- 1ENT Research Laboratory, Department of Otolaryngology-Head and Neck Surgery, Federal University of São Paulo, São Paulo, Brazil
- 2Department Biomedical Sciences, University of Antwerp, PPES Lab Proteinchemistry, Proteomics Epigenetic Signaling, Wilrijk, Belgium
- 3Department of Medicine, Nephrology Division, Federal University of São Paulo, São Paulo, Brazil
- 4Department of Immunology, Institute of Biomedical Sciences, University of São Paulo, São Paulo, Brazil
Mesenchymal stromal cells (MSCs) are considered adult progenitor stem cells and have been studied in a multitude of tissues. In this context, the microenvironment of nasal polyp tissue has several inflammatory cells, but their stroma compartment remains little elucidated. Hence, we isolated MSCs from nasal polyps Polyp-MSCs (PO-MSCs) and compared their molecular features and gene expression pattern with bone marrow-derived MSCs (BM-MSCs). Initially, both PO-MSCs and BM-MSCs were isolated, cultivated, and submitted to morphologic, differentiation, phenotypic, immunosuppressive, and gene expression assays. Compared to BM-MSCs, PO-MSCs showed normal morphology and similar osteogenic/adipogenic differentiation potential, but their immunophenotyping showed lack of immune-associated molecules (e.g., CD117, HLA-DR, PDL-1, and PDL-2), which was linked with less immunoregulatory abilities such as (i) inhibition of lymphocytes proliferation and (ii) regulatory T cell expansion. Furthermore, we detected in the PO-MSCs a distinct gene expression profile in comparison with BM-MSCs. PO-MSC expressed higher levels of progenitor stem cells specific markers (e.g., CD133 and ABCB1), while BM-MSCs showed elevated expression of cytokines and growth factors (e.g., FGF10, KDR, and GDF6). The gene ontology analysis showed that the differentially modulated genes in PO-MSC were related with matrix remodeling process and hexose and glucose transport. For BM-MSCs, the highly expressed genes were associated with behavior, angiogenesis, blood vessel morphogenesis, cell–cell signaling, and regulation of response to external stimulus. Thus, these results suggest that PO-MSCs, while sharing similar aspects with BM-MSCs, express a different profile of molecules, which presumably can be implicated in the development of nasal polyp tissue.
Introduction
Mesenchymal stromal cells (MSCs) are considered adult progenitor stem cells and have been studied in a set of pro-regenerative studies (1). MSCs are multipotent cells, with ability to differentiate into mesodermal cell lines (e.g., chondrocytes, adipocytes, and osteocytes) and can be obtained from several tissues (e.g., bone marrow, adipose tissue, umbilical cord, and muscle) (2); however, their presence in tissues affected by intense inflammation, as polyps, remains poorly elucidated.
Nasal polyposis (NP) is a chronic inflammatory condition of the upper airways, characterized by an overgrowth of paranasal sinus mucosa, with increase in eosinophil infiltration and high levels of interleukin 5 and eosinophil cationic protein (3, 4). The inflammatory reaction involves several cell types in NP and is primarily driven by a T helper-2 response (5, 6). Two major factors are related to nasal polyp formation: an abnormal remodeling response, creating a mechanical dysfunction (7, 8) and a lack of immune regulatory effects, favoring a severe inflammatory process (9).
In this sense, MSCs have a great therapeutic potential, showing specific immunomodulatory effects and an ability to directly or indirectly modulate the fibrotic process (10–12). These two MSCs abilities could have an immediate impact on NP, mitigating the tissue inflammation and rebalancing the remodeling process. In this context, our group and others’ recent studies have demonstrated a potential role of bone marrow-derived MSCs (BM-MSCs) in the modulation of several immune cells in inflamed nasal polyp tissue (13, 14). Considering this perspective, MSCs derived from nasal polyps could show different features, which would participate in the regulation of NP microenvironment, thus eliciting favorable conditions for polyp development.
Hence, in this study, we isolated MSCs from nasal polyp tissue [Polyp-MSCs (PO-MSCs)] and pointed out their main characteristics in comparison to classically known BM-MSCs. We demonstrated that PO-MSCs share similar aspects with classical BM-MSCs but have a different gene expression profile, which is associated with signaling pathways linked to stem cell biology, metabolic processes, and matrix remodeling.
Materials and Methods
MSC Isolation and Culture
The mononuclear cells for BM-MSCs isolation were collected from healthy donors at the Support Group for Children and Adolescents with Cancer in the Children’s São Paulo Hospital (GRAAC), after ethical approval and donors’ consent (n = 6, protocol no. 45/09, accession number: 30540214.0.0000.5505). PO-MSCs were collected after endoscopic polypectomy surgery in patients with NP (n = 4), according to ethical approval and donors’ consent at São Paulo Hospital, number: EPOS 12 (3). Both MSC subtypes were isolated according to Pezato et al. (13). Briefly, the transplantation plastic filters containing bone marrow cells were washed in PBS solution, and the cells were isolated using Ficoll-Hypaque method (Sigma, USA). The PO-MSCs were isolated from nasal polyp tissues by mechanical dissociation (using forceps and scissors), followed by 50 min of enzymatic digestion at 37°C (collagenase IV 1 mg/mL, Sigma). Both cells were washed in sterile PBS and filtered in a 70-μm filter (BD Biosciences, USA). After, both MSC subtypes were suspended and cultivated in 25 cm2 culture flasks (Corning, NY, USA) at 37°C in D-MEM low-glucose culture medium (45 mM NaHCO3, 10% FBS, 100 U/mL penicillin, 100 U/mL streptomycin, Gibco, USA) in a humidified atmosphere and 5% CO2.
In Vitro Differentiation Assays
The in vitro multipotent differentiation potential into mesenchymal lineages (i.e., adipocytes and osteoblasts) was assessed using the adipogenesis and osteogenesis Mesenchymal Stem Cell Kit (Millipore, USA), according to the manufacturer’s specifications.
Immunophenotyping
The immunophenotyping of both different types of MSCs was carried out using a specific set of antibodies (i.e., CD34, CD45, CD105, CD90, CD73, CD54, CD117, HLA-DR, PDL-1, and PDL-2, BD Bioscience, USA), according to the manufacturer’s recommendations. The cells were collected, and the immunostaining was adjusted to 1:100 of antibody dilution. Then, the cells were incubated with a specific antibody per 30 min at room temperature in FACs buffer (PBS + 2% FBS). Then, the cells were washed in PBS solution and suspended in FACs buffer for acquisition in a flow cytometer. The FACs Canto II (BD, Beckton Dickson) was used for cell acquisition, and the FlowJo software was used for data collection and analysis.
Lymphocyte Proliferative Assay and Treg Expansion
For investigate the immunosuppressive potential of PO-MSCs and BM-MSCs, these cells were cultivated with fresh peripheral blood mononuclear cell (PBMC) in two different lymphocytes/MSCs proportions: (i) 5:1 and (ii) 20:1. The lymphocytes were isolated from healthy donors by Ficoll-Hypaque method (Sigma, USA) and previously labeled with fluorescent dye, Cell Trace (Life Technologies, USA), following the manufacturer’s instructions. For proliferation assay, PBMCs were cultivated by 6 days with or without MSCs (from Polyp or BM) under anti-CD3/CD28 stimulus (1/2 μg/mL, respectively) with RPMI medium + 10% FBS in flat bottom 96-well plate (TPP, USA). Then, all non-adherent cells were collected, stained with anti-Foxp3, anti-CD4, and anti-CD8 conjugated antibodies (APC, FITC, and Percep, BD, Beckton Dickson) and subsequently analyzed by flow cytometry following protocols and acquisition parameters aforementioned.
