- College of Medical and Dental Sciences, Institute of Immunology and Immunotherapy, University of Birmingham, Birmingham, UK
Fat-associated lymphoid clusters (FALCs) are atypical lymphoid tissues that were originally identified in mouse and human mesenteries due to that they contain a high number of type 2 innate lymphoid cells/nuocytes/natural helper cells. FALCs are located on adipose tissues in mucosal surfaces such as the mediastinum, pericardium, and gonadal fat. Importantly, these clusters contain B1, B2 and T lymphocytes as well as myeloid and other innate immune cell populations. The developmental cues of FALC formation have started to emerge, showing that these clusters depend on a different set of molecules and cells than secondary lymphoid tissues for their formation. Here, we review the current knowledge on FALC formation, and we compare FALCs and omental milky spots and their responses to inflammation.
Introduction
The interactions between different types of immune cells are essential for both innate and adaptive immune responses to pathogens. Such interactions require strategically situated microenvironments to increase the chances that rare antigen-specific lymphocytes become activated. These specialized microenvironments are found in secondary lymphoid organs (SLOs) such as lymph nodes and Peyer’s patches. Specialized populations of CD45− fibroblastic and endothelial cells express an arrangement of cell adhesion molecules, chemokines, and survival factors that guide the recruitment, co-localization and interactions of bone marrow-derived cells to their specific areas being T cells and dendritic cells (DCs) to the T cell area while B cells and follicular T helper cells to the B cell follicles. The development and organization of secondary lymphoid tissues is dependent on signals by the tumor necrosis factor (TNF) family of proteins such as lymphotoxin αβ (LTαβ), lymphotoxin β receptor (LTβR), and TNF-TNF receptor I and the downstream pathways such as activation of the nuclear factor kappa B family of transcription factors. The target genes of these pathways include the cell adhesion molecules VCAM-1, ICAM-1, MAdCAM-1, and the chemokines CXCL13, CCL19, and CCL21. Several detailed reviews of the processes that mediate lymph node development have been published (1–3).
Lymph nodes are characterized by well-defined B and T cell areas. B cell areas contain follicles that are organized by a specific population of reticular cell expressing the B cell-attracting chemokine CXCL13. Paracortical T cell areas are organized by a different type of stromal cells named T zone reticular cells that express CCL21 and CCL19, which attract T cells, and DCs to facilitate their interactions (4).
In addition to secondary lymphoid tissues such as lymph nodes, there are a series of inducible lymphoid tissues present in mucosal surfaces such as bronchial-associated lymphoid tissues (BALT) in the lung (5–9), gut-associated lymphoid tissues that comprise the isolated lymphoid follicles in the intestine (ILFs) (10–12), tear duct-associated lymphoid tissues (13, 14), nasopharyngeal-associated lymphoid tissues (NALT) (15, 16) and portal tract-associated lymphoid tissues (17, 18) to cite a few examples (see Table 1). Recently a novel type of lymphoid tissue called fat-associated lymphoid clusters (FALCs) has been identified in the mesenteries of humans and mice (19).
The Structure of FALCs
Fat-associated lymphoid clusters are non-classical lymphoid tissues associated to adipocytes in mucosal surfaces, including omental, mesenteric, mediastinal, gonadal, and pericardial fat (19, 24, 25).
The frequency of FALCs varies among different adipose tissues (AT) in mice. Whereas gonadal AT has as little as 1–2 clusters, the omentum can harbor up to 80 clusters per depot in homeostatic conditions (24). This heterogeneity is also reflected in FALC size, which ranges from 100 to 500 µm in diameter (19).
Unlike lymph nodes, FALCs are not encapsulated and are in direct contact with surrounding adipocytes (19). The arrangement of leukocytes found in FALCs also differs from the organization of conventional SLOs. For instance, no discernible B and T cell compartmentalization areas are evident. Instead, FALCs from mesenteric AT are composed of a tight cluster of B220+ or IgM+ B cells, with variable numbers of CD4+ T cells and CD11b+ myeloid cells (19, 24). Both myeloid cell precursors (CD31/ER-MP58+) and mature macrophages (F4/80+) have been detected in omental FALCs, suggesting that these lymphoid clusters form permissive microenvironments where the former cells can proliferate locally to be a source of free macrophages within the peritoneal cavity (32). A similar process is likely to take place in FALCs in the mediastinum and pericardium (33, 34). Importantly, FALCs contain type 2 innate lymphoid cells (ILC2) that can support the proliferation of B1 cells through the expression of IL-5 (19).
