- Institute of Medical Microbiology and Infection Control, University Hospital of Frankfurt, Frankfurt am Main, Germany
Overcoming the first line of the innate immune system is a general hallmark of pathogenic microbes to avoid recognition and to enter the human host. In particular, spirochetes belonging to the Borrelia burgdorferi sensu lato complex have developed various means to counter the immune response and to successfully survive in diverse host environments for a prolonged period of time. In regard to complement resistance, Borrelia utilize a plethora of immune evasion strategies involves capturing of host-derived complement regulators, terminating complement activation as well as shedding of cell-destroying complement complexes to manipulate and to expeditiously inhibit human complement. Owing to their mode of action, the interacting surface-exposed proteins identified among B. burgdorferi sensu stricto (s.s.), Borrelia afzelii, Borrelia spielmanii, and Borrelia bavariensis can be classified into at least two major categories, namely, molecules that directly interfere with distinct complement components including BBK32, CspA, BGA66, BGA71, and a CD59-like protein or molecules, which indirectly counteract complement activation by binding various complement regulators such as Factor H, Factor H-like protein 1 (FHL-1), Factor H-related proteins FHR-1, FHR-2, or C4Bp. The latter group of genetically and structurally unrelated proteins has been collectively referred to as “complement regulator-acquiring surface proteins” and consists of CspA, CspZ, ErpA, ErpC, ErpP, and the as yet unidentified protein p43. This review focuses on the current knowledge of immune evasion mechanisms exhibited by Lyme disease spirochetes and highlights the role of complement-interfering, infection-associated molecules playing an important part in these processes. Deciphering the immune evasion strategies may provide novel avenues for improved diagnostic approaches and therapeutic interventions.
Introduction
The genus Borrelia (B.) comprises the causative agents of Lyme disease (LD) and relapsing fever (1–3). Concerning LD spirochetes, there are distinct species belonging to the Borrelia burgdorferi sensu lato complex of which six species including B. burgdorferi sensu stricto (s.s.), Borrelia afzelii, Borrelia garinii, Borrelia spielmanii, Borrelia bavariensis (formerly referred to as B. garinii OspA serotype 4), as well as candidatus Borrelia mayonii, are associated with human LD (4, 5). While Borrelia valaisiana, Borrelia lusitaniae, and Borrelia bissettii have been detected in human biopsies, the pathogenicity of these and the remaining borrelial species remains largely unclear (6–8).
To survive and establish a persistent infection in the human host, pathogens must evade the first line of host defense by counteracting complement as an essential part of innate immunity. This powerful surveillance system comprises a network of precursors, regulatory and inhibitory proteins that can be immediately activated upon recognition of invading microorganisms (9, 10). Despite the effectiveness and abundance of complement, LD spirochetes are able to overcome its destructive defense mechanisms (11–13). While attempting to decipher the molecular mechanisms of complement evasion, distinct complement-interfering and -inhibiting molecules of B. burgdorferi s.s., B. afzelii, B. spielmanii, and B. bavariensis have been identified (14, 15). This review focuses on the current knowledge of the molecular principles utilized by LD spirochetes to counteract complement at certain activation levels and on the borrelial proteins known to take part in complement inactivation.
Activation and Regulation of the Complement System
Complement operates as a cooperative network of inactive precursor molecules, fluid-phase and membrane-bound regulators, and inhibitors (16–20). The initiation of complement takes place in a cascade-like manner through three activation routes: the classical (CP), the lectin (LP), and the alternative pathway (AP), all of which converge in the generation of the highly reactive molecule, C3b (17, 19, 21, 22). The CP can be activated after binding of C1q to immune complexes (IgM and IgG) or charged molecules on the bacterial surface (23). In complex with C1q and C1r, C1s mediates cleavage of C4 and C2 leading to the formation of the C3 convertase, C4b2b. Activation of the LP is initiated by binding of mannan-binding lectin (MBL), ficolins (H-ficolin, L-ficolin, and M-ficolin), or collectins associated with MBL-associated serine proteases (MASP), to carbohydrates of microbial origin. After activation of MASP-2 by MASP-1, both proteases cleave C2 while MASP-2 is able to also cleave C4 to generate the identical C3 convertase. Finally, the AP is initiated by spontaneous hydrolysis of C3 followed by binding of C3b (opsonization) to different targets on the bacterial surface. Recruitment of Factor B (FB) followed by Factor D (FD)-mediated cleavage results in the formation of the membrane-bound C3 convertase C3bBb. To extend the half-life and to trigger the amplification of C3b (feedback loop), the C3 convertases of the AP are stabilized by properdin. Of note, deposition of large quantities of C3b on microbial surfaces is a prerequisite for opsonization and phagocytosis of invading pathogens. Upon binding of newly generated C3b molecules, the C4b2b and C3Bb complexes serve as precursors for the C5 convertases C4b2b3b and C3bBb3b. Cleavage of C5 into C5a and C5b by the C5 convertases initiates the unidirectional, sequential binding of the late components C6, C7, and C8 to C5b. Once the C5b–8 complex is formed, polymerization of multiple C9 molecules ensues, finally generating the pore-forming terminal complement complex [C5b–9, TCC, also referred to as membrane attack complex (MAC)], which promotes lysis of susceptible cells (19, 20). To avoid the detrimental effects of excessive complement activation, this surveillance system is tightly controlled by soluble and membrane-anchored regulators (21). The soluble regulators of the CP and LP include C1 esterase inhibitor (C1-INH) and C4b-binding protein (C4Bp), while the AP is primarily regulated by Factor H (FH) and Factor H-like protein 1 (FHL-1). Vitronectin, clusterin, and, in part, FH-related protein 1 (FHR-1) comprise the regulatory proteins of the terminal activation sequence (21, 24).