Gene Expression Profile of MSCs and In Silico Analysis
Total RNA from both bone marrow and nasal polyp MSC cells was extracted using an RNeasy Mini Kit (50) (Qiagen, South Korea), according to the manufacturer’s instructions. The concentration and integrity of RNA samples were, respectively, evaluated using a Nanodrop spectrophotometer (Thermo Scientific, USA) and 1% agarose gel electrophoresis (Gibco, USA). Furthermore, reverse transcription of total RNAs was performed using the RT2 First Strand Kit (Qiagen, South Korea). Global gene expression profile was performed in 96-well plates per each set, following the recommendations specified in the products’ catalogs: RT2 SYBR Green ROX qPCR Master Mix and Mesenchymal Stem Cells PCR array (84 genes; Qiagen, South Korea). Data analysis and normalization were performed using the free online software provided on the manufacturer’s website (Qiagen, South Korea). The signaling pathways associated with differentially modulated genes were performed in the GOminer web software (website or reference), according to the specificity in the default layout. The transcriptional factor enrichment analysis was carried out using the functional enrichment analysis web tool FUNRICH using standard default.
Statistical Analysis
All statistical analysis and graphs were performed using the GraphPad Prism 5 software (GraphPad, Inc., USA). Data were presented according to classical descriptive statistics. Results were tested for normal distribution by the Kolmogorov–Smirnov test with Dallal–Wilkinson–Lillie for a p-value. Categorical variables were expressed as percentages (%), and continuous variables (data) were presented as means ± SDs. The parametric Student’s t-test and One-way ANOVA test were used to assess differences between groups. For all analyses, a p-value ≤0.05 was considered statistically significant.
Results
In Vitro Characterization and Immunophenotyping of PO-MSCs and BM-MSCs
First, both MSC subtypes were isolated and submitted to standard culture conditions (see Figure S1 in Supplementary Material). These cells had fibroblastic-like morphology (Figures 1A,C) and similar differentiation potential into mesodermal lineages such as adipocytes and osteocytes (Figures 1B,D). The immunophenotyping analysis of PO-MSCs and BM-MSCs showed a negative expression for hematopoietic surface markers (CD34 and CD45) and a positive status for classical mesenchymal markers, such as CD105, CD90, CD73, and CD54 (Figure 1E; Figure S2 and Table S1 in Supplementary Material). However, the PO-MSCs in comparison with BM-MSCs had reduced expression of molecules related to immune regulation process, e.g., CD117, HLA-DR, PD-L1, and PD-L2 (Figure 1E; Figure S2 and Table S1 in Supplementary Material). The internal proliferation rates did not change along the passages showing no significant difference (data not showed); however, the global intranuclear Ki-67 expression (a proliferation pan marker) of BM-MSCs was higher than PO-MSCs (Figure 1F), suggesting that BM cells can present more proliferative status.
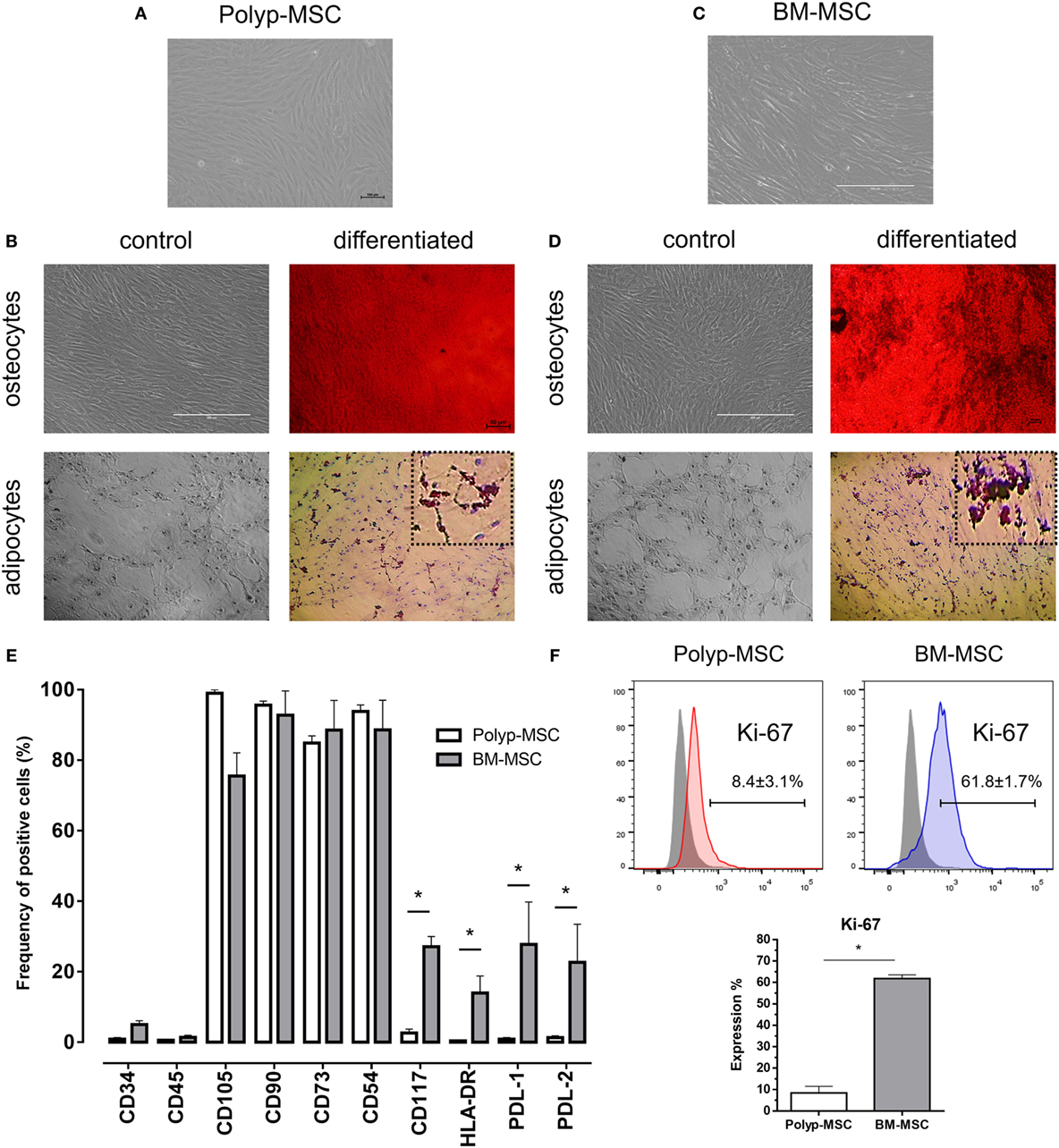
Figure 1. Characterization of Polyp-MSCs (PO-MSCs) and bone marrow-derived mesenchymal stem cells (BM-MSCs). (A) Fibroblast-like morphology of PO-MSCs; (B) mesodermal differentiation potential of PO-MSC; (C) fibroblast-like morphology of BM-MSCs; (D) mesodermal differentiation potential of BM-MSC; (E) immunophenotyping of mesenchymal stromal cells derived from polyp and bone marrow tissues; and (F) Ki-67 expression in PO-MSC and BM-MSCs. A similar morphology and differentiation potential into adipocyte- and osteocyte-like cells were observed in cultures of PO-MSCs and BM-MSCs. However, the PO-MSCs in comparison with BM-MSCs showed a decreasing in the expression of surface molecules related to immunoregulation (i.e., CD117, HLA-DR, PDL-1, and PDL-2) and proliferation (Ki-67) (*p < 0.05).
Immunosuppressive Assay of PO-MSCs and BM-MSCs in Coculture with CD4+ and CD8+ Lymphocytes
In order to elucidate the immunoregulatory potential of PO-MSCs, we performed a coculture assay of these cells with T lymphocytes and compared its functionality with BM-MSCs, which classically present strong immunomodulatory abilities. First, we observed that lymphocyte alone showed higher proliferation index (>60%) (Figure 2). After, we detected at two different dilutions (i.e., 5:1 and 20:1 lymphocytes/MSCs proportion) that both MSC subpopulations (PO-MSCs and BM-MSCs) presented capacities to suppress CD4+ and CD8+ lymphocytes proliferation; however, the immunosuppressive potential was inferior to PO-MSC when compared with BM-MSC, considering the 20:1 proportion (Figure 2). Interestingly, when we observed in this same coculture the frequency of CD4+/Foxp3+ cells, a subpopulation of regulatory T cell (Treg), its index was higher in BM-MSC presence than PO-MSC, considering the 5:1 proportion (Figure 3). However, no difference in Tregs frequency was verified in higher dilution (20:1 proportion) at both MSC populations: PO-MSCs and BM-MSCs (Figure 3).