B cell recruitment to mesenteric FALCs requires the presence of a network of stromal cells expressing the chemokine CXCL13 (24). These cells are found scattered along the lymphoid clusters and are thought to be different from follicular dendritic cells (FDCs), which require signaling through LTβR to induce CXCL13 expression (35). Importantly, FALCs are present in Cxcl13−/− mice although they are devoid of B cells and their size is smaller than in their wild type littermates.
Fat-associated lymphoid clusters are highly vascularized as shown by their close association to blood vessels (19, 24). Moreover, lymphoid clusters in the omentum have also been found to contain high endothelial venules (HEVs), a specialized type of post-capillary venules essential for lymphocyte trafficking (30, 36). In contrast, FALCs connection with lymphatic vessels remains to be further investigated (24, 25, 27, 37). Earlier evidence has shown that FALCs in the omentum can collect antigens and particles directly from fluids within the peritoneal cavity (27).
Developmental Requirements for FALC Formation
Conventional SLOs such as lymph nodes and Peyer’s patches develop in a timely manner during embryogenesis, independently of pathogen-induced inflammation (38). In contrast, FALCs develop postnatally and could be identified at around 2–3 weeks after birth, reaching a plateau at around 18 weeks of age in mice (24). Unlike classical SLOs, FALC formation is not dependent on LTβR signaling as shown by the occurrence of these clusters in Ltβr−/− and Ltα−/− mice (24). Furthermore, FALCs also form in Rag2−/− and Rorc−/− mice, which lack B and T cells and LTi/ILC3 cells, respectively. FALCs are not the only lymphoid structures that develop independently of LTi cells and LTβR signaling, as other mucosal-associated lymphoid tissues, but also follow these developmental requirements (see Table 1) (9, 13, 16, 39).
Fat-associated lymphoid clusters are absent in Rag2−/−Il2rg−/− mice, which lack lymphocytes and ILCs, indicating a requirement of the latter for their development (24). Moreover, FALC formation is defective in Tnfrsf1a−/−Tnfrsf1b−/− mice, which lack TNF receptors (TNFR1 and TNFR2) (24). Further analysis has shown that AT macrophages are the main source of TNF and that stromal cells respond to this cytokine via TNFR, leading to FALC formation (Figure 1) (24).
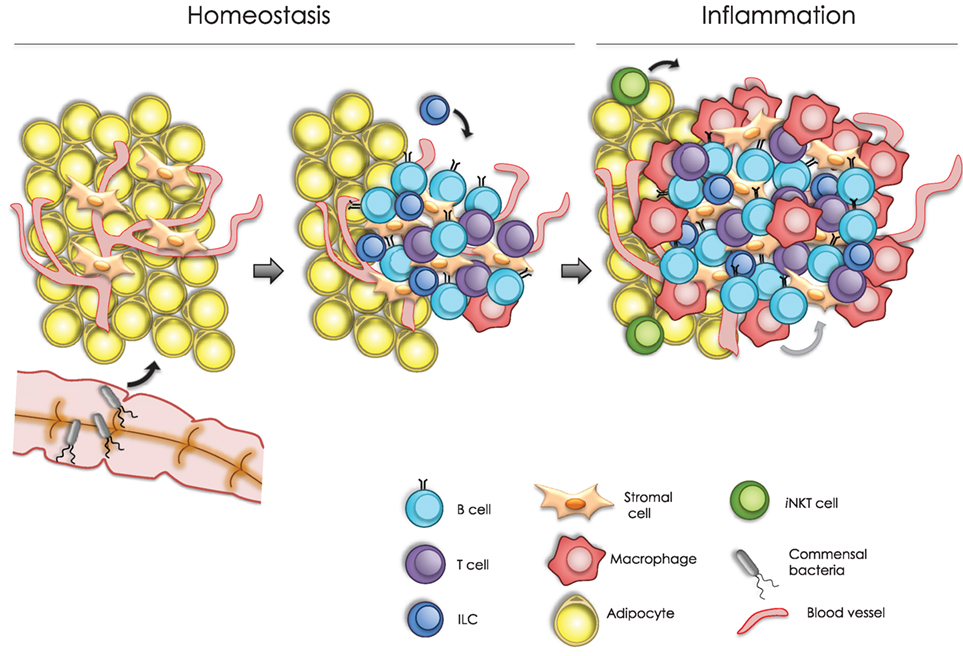
Figure 1. Model of fat associated lymphoid cluster (FALC) formation. In homeostasis, FALC formation can be modulated by different signals, including colonization by commensal microbiota, tumor necrosis factor (TNF) signaling, and type 2 innate lymphoid cells function. These signals may trigger cytokine production from local cells within the adipose tissue. For instance, CXCL13-expressing stromal cells can promote the recruitment of B cells into arranged lymphoid clusters. Following an inflammatory challenge, FALCs increase in number and size. Under these conditions, infiltrating macrophages are able to secrete TNF, which upon signaling through TNF receptors in stromal cells, promoting FALC growth. Moreover, invariant natural killer T cells stimulate FALC formation through the production of T helper type 2 cytokines, including IL-4 and IL-13, which may also promote the resolution of inflammation.