Diversity in Complement Susceptibility
Initial investigations showed that LD spirochetes differ substantially in their susceptibility to human serum and finally led to the classification of spirochetes into three main categories, serum-resistant, intermediately serum-resistant/sensitive or partially resistant, and serum-sensitive (25–27). It is worth mentioning that categorizing of spirochetes in these particular groups largely depends on technical parameters, e.g., serum collection and storage, serum and cell concentrations, incubation period, and the method of choice used to determine borrelial survival, making it difficult to compare the data published. Changing the experimental conditions can lead to differences in the phenotypic classification, in particular of intermediately serum-resistant/sensitive strains. Among LD spirochetes, B. burgdorferi s.s., B. afzelii, B. spielmanii, B. bavariensis, and Borrelia japonica are resistant to complement-mediated killing, B. bissettii was classified as intermediately serum-resistant and B. garinii, B. valaisiana, and B. lusitaniae comprise the group of highly susceptible spirochetes (13, 25–35). Furthermore, differences in serum susceptibility have been reported among certain B. valaisiana and B. garinii isolates (29, 36). Strikingly, the serum susceptibility pattern of LD spirochetes almost matches pathogenicity in humans with the exception of B. garinii known to frequently cause LD. The underlying molecular principles of how B. garinii circumvent complement-mediated killing are largely unknown and are still a matter of controversy. It is likely that pathogen-associated factors produced solely in the infected host or that interaction with host or tick-derived proteins upon the transmission process, e.g., plasminogen (37), Tick Salivary Lectin Pathway Inhibitor (TSLPI) (38), or Salp20 (39), may protect B. garinii from complement attack.
Borrelial Proteins Interacting with Human Complement Regulators
Inactivation of the Alternative Pathway by Binding Complement Regulators FH and FHL-1
In 1997, two independent studies demonstrated that serum-resistant strains exhibit significantly lower amounts of deposited activation products (C3, C6, and TCC) compared to serum-susceptible strains, leading to the assumption that the complement cascade is inhibited at the level of C3 and/or C5 activation; however, no underlying mechanism(s) were elucidated (26, 27). Several years later in 2001, OspE of B. burgdorferi s.s. (40) and the so-called Complement Regulator-Acquiring Surface Proteins (CRASP) of B. burgdorferi s.s. and B. afzelii (41, 42) were identified as ligands for FH and, in part, for FHL-1. Binding of these complement regulators by serum-resistant spirochetes inhibits activation at the central step of the complement cascade, C3 activation and the formation of C3 convertase, and thereby terminates the assembly and finally the integration of the TCC into the bacterial membrane (28, 42) (Figure 1A). Thereafter, several FH-binding proteins were detected in serum-resistant B. spielmanii, B. japonica, and B. bissettii isolates (31, 43, 44), while serum-sensitive B. garinii, B. lusitaniae, and B. valaisiana isolates did not bind functionally active FH (28, 30, 36, 42). The importance of disrupting complement activation at the level of C3 by surface-bound FH and FHL-1 was confirmed both in initial and follow-up studies investigating different borrelial species (29, 31–33). With the exception of serum-resistant B. bavariensis (45), almost all serum-resistant borrelial species are able to co-opt human FH and FHL-1 to protect themselves from complement-mediated killing, allowing LD spirochetes to survive in humans and in diverse, immune competent animal hosts (13).
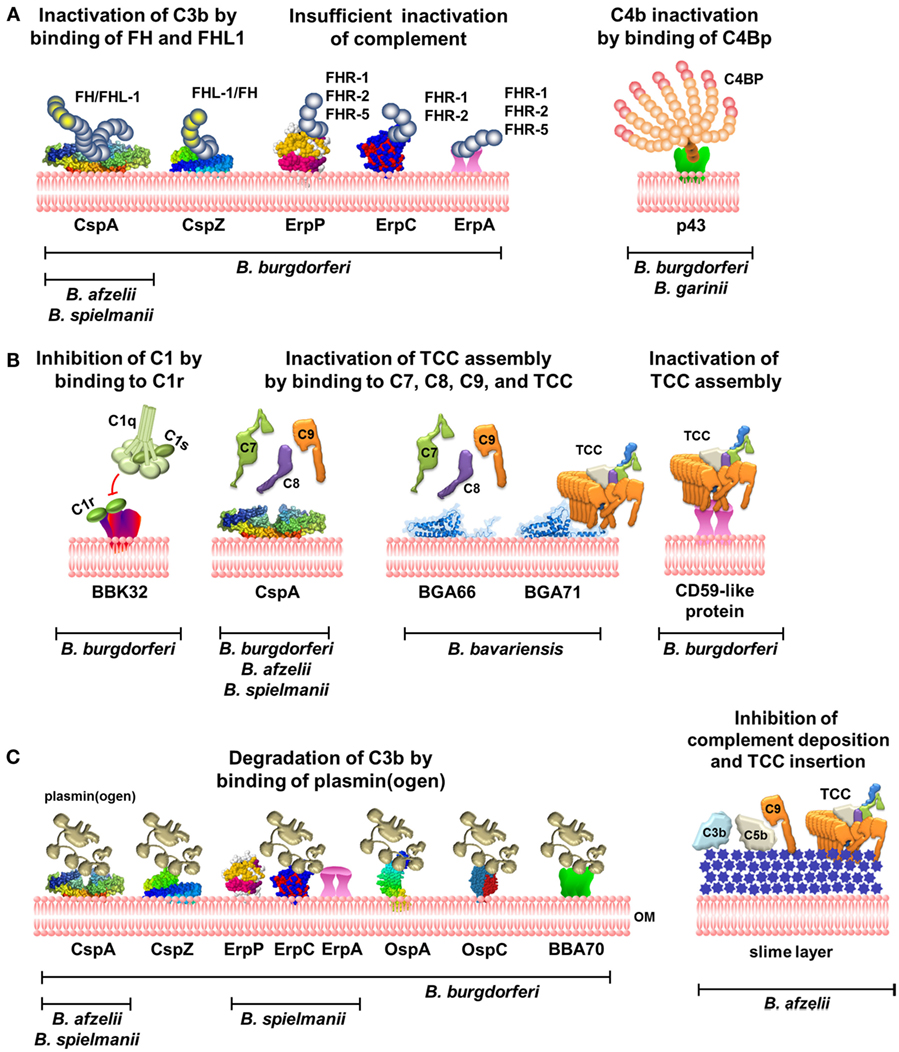
Figure 1. Complement evasion strategies of LD spirochetes. (A) Inhibition of the AP and CP by binding of complement regulators FH and FHL-1 to CspA and CspZ or C4Bp to p43. Binding of FHRs to ErpP, ErpC, and ErpA does not terminate complement activation. (B) Inhibition of the CP and TP by direct interaction of diverse borrelial proteins produced by distinct genospecies with C1r or late complement components. (C) Inactivation of C3b by binding of plasmin(ogen) by diverse borrelial proteins and prevention of complement deposition by the production of a mucoid layer. OM, outer membrane; TCC, terminal complement complex; FH, Factor H; C4Bp, C4b-binding protein.
