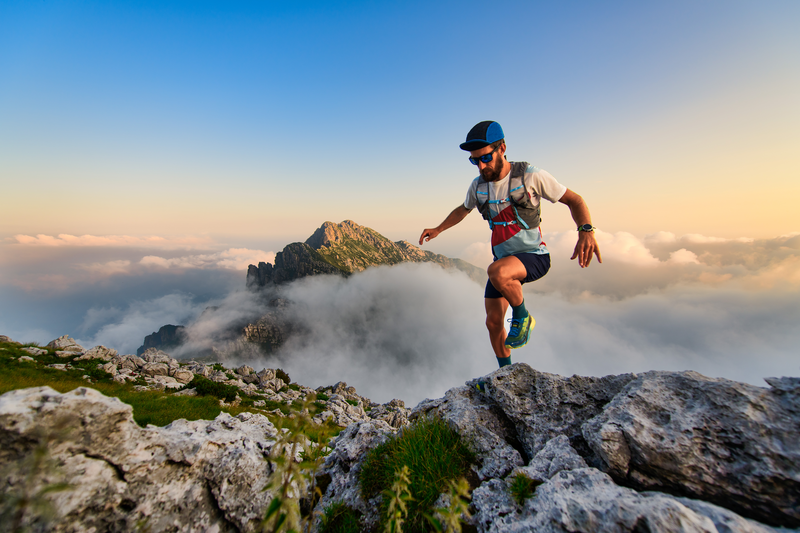
95% of researchers rate our articles as excellent or good
Learn more about the work of our research integrity team to safeguard the quality of each article we publish.
Find out more
REVIEW article
Front. Immunol. , 28 September 2016
Sec. HIV and AIDS
Volume 7 - 2016 | https://doi.org/10.3389/fimmu.2016.00383
This article is part of the Research Topic Immune mechanisms underlying the increased morbidity and mortality of HIV-exposed uninfected (HEU) children View all 12 articles
Infants born to human immunodeficiency virus (HIV) infected women are HIV-exposed but the majority remains uninfected [i.e., HIV-exposed uninfected (HEU)]. HEU infants suffer greater morbidity and mortality from infections compared to HIV-unexposed (HU) peers. The reason(s) for these worse outcomes are uncertain, but could be related to an altered immune system state. This review comprehensively summarizes the current literature investigating the adaptive and innate immune system of HEU infants. HEU infants have altered cell-mediated immunity, including impaired T-cell maturation with documented hypo- as well as hyper-responsiveness to T-cell activation. And although prevaccination vaccine-specific antibody levels are often lower in HEU than HU, most HEU infants mount adequate humoral immune response following primary vaccination with diphtheria toxoid, haemophilus influenzae type b, whole cell pertussis, measles, hepatitis B, tetanus toxoid, and pneumococcal conjugate vaccines. However, HEU infants are often found to have lower absolute neutrophil counts as compared to HU infants. On the other hand, an increase of innate immune cytokine production and expression of co-stimulatory markers has been noted in HEU infants, but this increase appears to be restricted to the first few weeks of life. The immune system of HEU children beyond infancy remains largely unexplored.
Mother-to-child transmission (MTCT) of human immunodeficiency virus (HIV) has been reduced from 14–48% (1) to less than 3% in both high-income countries (2–4) and resource-limited settings (5, 6). The vast majority of infants born to HIV-infected women, thus, are HIV-exposed uninfected (HEU). In countries with high prevalence of HIV infection, such as some parts of Sub-Saharan Africa, HEU infants can comprise a significant proportion reaching 30% of all infants (6).
The mortality rates of HEU infants are twice higher than HIV-unexposed (HU) peers in the second year of life in Zimbabwe (7), 4.6% through 4 months in Zambia (8), and 4.9% by 1 year in Africa (9). This relates in part to the elevated risk of HEU infants suffer from infectious disease; this is the case in both low- (10, 11) and high-income countries (12). Specifically, increased risk for contracting infections (13), higher risk for severity of infection (14–18), greater rates of hospitalization (15, 18), and mortality (7) have been documented in HEU as compared to HU infants. HEU infants appear particularly susceptible to invasive infection with Group B Streptococcus and Streptococcus pneumoniae invasive disease as well respiratory tract infections (14, 19, 20).
The underlying cause of the increased risk for infectious morbidity and mortality in HEU remains unknown, thus, can currently not be rectified. HEU infants have two unique exposures compared to their HU peers that have the potential to alter their developing immune system and with that potentially worsen their infectious disease outcomes: antiretroviral (ARV) drugs and maternal HIV infection (21). Some of the ARV drugs, such as zidovudine (ZDV), have mitochondrial toxicity likely due to inhibition of host cell gamma-polymerase and accumulation of somatic mitochondrial DNA mutations (22, 23), or due to direct interference with mitochondrial bioenergetics cascades (24, 25), and induction of reactive oxygen species formation leading to cell damage (26). In vitro studies have revealed that ZDV exposure inhibits hematopoietic progenitor cells, which may explain ARV’s associated decreased red blood cell, neutrophil, and lymphocyte counts (27, 28). ZDV also has the potential to impair the HEU infant’s innate immune system development (specifically granulocytes/macrophages) (27). Combination ARV therapy has been associated with larger and longer lasting suppressive effect on neonatal neutrophil and lymphocyte counts at age of 0–2 months as compared to ARV mono-therapy (28).