Fat-associated lymphoid cluster numbers are also greatly reduced in germ-free (GF) mice, suggesting a potential role of commensal flora in the formation of these structures (24). Interestingly, other lymphoid structures such as ILFs, which are found in the wall of the small intestine, are reduced or immature in GF mice (10). This suggests that similar to ILFs, the presence of commensal microbes or their by-products results in an inflammatory stimulus that induces the formation of FALCs. It will be interesting to test whether disruption of the intestinal epithelial barrier has an effect in the number and/or cellular composition of mesenteric FALCs (29).
Are FALCs Similar to Milky Spots (MS) of the Omentum?
Milky spots were first described by von Recklinghausen in 1863 as white spots in the omentum of rabbits (40). These structures were later characterized as highly vascularized accumulations of macrophages, B and T lymphocytes, and mast cells within the stroma of the greater omentum (41, 42). The omentum is generally divided in two parts, the greater and lesser omentum, depending on its position within the peritoneal cavity. Moreover, the omentum contains two distinguishable regions: a translucent collagenous membrane-like region and an adipose-rich region containing blood and lymphatic vessels, stromal cells, and clusters of immune cells (43). In mice and humans, MS have been found in the adipose region of the omentum, whereas in dogs, the aggregates of lymphoid cells are only found scattered in the translucent area (43, 44). However, no clear distinction in the exact location of MS within the omentum has been established. Interestingly, some authors have divided MS in two categories: vascularized and non-vascularized MS. Vascularized MS are supplied with blood vessels and are found in the omental fat region. On the other hand, non-vascularized MS do not have blood supply and are located in the omental membrane (45). Whether these features that translate into functional differences remain to be investigated.
A close examination showed no discernible differences between MS and the FALCs in mesenteries, mediastinum, and gonadal fat (see Table 2). In light of their similar characteristics but distinct locations and for clarity, we will be calling the omental MS as omFALCs while mesFALCs correspond to the clusters in the mesenteries, medFALCs the structures in the mediastinum, perFALC for the structures in the pericardium, and gonFALC the clusters present in gonadal fat.
FALC Respond to Inflammation and Immunization
MesFALCs and omFALCs increase in number and size in response to acute or chronic peritoneal inflammation (24, 27, 37). For instance, upon intraperitoneal (IP) injection of Zymosan, a yeast-derived ligand of Toll-like receptor 2, the abundance and size of mesFALCs increased significantly (24). Rag2−/− and Cd1d−/− mice failed to induce FALC formation in response to Zymosan-induced inflammation. Interestingly, IP injection of invariant natural killer T (iNKT) cells into Rag2−/− mice was able to restore mesFALC formation following Zymosan challenge, indicating a role of iNKT cells in this process (24). More specifically, activated iNKT cells can produce T helper type 2 (TH2) cytokines, including IL-4 and IL-13, which may have redundant roles in the formation of FALCs following peritoneal inflammation in the BALB/c strain background (Figure 1) (24).
Likewise, IP injection of lipid A, a component of bacterial lipopolysaccharide, leads to an increase in the number of B1 cells and macrophages in the omentum (29). Moreover, IP injection of polydextran particles or polyacrylamide beads increases the number and size of omFALC (37, 46). Different models of inflammation including TNF injection, Escherichia coli infection, and cecal ligation lead to the influx of neutrophils to the omFALC via HEVs (36).