Concerning the FH/FHL-1/FHR interacting molecules, up to five distinct outer surface lipoproteins, collectively termed CRASP, have been identified, comprising three genetically unrelated groups with partially overlapping biological functions (Table 1) (14, 15, 41). Owing to the genetic composition, different combinations of these proteins can be exposed at the surface of a particular isolate. For historical reasons, a variety of names have been introduced at the time of description and are still found in the literature, leading to considerable confusion about their identities and biological functions. As a simplification, the synonyms and additional designations of the protein and gene names of CRASPs along with their specific functional properties are summarized in Table 1. With regard to the reference type strain B. burgdorferi s.s. B31, the identified FH/FHL-1/FHR-binding proteins consists of CspA (CRASP-1, BBA68), CspZ (CRASP-2, BBH06), ErpP (CRASP-3, BBN38), ErpC (CRASP-4), and ErpA (CRASP-5, BBP38) (14). Due to their different functions, structures, gene organization, etc., OspE homologous proteins are collectively referred to as OspE-related proteins (Erp) proteins (46).
CspA is the predominant FH and FHL-1 binding protein of B. burgdorferi s.s. and belongs to the paralogous protein family PFam54, of which 11 paralogs are produced in strain B31. Except for CspA, none of the other PFam54 members interact with FH and FHL-1, despite the high sequence homology, suggesting that these proteins possess other, as yet unknown functions (47). Moreover, irrespective of geographical origin, CspA paralogs among B. burgdorferi s.s. isolates are highly conserved (48). More importantly, structure refinements have disclosed a homodimer as the biologically relevant architecture of CspA (Figure 1A) (49). Although sequence differences within the C-terminal region may account for the inability of CspA paralogs to bind FH and FHL-1, further investigations are necessary to satisfactorily clarify this issue. Initial studies revealed a strong binding affinity of both complement regulators to CspA, accompanied by a powerful capacity to inactivate C3b in the presence of Factor I (50, 51) (Figure 1A). The importance of CspA in facilitating complement resistance of B. burgdorferi s.s. has been clearly demonstrated by generating a cspA-deficient mutant and strains complemented with the cspA gene (52–54). More recently, CspA has been demonstrated to possess additional functions: this protein directly interacts with components of the terminal pathway (C7, C8, and C9) as well as plasmin(ogen), thereby terminating TCC assembly and upon activation to plasmin also promoting degradation of C3b (54, 55) (Figures 1B,C). CspA orthologs, sharing identical biological functions, were also identified in B. afzelii and B. spielmanii. All of these orthologs belong to the PFam54 protein family, but the loci of the encoding genes differ from cspA of B. burgdorferi s.s. The orthologs display the same inactivating properties as CspA, impart resistance to complement-mediated killing, and bind complement components FH, FHL-1, C7, C8, and C9 as well as plasmin(ogen) (43, 55–57). These findings suggest that CspA is an important serum resistance factor of B. burgdorferi s.s., B. afzelii, and B. spielmanii. CspA is produced during tick feeding, shortly after transmission to the mammalian host and during transmission to feeding, naïve ticks but not in the midgut of unfed ticks, suggesting that CspA protect spirochetes from complement attack during established infection (58, 59).
Borrelia burgdorferi s.s. produces an additional FH and FHL-1-binding protein, CspZ, which independently provides borrelial cells with resistance to human complement (60, 61) (Figure 1A; Table 1). Once FH or FHL-1 binds to the CspZ-producing spirochetes, termination of the complement cascade takes place at the activation level of C3 as demonstrated by the decay of the C3 convertase, an increase of C3b degradation products, and the lack of deposited TCC (60, 61). Although cspZ sequences were identified in numerous genospecies associated with LD, including B. afzelii, B. garinii, B. spielmanii, B. bavariensis, and B. bissettii, none of the other CspZ proteins interact with FH and FHL-1 (62–65). Although CspZ is produced during mammalian infection and elicits a robust antibody response (66), additional studies revealed that this protein does not protect mice from infections and, if at all, is only partially required for infection (63, 67, 68). In addition, CspZ like CspA has been identified as a plasmin(ogen)-binding molecule, enabling B. burgdorferi s.s. to degrade surface-bound C3 and C3b (57) (Figure 1C). Of note, the efficacy in degrading C3/C3b by plasmin is much less pronounced, compared to the C3b inactivation capacity of FH and FHL-1 (55). In the case of strong complement activation initiated by the AP, generation of high amounts of C3b cannot be sufficiently inactivated by surface-bound plasmin; thus, more adequate inhibitors such as FH or FHL-1 are required to overcome the feedback loop.
Lyme disease spirochetes produce a number of polymorphic proteins belonging to the OspE/F paralogous protein family PFam162, of which ErpA (BBP38), ErpC, and ErpP (BBN38) have been reported to bind FH as well as FHR-1, FHR-2, and in part FHR-5 (40, 50, 69–74) (Figure 1A; Table 1). Despite binding of FH to purified Erp proteins, there are several lines of evidence indicating that the same molecules, when exposed to the bacterial surface, do not confer protection of LD spirochetes from deposition of C3 and TCC (14, 70, 74). Spirochetes producing Erp proteins, but lacking CspA and CspZ, display a susceptible phenotype and are readily killed by complement (52, 70, 74). Owing to the Erp proteins strong affinity for FHRs (50), FH might be displaced from the bacterial surface, with a concurrent loss of its complement regulatory functions and, as such, is unable to protect the cells from the deleterious effects of complement.