Even when the neonate escapes HIV infection, the HIV-infected maternal–fetus interface may present an altered environment for fetal growth and development. HIV-infected women are at increased risk for chorioamnionitis and deciduitis (29). Increased infection or inflammation of the uterine environment exposes the developing immune system of the neonate to antigens and a potentially pro-inflammatory milieu of cytokines and chemokines. It is also noteworthy that the vaginal microbiota appears to be altered in HIV-infected women (30), which may be of importance for early infancy colonization with microbes. The sum of these effects is conceptualized as an “active womb” of HIV-infected women that has the potential to prime and alter the development of the neonatal immune system.
We here review what is known about altered function (both adaptive and innate) during early life immune ontogeny of HEU infants.
Previous studies described both the quantitative and functional measures of the cell-mediated immunity (CMI) of HEU infants. Data on the quantity and quality (function) of CMI among HEU infants are derived mainly from observational studies. Moreover, these studies are difficult to interpret and their results are inconsistent, challenging the ability to draw a definite conclusion. This is further complicated by variability of the cohort characteristics reported (age at enrollment, settings, ethnicity, time span of follow-up) and laboratory methodology (antigenic stimulus, functional test) utilized.
The most reported immunological abnormality of HEU infants pertains to the frequency of immune cell subsets. Cluster of differentiation (CD) 4 T-cells have been relatively well studied in HEU infants, owing to both the vulnerability of CD4 T-cells to HIV infection and their important role as regulators of the immune system and acquired immunity. Lower CD4 T-cell counts (28, 31–37) and to a lesser extent lower CD8 T-cell counts (32, 33) have been reported in multiple studies contrasting HEU infants to HU peers. Maternal HIV viral load has been proposed as a correlate for subsequent HEU T-cells counts. At 2 and 6 months of age, HEU infants born to mothers with viral load >1000 copies/ml had lower CD4 T-cell counts compared to HEU infants born to mothers with viral load <50 copies/ml at the time of delivery (35). Decreased counts of circulating CD4 T-cells may limit antigenic coverage and subsequent response, eventually cumulating in increased severity of infections.
However, differences between HEU vs. HU T-cell counts may be more nuanced. It has been proposed that the difference in the quantity of circulating T-cells detected in HEU infants is in part due to difference in frequencies of subsets of CD4 T-cells. HEU newborns had lower CD4 to CD8 T-cell ratio, lower CD4 naïve, and CD8 naïve T-cell percentages, increased percentage of activated (CD8+ CD38bright) CD8 T-cells and memory (CD4+ CD45RO+) T-cells, augmented double-negative (CD3+ CD4− CD8−) and immature (CD4− CD8− CD5− CD44+) T-cells when compared to HU newborns (34). The elevated number of immature (CD4− CD8− CD5− CD44+) T-cells suggests that the physiologic thymic maturation pathway may be impaired in HEU children (34). In another study, activated (CD4+ HLA− DR+ CD38+) and memory (CD4+ CD45RA− RO+) T-cells were higher among HEU infants compared to infants born to HIV-uninfected women (38). The increase in the percentage of activated and memory T-cells and the diminished numbers of naïve T-cells suggests that this subset of lymphocytes had experienced antigenic exposure that could have resulted from either in utero exposure of the fetus to antigens or to non-antigen specific cell differentiation.
The altered numbers and subtypes of T-cells in HEU infants may or may not result in impaired T-cell immune function (21).
Several studies have shown that HEU infants express altered CMI function and display impaired T-cell maturation. The CMI response of HEU infants has been studied primarily by measuring cytokine production and cell proliferation to both HIV-specific and non-HIV-specific antigens with reports of hyper- or hypo-activity depending on the stimulant (Table 1). It is proposed that increased CMI response in HEU infants may result from in utero exposure to HIV antigens and/or other antigens related to other infections in the HIV-infected mothers. By contrast, attenuation of the CMI response might be related to limited CD4 T-cells repertoire and progenitor cell dysfunction that might lead to T-cells with compromised function.
HIV-Specific T-Cell Response
It has been fairly well established that following exposure to HIV antigens, cytotoxic T lymphocyte responses can be elicited among HEU children aged 2–35 months (45–48). Following stimulation with HIV peptides, the production of interleukin (IL)-2 in mononuclear cells was also observed. Specifically, HEU cord blood leukocytes produced IL-2 following stimulation with HIV antigen in 40–42% of HEU infants (39, 40). However, limited data suggest that cord blood mononuclear cells of HEU newborns fail to proliferate in response to synthetic HIV envelope peptides (41). The clinical significance of these findings thus is currently unclear as IL-2 has the potential to promote the expansion and influence the differentiation of effector CD4 T-cells into different subtypes: Th1, Th2, Th17, and T regulatory cells (49). While the aforementioned studies (45–48) may suggest that HEU infants have protective CMI responses to HIV, functionally this may not translate into effective protection. CD4 T-cells from HEU infants that were infected in vitro with HIV had higher levels of p24 protein as compared to healthy controls (50). This suggests that CD4 T-cells of HEU infants could in fact be more susceptible to in vitro HIV infection as compared to healthy controls (50).