Earlier evidence of the mechanisms by which FALCs respond to immunization comes from studies on immune responses in the omentum. Using an elegant mouse model that lacks spleen, lymph nodes, and Peyer’s patches (SLP mice), Rangel-Moreno, Randall, and colleagues have shown that omFALC are sufficient to respond to an immunological challenge and support T cell responses, immunoglobulin switching, and moderate affinity maturation (30). It is possible that the antigen-specific immune response shown in serum in SLP mice upon IP immunization that had been attributed to the omFALC may have a relative contribution from mesFALCs. Peritoneal immunization resulted in a marked recruitment of macrophages to the omFALC similar to what has been shown in mesFALCs (24). OmFALCs were shown to collect IP injected fluorophore-labeled antigens or GFP-labeled tumor cells. Peritoneal cell migration to the omentum is mediated by mechanisms that are both dependent and independent of chemokines, but clustering of B and T lymphocytes is dependent on the latter.
Macrophages are the most important cell type to engulf and eliminate bacteria in omFALCs (30). Indeed, it has been shown in a diet-induced obesity model that macrophages in gonFALC are able to proteolytically process antigen and present it in the context of MHC II complexes, indicating that they can function as antigen-presenting cells to induce T cell proliferation (47). Furthermore, IP immunizations with T-cell-dependent antigens showed that antigen-specific B cells undergo expansion, Ig switching, and acquired markers of GC reaction further indicating that adaptive immune responses take place in mesFALCs (24).
Mesenteric FALCs are also able to respond to intestinal helminth infection (Nippostrongylus brasiliensis) via ILC2 activation and expression of the TH2 cytokines IL-5 and IL-13. These cytokines are produced in response to IL-33 and IL-25 and promote goblet cell hyperplasia and helminth expulsion (19, 48). Likewise, a recent report has shown that medFALCs and perFALCs respond to both lung infection with the nematode Litomosoides sigmodontis and lung inflammation via fungal allergen inhalation (33). Under these conditions, medFALC stromal cells produce IL-33, which leads to ILC2 activation and IL-5 secretion. This in turn promotes the recruitment and activation of B cells and culminates in the production of natural IgM antibodies (Ab) for local protection (33).
A recent report has shown that medFALC are present in large numbers in two animal models of autoimmunity that develop a phenotype similar to human systemic lupus erythematosus (SLE) (49). The MRL/MpJ-lpr mouse model carries a mutation in the gene encoding Fas, a cell membrane receptor that induces caspase activation and apoptosis. This mutant strain develops a severe autoimmune syndrome with lymphadenopathy, splenomegaly, and the production of anti-double strand DNA (dsDNA) Ab as well as immune complex deposition in several organs including kidney, resembling human SLE. The BXSB/MpJ-Yaa mice developed systemic autoimmunity, with males being more affected due to a locus called autoimmune accelerator located in the Y chromosome. Both strains showed large medFALCs size that correlated with a significant increased level of immune cell infiltration in the lungs with respect to their control strains (49). It will be interesting to assess whether medFALCs in the MRL/MpJ-lpr model contained anti dsDNA Ab-forming B cells.
Several reports indicate a link between FALCs and cancer metastasis in the peritoneum. A study has shown a direct correlation between the presence and number of FALCs and the colonization of the clusters by ovarian cancer cells, with the omentum containing the largest number of FALCs and metastasis/foci of tumor cells (50). The foci formation by tumor cells in FALCs is not affected by the absence of lymphocytes, indicating a role for specialized stromal cells, endothelium, and adipocytes in the clusters that facilitate malignant cell recruitment. Similarly, IP injection of gastric and colon cancer cells resulted in their rapid migration and survival in omental and mesFALCs (51–53). These reports together with the evidence of metastasis formation in the omentum and peritoneal cavity for some human cancers (54) indicate the presence of a favorable microenvironment in FALCs that allows the tumor cells to thrive and form micrometastasis in these tissues. Along this line, some reports have shown that gastric, colon, and some liver cancer cells express the chemokine receptors CCR4 and CXCR4, whereas cell populations from omFALCs and mesenteries can express their ligands (CCL22 and CXCL12), which may favor their migration to these structures (51, 52, 55).
Conclusion
The rapid formation of FALCs following inflammation or infection, the changes in cellular composition and the presence of innate immune cells and B cells undergoing a germinal center reaction indicate a central role for these clusters in the formation of local immune responses.