Besides binding of complement components, ErpA, ErpC, and ErpP, as well as other Erp orthologs, are known to serve as potential ligands for plasmin(ogen) (Figure 1C) (75, 76). As mentioned previously, the role of activated plasmin in complement evasion of LD spirochetes requires further investigations. Although additional FH-binding Erp orthologs were identified in in vitro cultivated B. garinii, Borrelia andersonii, B. japonica, Borrelia turdi, and Borrelia tanukii isolates (44, 72, 77), the impact of these molecules on complement resistance has never been confirmed, in particular Erp proteins of B. garinii. Concerning additional FH/FHL-1-binding proteins, no data are currently available on other LD Borrelia species.
Inactivation of the Classical Pathway by Binding Complement Regulator C4Bp
The role of C4Bp, the key regulator of the CP, in immune evasion of LD spirochetes is still a matter of controversy. Pietikainen et al. have observed binding of C4Bp in serum-resistant B. burgdorferi s.s. and B. afzelii as well as in serum-sensitive B. garinii isolates (78) (Figure 1A). C4Bp bound to the borrelial surface in concert with Factor I maintained its complement regulatory activity and inactivated C4b (78). Along with the determination of C4Bp binding, a 43-kDa protein, tentatively designated p43 was identified in B. burgdorferi strains B31 and N40, and B. garinii strains g46 and g50 (78). However, other studies failed to show C4Bp binding by B. burgdorferi s.s. LW2, B. garinii G1, B. valaisiana (n = 3), and B. bavariensis strains (n = 8) (36, 45), possibly due to different techniques or antibodies used for the detection of C4Bp. In addition, taking into consideration that B. garinii cells are killed in serum concentrations >20%, the physiological relevance of C4Bp in promoting complement resistance remains to be determined.
Borrelial Proteins Displaying Complement-Inhibitory Activity
More recently, a novel immune evasion mechanism has been described by which B. burgdorferi s.s. specifically blocks CP activation (79). This study depicts BBK32 as the first protein that binds to C1r in a non-covalent manner and thereby preventing autocatalysis of this proenzyme and subsequently the cleavage of C1s, leaving the C1q complex in an inactive enzymatic state (Figure 1B; Table 1). By interfering with C1r, BBK32 acts as a potent inhibitor of the CP without affecting the LP and AP.
Unlike other serum-resistant LD spirochetes, B. bavariensis binds neither FH/FHL-1 nor other complement regulators such as C4Bp or C1-Inhibitor (45). Detailed analysis revealed two proteins, BGA66 and BGA71 as novel complement inhibitors. They belong to the PFam54 protein family and share 51 and 41% sequence identities, respectively, to CspA. Both proteins interact with components of the terminal pathway, in particular C7, C8, and C9, and also with the assembled TCC (45) (Figure 1B; Table 1). Binding of the borrelial proteins to various components of the terminal pathway affects TCC formation by (i) inhibiting C9 auto-polymerization, (ii) terminating TCC assembly, and (iii) preventing integration of the functional pore-forming complex. Moreover, BGA66 and BGA71 are simultaneously produced in all B. bavariensis strains investigated, but each protein by itself displays anti-complement activity and renders transformed spirochetes resistant to complement-mediated killing. Despite the structural similarities to CspA, BGA66 and BGA71 do not bind the potent complement regulators FH and FHL-1 (45). One might speculate that termination of the final activation steps may result in a somewhat weaker complement inactivation capacity. However, this does not appear to be the case as CspA and CspZ-producing and FH/FHL-1-binding spirochetes did not show a different resistance phenotype compared to BGA66- or BGA71-producing cells, indicating that inhibition of the terminal pathway is as efficient as blocking complement activation at the level of C3.
Borrelia burgdorferi s.s. also produces a CD59-like protein, which preferentially bind to C9 and to some extent to the β-subunit of C8 (80) (Figure 1B; Table 1). By using anti-CD59 antibodies, the functional activity of this protein could be blocked, rendering the spirochetes susceptible to complement-mediated killing. Although this surface-exposed protein has never been identified, the binding properties of the CD59-like protein suggest a role in inactivating the terminal pathway of complement. Despite the overlapping complement-inhibitory activities, the CD59-like protein is not identical with BGA66 and BGA71.
Further Proteins and Structures Involved in Complement Resistance of LD Spirochetes
Besides the already mentioned molecules, which interacting with complement in multiple ways, additional proteins have been described that also bind human plasmin(ogen), e.g., OspA, OspC, and BBA70 (81–83) (Figure 1C). For the latter, degradation of C3 and, in part, C5 has been demonstrated. Whether the interactions of OspC and BBA70, known to be expressed in the mammalian host, might support complement inactivation in vivo is not known.
Initial studies of the molecular principles of complement resistance, focusing on the amounts of deposited complement components, revealed an amorphous structure of high density that surrounds the entire cell envelope and, apparently, acts as a physical barrier, preventing the insertion of the formed TCC into the bacterial membrane of serum-resistant cells (Figure 1C) (32). Currently, no data are available on the composition and content of this so-called “slime layer” and whether this structure is present in LD spirochetes other than B. afzelii (32). Thus, further studies are required to verify the precise nature of this morphological substance.
Future Directions
Over the last decades, numerous molecules have been identified that interact with the innate immune system in multiple ways to influence or terminate complement at distinct activation levels, e.g., initiation of the CP, C3 activation by the AP, and formation of the TCC. Knowledge of the proteins involved in the interaction with complement has allowed for a better understanding of molecular principles of complement evasion developed by LD spirochetes. Future investigations will undoubtedly identify additional complement-interacting molecules required for evading the innate immune response of different animals, including reservoir hosts, and provide insight into whether these proteins might function in a host-specific manner during the infection process.
Author Contributions
PK prepared the figure and table and wrote the manuscript.
Conflict of Interest Statement
The author declares that the research was conducted in the absence of any commercial or financial relationships that could be construed as a potential conflict of interest.
Acknowledgments
The author thanks Arno Koenigs and Yi-Pin Lin for critical reading and helpful suggestions on the manuscript.