Non-HIV-Specific T-Cell Response
While HIV-specific responses are of interest when considering MTCT and immunity to HIV, more traditional investigation of immune function has relied on generic stimuli to elicit CMI responses. In response to Staphylococcus aureus Cowan stimulation, infants of HIV-infected mothers had reduced cord blood mononuclear cell production of IL-12, a key Th1-response supporting cytokine, when compared to infants born to HIV-negative mothers (42). This suggests a possible suppressive effect of HIV exposure on Th1 immunity. However, in response to another polyclonal stimulus [phytohemagglutinin (PHA)], reduced IL-2 has been observed among HEU infants (38) as compared to HU infants. In the latter study, a stronger production of IFN-γ to IL-10 (the ratio of PHA-stimulated IFN-γ to IL-10 production) of cord blood cells was observed among HEU than HIV-infected infants (51). No HIV-infected infant produced more IFN-γ than IL-10, while 43% of HIV-uninfected infants produced higher IFN-γ than IL-10 (51). It is important to note that these stimuli activated cells via different, and often poorly understood mechanisms (52–59).
Studies have used different methods, culture techniques, timing, and stimuli for assessing HEU T-cell proliferation with evidence of both hypo and hyper proliferation. Higher lymphoproliferative responses to polyclonal T-cell activators (anti-CD3 and anti-CD28) were observed in cell cultures from HEU neonates born to HIV-infected mothers with uncontrolled HIV replication compared to HEU neonates born to HIV-infected mothers with undetectable viral load and HU (41).
Other groups used different mitogenic stimuli and reported increased proliferative responses among HEU infants to staphylococcal enterotoxin B at 6 and 14 weeks of life (44), and to PHA at 6–8 months of life (43) as compared to HU infants. Assessment of HEU infant T-cell proliferative responses to BCG vaccination have provided discordant results, with no difference at 2 weeks (36), weaker response at 6–8 months (43) and at 10 weeks of life (36), and stronger response at both 6 and 14 weeks of life (44) when comparing HEU infants to HU controls.
In summary, the most consistent findings are that HEU infants have lower CD4 T-cell, lower CD8 T-cell counts, increased activated and memory T-cells as compared to infants born to HIV-uninfected women and there is compelling evidence that HEU infants have HIV-specific cellular memory response. HIV and associated antigenic exposure can take place during gestation. Data support that HIV can cross the placenta during gestation as HIV has been detected in fetal tissues (60). HIV exposure appears to be able to activate HIV-specific memory responses (45–48, 61). The HIV-specific T-cell immune response, at least in part, is suppressed by Treg cells (61). Depletion of Treg cells increased HIV-specific T-cell immune responses among HEU infants (61).
It should be noted that there is controversy regarding the T-cell response to non-HIV-specific stimulation. Mixed hyper- and/or hypo-responsiveness to T-cell activation and the different pro-inflammatory cytokines profile may stem from different experimental conditions, variable population characteristics, maternal HIV viral load, and ARV exposure.
If the HIV-infected in utero milieu leads to priming of the HEU immune system, it follows that this takes place in a complex manner. This may be a simplified explanation for the complex reality that leads to diversity in HEU hyper- vs. hypo-responsiveness that may be deemed immune dysregulation. It further stands to reason that there will be population variation in such immune dysregulation, which may explain both discordant results and how many HEU infants go on to lead relatively healthy lives compared to some of their peers. Ultimately, it is suspected that for some stimuli the afflicted HEU infant will be hyper-responsive, while being normal or hypo-responsive to other stimuli.
The quantity and function of passively acquired maternal antibodies conveyed to HEU infants is reviewed in a companion article of this Research Topic by Abu Raya et al. (62). Primary vaccination during infancy is of critical importance for prevention of infectious morbidity and mortality. It should be noted that the immunogenicity of primary vaccination according to the Expanded Program on Immunization (EPI), a World Health Organization initiative to expand vaccination programs throughout the world, was also evaluated among HEU infants. The summarized literature on HEU response to vaccination can be found in the following section (Table 2).
Data on the immunogenicity and seroprotection provided to HEU infants following primary immunization with hepatitis B vaccine (HBV) suggest that the majority of HEU infants produce adequate humoral immune response.
In South Africa, prevaccination (at 2 and 6 weeks) hepatitis B surface antigen (HBsAg) IgG levels, presumed maternally derived, were significantly higher among HEU than HU infants, with one-quarter of HEU infants having sero-protective levels (HBsAg IgG > 10 mIU/ml) (63). Following primary vaccination according to the EPI, HBsAg IgG levels at 3 months (after two HBV doses given at 6 and 10 weeks of age) were similar between HEU and HU infants and continued to be equivalent through the first 2 years of life (63). A subsequent study of South African HEU infants reported that >99% of HEU infants developed sero-protective antibody levels (HBsAg IgG >10 mIU/ml) 1 month after completing the primary HBV series (64). This small South African series would suggest that HEU infants do indeed produce adequate acquired immunity in response to HBV vaccination.