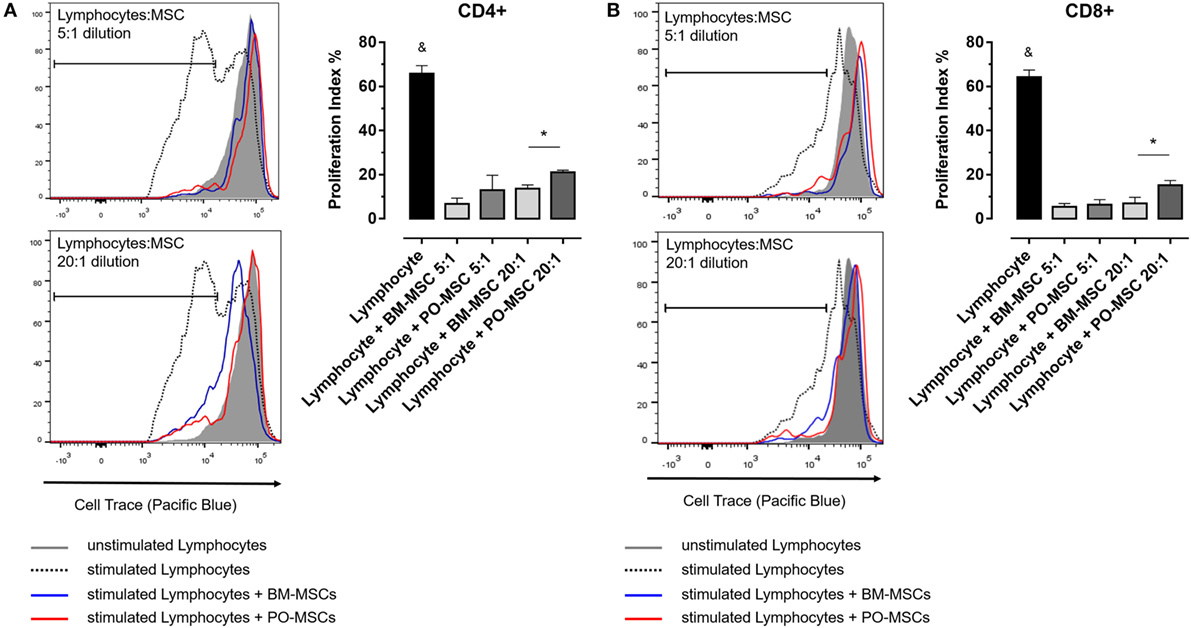
Figure 2. Lymphocyte proliferation assay in coculture with Polyp-MSCs (PO-MSCs) and bone marrow-derived MSCs (BM-MSCs). (A) Lymphocytes were stimulated with anti-CD3/CD28 antibodies per 6 day and were cocultivated in presence of PO-MSCs or BM-MSCs in two different dilutions with 5:1 and 20:1 of lymphocytes/MSCs proportion. (A,B) Refer, respectively, to proliferation index of CD4+ and CD8+ lymphocytes in different conditions [i.e., without mesenchymal stromal cells (MSCs), with MSCs at 5:1 and 20:1 dilutions]. It was observed that both MSCs inhibited the total lymphocytes proliferation; however, PO-MSCs in comparison with BM-MSC had less immunosuppressive response at higher dilution (e.g., 20:1 proportion) (&p < 0.05 in comparison to all groups and *p < 0.05 in comparison to BM-MSC in 20:1 dilution with lymphocytes).
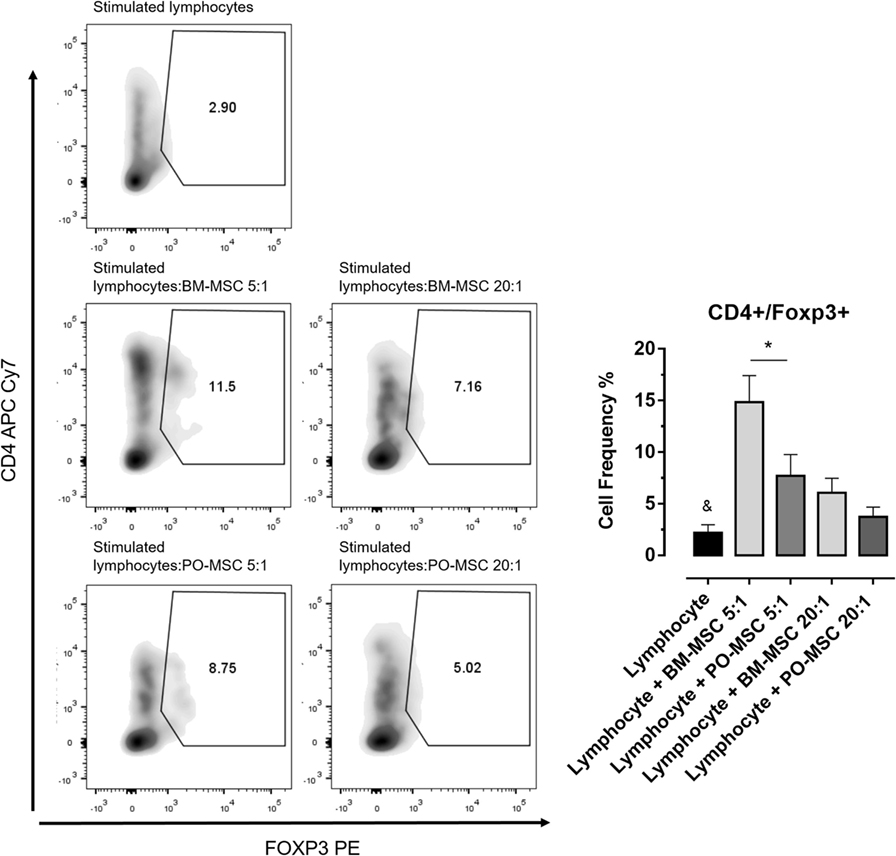
Figure 3. Frequency of regulatory T cell (Treg) (CD4+/Foxp3+) in coculture with Polyp-MSCs (PO-MSCs) and bone marrow-derived MSCs (BM-MSCs). During coculture assay with CD4+ lymphocytes and mesenchymal stromal cells (Polyp and BM), it was possible to detected that only BM-MSC had strong abilities to promote the expansion of Treg population (CD4+/Foxp3+) at 5:1 dilution. Although PO-MSCs have expanded Tregs, its index was inferior to BM-MSC considering the 5:1 and 20:1 dilutions (&p < 0.05 in comparison to all groups and *p < 0.05 in comparison to BM-MSC in 5:1 dilution with lymphocytes).
Analysis of the Gene Expression Profile of PO-MSCs in Comparison with BM-MSCs
To improve our extensive characterization between these two MSC subpopulations derived from different tissues, we performed the global gene expression profile, covering 84 genes associated with mesenchymal stem cell biology. The volcano plot analysis showed the significant modulation of specific genes, which were up- and downmodulated in the PO-MSCs in comparison with BM-MSCs (Figure 4A). More specifically, using the Venn Diagram strategy, we detected 15 upregulated genes, 23 downregulated genes, and 46 genes similarly expressed in PO-MSCs when compared with BM-MSCs (Figure 4B; Figure S3A in Supplementary Material). Subsequently, using heat map analysis, it was possible to observe the global differences in gene expression profile (green/down and red/up colors) between the two assessed cell types, as well as the most representative clustering groups of samples and genes for each cell preparations (Figure 4C; Figure S3B in Supplementary Material). Interestingly, several genes were modulated in the comparison of PO-MSCs versus BM-MSCs, and the samples of each different tissue were grouped in distinctive clusters, demonstrating a similar transcriptional pattern between samples derived from polyp tissues and bone marrow tissues (Figure 4C).