References
1. Stanek G, Reiter M. The expanding Lyme Borrelia complex-clinical significance of genomic species? Clin Microbiol Infect (2011) 17(4):487–93. doi:10.1111/j.1469-0691.2011.03492.x
2. Cutler SJ. Relapsing fever – a forgotten disease revealed. J Appl Microbiol (2010) 108(4):1115–22. doi:10.1111/j.1365-2672.2009.04598.x
3. Radolf JD, Caimano MJ, Stevenson B, Hu LT. Of ticks, mice and men: understanding the dual-host lifestyle of Lyme disease spirochaetes. Nat Rev Microbiol (2012) 10(2):87–99. doi:10.1038/nrmicro2714
4. Pritt BS, Mead PS, Johnson DK, Neitzel DF, Respicio-Kingry LB, Davis JP, et al. Identification of a novel pathogenic Borrelia species causing Lyme borreliosis with unusually high spirochaetaemia: a descriptive study. Lancet Infect Dis (2016) 16(5):556–64. doi:10.1016/S1473-3099(15)00464-8
5. Franke J, Hildebrandt A, Dorn W. Exploring gaps in our knowledge on Lyme borreliosis spirochaetes – updates on complex heterogeneity, ecology, and pathogenicity. Ticks Tick Borne Dis (2013) 4(1–2):11–25. doi:10.1016/j.ttbdis.2012.06.007
6. Diza E, Papa A, Vezyri E, Tsounis S, Milonas I, Antoniadis A. Borrelia valaisiana in cerebrospinal fluid. Emerg Infect Dis (2004) 10(9):1692–3. doi:10.3201/eid1009.030439
7. Rijpkema SG, Tazelaar DJ, Molkenboer MJ, Noordhoek GT, Plantinga G, Schouls LM, et al. Detection of Borrelia afzelii, Borrelia burgdorferi sensu stricto, Borrelia garinii and group VS116 by PCR in skin biopsies of patients with erythema migrans and acrodermatitis chronica atrophicans. Clin Microbiol Infect (1997) 3(1):109–16. doi:10.1111/j.1469-0691.1997.tb00259.x
8. Rudenko N, Golovchenko M, Mokracek A, Piskunova N, Ruzek D, Mallatova N, et al. Detection of Borrelia bissettii in cardiac valve tissue of a patient with endocarditis and aortic valve stenosis in the Czech Republic. J Clin Microbiol (2008) 46(10):3540–3. doi:10.1128/JCM.01032-08
9. Lambris JD, Ricklin D, Geisbrecht BV. Complement evasion by human pathogens. Nat Rev Micro (2008) 6(2):132–42. doi:10.1038/nrmicro1824
10. Berends ET, Kuipers A, Ravesloot MM, Urbanus RT, Rooijakkers SH. Bacteria under stress by complement and coagulation. FEMS Microbiol Rev (2014) 38(6):1146–71. doi:10.1111/1574-6976.12080
11. de Taeye SW, Kreuk L, van Dam AP, Hovius JW, Schuijt TJ. Complement evasion by Borrelia burgdorferi: it takes three to tango. Trends Parasitol (2013) 29(3):119–28. doi:10.1016/j.pt.2012.12.001
12. Embers ME, Ramamoorthy R, Philipp MT. Survival strategies of Borrelia burgdorferi, the etiologic agent of Lyme disease. Microbes Infect (2004) 6(3):312–8. doi:10.1016/j.micinf.2003.11.014
13. Kurtenbach K, De Michelis S, Etti S, Schafer SM, Sewell HS, Brade V, et al. Host association of Borrelia burgdorferi sensu lato-the key role of host complement. Trends Microbiol (2002) 10(2):74–9. doi:10.1016/S0966-842X(01)02298-3
14. Kraiczy P, Stevenson B. Complement regulator-acquiring surface proteins of Borrelia burgdorferi: structure, function and regulation of gene expression. Ticks Tick Borne Dis (2013) 4(1–2):26–34. doi:10.1016/j.ttbdis.2012.10.039
16. Walport MJ. Complement – second of two parts. N Engl J Med (2001) 344(15):1140–4. doi:10.1056/nejm200104123441506
17. Walport MJ. Complement – first of two parts. N Engl J Med (2001) 344(14):1058–66. doi:10.1056/nejm200104053441406
18. Zipfel PF. Complement and Immune defense: from innate immunity to human diseases. Immunol Lett (2009) 126:1–7. doi:10.1016/j.imlet.2009.07.005
19. Ricklin D, Hajishengallis G, Yang K, Lambris JD. Complement: a key system for immune surveillance and homeostasis. Nat Immunol (2010) 11(9):785–97. doi:10.1038/ni.1923
20. Trouw LA, Daha MR. Role of complement in innate immunity and host defense. Immunol Lett (2011) 138(1):35–7. doi:10.1016/j.imlet.2011.02.014
21. Zipfel PF, Skerka C. Complement regulators and inhibitory proteins. Nat Rev Immunol (2009) 9(10):729–40. doi:10.1038/nri2620
22. Merle NS, Church SE, Fremeaux-Bacchi V, Roumenina LT. Complement system part I – molecular mechanisms of activation and regulation. Front Immunol (2015) 6:262. doi:10.3389/fimmu.2015.00262
23. Kishore U, Ghai R, Greenhough TJ, Shrive AK, Bonifati DM, Gadjeva MG, et al. Structural and functional anatomy of the globular domain of complement protein C1q. Immunol Lett (2004) 95(2):113–28. doi:10.1016/j.imlet.2004.06.015
24. Zipfel PF, Heinen S, Jozsi M, Skerka C. Complement and diseases: defective alternative pathway control results in kidney and eye diseases. Mol Immunol (2006) 43(1–2):97–106. doi:10.1016/j.molimm.2005.06.015
25. Brade V, Kleber I, Acker G. Differences of two Borrelia burgdorferi strains in complement activation and serum resistance. Immunobiology (1992) 185(5):453–65. doi:10.1016/S0171-2985(11)80087-2
26. Breitner-Ruddock S, Würzner R, Schulze J, Brade V. Heterogeneity in the complement-dependent bacteriolysis within the species of Borrelia burgdorferi. Med Microbiol Immunol (1997) 185(4):253–60. doi:10.1007/s004300050038
27. van Dam AP, Oei A, Jaspars R, Fijen C, Wilske B, Spanjaard L, et al. Complement-mediated serum sensitivity among spirochetes that cause Lyme disease. Infect Immun (1997) 65(4):1228–36.
