181dda225
91. Wiernik A, Foley B, Zhang B, Verneris MR, Warlick E, Gleason MK, et al. Targeting natural killer cells to acute myeloid leukemia in vitro with a CD16 x 33 bispecific killer cell engager and ADAM17 inhibition. Clin Cancer Res (2013) 19(14):3844–55. doi:10.1158/1078-0432.CCR-13-0505
92. Hudak JE, Canham SM, Bertozzi CR. Glycocalyx engineering reveals a Siglec-based mechanism for NK-cell immunoevasion. Nat Chem Biol (2014) 10(1):69–75. doi:10.1038/nchembio.1388
93. Xiao H, Woods EC, Vukojicic P, Bertozzi CR. Precision glycocalyx editing as a strategy for cancer immunotherapy. Proc Natl Acad Sci U S A (2016) 113(37):10304–9. doi:10.1073/pnas.1608069113
94. Arpinati M, Curti A. Immunotherapy in acute myeloid leukemia. Immunotherapy (2014) 6(1):95–106. doi:10.2217/imt.13.152
95. Bachanova V, Burns LJ, McKenna DH, Curtsinger J, Panoskaltsis-Mortari A, Lindgren BR, et al. Allogeneic natural killer cells for refractory lymphoma. Cancer Immunol Immunother (2010) 59(11):1739–44. doi:10.1007/s00262-010-0896-z
96. Brehm C, Huenecke S, Esser R, Kloess S, Quaiser A, Betz S, et al. Interleukin-2-stimulated natural killer cells are less susceptible to mycophenolate mofetil than non-activated NK cells: possible consequences for immunotherapy. Cancer Immunol Immunother (2014) 63(8):821–33. doi:10.1007/s00262-014-1556-5
97. Brehm C, Huenecke S, Quaiser A, Esser R, Bremm M, Kloess S, et al. IL-2 stimulated but not unstimulated NK cells induce selective disappearance of peripheral blood cells: concomitant results to a phase I/II study. PLoS One (2011) 6(11):e27351. doi:10.1371/journal.pone.0027351
98. Geller MA, Ivy JJ, Ghebre R, Downs LS Jr, Judson PL, Carson LF, et al. A phase II trial of carboplatin and docetaxel followed by radiotherapy given in a “Sandwich” method for stage III, IV, and recurrent endometrial cancer. Gynecol Oncol (2011) 121(1):112–7. doi:10.1016/j.ygyno.2010.12.338
99. Kloess S, Huenecke S, Piechulek D, Esser R, Koch J, Brehm C, et al. IL-2-activated haploidentical NK cells restore NKG2D-mediated NK-cell cytotoxicity in neuroblastoma patients by scavenging of plasma MICA. Eur J Immunol (2010) 40(11):3255–67. doi:10.1002/eji.201040568
100. Kottaridis PD, North J, Tsirogianni M, Marden C, Samuel ER, Jide-Banwo S, et al. Two-stage priming of allogeneic natural killer cells for the treatment of patients with acute myeloid leukemia: a phase I trial. PLoS One (2015) 10(6):e0123416. doi:10.1371/journal.pone.0123416
101. Penack O, Gentilini C, Fischer L, Asemissen AM, Scheibenbogen C, Thiel E, et al. CD56dimCD16neg cells are responsible for natural cytotoxicity against tumor targets. Leukemia (2005) 19(5):835–40. doi:10.1038/sj.leu.2403704
102. Stern M, Passweg JR, Meyer-Monard S, Esser R, Tonn T, Soerensen J, et al. Pre-emptive immunotherapy with purified natural killer cells after haploidentical SCT: a prospective phase II study in two centers. Bone Marrow Transplant (2013) 48(3):433–8. doi:10.1038/bmt.2012.162
103. Ullrich E, Koch J, Cerwenka A, Steinle A. New prospects on the NKG2D/NKG2DL system for oncology. Oncoimmunology (2013) 2(10):e26097. doi:10.4161/onci.26097
104. Gunther S, Ostheimer C, Stangl S, Specht HM, Mozes P, Jesinghaus M, et al. Correlation of Hsp70 serum levels with gross tumor volume and composition of lymphocyte subpopulations in patients with squamous cell and adeno non-small cell lung cancer. Front Immunol (2015) 6:556. doi:10.3389/fimmu.2015.00556