Understanding what signals and cells are essential to FALC formation in homeostasis and following infection will allow inducing their formation to support antitumor responses. Conversely, it may be possible to reduce or preclude FALC formation during aberrant immune responses or peritoneal inflammation.
The physiological significance of the close association of FALCs with visceral AT remains to be further investigated. It is tempting to hypothesize that FALCs have an additional function in supporting type 2 ILCs and other immune cells that ultimately maintain the homeostasis of AT and whole body metabolism. Ultimately, understanding FALC formation in the mesenteries, omentum, and gonadal fat may facilitate the design of therapies to target low-grade chronic inflammation and other symptoms associated with obesity and metabolic syndrome.
Author Contributions
SC-M prepared the tables and figure. SC-M and JC wrote the manuscript.
Conflict of Interest Statement
The authors declare that the research was conducted in the absence of any commercial or financial relationships that could be construed as a potential conflict of interest.
Funding
This work was supported by a grant from the Biotechnology and Biological Sciences Research Council (BB/K009400/1) to JC and the College of Medical and Dental Sciences of the University of Birmingham.
References
1. Randall TD, Carragher DM, Rangel-Moreno J. Development of secondary lymphoid organs. Annu Rev Immunol (2008) 26:627–50. doi:10.1146/annurev.immunol.26.021607.090257
2. Ruddle NH, Akirav EM. Secondary lymphoid organs: responding to genetic and environmental cues in ontogeny and the immune response. J Immunol (2009) 183:2205–12. doi:10.4049/jimmunol.0804324
3. van de Pavert SA, Mebius RE. New insights into the development of lymphoid tissues. Nat Rev Immunol (2010) 10:664–74. doi:10.1038/nri2832
4. Fletcher AL, Acton SE, Knoblich K. Lymph node fibroblastic reticular cells in health and disease. Nat Rev Immunol (2015) 15:350–61. doi:10.1038/nri3846
5. Fleige H, Haas JD, Stahl FR, Willenzon S, Prinz I, Forster R. Induction of BALT in the absence of IL-17. Nat Immunol (2011) 13(1):1. doi:10.1038/ni.2167
6. Fleige H, Ravens S, Moschovakis GL, Bölter J, Willenzon S, Sutter G, et al. IL-17-induced CXCL12 recruits B cells and induces follicle formation in BALT in the absence of differentiated FDCs. J Exp Med (2014) 211(4):643–51. doi:10.1084/jem.20131737
7. Hwang JY, Randall TD, Silva-Sanchez A. Inducible bronchus-associated lymphoid tissue: taming inflammation in the lung. Front Immunol (2016) 7:258. doi:10.3389/fimmu.2016.00258
8. Kocks JR, Davalos-Misslitz AC, Hintzen G, Ohl L, Forster R. Regulatory T cells interfere with the development of bronchus-associated lymphoid tissue. J Exp Med (2007) 204:723–34. doi:10.1084/jem.20061424
9. Rangel-Moreno J, Carragher DM, de la Luz Garcia-Hernandez M, Hwang JY, Kusser K, Hartson L, et al. The development of inducible bronchus-associated lymphoid tissue depends on IL-17. Nat Immunol (2011) 12:639–46. doi:10.1038/ni.2053
10. Baptista AP, Olivier BJ, Goverse G, Greuter M, Knippenberg M, Kusser K, et al. Colonic patch and colonic SILT development are independent and differentially regulated events. Mucosal Immunol (2013) 6:511–21. doi:10.1038/mi.2012.90
11. Hamada H, Hiroi T, Nishiyama Y, Takahashi H, Masunaga Y, Hachimura S, et al. Identification of multiple isolated lymphoid follicles on the antimesenteric wall of the mouse small intestine. J Immunol (2002) 168:57–64. doi:10.4049/jimmunol.168.1.57
12. Lorenz RG, Chaplin DD, McDonald KG, McDonough JS, Newberry RD. Isolated lymphoid follicle formation is inducible and dependent upon lymphotoxin-sufficient B lymphocytes, lymphotoxin beta receptor, and TNF receptor I function. J Immunol (2003) 170:5475–82. doi:10.4049/jimmunol.170.11.5475