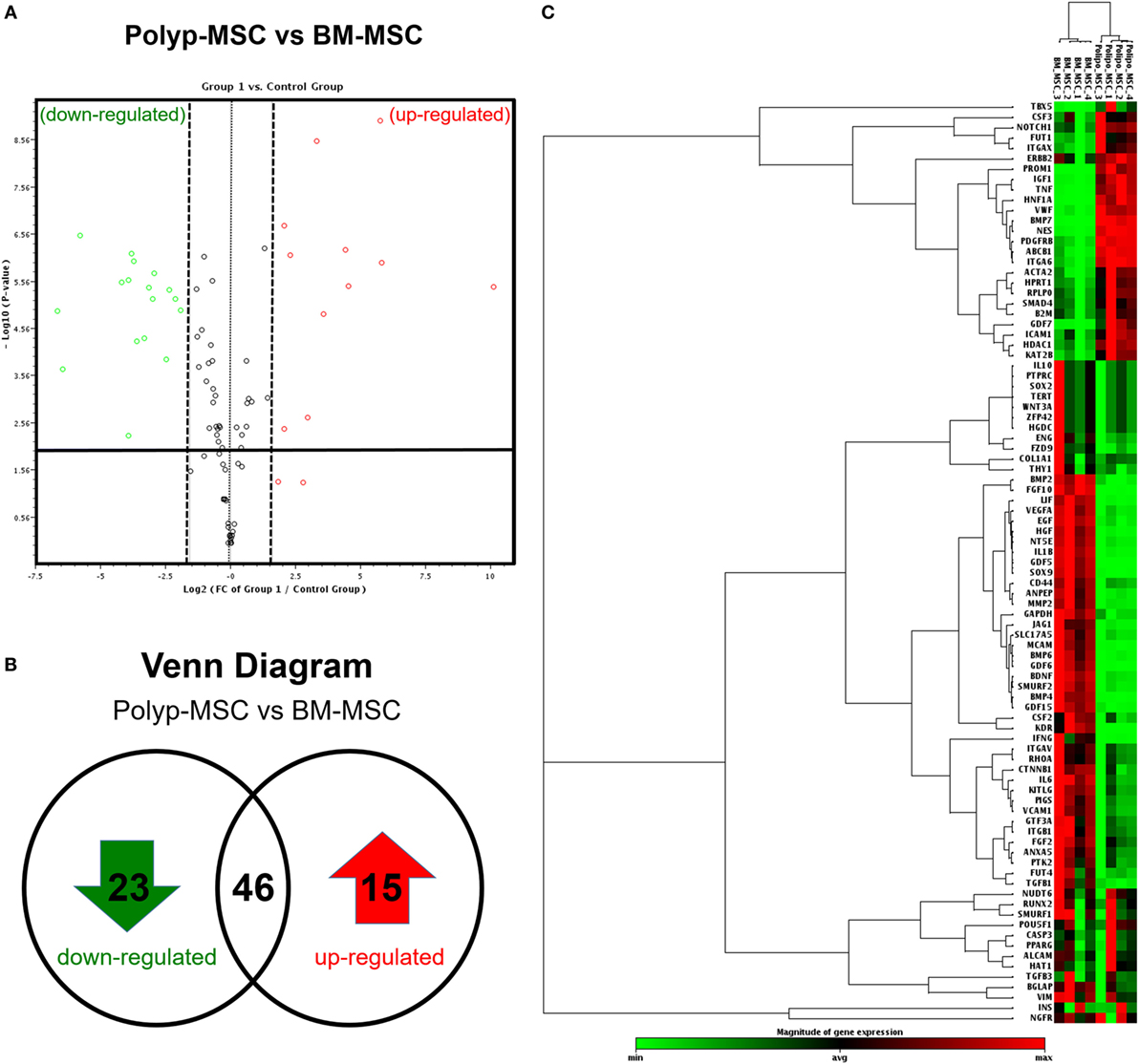
Figure 4. Global gene expression profile. (A) Volcano-plot graph showing the most representative upregulated (red) and downregulated (green) genes in Polyp-MSC (PO-MSC) in comparison with bone marrow-derived MSC (BM-MSC); (B) Venn diagram illustrating the number of upregulated/downregulated genes; and (C) heat map-cluster analysis demonstrating a global difference in gene expression, as well as the most representative clusters of samples and genes. Interestingly, there were several modulated genes in the comparison of PO-MSCs versus BM-MSCs, and the mesenchymal stromal cells from each tissue (Polyp and bone marrow) showed a different profile, being grouped in the same group.
PO-MSCs and BM-MSCs Have Different Transcriptional Profiles
In an attempt to elucidate the differentially modulated genes in the PO-MSCs in comparison with BM-MSCs, we carried out the fold-change analysis and pointed out the most significant upregulated or downregulated genes. For PO-MSCs, we found PROM1, HNF1A, BMP7, TNF, ABCB1, IGF1, NES, GDF7, TBX5, VWF, FUT1, PDGFRB, CSF3, ITGAX, and ITGA6 as the most often expressed genes, and for BM-MSCs, we detected GDF6, KDR, FGF10, GDF5, IFNG, SOX9, IL1B, LIF, BMP6, MCAM, GDF15, HGF, BDNF, JAG1, NT5E, SMURF2, VEGFA, FZD9, BMP4, MMP2, IL6, EGF, and FUT4 as the most upregulated ones (Figure 5). Surprisingly, the PO-MSCs in comparison with BM-MSCs showed higher expression of PROM1 or CD133 and ABCB1, which are considered progenitor markers, suggesting a different stem cell property for this MSC subtype. On the other hand, the BM-MSCs showed a strong regulation of genes associated with cytokines and growth factors (i.e., FGF10, KDR, and GDF6), which are particular for these BM-MSC subsets (Figure 5).
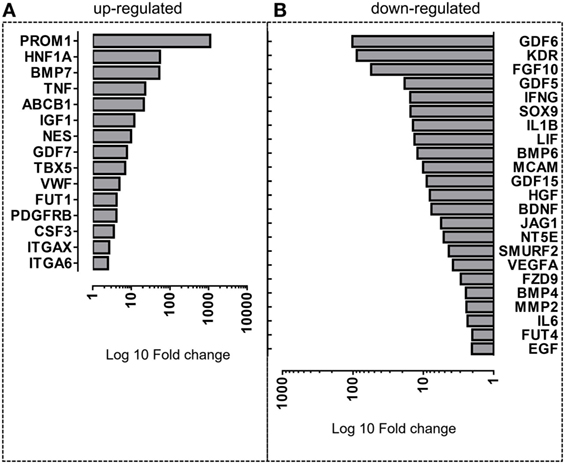
Figure 5. Differentially expressed genes in Polyp-MSCs (PO-MSCs) in comparison with bone marrow-derived MSCs (BM-MSCs). Bar plot representing the most upmodulated (A) and downmodulated (B) genes in the comparison of PO-MSC versus BM-MSC. A differential gene expression profile is observed between PO-MSCs and BM-MSCs, suggesting a distinct transcriptional background for these cell populations.
Signaling Pathways Associated with Upregulated Genes in PO-MSCs and in BM-MSCs
To elucidate some interesting signaling pathways associated with most upmodulated/downmodulated genes in PO-MSCs in comparison with BM-MSCs, we performed the gene ontology analysis and searched for biological pathways that were significantly enriched with those genes. Using this in silico analysis, we detected alcohol metabolic process, hexose transport, glucose transport, carbohydrate metabolic process, and monosaccharide metabolic process as most significant biological pathways associated with upregulated genes in PO-MSCs (Figure 6A). In contrast, for BM-MSCs, we found behavior, angiogenesis, blood vessel morphogenesis, cell–cell signaling, and regulation of external stimulus response as the most enriched signaling pathways linked to upregulated genes. Altogether, these findings suggest that PO-MSC and BM-MSC are different MSC cell subpopulations, showing distinct transcriptional background, which can reflect their specific biological properties for each tissue’s localization (polyp tissue and bone marrow) (Figure 6B).