28. Alitalo A, Meri T, Ramo L, Jokiranta TS, Heikkila T, Seppala IJT, et al. Complement evasion by Borrelia burgdorferi: serum-resistant strains promote C3b inactivation. Infect Immun (2001) 69(6):3685–91. doi:10.1128/iai.69.6.3685-3691.2001
29. Bhide MR, Travnicek M, Levkutova M, Curlik J, Revajova V, Levkut M. Sensitivity of Borrelia genospecies to serum complement from different animals and human: a host-pathogen relationship. FEMS Immunol Med Microbiol (2005) 43(2):165–72. doi:10.1016/j.femsim.2004.07.012
30. Dieterich R, Hammerschmidt C, Richter D, Skerka C, Wallich R, Matuschka FR, et al. Inadequate binding of immune regulator factor H is associated with sensitivity of Borrelia lusitaniae to human complement. Infect Immun (2010) 78(11):4467–76. doi:10.1128/IAI.00138-10
31. Herzberger P, Siegel C, Skerka C, Fingerle V, Schulte-Spechtel U, van Dam A, et al. Human pathogenic Borrelia spielmanii sp. nov. resists complement-mediated killing by direct binding of immune regulators factor H and factor H-like protein 1. Infect Immun (2007) 75(10):4817–25. doi:10.1128/iai.00532-07
32. Kraiczy P, Hunfeld KP, Breitner-Ruddock S, Wurzner R, Acker G, Brade V. Comparison of two laboratory methods for the determination of serum resistance in Borrelia burgdorferi isolates. Immunobiology (2000) 201(3–4):406–19. doi:10.1016/S0171-2985(00)80094-7
33. Kurtenbach K, Sewell H-S, Ogden NH, Randolph SE, Nuttall PA. Serum complement sensitivity as a key factor in Lyme disease ecology. Infect Immun (1998) 66(3):1248–51.
34. Patarakul K, Cole MF, Hughes CAN. Complement resistance in Borrelia burgdorferi strain 297: outer membrane proteins prevent MAC formation at lysis susceptible sites. Microb Pathog (1999) 27(1):25–41. doi:10.1006/mpat.1999.0280
35. Ticha L, Golovchenko M, Oliver JH Jr, Grubhoffer L, Rudenko N. Sensitivity of Lyme borreliosis spirochetes to serum complement of regular zoo animals: potential reservoir competence of some exotic vertebrates. Vector Borne Zoonotic Dis (2016) 16(1):13–9. doi:10.1089/vbz.2015.1847
36. Schwab J, Hammerschmidt C, Richter D, Skerka C, Matuschka FR, Wallich R, et al. Borrelia valaisiana resist complement-mediated killing independently of the recruitment of immune regulators and inactivation of complement components. PLoS One (2013) 8(1):e53659. doi:10.1371/journal.pone.0053659
37. Klempner MS, Noring R, Epstein MP, McCloud B, Rogers RA. Binding of human urokinase type plasminogen activator and plasminogen to Borrelia species. J Infect Dis (1996) 174(1):97–104. doi:10.1093/infdis/174.1.97
38. Schuijt TJ, Coumou J, Narasimhan S, Dai J, Deponte K, Wouters D, et al. A tick mannose-binding lectin inhibitor interferes with the vertebrate complement cascade to enhance transmission of the Lyme disease agent. Cell Host Microbe (2011) 10(2):136–46. doi:10.1016/j.chom.2011.06.010
39. Tyson K, Elkins C, Patterson H, Fikrig E, de Silva A. Biochemical and functional characterization of Salp20, an Ixodes scapularis tick salivary protein that inhibits the complement pathway. Insect Mol Biol (2007) 16(4):469–79. doi:10.1111/j.1365-2583.2007.00742.x
40. Hellwage J, Meri T, Heikkila T, Alitalo A, Panelius J, Lahdenne P, et al. The complement regulator factor H binds to the surface protein OspE of Borrelia burgdorferi. J Biol Chem (2001) 276(11):8427–35. doi:10.1074/jbc.M007994200
41. Kraiczy P, Skerka C, Brade V, Zipfel PF. Further characterization of complement regulator-acquiring surface proteins of Borrelia burgdorferi. Infect Immun (2001) 69(12):7800–9. doi:10.1128/iai.69.12.7800-7809.2001
42. Kraiczy P, Skerka C, Kirschfink M, Brade V, Peter F. Zipfel: immune evasion of Borrelia burgdorferi by acquisition of human complement regulators FHL-1/reconectin and Factor H. Eur J Immunol (2001) 31(6):1674–84. doi:10.1002/1521-4141(200106)31:6<1674::AID-IMMU1674>3.0.CO;2-2
43. Herzberger P, Siegel C, Skerka C, Fingerle V, Schulte-Spechtel U, Wilske B, et al. Identification and characterization of the factor H and FHL-1 binding complement regulator-acquiring surface protein 1 of the Lyme disease spirochete Borrelia spielmanii sp. nov. Int J Med Microbiol (2009) 299(2):141–54. doi:10.1016/j.ijmm.2008.06.005
44. Bhide MR, Escudero R, Camafeita E, Gil H, Jado I, Anda P. Complement factor H binding by different Lyme disease and relapsing fever Borrelia in animals and human. BMC Res Notes (2009) 2:134. doi:10.1186/1756-0500-2-134
45. Hammerschmidt C, Klevenhaus Y, Koenigs A, Hallstrom T, Fingerle V, Skerka C, et al. BGA66 and BGA71 facilitate complement resistance of Borrelia bavariensis by inhibiting assembly of the membrane attack complex. Mol Microbiol (2016) 99(2):407–24. doi:10.1111/mmi.13239
46. Stevenson B, Tilly K, Rosa PA. A family of genes located on four separate 32-kilobase circular plasmids in Borrelia burgdorferi B31. J Bacteriol (1996) 178(12):3508–16.
47. Kraiczy P, Skerka C, Zipfel PF, Brade V. Complement regulator-acquiring surface proteins of Borrelia burgdorferi: a new protein family involved in complement resistance. Wien Klin Wochenschr (2002) 114(13–14):568–73.