13. Nagatake T, Fukuyama S, Kim DY, Goda K, Igarashi O, Sato S, et al. Id2-, RORgammat-, and LTbetaR-independent initiation of lymphoid organogenesis in ocular immunity. J Exp Med (2009) 206:2351–64. doi:10.1084/jem.20091436
14. Paulsen FP, Paulsen JI, Thale AB, Tillmann BN. Mucosa-associated lymphoid tissue in human efferent tear ducts. Virchows Arch (2000) 437:185–9. doi:10.1007/s004280000248
15. Asanuma H, Thompson AH, Iwasaki T, Sato Y, Inaba Y, Aizawa C, et al. Isolation and characterization of mouse nasal-associated lymphoid tissue. J Immunol Methods (1997) 202:123–31. doi:10.1016/S0022-1759(96)00243-8
16. Fukuyama S, Hiroi T, Yokota Y, Rennert PD, Yanagita M, Kinoshita N, et al. Initiation of NALT organogenesis is independent of the IL-7R, LTbetaR, and NIK signaling pathways but requires the Id2 gene and CD3- CD4+ CD45+ cells. Immunity (2002) 17:31–40. doi:10.1016/S1074-7613(02)00339-4
17. Grant AJ, Goddard S, Ahmed-Choudhury J, Reynolds G, Jackson DG, Briskin M, et al. Hepatic expression of secondary lymphoid chemokine (CCL21) promotes the development of portal-associated lymphoid tissue in chronic inflammatory liver disease. Am J Pathol (2002) 160:1445–55. doi:10.1016/S0002-9440(10)62570-9
18. Yoneyama H, Matsuno K, Zhang Y, Murai M, Itakura M, Ishikawa S, et al. Regulation by chemokines of circulating dendritic cell precursors, and the formation of portal tract–associated lymphoid tissue, in a granulomatous liver disease. J Exp Med (2000) 193:35–49. doi:10.1084/jem.193.1.35
19. Moro K, Yamada T, Tanabe M, Takeuchi T, Ikawa T, Kawamoto H, et al. Innate production of T(H)2 cytokines by adipose tissue-associated c-Kit(+)Sca-1(+) lymphoid cells. Nature (2010) 463:540–4. doi:10.1038/nature08636
20. Moyron-Quiroz JE, Rangel-Moreno J, Kusser K, Hartson L, Sprague F, Goodrich S, et al. Role of inducible bronchus associated lymphoid tissue (iBALT) in respiratory immunity. Nat Med (2004) 10:927–34. doi:10.1038/nm1091
21. Velaga S, Herbrand H, Friedrichsen M, Jiong T, Dorsch M, Hoffmann MW, et al. Chemokine receptor CXCR5 supports solitary intestinal lymphoid tissue formation, B cell homing, and induction of intestinal IgA responses. J Immunol (2009) 182:2610–9. doi:10.4049/jimmunol.0801141
22. Harmsen A, Kusser K, Hartson L, Tighe M, Sunshine MJ, Sedgwick JD, et al. Cutting edge: organogenesis of nasal-associated lymphoid tissue (NALT) occurs independently of lymphotoxin-alpha (LT alpha) and retinoic acid receptor-related orphan receptor-gamma, but the organization of NALT is LT alpha dependent. J Immunol (2002) 168:986–90. doi:10.4049/jimmunol.168.3.986
23. Rangel-Moreno J, Moyron-Quiroz J, Kusser K, Hartson L, Nakano H, Randall TD. Role of CXC chemokine ligand 13, CC chemokine ligand (CCL) 19, and CCL21 in the organization and function of nasal-associated lymphoid tissue. J Immunol (2005) 175:4904–13. doi:10.4049/jimmunol.175.8.4904
24. Bénézech CC, Luu N-T, Walker JA, Kruglov AA, Loo Y, Nakamura K, et al. Inflammation-induced formation of fat-associated lymphoid clusters. Nat Immunol (2015) 16:819–28. doi:10.1038/ni.3215
25. Elewa YH, Ichii O, Otsuka S, Hashimoto Y, Kon Y. Characterization of mouse mediastinal fat-associated lymphoid clusters. Cell Tissue Res (2014) 357:731–41. doi:10.1007/s00441-014-1889-6
26. Shimotsuma M, Takahashi T, Kawata M, Dux K. Cellular subsets of the milky spots in the human greater omentum. Cell Tissue Res (1991) 264:599–601. doi:10.1007/BF00319049
27. DiPaolo N, Sacchi G, Garosi G, Sansoni E, Bargagli L, Ponzo P, et al. Omental milky spots and peritoneal dialysis – review and personal experience. Perit Dial Int (2005) 25:48–57.