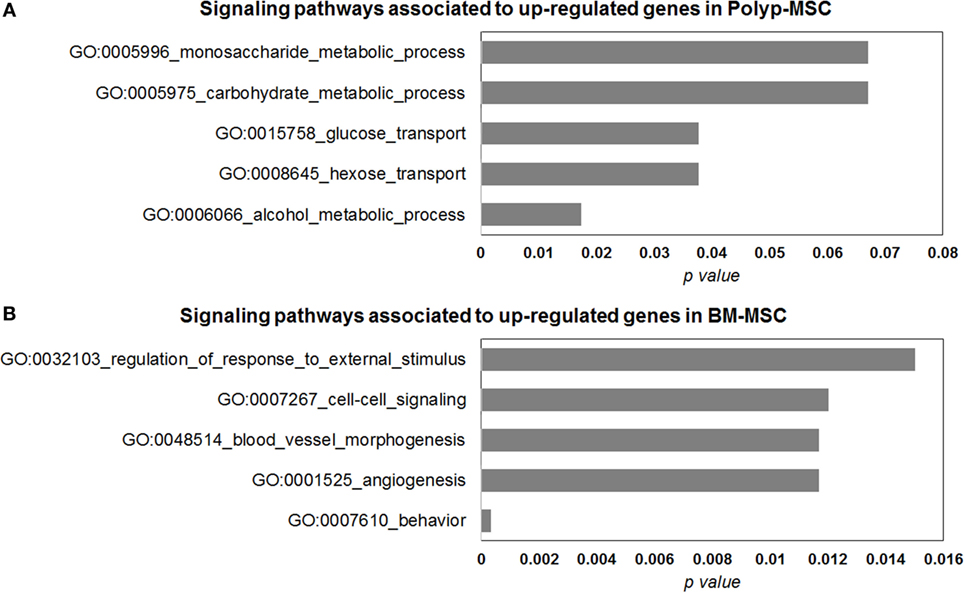
Figure 6. Gene ontology analysis of differentially modulated genes in Polyp-MSCs (PO-MSCs) in comparison with bone marrow-derived MSCs (BM-MSCs). (A,B) Gene ontology analysis showing genes regulated (up/down) in PO-MSCs in comparison with BM-MSCs, which are predicted to be enriched in different signaling pathways. For the genes upregulated in PO-MSC, an association was found with alcohol metabolic process, hexose transport, glucose transport, carbohydrate metabolic process, and monosaccharide metabolic process, while for genes upregulated in BM-MSCs, the association was linked to behavior response, angiogenesis, blood vessel morphogenesis, cell–cell signaling, and regulation of external stimulus response.
Transcription Factor Enrichment Analysis of Upregulated Genes in PO-MSCs and in BM-MSCs
In search for functional regulatory association regarding upregulated genes in PO-MSC or in BM-MSC, we empirically pointed out the most often associated transcriptional factors that were enriched with these genes. PO-MSCs showed the representative participation of POU2F1 (15.7%, p = 0.03) and TFAP4 (12.5%, p = 0.02) as main transcriptional regulators at upregulated genes, which are related to cancer stem cells, cell cycle, and histone regulation (Figure 7A). On the other hand, the transcription factors most often associated with upregulated genes in BM-MSCs were EGR-1 (22.5%, p < 0.01) and NFIC (18.4%, p < 0.01), which are linked to cell proliferation, differentiation, apoptosis, and cell growth (Figure 7B). Thus, based on transcription-binding sites regulation pattern, these cells can represent different MSC subtypes, which modulate their transcriptional profile according to the distinct interaction with specific microenvironments in which these cells are found (polyp tissue and bone marrow).
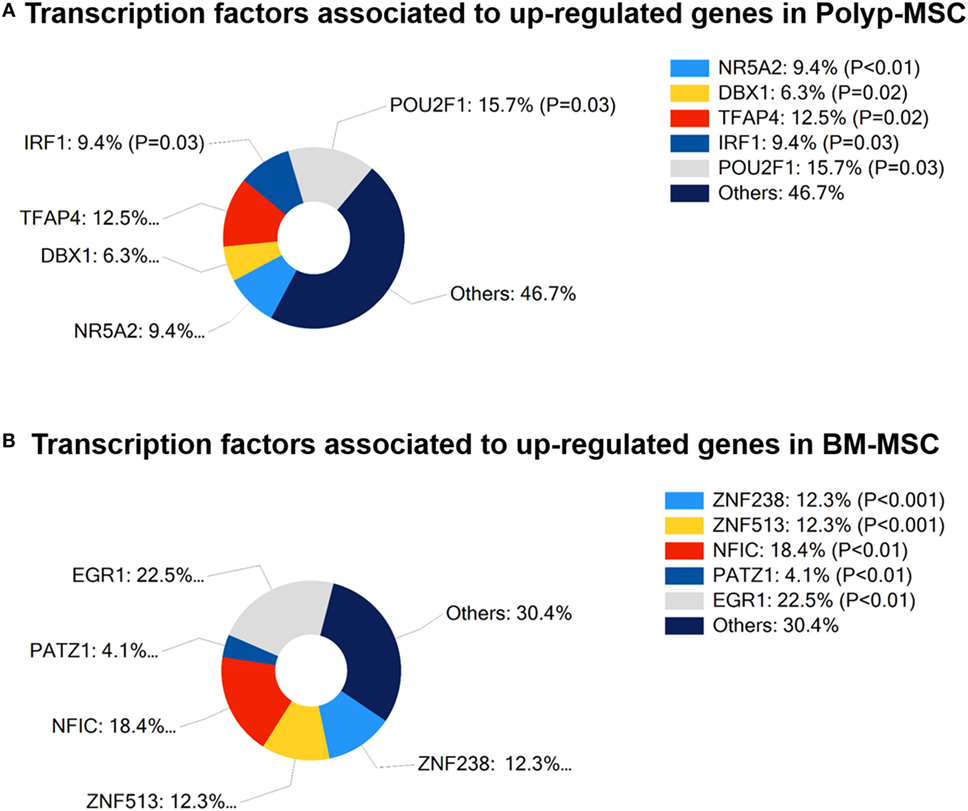
Figure 7. Transcription factors associated with differentially modulated genes in Polyp-MSCs (PO-MSCs) and in bone marrow-derived MSCs (BM-MSCs). (A) Percentage of participation of transcriptional regulators in upregulated genes in PO-MSCs. (B) Contribution (%) of main transcriptional regulators associated with upregulated genes in BM-MSCs. It is possible to observe that the transcription factors most often associated with modulated genes in PO-MSC were POU2F1 (15.7%, p = 0.03) and TFAP4 (12.5%, p = 0.02), while EGR-1 (22.5%, p < 0.01) and NFIC (18.4, p < 0.01) were predicted as main transcriptional regulators in BM-MSC.
Discussion
Mesenchymal stromal cells can be isolated from almost all tissues and effectively cultured in vitro. Although their actual properties and biological functions have not been completely elucidated, these cells have been shown to represent an important approach in several clinical applications and in the regulation of immunocompetent cell responses. Human nasal-derived MSCs have been isolated mainly from inferior turbinate tissue and from olfactory tissue by several groups, and it has been suggested to have a valuable role in tissue engineering and regenerative medicine (15–20).