48. McDowell JV, Hovis KM, Zhang H, Tran E, Lankford J, Marconi RT. Evidence that the BBA68 protein (BbCRASP-1) of the Lyme disease spirochetes does not contribute to factor H-mediated immune evasion in humans and other animals. Infect Immun (2006) 74(5):3030–4. doi:10.1128/iai.74.5.3030-3034.2006
49. Cordes FS, Roversi P, Kraiczy P, Simon MM, Brade V, Jahraus O, et al. A novel fold for the factor H-binding protein BbCRASP-1 of Borrelia burgdorferi. Nat Struct Mol Biol (2005) 12(3):276–7. doi:10.1038/nsmb902
50. Haupt K, Kraiczy P, Wallich R, Brade V, Skerka C, Zipfel P. Binding of human factor H-related protein 1 to serum-resistant Borrelia burgdorferi is mediated by borrelial complement regulator-acquiring surface proteins. J Infect Dis (2007) 196(1):124–33. doi:10.1086/518509
51. Kraiczy P, Hellwage J, Skerka C, Becker H, Kirschfink M, Simon MM, et al. Complement resistance of Borrelia burgdorferi correlates with the expression of BbCRASP-1, a novel linear plasmid-encoded surface protein that interacts with human factor H and FHL-1 and is unrelated to Erp proteins. J Biol Chem (2004) 279(4):2421–9. doi:10.1074/jbc.M308343200
52. Brooks CS, Vuppala SR, Jett AM, Alitalo A, Meri S, Akins DR. Complement regulator-acquiring surface protein 1 imparts resistance to human serum in Borrelia burgdorferi. J Immunol (2005) 175(5):3299–308. doi:10.4049/jimmunol.175.5.3299
53. Kenedy MR, Vuppala SR, Siegel C, Kraiczy P, Akins DR. CspA-mediated binding of human factor H inhibits complement deposition and confers serum resistance in Borrelia burgdorferi. Infect Immun (2009) 77(7):2773–82. doi:10.1128/IAI.00318-09
54. Hallström T, Siegel C, Morgelin M, Kraiczy P, Skerka C, Zipfel PF. CspA from Borrelia burgdorferi inhibits the terminal complement pathway. MBio (2013) 4(4):e481–413. doi:10.1128/mBio.00481-13
55. Hammerschmidt C, Koenigs A, Siegel C, Hallstrom T, Skerka C, Wallich R, et al. Versatile roles of CspA orthologs in complement inactivation of serum-resistant Lyme disease spirochetes. Infect Immun (2014) 82(1):380–92. doi:10.1128/IAI.01094-13
56. Wallich R, Pattathu J, Kitiratschky V, Brenner C, Zipfel PF, Brade V, et al. Identification and functional characterization of complement regulator-acquiring surface protein 1 of the Lyme disease spirochetes Borrelia afzelii and Borrelia garinii. Infect Immun (2005) 73(4):2351–9. doi:10.1128/iai.73.4.2351-2359.2005
57. Hallström T, Haupt K, Kraiczy P, Hortschansky P, Wallich R, Skerka C, et al. Complement regulator-acquiring surface protein 1 of Borrelia burgdorferi binds to human bone morphogenic protein 2, several extracellular matrix proteins, and plasminogen. J Infect Dis (2010) 202(3):490–8. doi:10.1086/653825
58. Bykowski T, Woodman ME, Cooley AE, Brissette CA, Brade V, Wallich R, et al. Coordinated expression of Borrelia burgdorferi complement regulator-acquiring surface proteins during the Lyme disease spirochete’s mammal-tick infection cycle. Infect Immun (2007) 75(9):4227–36. doi:10.1128/iai.00604-07
59. von Lackum K, Miller JC, Bykowski T, Riley SP, Woodman ME, Brade V, et al. Borrelia burgdorferi regulates expression of complement regulator-acquiring surface protein 1 during the mammal-tick infection cycle. Infect Immun (2005) 73(11):7398–405. doi:10.1128/iai.73.11.7398-7405.2005
60. Hartmann K, Corvey C, Skerka C, Kirschfink M, Karas M, Brade V, et al. Functional characterization of BbCRASP-2, a distinct outer membrane protein of Borrelia burgdorferi that binds host complement regulators factor H and FHL-1. Mol Microbiol (2006) 61(5):1220–36. doi:10.1111/j.1365-2958.2006.05318.x
61. Siegel C, Schreiber J, Haupt K, Skerka C, Brade V, Simon MM, et al. Deciphering the ligand-binding sites in the Borrelia burgdorferi complement regulator-acquiring surface protein 2 required for interactions with the human immune regulators factor H and factor H-like protein 1. J Biol Chem (2008) 283(50):34855–63. doi:10.1074/jbc.M805844200
62. Kraiczy P, Schreiber J, Skerka C, Haupt K, Brade V, Wallich R, et al. Assessment of the regions within complement regulator-acquiring surface protein (CRASP)-2 of Borrelia burgdorferi required for interaction with host immune regulators FHL-1 and factor H Intern. J Med Microbiol (2008) 298(1):268–71.