105. Krause SW, Gastpar R, Andreesen R, Gross C, Ullrich H, Thonigs G, et al. Treatment of colon and lung cancer patients with ex vivo heat shock protein 70-peptide-activated, autologous natural killer cells: a clinical phase i trial. Clin Cancer Res (2004) 10(11):3699–707. doi:10.1158/1078-0432.CCR-03-0683
106. Specht HM, Ahrens N, Blankenstein C, Duell T, Fietkau R, Gaipl US, et al. Heat shock protein 70 (Hsp70) peptide activated natural killer (NK) cells for the treatment of patients with non-small cell lung cancer (NSCLC) after radiochemotherapy (RCTx) – from preclinical studies to a clinical phase II trial. Front Immunol (2015) 6:162. doi:10.3389/fimmu.2015.00162
107. Cartron G, Dacheux L, Salles G, Solal-Celigny P, Bardos P, Colombat P, et al. Therapeutic activity of humanized anti-CD20 monoclonal antibody and polymorphism in IgG Fc receptor FcgammaRIIIa gene. Blood (2002) 99(3):754–8. doi:10.1182/blood.V99.3.754
108. Goede V, Fischer K, Busch R, Engelke A, Eichhorst B, Wendtner CM, et al. Obinutuzumab plus chlorambucil in patients with CLL and coexisting conditions. N Engl J Med (2014) 370(12):1101–10. doi:10.1056/NEJMoa1313984
109. Junttila TT, Parsons K, Olsson C, Lu Y, Xin Y, Theriault J, et al. Superior in vivo efficacy of afucosylated trastuzumab in the treatment of HER2-amplified breast cancer. Cancer Res (2010) 70(11):4481–9. doi:10.1158/0008-5472.CAN-09-3704
110. Messersmith WA, Ahnen DJ. Targeting EGFR in colorectal cancer. N Engl J Med (2008) 359(17):1834–6. doi:10.1056/NEJMe0806778
111. Yu AL, Gilman AL, Ozkaynak MF, London WB, Kreissman SG, Chen HX, et al. Anti-GD2 antibody with GM-CSF, interleukin-2, and isotretinoin for neuroblastoma. N Engl J Med (2010) 363(14):1324–34. doi:10.1056/NEJMoa0911123
112. Benson DM Jr, Bakan CE, Mishra A, Hofmeister CC, Efebera Y, Becknell B, et al. The PD-1/PD-L1 axis modulates the natural killer cell versus multiple myeloma effect: a therapeutic target for CT-011, a novel monoclonal anti-PD-1 antibody. Blood (2010) 116(13):2286–94. doi:10.1182/blood-2010-02-271874
113. Benson DM Jr, Cohen AD, Jagannath S, Munshi NC, Spitzer G, Hofmeister CC, et al. A phase I trial of the anti-KIR antibody IPH2101 and lenalidomide in patients with relapsed/refractory multiple myeloma. Clin Cancer Res (2015) 21(18):4055–61. doi:10.1158/1078-0432.CCR-15-0304
114. Chan CJ, Martinet L, Gilfillan S, Souza-Fonseca-Guimaraes F, Chow MT, Town L, et al. The receptors CD96 and CD226 oppose each other in the regulation of natural killer cell functions. Nat Immunol (2014) 15(5):431–8. doi:10.1038/ni.2850
115. Gleason MK, Lenvik TR, McCullar V, Felices M, O’Brien MS, Cooley SA, et al. Tim-3 is an inducible human natural killer cell receptor that enhances interferon gamma production in response to galectin-9. Blood (2012) 119(13):3064–72. doi:10.1182/blood-2011-06-360321
116. Guo Y, Feng X, Jiang Y, Shi X, Xing X, Liu X, et al. PD1 blockade enhances cytotoxicity of in vitro expanded natural killer cells towards myeloma cells. Oncotarget (2016) 7(30):48360–74. doi:10.18632/oncotarget.10235
117. Vey N, Bourhis JH, Boissel N, Bordessoule D, Prebet T, Charbonnier A, et al. A phase 1 trial of the anti-inhibitory KIR mAb IPH2101 for AML in complete remission. Blood (2012) 120(22):4317–23. doi:10.1182/blood-2012-06-437558