In this study, we have isolated MSCs from abnormal nasal polyp tissue (PO-MSC) and compared their gene expression profile and immune phenotype with BM-MSCs. Our results showed that several MSCs immune-associated membrane markers CD117, HLA-DR, PD-L1, and PD-L2 were not expressed in PO-MSCs but were expressively present in BM-MSCs. These markers are mainly associated with the immuneregulatory capacity of MSCs. For instance, PD-L1 molecule is a component of the T-lymphocyte costimulatory pathway and plays a crucial role in controlling T-cell proliferation and immunosuppression, and hence, it prevents tissue damage and autoimmunity (21, 22). Furthermore, PD-1 ligand 2 (PD-L2) may also inhibit T-cell receptor-mediated proliferation and cytokine production by CD4+-activated T-cells (23). PD-L1 and PD-L2 have been reported to be highly expressed by human placenta MSCs, which have a strong effect on adhesion, migration, and immunosuppression mechanisms of T-cells (24). Considering this perspective, we performed here a classical immunosuppressive coculture assay with both MSC subtypes (Polyp and BM) in contact with CD4+ and CD8+ lymphocytes. We observed that both MSC populations suppressed lymphocytes proliferation; however, PO-MSCs were less effective at higher dilutions. In addition, this PO-MSC also promotes reduced Treg expansion when compared with BM-MSC at same proportion. These results suggest that, unlikely BM-MSCs, PO-MSCs may have limited capacity to modulate T-cell-mediated immune response by a mechanism apparently mediated by low expression of immunoregulatory surface markers (i.e., PD-L1 and PD-L2). A previous study of our group showed this mechanism when coculture of MSCs and lymphocytes were seeded with anti-PD-L1 inhibitor, and no Treg expansion or lymphocyte repression was observed (25). Thus, all this finding raises questions regarding the presence of strong anti-inflammatory properties in PO-MSCs, as classically observed in BM-MSCs (11, 13). Moreover, we identified that PO-MSCs, in comparison with BM-MSCs, expressed very lower levels of HLA-DR surface marker. Although BM-MSCs already is known to present low levels of HLA-DR (26), this finding can suggest an additional immunogenic advantage for PO-MSCs, since HLA-DR is widely involved with tissue engraftment and rejection (27) and its low expression suggests an therapeutic advantage for MSC derived from nasal polyp tissue. In fact, we assume that this fundamental tolerating ability was not tested in this study, and this point needs to be investigated in further works involving tumorigenesis assays.
To analyze the molecular properties of PO-MSCs, we carried out a specific gene expression assay comprising the most relevant stem cell gene profile. We found a set of 15 genes that were statistically overexpressed in PO-MSCs, when compared to BM-MSCs. Interestingly, we observed that three genes (i.e., PROM1, HNF1A, and BMP7) had a higher fold-change index (>50-fold regulation) in PO-MSCs than in BM-MSCs. The PROM1, also known as CD133, is a gene that encodes a transmembrane glycoprotein, which is expressed in human hematopoietic stem cells and mouse neuroepithelial cells (28, 29). This molecule is considered a key biomarker for isolation and characterization of progenitor hematopoietic stem cells (30) and plays a crucial role in maintaining stem cell properties by suppressing cell differentiation and regulating crucial cellular events such as regeneration, differentiation, and metabolism. The PROM1 and Nestin (NES) have been reported to be strongly expressed in ectomesenchymal stem cells derived from rat nasal respiratory mucosa (31). These genes are also highly expressed in cells from the nervous system, as observed by Weigmann et al. (29), suggesting that PO-MSCs may have a possible potential to differentiate into neuronal-like cells as nasal MSCs. Otherwise, this phenotype can also represent basal cells from the olfactory epithelium, which act as supportive stem cells for the neuroepithelial lineages and could be located inside of PO-MSC population, expressing higher levels of PROM1; however, this association needs also to be demonstrated in further investigations.
The HNF1, which was also upregulated in PO-MSCs, is a transcriptional activator that regulates the tissue-specific expression of multiple genes, especially in pancreatic islet and liver cells. HNF1 is also included in the human embryonic stem cell pluripotency pathway, where it regulates the transcription of other genes involved in cell growth, cell adhesion, epithelial formation, immune system, and inflammation, including TNF (32). Additionally, HNF1 may indirectly regulate the expression of BMP7, which encodes a complex of adherent junction proteins. These molecules are crucial for the formation and maintenance of epithelial cell layers, and hence, they participate in cell growth regulation (33). In NP context, the HNF1/BMP7 axis is important for epithelium integrity and is associated with TGF-β expression during the remodeling process (34–38). Altogether, these genes are closely associated with NP remodeling process, suggesting a prospective role of PO-MSCs in the modulation of NP microenvironment.
In our global expression gene analysis, we also detected four genes (i.e., GDF6, KDR, FGF10, and GDF5) that were significantly downregulated (more than 20-fold regulation) in the PO-MSCs when compared to BM-MSCs. The growth differentiation factors GDF5 and GDF6 are part of the TGF superfamily, which regulates cell proliferation and differentiation, bone and cartilage formation, and skeletal development. BM-MSCs have a strong capacity to differentiate into osteoblasts (39), and it is not surprising to find a broad expression of osteoblastic factors upregulated in these cells. However, the transcription level deficiency of GDF5 and GDF6 in PO-MSCs could not be totally clarified in this study. GDF-5 has been shown to play a crucial role not only in several musculoskeletal processes (40, 41) in several studies but also in cell proliferation, inflammation, and extracellular matrix formation (42). The role of this factor in the expression of metalloproteinases and their inhibitors and the influence in healing during tendon injuries were also demonstrated by Park et al. (43). It has been also suggested that GDF6 is involved in tissue vascular regeneration and angiogenesis through regulation of the expression and the signaling of Smads molecules (44). However, it is noteworthy mentioning that all this evidence is based on the results obtained from studies performed in vitro or in animal models, which use mainly exogenously administrated or recombinant GDFs isoforms. Hence, the exact role of these growth differentiation factors in PO-MSCs still remains unclear.
Complementary to gene expression profile, our in silico prediction analysis showed that POU2F1 and TFAP4 motifs are predicted to be enriched in PO-MSCs. These transcription regulators are mainly related to cancer stem cells, cell cycle, and histone regulation, attributing an internal transcriptional regulatory potential to MSC from NP tissue (45). On the other hand, we found EGR-1 and NFIC as predicted transcription factors to be regulated in BM-MSCs. These molecules are linked to cell proliferation, differentiation, apoptosis, and growth, suggesting that bone marrow MSC has a prominent role as a cellular regulator, mainly through the secretion of wide range of growth factors (46, 47).
Considering this evidence, we have demonstrated that although PO-MSCs and BM-MSCs share some features (i.e., morphology, surfaces markers, and differentiation capacity), they have a different transcriptional regulation profile and possibly distinct physiological function. Since that polyp is an abnormal tissues that grow over chronic inflammatory condition (3), this observation is in line with a previous study showing that human MSC from ethmoid sinus mucosa has different proliferation capability and distinct expression of immunomodulatory cytokines when compared to MSCs isolated from the maxillary sinus, ethmoidal mucosa, and inferior turbinate (15); these findings support the idea that the inferior turbinate mucosa has different molecular, cellular, and mechanical properties, when compared with middle meatus mucosa (8). Similarly, as expected, our study also suggests that MSC from abnormal polyp nasal mucosa has distinct molecular pattern in comparison with MSCs from normal bone marrow tissues.
Recently, two studies reported the isolation of MSCs from nasal polyp tissue and their results completely support our findings (14, 15). In addition, in our study here presented we went one step further and provided support to the concept that MSCs may have inherent properties and a gene/protein expression pattern according to their anatomical location and their interaction with specific microenvironments. It is remarkable that the most significant differentially expressed genes in PO-MSCs were partially linked to metabolic and ECM/tissue remodeling/regeneration processes, which are signaling pathways clearly affected in nasal polyp disease (48). Nevertheless, considering PO-MSCs as a possible therapeutic alternative for tissue regeneration, we need to know the implications of this gene expression profile in vivo, as well as clarify the intrinsic association of these cells with their pro-inflammatory environment.