63. Rogers EA, Abdunnur SV, McDowell JV, Marconi RT. Comparative analysis of the properties and ligand binding characteristics of CspZ, a factor H binding protein, derived from Borrelia burgdorferi isolates of human origin. Infect Immun (2009) 77(10):4396–405. doi:10.1128/IAI.00393-09
64. Rogers EA, Marconi RT. Delineation of species-specific binding properties of the CspZ protein (BBH06) of Lyme disease spirochetes: evidence for new contributions to the pathogenesis of Borrelia spp. Infect Immun (2007) 75(11):5272–81. doi:10.1128/iai.00850-07
65. Schutzer SE, Fraser-Liggett CM, Qiu WG, Kraiczy P, Mongodin EF, Dunn JJ, et al. Whole-genome sequences of Borrelia bissettii, Borrelia valaisiana, and Borrelia spielmanii. J Bacteriol (2012) 194(2):545–6. doi:10.1128/JB.06263-11
66. Kraiczy P, Seling A, Brissette CA, Rossmann E, Hunfeld KP, Bykowski T, et al. Borrelia burgdorferi complement regulator-acquiring surface protein 2 (CspZ) as a serological marker of human Lyme disease. Clin Vaccine Immunol (2008) 15(3):484–91. doi:10.1128/CVI.00415-07
67. Coleman AS, Yang X, Kumar M, Zhang X, Promnares K, Shroder D, et al. Borrelia burgdorferi complement regulator-acquiring surface protein 2 does not contribute to complement resistance or host infectivity. PLoS One (2008) 3(8):3010e. doi:10.1371/journal.pone.0003010
68. Lin T, Gao L, Zhang C, Odeh E, Jacobs MB, Coutte L, et al. Analysis of an ordered, comprehensive STM mutant library in infectious Borrelia burgdorferi: insights into the genes required for mouse infectivity. PLoS One (2012) 7(10):e47532. doi:10.1371/journal.pone.0047532
69. Alitalo A, Meri T, Lankinen H, Seppala I, Lahdenne P, Hefty PS, et al. Complement inhibitor factor H binding to Lyme disease spirochetes is mediated by inducible expression of multiple plasmid-encoded outer surface protein E paralogs. J Immunol (2002) 169(7):3847–53. doi:10.4049/jimmunol.169.7.3847
70. Hammerschmidt C, Hallstrom T, Skerka C, Wallich R, Stevenson B, Zipfel PF, et al. Contribution of the infection-associated complement regulator-acquiring surface protein 4 (ErpC) to complement resistance of Borrelia burgdorferi. Clin Dev Immunol (2012) 2012:349657. doi:10.1155/2012/349657
71. Hovis KM, Tran E, Sundy CM, Buckles E, McDowell JV, Marconi RT. Selective binding of Borrelia burgdorferi OspE paralogs to factor H and serum proteins from diverse animals: possible expansion of the role of OspE in Lyme disease pathogenesis. Infect Immun (2006) 74(3):1967–72. doi:10.1128/iai.74.3.1967-1972.2006
72. McDowell JV, Wolfgang J, Tran E, Metts MS, Hamilton D, Marconi RT. Comprehensive analysis of the factor H binding capabilities of Borrelia species associated with Lyme disease: delineation of two distinct classes of factor H binding proteins. Infect Immun (2003) 71(6):3597–602. doi:10.1128/iai.71.6.3597-3602.2003
73. Metts MS, McDowell JV, Theisen M, Hansen PR, Marconi RT. Analysis of the OspE determinants involved in binding of factor H and OspE-targeting antibodies elicited during Borrelia burgdorferi infection in mice. Infect Immun (2003) 71(6):3587–96. doi:10.1128/iai.71.6.3587-3596.2003
74. Siegel C, Hallström T, Skerka C, Eberhardt H, Uzonyi B, Beckhaus T, et al. Complement factor H-related proteins CFHR2 and CFHR5 represent novel ligands for the infection-associated CRASP proteins of Borrelia burgdorferi. PLoS One (2010) 5(10):e13519. doi:10.1371/journal.pone.0013519
75. Brissette CA, Haupt K, Barthel D, Cooley AE, Bowman A, Skerka C, et al. Borrelia burgdorferi infection-associated surface proteins ErpP, ErpA, and ErpC bind human plasminogen. Infect Immun (2009) 77(1):300–6. doi:10.1128/IAI.01133-08
76. Seling A, Siegel C, Fingerle V, Jutras BL, Brissette CA, Skerka C, et al. Functional characterization of Borrelia spielmanii outer surface proteins that interact with distinct members of the human factor H protein family and with plasminogen. Infect Immun (2010) 78(1):39–48. doi:10.1128/IAI.00691-09
77. Alitalo A, Meri T, Comstedt P, Jeffery L, Tornberg J, Strandin T, et al. Expression of complement factor H binding immunoevasion proteins in Borrelia garinii isolated from patients with neuroborreliosis. Eur J Immunol (2005) 35(10):3043–53. doi:10.1002/eji.200526354
78. Pietikainen J, Meri T, Blom AM, Meri S. Binding of the complement inhibitor C4b-binding protein to Lyme disease Borreliae. Mol Immunol (2010) 47(6):1299–305. doi:10.1016/j.molimm.2009.11.028
79. Garcia BL, Zhi H, Wager B, Hook M, Skare JT. Borrelia burgdorferi BBK32 inhibits the classical pathway by blocking activation of the C1 complement complex. PLoS Pathog (2016) 12(1):e1005404. doi:10.1371/journal.ppat.1005404
80. Pausa M, Pellis V, Cinco M, Giulianini PG, Presani G, Perticarari S, et al. Serum-resistant strains of Borrelia burgdorferi evade complement-mediated killing by expressing a CD59-like complement inhibitory molecule. J Immunol (2003) 170(6):3214–22. doi:10.4049/jimmunol.170.6.3214
81. Fuchs H, Wallich R, Simon MM, Kramer MD. The outer surface protein A of the spirochete Borrelia burgdorferi is a plasmin(ogen) receptor. Proc Natl Acad Sci U S A (1994) 91(26):12594–8. doi:10.1073/pnas.91.26.12594
82. Koenigs A, Hammerschmidt C, Jutras BL, Pogoryelov D, Barthel D, Skerka C, et al. BBA70 of Borrelia burgdorferi is a novel plasminogen-binding protein. J Biol Chem (2013) 288(35):25229–43. doi:10.1074/jbc.M112.413872
Keywords: spirochetes, Borrelia, Lyme disease, complement, immune evasion, complement regulator, innate immunity
Citation: Kraiczy P (2016) Hide and Seek: How Lyme Disease Spirochetes Overcome Complement Attack. Front. Immunol. 7:385. doi: 10.3389/fimmu.2016.00385
Received: 08 August 2016; Accepted: 13 September 2016;
Published: 26 September 2016
Edited by:
Monica E. Embers, Tulane University, USAReviewed by:
Jon Skare, Texas A&M Health Science Center, USACatherine Ayn Brissette, University of North Dakota, USA
Copyright: © 2016 Kraiczy. This is an open-access article distributed under the terms of the Creative Commons Attribution License (CC BY). The use, distribution or reproduction in other forums is permitted, provided the original author(s) or licensor are credited and that the original publication in this journal is cited, in accordance with accepted academic practice. No use, distribution or reproduction is permitted which does not comply with these terms.
*Correspondence: Peter Kraiczy, a3JhaWN6eSYjeDAwMDQwO2VtLnVuaS1mcmFua2Z1cnQuZGU=