118. Westin JR, Chu F, Zhang M, Fayad LE, Kwak LW, Fowler N, et al. Safety and activity of PD1 blockade by pidilizumab in combination with rituximab in patients with relapsed follicular lymphoma: a single group, open-label, phase 2 trial. Lancet Oncol (2014) 15(1):69–77. doi:10.1016/S1470-2045(13)70551-5
119. Boissel L, Betancur M, Lu W, Wels WS, Marino T, Van Etten RA, et al. Comparison of mRNA and lentiviral based transfection of natural killer cells with chimeric antigen receptors recognizing lymphoid antigens. Leuk Lymphoma (2012) 53(5):958–65. doi:10.3109/10428194.2011.634048
120. Boissel L, Betancur-Boissel M, Lu W, Krause DS, Van Etten RA, Wels WS, et al. Retargeting NK-92 cells by means of CD19- and CD20-specific chimeric antigen receptors compares favorably with antibody-dependent cellular cytotoxicity. Oncoimmunology (2013) 2(10):e26527. doi:10.4161/onci.26527
121. Chen X, Han J, Chu J, Zhang L, Zhang J, Chen C, et al. A combinational therapy of EGFR-CAR NK cells and oncolytic herpes simplex virus 1 for breast cancer brain metastases. Oncotarget (2016) 7(19):27764–77. doi:10.18632/oncotarget.8526
122. Chu J, Deng Y, Benson DM, He S, Hughes T, Zhang J, et al. CS1-specific chimeric antigen receptor (CAR)-engineered natural killer cells enhance in vitro and in vivo antitumor activity against human multiple myeloma. Leukemia (2014) 28(4):917–27. doi:10.1038/leu.2013.279
123. Esser R, Muller T, Stefes D, Kloess S, Seidel D, Gillies SD, et al. NK cells engineered to express a GD2 -specific antigen receptor display built-in ADCC-like activity against tumour cells of neuroectodermal origin. J Cell Mol Med (2012) 16(3):569–81. doi:10.1111/j.1582-4934.2011.01343.x
124. Genssler S, Burger MC, Zhang C, Oelsner S, Mildenberger I, Wagner M, et al. Dual targeting of glioblastoma with chimeric antigen receptor-engineered natural killer cells overcomes heterogeneity of target antigen expression and enhances antitumor activity and survival. Oncoimmunology (2016) 5(4):e1119354. doi:10.1080/2162402X.2015.1119354
125. Han J, Chu J, Keung Chan W, Zhang J, Wang Y, Cohen JB, et al. CAR-engineered NK cells targeting wild-type EGFR and EGFRvIII enhance killing of glioblastoma and patient-derived glioblastoma stem cells. Sci Rep (2015) 5:11483. doi:10.1038/srep11483
126. Konstantinidis KV, Alici E, Aints A, Christensson B, Ljunggren HG, Dilber MS. Targeting IL-2 to the endoplasmic reticulum confines autocrine growth stimulation to NK-92 cells. Exp Hematol (2005) 33(2):159–64. doi:10.1016/j.exphem.2004.11.003
127. Liu H, Yang B, Sun T, Lin L, Hu Y, Deng M, et al. Specific growth inhibition of ErbB2 expressing human breast cancer cells by genetically modified NK92 cells. Oncol Rep (2015) 33(1):95–102. doi:10.3892/or.2014.3548
128. Miller JS, Soignier Y, Panoskaltsis-Mortari A, McNearney SA, Yun GH, Fautsch SK, et al. Successful adoptive transfer and in vivo expansion of human haploidentical NK cells in patients with cancer. Blood (2005) 105(8):3051–7. doi:10.1182/blood-2004-07-2974
129. Muller T, Uherek C, Maki G, Chow KU, Schimpf A, Klingemann HG, et al. Expression of a CD20-specific chimeric antigen receptor enhances cytotoxic activity of NK cells and overcomes NK-resistance of lymphoma and leukemia cells. Cancer Immunol Immunother (2008) 57(3):411–23. doi:10.1007/s00262-007-0383-3
130. Nagashima S, Mailliard R, Kashii Y, Reichert TE, Herberman RB, Robbins P, et al. Stable transduction of the interleukin-2 gene into human natural killer cell lines and their phenotypic and functional characterization in vitro and in vivo. Blood (1998) 91(10):3850–61.