The prevalence of non-responders to primary series of HBV was also described. Among a cohort of Brazilian HEU infants vaccinated with HBV (at birth, 1 and 6 months), 6.7% of HEU infants were non-responders to HBV (HBsAg IgG <10 mIU/ml) 1 month after the third dose of HBV (65). This study supports earlier findings which reported that about 8% of HEU infants did not respond to HBV (66) and more recent data from India which found that of HEU infants vaccinated with three doses of recombinant HBV (at 6, 10, and 14 weeks), 11.5% were non-responders 3 months after the third dose of the vaccine (67). The reported prevalence of non-responders to HBV among HEU infants, 6.7–11.5% (65–67) is comparable to the 5–10% of non-responders reported among healthy population (73).
In sum, though timing of vaccination and population characteristics are variable, it does appear that the majority of HEU infants are capable of mounting a sufficient immune response for HBV vaccination to confer adequate seroprotection. What remains to be determined is how resilient these responses are and whether they wane over time. There may also be a sub-population within HEU infants that are at higher risk and do not mount adequate immune responses to HBV vaccination.
Studies that evaluated the immunogenicity and seroprotection following tetanus toxoid (TT) vaccination reports that HEU infants develop robust immune response. One month following the completion of the vaccination series with diphtheria-tetanus toxoid-cellular pertussis-Haemophilus influenzae type b (DTP-Hib) (given at 2, 4, and 6 months) in Brazil, all HEU infants showed sero-protective immune response to TT (anti-TT IgG >0.1 IU/ml) (65). And following the completion of 3 DTP vaccines doses (at 6, 10, and 14 weeks) in South Africa, anti-TT IgG levels were similar between HU and HEU infants, and the majority of HEU developed sero-protective levels to TT (63, 64, 68). Lowest prevaccination anti-TT IgG levels were associated with greatest vaccine-induced immune responses at 16 weeks (68).
Data also support that HEU infants mount a quantitative immune response to diphtheria toxoid (DT) vaccine that is comparable to HU infants. Specifically, Brazilian HEU infants vaccinated with DTP-Hib (at 2, 4, and 6 months) has been shown to have similar anti-DT IgG levels compared to infants not exposed to HIV, 1 month after the 3rd dose of the vaccine (65). Moreover, the percentage of non-responders to DT vaccine (anti-DT IgG <0.1 IU/ml) was not significantly different between the groups, with 94.7% of HEU subjects and 98.2% of HU infants, having protective levels against DT after completion of primary series of three DTP doses (65). These findings were supported by a study in South Africa that found antibody levels against DT to be similar between HEU and HU, 1 month following the completion of primary vaccination series with diphtheria-toxoid, tetanus-toxoid, whole cell pertussis-HibCV (DTwP-HibC) according to the EPI (at 6, 10, and 14 weeks) (64). In addition, a high proportion (>99%) of HEU children developed sero-protective levels to DT vaccine (anti-DT IgG ≥ to 0.01 and 0.1 IU/ml) (64).
Though limited to only two studies on the subject, it appears as though HEU infants are not significantly different from peers with respect to response to DT vaccine.
Evaluation of the immune response to 7-valent pneumococcal conjugate vaccine (PCV) showed higher pneumococcal-specific antibody levels following the second (68) and third 7-valent PCV-doses (69) in HEU infants as compared to HU controls. Specifically, Jones et al. found that among a cohort of South African HEU infants vaccinated with two doses of 7-valent PCV (at 6 and 14 weeks), HEU infants had higher levels of pneumococcal-specific antibody levels than HU at 16 weeks of age (68). Moreover, infants with the lowest prevaccination pneumococcal-specific antibody levels had the most robust immune responses to the pneumococcal vaccine at 16 weeks (68). However, the clinical significance of these robust results is complicated by lack of well-established correlate of protection for collective antibody response to pneumococcal vaccine (68).
The largest study to date to report the immune response following primary PCV immunization in HEU infants described a cohort of HEU infants in South Africa (69). Prevaccination (age <6 weeks), HEU infants had lower titers and lower proportion of children with serotype-specific anti-capsular antibody ≥0.35 μg/ml to all serotypes (4, 6B, 9V, 14, 18c, 19F, and 23 F) compared to HU infants (69). At 10 weeks, antibody levels following the first 7-valent PCV-dose (given at age 6 weeks) were lower for four serotypes (6B, 14, 19F, and 23F) and the proportion of children who had serotype-specific anti-capsular antibody ≥0.35 μg/ml were lower for most serotypes (6B, 9V, 14, 19F, and 23F) in HEU as compared to HU infants (69). Following the second 7-valent PCV-dose (at 10 weeks), the antibody levels measured at 14 weeks of age were similar in HEU and HU infants for the majority of serotypes (6B, 9V, 14, 18c, 19F, and 23 F) (69). Three to six weeks following the third 7-valent PCV-dose (given at 14 weeks), the serotype-specific anti-capsular antibody levels were higher among HEU infants as compared to HU infants for all serotypes (69). When integrated, these data suggest that a two-dose primary series schedule in HEU children may afford similar protection against vaccine serotype-specific invasive pneumococcal disease as compared to HU children. However, it is noteworthy that the functionality of anti-pneumococcal antibodies as a correlate of protection is not well founded, and when assessed for opsonophagocytic activity there was an absence of consistency in the pattern between HEU and HU infants (69).