28. Lumeng CN, Liu J, Geletka L, Delaney C, Delproposto J, Desai A, et al. Aging is associated with an increase in T cells and inflammatory macrophages in visceral adipose tissue. J Immunol (2011) 187:6208–16. doi:10.4049/jimmunol.1102188
29. Ha SA, Tsuji M, Suzuki K, Meek B, Yasuda N, Kaisho T, et al. Regulation of B1 cell migration by signals through Toll-like receptors. J Exp Med (2006) 203:2541–50. doi:10.1084/jem.20061041
30. Rangel-Moreno J, Moyron-Quiroz JE, Carragher DM, Kusser K, Hartson L, Moquin A, et al. Omental milky spots develop in the absence of lymphoid tissue-inducer cells and support B and T cell responses to peritoneal antigens. Immunity (2009) 30:731–43. doi:10.1016/j.immuni.2009.03.014
31. Krist LFG, Koenen H, Calame W, van der Harten JJ, van der Linden JC, Eestermans IL, et al. Ontogeny of milky spots in the human greater omentum – an immunochemical study. Anat Rec (1997) 249:399–404. doi:10.1002/(SICI)1097-0185(199711)249:3<399::AID-AR11>3.0.CO;2-J
32. Wijffels JF, Hendrickx RJ, Steenbergen JJ, Eestermans IL, Beelen RH. Milky spots in the mouse omentum may play an important role in the origin of peritoneal macrophages. Res Immunol (1992) 143:401–9. doi:10.1016/S0923-2494(05)80072-0
33. Jackson-Jones LH, Duncan SM, Magalhaes MS, Campbell SM, Maizels RM, McSorley HJ, et al. Fat-associated lymphoid clusters control local IgM secretion during pleural infection and lung inflammation. Nat Commun (2016) 7:12651. doi:10.1038/ncomms12651
34. Jenkins SJ, Ruckerl D, Cook PC, Jones LH, Finkelman FD, van Rooijen N, et al. Local macrophage proliferation, rather than recruitment from the blood, is a signature of TH2 inflammation. Science (2011) 332:1284–8. doi:10.1126/science.1204351
35. Ngo VN, Korner H, Gunn MD, Schmidt KN, Riminton DS, Cooper MD, et al. Lymphotoxin a/b and tumor necrosis factor are required for stromal cell expression of homing chemokines in B and T cell areas of the spleen. J Exp Med (1999) 189:403–12. doi:10.1084/jem.189.2.403
36. Buscher K, Wang H, Zhang X, Striewski P, Wirth B, Saggu G, et al. Protection from septic peritonitis by rapid neutrophil recruitment through omental high endothelial venules. Nat Commun (2016) 7:10828. doi:10.1038/ncomms10828
37. Litbarg NO, Gudehithlu KP, Sethupathi P, Arruda JA, Dunea G, Singh AK. Activated omentum becomes rich in factors that promote healing and tissue regeneration. Cell Tissue Res (2007) 328:487–97. doi:10.1007/s00441-006-0356-4
38. Carragher DM, Rangel-Moreno J, Randall TD. Ectopic lymphoid tissues and local immunity. Semin Immunol (2008) 20:26–42. doi:10.1016/j.smim.2007.12.004
39. Randall TD, Mebius RE. The development and function of mucosal lymphoid tissues: a balancing act with micro-organisms. Mucosal Immunol (2014) 7:455–66. doi:10.1038/mi.2014.11
40. Recklinghausen FV. Uber Eiter und Bindesgewebs-korperchen. Vir Arch Pathol Anat (1863) 28:157–66. doi:10.1007/BF01930779
41. Krist LFG, Eestermans IL, Steenbergen JJE, Hoefsmit ECM, Cuesta MA, Meyer S, et al. Cellular composition of milky spots in the human greater omentum: an immunochemical and ultrastructural study. Anat Rec (1995) 241:163–74. doi:10.1002/ar.1092410204
42. Yildirim A, Aktas A, Nergiz Y, Akkus M. Analysis of human omentum-associated lymphoid tissue components with S-100 – an immunohistochemical study. Rom J Morphol Embryol (2010) 51:759–64.