131. Ni Z, Knorr DA, Kaufman DS. Hematopoietic and nature killer cell development from human pluripotent stem cells. Methods Mol Biol (2013) 1029:33–41. doi:10.1007/978-1-62703-478-4_3
132. Oelsner S, Wagner J, Friede ME, Pfirrmann V, Genssler S, Rettinger E, et al. Chimeric antigen receptor-engineered cytokine-induced killer cells overcome treatment resistance of pre-B-cell acute lymphoblastic leukemia and enhance survival. Int J Cancer (2016) 139(8):1799–809. doi:10.1002/ijc.30217
133. Romanski A, Uherek C, Bug G, Seifried E, Klingemann H, Wels WS, et al. CD19-CAR engineered NK-92 cells are sufficient to overcome NK-cell resistance in B-cell malignancies. J Cell Mol Med (2016) 20(7):1287–94. doi:10.1111/jcmm.12810
134. Schirrmann T, Pecher G. Human natural killer cell line modified with a chimeric immunoglobulin T-cell receptor gene leads to tumor growth inhibition in vivo. Cancer Gene Ther (2002) 9(4):390–8. doi:10.1038/sj.cgt.7700453
135. Schonfeld K, Sahm C, Zhang C, Naundorf S, Brendel C, Odendahl M, et al. Selective inhibition of tumor growth by clonal NK cells expressing an ErbB2/HER2-specific chimeric antigen receptor. Mol Ther (2015) 23(2):330–8. doi:10.1038/mt.2014.219
136. Tam YK, Maki G, Miyagawa B, Hennemann B, Tonn T, Klingemann HG. Characterization of genetically altered, interleukin 2-independent natural killer cell lines suitable for adoptive cellular immunotherapy. Hum Gene Ther (1999) 10(8):1359–73. doi:10.1089/10430349950018030
137. Uherek C, Tonn T, Uherek B, Becker S, Schnierle B, Klingemann HG, et al. Retargeting of natural killer-cell cytolytic activity to ErbB2-expressing cancer cells results in efficient and selective tumor cell destruction. Blood (2002) 100(4):1265–73.
138. Woll PS, Grzywacz B, Tian X, Marcus RK, Knorr DA, Verneris MR, et al. Human embryonic stem cells differentiate into a homogeneous population of natural killer cells with potent in vivo antitumor activity. Blood (2009) 113(24):6094–101. doi:10.1182/blood-2008-06-165225
139. Yang B, Liu H, Shi W, Wang Z, Sun S, Zhang G, et al. Blocking transforming growth factor-beta signaling pathway augments antitumor effect of adoptive NK-92 cell therapy. Int Immunopharmacol (2013) 17(2):198–204. doi:10.1016/j.intimp.2013.06.003
140. Zhang J, Sun R, Wei H, Zhang J, Tian Z. Characterization of interleukin-15 gene-modified human natural killer cells: implications for adoptive cellular immunotherapy. Haematologica (2004) 89(3):338–47.
141. Zhang J, Sun R, Wei H, Zhang J, Tian Z. Characterization of stem cell factor gene-modified human natural killer cell line, NK-92 cells: implication in NK-cell-based adoptive cellular immunotherapy. Oncol Rep (2004) 11(5):1097–106. doi:10.3892/or.11.5.1097
142. Sander JD, Joung JK. CRISPR-Cas systems for editing, regulating and targeting genomes. Nat Biotechnol (2014) 32(4):347–55. doi:10.1038/nbt.2842
Keywords: HIV, cancer, natural killer cells, ADCC, antiretroviral therapy, lymphoma, eradication and control
Citation: Leal FE, Premeaux TA, Abdel-Mohsen M and Ndhlovu LC (2017) Role of Natural Killer Cells in HIV-Associated Malignancies. Front. Immunol. 8:315. doi: 10.3389/fimmu.2017.00315
Received: 16 December 2016; Accepted: 06 March 2017;
Published: 21 March 2017
Edited by:
Sandra Laurence Lopez-Verges, Instituto Conmemorativo Gorgas de Estudios de la Salud, PanamaReviewed by:
Roland Jacobs, Hannover Medical School, GermanyAmedeo Amedei, University of Florence, Italy
Copyright: © 2017 Leal, Premeaux, Abdel-Mohsen and Ndhlovu. This is an open-access article distributed under the terms of the Creative Commons Attribution License (CC BY). The use, distribution or reproduction in other forums is permitted, provided the original author(s) or licensor are credited and that the original publication in this journal is cited, in accordance with accepted academic practice. No use, distribution or reproduction is permitted which does not comply with these terms.
*Correspondence: Lishomwa C. Ndhlovu, bG5kaGxvdnVAaGF3YWlpLmVkdQ==
†Present address: Mohamed Abdel-Mohsen, The Wistar Institute, Philadelphia, PA, USA