Current data on the immunogenicity of Haemophilus influenzae type b (Hib) vaccine administered to HEU infants is reassuring as HEU infants vaccinated according to the EPI mounted robust and immune responses that were similar to HU infants following vaccination with Hib vaccine.
Within 1 month following the completion of primary immunization (6, 10, and 14 weeks), South African HEU infants had similar anti-Hib antibody levels as compared with HU at the age of 16 weeks (64, 68). Gaensbauer et al. reported findings from a cohort of Ugandan HEU infants vaccinated with Hib polysaccharide conjugate vaccine at 6, 10, and 14 weeks of age. By 12 weeks of age (following two Hib vaccine doses), 91% of HEU had protective levels (anti-Hib IgG >1.0 μg/ml), as did all infants by 24 weeks after three doses. Interestingly, maternally derived anti-Hib IgG levels at birth did not correlate with anti-Hib IgG levels at 12 or 24 weeks. At 48 weeks, the vast majority (98.3%) of HEU still retained protective levels (70).
The duration of HEU infant immune response to primary Hib vaccination has been derived from longitudinal studies. Data from a South African cohort showed that although prevaccination anti-Hib IgG levels (at 2 and 6 weeks) were lower among HEU compared to HU infants, antibody levels were similar between both groups at 3 months (after two doses given at 6 and 10 weeks) and until 2 years of age (63).
Thus, current data suggest that HEU infants mount immune response to Hib vaccine similar to HU infants that lasted until 2 years of age.
Several studies have demonstrated that HEU infants mount higher immune response to primary vaccination with whole cell pertussis vaccine (given according to the EPI) compared to HU infants (63, 64, 68). Jones et al. reported data from a South African cohort and found that despite having lower anti-pertussis titers at birth, HEU infants had higher anti-pertussis antibody levels compared to HU 2 weeks following the completion of primary vaccination with thee doses of DTP-Hib vaccine (at 6, 10, and 14 weeks) (68). Infants with the lowest prevaccination anti-pertussis levels exhibited the greatest vaccine responses at 16 weeks (68). Reikie et al. has found that at 3 months, anti-pertussis IgG levels were similar between South African cohort of HEU and HU infants after two doses of DTP vaccine (at 6, 10 weeks), and higher for HEU as compared to HU at the age of 6 and 12 months (63). Moreover, Simani et al. reported that 1 month following the primary DTP-HibC vaccine series (at 6, 10, and 14 weeks) the anti-pertussis-toxoid titers were higher in HEU than in HU infants (64). It should be noted that the aforementioned studies used whole cell pertussis vaccine as compared to acellular pertussis vaccine that is currently employed by most developed countries. Moreover, the read out measure is critical as measuring collective antibody response to multiple antigens (63, 68) might be less accurate than antigen-specific responses. The lack of well-established sero-protective antibody levels is another challenge for extrapolating these finding to clinical settings. Overall, though more data are required on acellular pertussis vaccination among HEU infants, it does appear as though HEU infants mount satisfactory responses to whole cell pertussis vaccination.
Measles vaccine generated both comparable (63) and lower immune response (71) among HEU infants as compared to HU infants.
The proportion of South African HEU infants with sero-protective titers against measles was similar compared with HU infants, 28 weeks following the first measles vaccine dose (administered at nearly 40 weeks of age) and 2 weeks after the booster dose (administered at nearly 68 weeks of age) (71). However, waning immunity in HEU was noticed 9.5 months post booster, when HU had higher anti-measles titers and were more likely to have seroprotective titers (94.3%) compared with HEU (79.6%) infants, bringing the durability of HEU immune response into question (71). In contrast to the previous findings, the average levels of anti-measles virus antibodies were nearly indistinguishable between South African HEU and HU infants prevaccination (at 2 and 6 weeks), at 12 months (post 1st vaccine dose given at age of 9 months) and at 2 years (post 2nd vaccine dose given at 18 months) (63).
The long-term vaccine-induced immune memory against measles, rubella, and mumps was recently explored (72). A large US cohort of HEU children was evaluated at the age of 7–15 years, among which 87% of HEU infants had received two doses of measles, mumps, and rubella vaccine. The prevalence of measles seroprotection, rubella seroprotection, and mumps seropositivity were 99, 98, and 97%, respectively (72). Thus, although limited to one study, it appears that HEU infants retain long-term immunity to the measles, mumps, and rubella vaccine.
The innate immune system is the immediate, first line of defense against infectious agents that react rapidly upon encountering a pathogen. It is composed of different cells, including neutrophils, dendritic cells (DCs), monocytes, and natural killer (NK) cells.