43. Wilkosz S, Ireland G, Khwaja N, Walker M, Butt R, Giorgio-Miller AD, et al. A comparative study of the structure of human and murine greater omentum. Anat Embryol (2005) 209:251–61. doi:10.1007/s00429-004-0446-6
44. Huyghe S, de Rooster H, Doom M, Van den Broeck W. The microscopic structure of the omentum in healthy dogs: the mystery unravelled. Anat Histol Embryol (2016) 45:209–18. doi:10.1111/ahe.12189
45. Takemori N, Hirai K, Onodera R, Saito N, Namiki M. Light and electron microscopic study of omental milky spots in New Zealand Black mice, with special reference to the extramedullary hematopoiesis. Anat Embryol (1994) 189:215–26. doi:10.1007/BF00239009
46. Shah S, Lowery E, Braun RK, Martin A, Huang N, Medina M, et al. Cellular basis of tissue regeneration by omentum. PLoS One (2012) 7:e38368. doi:10.1371/journal.pone.0038368
47. Morris DL, Cho KW, Delproposto JL, Oatmen KE, Geletka LM, Martinez-Santibanez G, et al. Adipose tissue macrophages function as antigen-presenting cells and regulate adipose tissue CD4+ T cells in mice. Diabetes (2013) 62:2762–72. doi:10.2337/db12-1404
48. von Moltke J, Ji M, Liang HE, Locksley RM. Tuft-cell-derived IL-25 regulates an intestinal ILC2-epithelial response circuit. Nature (2016) 529:221–5. doi:10.1038/nature16161
49. Elewa YH, Ichii O, Kon Y. Comparative analysis of mediastinal fat-associated lymphoid cluster development and lung cellular infiltration in murine autoimmune disease models and the corresponding normal control strains. Immunology (2016) 147:30–40. doi:10.1111/imm.12539
50. Clark R, Krishnan V, Schoof M, Rodriguez I, Theriault B, Chekmareva M, et al. Milky spots promote ovarian cancer metastatic colonization of peritoneal adipose in experimental models. Am J Pathol (2013) 183:576–91. doi:10.1016/j.ajpath.2013.04.023
51. Cao L, Cao X, Zhang Y, Sun XT. Omental milky spots in screening gastric cancer stem cells. Neoplasma (2011) 58:20–6. doi:10.4149/neo_2011_01_20
52. Kasagi Y, Harada Y, Morodomi Y, Iwai T, Saito S, Yoshida K, et al. Peritoneal dissemination requires an Sp1-dependent CXCR4/CXCL12 signaling axis and extracellular matrix-directed spheroid formation. Cancer Res (2016) 76:347–57. doi:10.1158/0008-5472.CAN-15-1563
53. Sedlacek AL, Gerber SA, Randall TD, van Rooijen N, Frelinger JG, Lord EM. Generation of a dual-functioning antitumor immune response in the peritoneal cavity. Am J Pathol (2013) 183:1318–28. doi:10.1016/j.ajpath.2013.06.030
54. Koppe MJ, Nagtegaal ID, de Wilt JH, Ceelen WP. Recent insights into the pathophysiology of omental metastases. J Surg Oncol (2014) 110:670–5. doi:10.1002/jso.23681
Keywords: lymphoid tissues, fat-associated lymphoid clusters, peritoneal inflammation, peritoneal immune responses, tertiary lymphoid structures
Citation: Cruz-Migoni S and Caamaño J (2016) Fat-Associated Lymphoid Clusters in Inflammation and Immunity. Front. Immunol. 7:612. doi: 10.3389/fimmu.2016.00612
Received: 08 October 2016; Accepted: 05 December 2016;
Published: 21 December 2016
Edited by:
Andreas Habenicht, Ludwig Maximilian University of Munich, GermanyReviewed by:
Mark Christopher Coles, University of York, UKAndrea Brendolan, San Raffaele Hospital (IRCCS), Italy
Copyright: © 2016 Cruz-Migoni and Caamaño. This is an open-access article distributed under the terms of the Creative Commons Attribution License (CC BY). The use, distribution or reproduction in other forums is permitted, provided the original author(s) or licensor are credited and that the original publication in this journal is cited, in accordance with accepted academic practice. No use, distribution or reproduction is permitted which does not comply with these terms.
*Correspondence: Sara Cruz-Migoni, cy5iLmNydXptaWdvbmlAYmhhbS5hYy51aw==;
Jorge Caamaño, ai5jYWFtYW5vQGJoYW0uYWMudWs=