In conclusion, in this study, we compared two MSC populations and demonstrated the lack of immunoregulatory markers and a different transcriptional profile in PO-MSC in comparison to BM-MSCs, suggesting that this distinct molecular pattern may be indirectly implicated in the development of nasal polyp tissue.
Author Contributions
Conceptualization: PW and RP. Investigation and resources: PW, RP, JA, CP-N, WB, and DA. Supervision: RP, CP-N, WB, NC, and LG. Writing: RP, DA, and CP-N.
Conflict of Interest Statement
The authors declare that the research was conducted in the absence of any commercial or financial relationships that could be construed as a potential conflict of interest.
Acknowledgments
The authors thank all collaborators who have contributed for this work providing support in experimental assays and writing steps.
Funding
This study was specifically supported by Fundação de Amparo à Pesquisa do Estado de São Paulo (FAPESP, accession number: 12/02270-2) and Conselho Nacional de Desenvolvimento Científico e Tecnológico (CNPq).
Supplementary Material
The Supplementary Material for this article can be found online at http://journal.frontiersin.org/article/10.3389/fimmu.2017.00039/full#supplementary-material.
References
1. Bassi Ê, de Almeida DC, Moraes-Vieira PM, Câmara NO. Exploring the role of soluble factors associated with immune regulatory properties of mesenchymal stem cells. Stem Cell Rev (2012) 8(2):329–42. doi: 10.1007/s12015-011-9311-1
2. de Almeida DC, Donizetti-Oliveira C, Barbosa-Costa P, Origassa CS, Câmara NO. In search of mechanisms associated with mesenchymal stem cell-based therapies for acute kidney injury. Clin Biochem Rev (2013) 34(3):131–44.
3. Fokkens WJ, Lund VJ, Mullol J, Bachert C, Alobid I, Baroody F, et al. European position paper on rhinosinusitis and nasal polyps. Rhinol Suppl (2012) 2012(23):3 p preceding table of contents, 1–298.
4. Bachert C, Zhang N, Holtappels G, De Lobel L, van Cauwenberge P, Liu S, et al. Presence of IL-5 protein and IgE antibodies to staphylococcal enterotoxins in nasal polyps is associated with comorbid asthma. J Allergy Clin Immunol (2010) 126(5):.e1–6. doi:10.1016/j.jaci.2010.07.007
5. Pawankar R, Nonaka M. Inflammatory mechanisms and remodeling in chronic rhinosinusitis and nasal polyps. Curr Allergy Asthma Rep (2007) 7(3):202–8. doi:10.1007/s11882-007-0073-4
6. Pezato R, Świerczyńska-Krępa M, Niżankowska-Mogilnicka E, Derycke L, Bachert C, Pérez-Novo CA. Role of imbalance of eicosanoid pathways and staphylococcal superantigens in chronic rhinosinusitis. Allergy (2012) 67(11):1347–56. doi:10.1111/all.12010
7. Pezato R, Voegels RL, Pinto Bezerra TF, Perez-Novo C, Stamm AC, Gregorio LC. Mechanical dysfunction in the mucosal oedema formation of patients with nasal polyps. Rhinology (2014) 52(2):162–6. doi:10.4193/Rhin
8. Pezato R, Voegels RL, Stamm AC, Gregório LC. Why we should avoid using inferior turbinate tissue as control to nasal polyposis studies. Acta Otolaryngol (2016) 136(9):973–5. doi:10.3109/00016489.2016.1166262
9. Zhang N, Van Zele T, Perez-Novo C, Van Bruaene N, Holtappels G, DeRuyck N, et al. Different types of T-effector cells orchestrate mucosal inflammation in chronic sinus disease. J Allergy Clin Immunol (2008) 122(5):961–8. doi:10.1016/j.jaci.2008.07.008
10. Semedo P, Correa-Costa M, Antonio Cenedeze M, Maria Avancini Costa Malheiros D, Antonia dos Reis M, Shimizu MH, et al. Mesenchymal stem cells attenuate renal fibrosis through immune modulation and remodeling properties in a rat remnant kidney model. Stem Cells (2009) 27(12):3063–73. doi:10.1002/stem.214
11. Silva FdS, Ramos RN, de Almeida DC, Bassi EJ, Gonzales RP, Miyagi SP, et al. Mesenchymal stem cells derived from human exfoliated deciduous teeth (SHEDs) induce immune modulatory profile in monocyte-derived dendritic cells. PLoS One (2014) 9(5):e98050. doi:10.1371/journal.pone.0098050
12. Qi Y, Jiang D, Sindrilaru A, Stegemann A, Schatz S, Treiber N, et al. TSG-6 released from intradermally injected mesenchymal stem cells accelerates wound healing and reduces tissue fibrosis in murine full-thickness skin wounds. J Invest Dermatol (2014) 134(2):526–37. doi:10.1038/jid.2013.328
13. Pezato R, de Almeida DC, Bezerra TF, Silva FdS, Perez-Novo C, Gregório LC, et al. Immunoregulatory effects of bone marrow-derived mesenchymal stem cells in the nasal polyp microenvironment. Mediators Inflamm (2014) 2014:583409. doi:10.1155/2014/583409
14. Cho KS, Kim YW, Kang MJ, Park HY, Hong SL, Roh HJ. Immunomodulatory effect of mesenchymal stem cells on T lymphocyte and cytokine expression in nasal polyps. Otolaryngol Head Neck Surg (2014) 150(6):1062–70. doi:10.1177/0194599814525751
15. Cho JS, Park JH, Kang JH, Kim SE, Park IH, Lee HM. Isolation and characterization of multipotent mesenchymal stem cells in nasal polyps. Exp Biol Med (Maywood) (2015) 240(2):185–93. doi:10.1177/1535370214553898
16. Cho KS, Park HY, Roh HJ, Bravo DT, Hwang PH, Nayak JV. Human ethmoid sinus mucosa: a promising novel tissue source of mesenchymal progenitor cells. Stem Cell Res Ther (2014) 5(1):15. doi:10.1186/scrt404
17. Park JH, Park JY, Nam IC, Hwang SH, Kim CS, Jung JW, et al. Human turbinate mesenchymal stromal cell sheets with bellows graft for rapid tracheal epithelial regeneration. Acta Biomater (2015) 25:56–64. doi:10.1016/j.actbio.2015.07.014
18. Bas E, Van De Water TR, Lumbreras V, Rajguru S, Goss G, Hare JM, et al. Adult human nasal mesenchymal-like stem cells restore cochlear spiral ganglion neurons after experimental lesion. Stem Cells Dev (2014) 23(5):502–14. doi:10.1089/scd.2013.0274
19. Duan D, Lu M. Olfactory mucosa: a rich source of cell therapy for central nervous system repair. Rev Neurosci (2015) 26(3):281–93. doi:10.1515/revneuro-2014-0065
20. Goldstein BJ, Hare JM, Lieberman S, Casiano R. Adult human nasal mesenchymal stem cells have an unexpected broad anatomic distribution. Int Forum Allergy Rhinol (2013) 3(7):550–5. doi:10.1002/alr.21153
21. Gu YZ, Xue Q, Chen YJ, Yu GH, Qing MD, Shen Y, et al. Different roles of PD-L1 and FasL in immunomodulation mediated by human placenta-derived mesenchymal stem cells. Hum Immunol (2013) 74(3):267–76. doi:10.1016/j.humimm.2012.12.011
22. Francisco LM, Sage PT, Sharpe AH. The PD-1 pathway in tolerance and autoimmunity. Immunol Rev (2010) 236:219–42. doi:10.1111/j.1600-065X.2010.00923.x
23. Latchman Y, Wood CR, Chernova T, Chaudhary D, Borde M, Chernova I, et al. PD-L2 is a second ligand for PD-1 and inhibits T cell activation. Nat Immunol (2001) 2(3):261–8. doi:10.1038/85330
24. Wang G, Zhang S, Wang F, Li G, Zhang L, Luan X. Expression and biological function of programmed death ligands in human placenta mesenchymal stem cells. Cell Biol Int (2013) 37(2):137–48. doi:10.1002/cbin.10024
25. Bassi Ê, Moraes-Vieira PM, Moreira-Sá CS, Almeida DC, Vieira LM, Cunha CS, et al. Immune regulatory properties of allogeneic adipose-derived mesenchymal stem cells in the treatment of experimental autoimmune diabetes. Diabetes (2012) 61(10):2534–45. doi:10.2337/db11-0844
26. Dighe PA, Viswanathan P, Mruthunjaya AK, Seetharam RN. Effect of bFGF on HLA-DR expression of human bone marrow-derived mesenchymal stem cells. J Stem Cells (2013) 8(1):43–57.