In the 1980s, in vitro data showed that the development of the innate immune system can be impacted by ARVs as ZDV has been shown to suppress hematopoietic stem cells progenitors of granulocyte and macrophages (27). Several studies followed and evaluated the quantity and the function of innate immune system cells of HEU infants (Table 3).
HIV and ARVs exposed infants have lower neutrophil counts than HIV and ARVs unexposed infants (74). Data suggest that suppression of hematopoietic stem cell progenitors is the most plausible mechanism as reduced neutrophil levels have been shown to be non-immune mediated (74) and ARV exposure has been shown to affect two other lineages (platelets and lymphocytes) (79, 80).
The dynamics of neutrophil counts over time was described. In HIV and ARV-exposed infants (90/92 confirmed HEU), neutrophil levels were lower than in HIV and ARV unexposed infants from birth until 20 months of age, reaching statistical significance at 5 weeks and 8 months (74). In another study, neutrophil levels of HEU subjects, although not compared to HU controls, were high shortly after birth, declining to reach a nadir at 2 months, then increasing until approximately 7 months; after this, the increase was more gradual before stabilizing at 3.5 years (75).
Exposure to ARVs is associated with decrease in neutrophil levels lasting till 18 months of age (33) and up to 8 years of age in another study (75). Thus, it appears that ARV exposure have a long-term effect on neutrophil counts. However, whether this long-term effect is affected by the timing of ARV exposure (in utero, neonatal or both) has not been established yet.
Circulating DCs are human antigen-presenting cells (APC) and are the precursors of tissue and lymphoid organ DCs; conventional DCs [cDCs, also known as myeloid DCs (mDCs)] and plasmacytoid DC (pDC) are the two main subsets of human DCs. Both cell types mediate the downstream immune response based on the cytokines they secrete (81).
Few studies described the frequency and function of DCs among HEU infants. HEU newborns are reported as having a higher percentage of mDCs than HU newborns at birth in one study (77), but all other currently available data suggest that HEU and HU infants have similar proportion of cDCS and pDCs during the first 3–12 months (77) and during the first year of life (76). It must be noted that these reports were based on proportion rather than absolute count; thus, it is at this time inconclusive whether DCs counts are affected by perinatal ARV and HIV exposure.
Data on the function of DCs among HEU infants are also limited, and is based on reports of in vitro study of TLR agonists and cytokine responses.
At birth, mDCs of HEU infants exhibited higher responsiveness to stimulation with LPS (via TLR4) than HU (77). As compared to HU, a greater proportion of HEU mDCs responded to bacterial ligand stimulation (PAM via TLR1/2 and LPS via TLR4) by secretion of pro-inflammatory cytokines (TNF-alpha, IL-6, IL-12) up to 6 weeks of age (76). Beyond 6 weeks and until 1 year of age, the response of mDCs to bacterial ligands was comparable between HEU and HU infants (76).
At birth, pDCs of HEU and HU responded similarly to CpG ODN stimulation (via TLR9) (77) and secreted similar levels of IFN-α, indicating a normal stimulated pDC function (77). In another study, a comparable proportion of pDCs that responded to bacterial ligands by secretion of inflammatory cytokines were reported among HEU and HU infants until 1 year of age (76).
Taken together, existing data suggest that gestational HIV-1 exposure increases HEU infants’ mDCs responsiveness at birth and enhances a pro-inflammatory response to bacterial ligand stimulation up to 6 weeks of life that normalizes by 1 year of age. This is limited to only a pair of studies and the clinical significance of these findings is yet unknown.
Limited data exists on the quantity and function of monocytes among HEU infants. Monocyte counts were lower in the HIV- and ARV-exposed infants than in unexposed controls from birth until 8 months of age, reaching statistical significance at 5 weeks of age (74). However, in another study, the proportions of monocytes in HEU as compared to HU infants were not statistically different during the first year of life (76). The difference in absolute levels (74) of monocytes might have stronger clinical implications that the reported proportion (76).
Monocyte activity has been assayed though whole blood response to different TLR ligands, and has revealed differences between HEU and HU monocyte cytokine production during only the first 6 months of life (71). Specifically, a higher proportion monocytes from HEU infants mounted pro-inflammatory cytokine response (TNF-α, IL-6, IL-12) to bacterial ligands (PAM via TLR1/2 and LPS via TLR4) as compared to HU (76). Conversely, HU infants’ monocytes responded more strongly to viral ligand stimulation (pI:C and R848) at 6 months. By 1 year of age, the differences in the innate immune system activation between HEU and HU monocytes completely disappeared (76). This study suggest that HEU monocytes may be affected in function, leading to stronger immune response toward bacterial threats at the cost of weaker viral responses during early life, yet this stands to be repeated and tested further.
Natural killer cells are directly cytotoxic, often to virally infected cells, and do not require guidance by other cells. Currently, there is very limited data on the quantity and function of NK cells among HEU as compared to HU infants. At birth, the percentage of total NK cells and the percentage of different NK cells subsets (CD56 bright, CD56+ CD16+, CD56− CD16+) was not significantly different between HEU and HU infants. The total NK cell percentage did not change significantly in both HEU and HU over the first year of life (78).
Functional comparison demonstrated a significant decline in the percentage of activated NK cells (CD56dim subset) and perforin positive NK cells among HEU infants over the first year of life (78). By contrast, over time HU infants demonstrated an increase in perforin positive NK cells, a marker of cytolytic potential (78). The poor expression of perforin among HEU infants might have clinical implications as it might compromise the ability of HEU infants to control viral infection (78).
Current evidence suggests that during early life, the developing immune system of HEU infants differs from HU peers. The etiology of this altered immune ontogeny appears to be multifactorial, including in utero exposure to ARVs, HIV antigens and/or other antigens related to other infections in HIV-infected mothers. Few longitudinal studies describe their dynamics over time. Although HEU infants are at increased risk for infectious disease morbidity and mortality, their quantitative humoral immune response to primary vaccination is adequate. This suggests that other mechanisms, including impaired qualitative antibody response, altered CMI, impaired innate immunity or infections caused by pathogens other than those covered by existing vaccines may be at the heart of increased morbidity and mortality of HEU infants. Transient innate immune system activation leading to enhanced pro-inflammatory response to bacterial ligands stimulation or immune tolerance to viral ligands has been reported among HEU infants. The origins of this innate immune system activation might stem from increased infection or inflammation of the uterine environment that exposes the developing immune system of the neonate with antigen and a potentially pro-inflammatory milieu. This has the potential to prime the neonatal immune system in utero. The idea of priming of the innate immune system is supported by recent evidence on memory of the innate immune system (82). Whether the postnatal differential pathogen-specific innate immune cells activation affects the susceptibility of HEU infants to severe infections should be further investigated.
Synthesizing a clear model for the changes of the developing immune system is challenging as studies have enrolled different HEU population at different ages; HEU subjects were exposed to different maternal viral loads and ARV regimens; the laboratory methods employed, the experimental conditions and the read out measurements were also different among studies. These studies still do have merit, as they do suggest that the perinatal environment may lead to an altered immune system.
BA, TK, DM, and AM searched the scientific literature; BA and DM wrote the first draft; BA, TK, DM, and AM reviewed and edited the manuscript; BA, TK, DM, and AM approved the final version.
The authors declare that the research was conducted in the absence of any commercial or financial relationships that could be construed as a potential conflict of interest.
1. John GC, Kreiss J. Mother-to-child transmission of human immunodeficiency virus type 1. Epidemiol Rev (1996) 18(2):149–57. doi: 10.1093/oxfordjournals.epirev.a017922
2. Burns DN, Mofenson LM. Pediatric HIV-1 infection. Lancet (1999) 354(Suppl 2):SII1–6. doi:10.1016/S0140-6736(99)90249-0
3. Kind C, Rudin C, Siegrist CA, Wyler CA, Biedermann K, Lauper U, et al. Prevention of vertical HIV transmission: additive protective effect of elective Cesarean section and zidovudine prophylaxis. AIDS (1998) 12:205–10. doi:10.1097/00002030-199802000-00011
4. European Collaborative Study. Mother-to-child transmission of HIV infection in the era of highly active antiretroviral therapy. Clin Infect Dis (2005) 40:458–65. doi:10.1086/427287
5. Chasela CS, Hudgens MG, Jamieson DJ, Kayira D, Hosseinipour MC, Kourtis AP, et al. Maternal or infant antiretroviral drugs to reduce HIV-1 transmission. N Engl J Med (2010) 362(24):2271–81. doi:10.1056/NEJMoa0911486
6. Shapiro RL, Hughes MD, Ogwu A, Kitch D, Lockman S, Moffat C, et al. Antiretroviral regimens in pregnancy and breast feeding in Botswana. N Engl J Med (2010) 362(24):2282–94. doi:10.1056/NEJMoa0907736
7. Marinda E, Humphrey JH, Iliff PJ, Mutasa K, Nathoo KJ, Piwoz EG, et al. Child mortality according to maternal and infant HIV status in Zimbabwe. Pediatr Infect Dis J (2007) 26(6):519–26. doi:10.1097/01.inf.0000264527.69954.4c
8. Kuhn L, Kasonde P, Sinkala M, Kankasa C, Semrau K, Scott N, et al. Does severity of HIV disease in HIV-infected mothers affect mortality and morbidity among their uninfected infants? Clin Infect Dis (2005) 41(11):1654–61. doi:10.1086/498029