27. Aggarwal S, Pittenger MF. Human mesenchymal stem cells modulate allogeneic immune cell responses. Blood (2005) 105(4):1815–22. doi:10.1182/blood-2004-04-1559
28. Miraglia S, Godfrey W, Yin AH, Atkins K, Warnke R, Holden JT, et al. A novel five-transmembrane hematopoietic stem cell antigen: isolation, characterization, and molecular cloning. Blood (1997) 90(12):5013–21.
29. Weigmann A, Corbeil D, Hellwig A, Huttner WB. Prominin, a novel microvilli-specific polytopic membrane protein of the apical surface of epithelial cells, is targeted to plasmalemmal protrusions of non-epithelial cells. Proc Natl Acad Sci U S A (1997) 94(23):12425–30. doi:10.1073/pnas.94.23.12425
30. Yin AH, Miraglia S, Zanjani ED, Almeida-Porada G, Ogawa M, Leary AG, et al. AC133, a novel marker for human hematopoietic stem and progenitor cells. Blood (1997) 90(12):5002–12.
31. Chen Q, Zhang Z, Liu J, He Q, Zhou Y, Shao G, et al. A fibrin matrix promotes the differentiation of EMSCs isolated from nasal respiratory mucosa to myelinating phenotypical Schwann-like cells. Mol Cells (2015) 38(3):221–8. doi:10.14348/molcells.2015.2170
32. Servitja JM, Pignatelli M, Maestro MA, Cardalda C, Boj SF, Lozano J, et al. Hnf1alpha (MODY3) controls tissue-specific transcriptional programs and exerts opposed effects on cell growth in pancreatic islets and liver. Mol Cell Biol (2009) 29(11):2945–59. doi:10.1128/MCB.01389-08
33. Zeisberg M, Shah AA, Kalluri R. Bone morphogenic protein-7 induces mesenchymal to epithelial transition in adult renal fibroblasts and facilitates regeneration of injured kidney. J Biol Chem (2005) 280(9):8094–100. doi:10.1074/jbc.M413102200
34. Holgate ST, Holloway J, Wilson S, Bucchieri F, Puddicombe S, Davies DE. Epithelial-mesenchymal communication in the pathogenesis of chronic asthma. Proc Am Thorac Soc (2004) 1(2):93–8. doi:10.1513/pats.2306034
35. Bousquet J, Jacot W, Vignola AM, Bachert C, Van Cauwenberge P. Allergic rhinitis: a disease remodeling the upper airways? J Allergy Clin Immunol (2004) 113(1):43–9. doi:10.1016/j.jaci.2003.09.047
36. Van Bruaene N, Derycke L, Perez-Novo CA, Gevaert P, Holtappels G, De Ruyck N, et al. TGF-beta signaling and collagen deposition in chronic rhinosinusitis. J Allergy Clin Immunol (2009) 124(2):.e1–2. doi:10.1016/j.jaci.2009.04.013
37. Balsalobre L, Pezato R, Perez-Novo C, Alves MT, Santos RP, Bachert C, et al. Epithelium and stroma from nasal polyp mucosa exhibits inverse expression of TGF-β1 as compared with healthy nasal mucosa. J Otolaryngol Head Neck Surg (2013) 42:29. doi:10.1186/1916-0216-42-29
38. Peretto P, Cummings D, Modena C, Behrens M, Venkatraman G, Fasolo A, et al. BMP mRNA and protein expression in the developing mouse olfactory system. J Comp Neurol (2002) 451(3):267–78. doi:10.1002/cne.10343
39. Heino TJ, Hentunen TA. Differentiation of osteoblasts and osteocytes from mesenchymal stem cells. Curr Stem Cell Res Ther (2008) 3(2):131–45. doi:10.2174/157488808784223032
40. Aspenberg P. Stimulation of tendon repair: mechanical loading, GDFs and platelets. A mini-review. Int Orthop (2007) 31(6):783–9. doi:10.1007/s00264-007-0398-6
41. Oshin AO, Caporali E, Byron CR, Stewart AA, Stewart MC. Phenotypic maintenance of articular chondrocytes in vitro requires BMP activity. Vet Comp Orthop Traumatol (2007) 20(3):185–91.
42. Hogan M, Girish K, James R, Balian G, Hurwitz S, Chhabra AB. Growth differentiation factor-5 regulation of extracellular matrix gene expression in murine tendon fibroblasts. J Tissue Eng Regen Med (2011) 5(3):191–200. doi:10.1002/term.304
43. Park A, Hogan MV, Kesturu GS, James R, Balian G, Chhabra AB. Adipose-derived mesenchymal stem cells treated with growth differentiation factor-5 express tendon-specific markers. Tissue Eng Part A (2010) 16(9):2941–51. doi:10.1089/ten.tea.2009.0710
44. Goumans MJ, Valdimarsdottir G, Itoh S, Rosendahl A, Sideras P, ten Dijke P. Balancing the activation state of the endothelium via two distinct TGF-beta type I receptors. EMBO J (2002) 21(7):1743–53. doi:10.1093/emboj/21.7.1743
45. Schild-Poulter C, Shih A, Yarymowich NC, Haché RJ. Down-regulation of histone H2B by DNA-dependent protein kinase in response to DNA damage through modulation of octamer transcription factor 1. Cancer Res (2003) 63(21):7197–205.
46. Kerpedjieva SS, Kim DS, Barbeau DJ, Tamama K. EGFR ligands drive multipotential stromal cells to produce multiple growth factors and cytokines via early growth response-1. Stem Cells Dev (2012) 21(13):2541–51. doi:10.1089/scd.2011.0711
47. Hsu YC, Osinski J, Campbell CE, Litwack ED, Wang D, Liu S, et al. Mesenchymal nuclear factor I B regulates cell proliferation and epithelial differentiation during lung maturation. Dev Biol (2011) 354(2):242–52. doi:10.1016/j.ydbio.2011.04.002
Keywords: mesenchymal stromal cells, nasal polyposis, gene expression, immunoregulation, stem cells
Citation: Oliveira PWB, Pezato R, Agudelo JSH, Perez-Novo CA, Berghe WV, Câmara NO, de Almeida DC and Gregorio LC (2017) Nasal Polyp-Derived Mesenchymal Stromal Cells Exhibit Lack of Immune-Associated Molecules and High Levels of Stem/Progenitor Cells Markers. Front. Immunol. 8:39. doi: 10.3389/fimmu.2017.00039
Received: 02 September 2016; Accepted: 10 January 2017;
Published: 30 January 2017
Edited by:
Takayuki Yoshimoto, Tokyo Medical University, JapanReviewed by:
Peiman Hematti, University of Wisconsin-Madison, USATerumasa Umemoto, Kumamoto University, Japan
Copyright: © 2017 Oliveira, Pezato, Agudelo, Perez-Novo, Berghe, Câmara, de Almeida and Gregorio. This is an open-access article distributed under the terms of the Creative Commons Attribution License (CC BY). The use, distribution or reproduction in other forums is permitted, provided the original author(s) or licensor are credited and that the original publication in this journal is cited, in accordance with accepted academic practice. No use, distribution or reproduction is permitted which does not comply with these terms.
*Correspondence: Rogério Pezato, pezatobau@ig.com.br