9. Newell ML, Coovadia H, Cortina-Borja M, Rollins N, Gaillard P, Dabis F, et al. Mortality of infected and uninfected infants born to HIV-infected mothers in Africa: a pooled analysis. Lancet (2004) 364(9441):1236–43. doi:10.1016/S0140-6736(04)17140-7
10. Filteau S. The HIV-exposed, uninfected African child. Trop Med Int Health (2009) 14(3):276–87. doi:10.1111/j.1365-3156.2009.02220.x
11. Singh HK, Gupte N, Kinikar A, Bharadwaj R, Sastry J, Suryavanshi N, et al. High rates of all-cause and gastroenteritis-related hospitalization morbidity and mortality among HIV-exposed Indian infants. BMC Infect Dis (2011) 11:193. doi:10.1186/1471-2334-11-193
12. Epalza C, Goetghebuer T, Hainaut M, Prayez F, Barlow P, Dediste A, et al. High incidence of invasive group B streptococcal infections in HIV-exposed uninfected infants. Pediatrics (2010) 126(3):e631–8. doi:10.1542/peds.2010-0183
13. Kuhn L, Thea DM, Aldrovandi GM. Bystander effects: children who escape infection but not harm. J Acquir Immune Defic Syndr (2007) 46(5):517–8. doi:10.1097/QAI.0b013e31814d6600
14. Mussi-Pinhata MM, Motta F, Freimanis-Hance L, de Souza R, Szyld E, Succi RC, et al. Lower respiratory tract infections among human immunodeficiency virus-exposed, uninfected infants. Int J Infect Dis (2010) 14(Suppl 3):e176–82. doi:10.1016/j.ijid.2010.01.006
15. Koyanagi A, Humphrey JH, Ntozini R, Nathoo K, Moulton LH, Iliff P, et al. Morbidity among human immunodeficiency virus-exposed but uninfected, human immunodeficiency virus-infected, and human immunodeficiency virus-unexposed infants in Zimbabwe before availability of highly active antiretroviral therapy. Pediatr Infect Dis J (2011) 30(1):45–51. doi:10.1097/INF.0b013e3181ecbf7e
16. McNally LM, Jeena PM, Gajee K, Thula SA, Sturm AW, Cassol S, et al. Effect of age, polymicrobial disease, and maternal HIV status on treatment response and cause of severe pneumonia in South African children: a prospective descriptive study. Lancet (2007) 369(9571):1440–51. doi:10.1016/S0140-6736(07)60670-9
17. Fawzy A, Arpadi S, Kankasa C, Sinkala M, Mwiya M, Thea DM, et al. Early weaning increases diarrhea morbidity and mortality among uninfected children born to HIV-infected mothers in Zambia. J Infect Dis (2011) 203(9):1222–30. doi:10.1093/infdis/jir019
18. Slogrove A, Reikie B, Naidoo S, De Beer C, Ho K, Cotton M, et al. HIV-exposed uninfected infants are at increased risk for severe infections in the first year of life. J Trop Pediatr (2012) 58(6):505–8. doi:10.1093/tropej/fms019
19. Adler C, Haelterman E, Barlow P, Marchant A, Levy J, Goetghebuer T. Severe infections in HIV-exposed uninfected infants born in a European country. PLoS One (2015) 10(8):e0135375. doi:10.1371/journal.pone.0135375
20. Mussi-Pinhata MM, Freimanis L, Yamamoto AY, Korelitz J, Pinto JA, Cruz ML, et al. Infectious disease morbidity among young HIV-1-exposed but uninfected infants in Latin American and Caribbean countries: the National Institute of Child Health and Human Development International Site Development Initiative Perinatal Study. Pediatrics (2007) 119(3):e694–704. doi:10.1542/peds.2006-1856
21. Afran L, Garcia Knight M, Nduati E, Urban BC, Heyderman RS, Rowland-Jones SL. HIV-exposed uninfected children: a growing population with a vulnerable immune system?: HEU children: a vulnerable immune system? Clin Exp Immunol (2014) 176(1):11–22. doi:10.1111/cei.12251
22. Brinkman K, ter Hofstede HJ, Burger DM, Smeitink JA, Koopmans PP. Adverse effects of reverse transcriptase inhibitors: mitochondrial toxicity as common pathway. AIDS (1998) 12(14):1735–44. doi:10.1097/00002030-199814000-00004
23. Venhoff N, Walker UA. Mitochondrial disease in the offspring as a result of antiretroviral therapy. Expert Opin Drug Saf (2006) 5(3):373–81. doi:10.1517/14740338.5.3.373
24. Foster C, Lyall H. HIV and mitochondrial toxicity in children. J Antimicrob Chemother (2008) 61(1):8–12. doi:10.1093/jac/dkm411
25. Scruggs ER, Dirks Naylor AJ. Mechanisms of zidovudine-induced mitochondrial toxicity and myopathy. Pharmacology (2008) 82(2):83–8. doi:10.1159/000134943
26. Yamaguchi T, Katoh I, Kurata S. Azidothymidine causes functional and structural destruction of mitochondria, glutathione deficiency and HIV-1 promoter sensitization: AZT causes mitochondrial dysfunction. Eur J Biochem (2002) 269(11):2782–8. doi:10.1046/j.1432-1033.2002.02954.x
27. Dainiak N, Worthington M, Riordan MA, Kreczko S, Goldman L. 3′-Azido-3′-deoxythymidine (AZT) inhibits proliferation in vitro of human haematopoietic progenitor cells. Br J Haematol (1988) 69(3):299–304. doi:10.1111/j.1365-2141.1988.tb02366.x
28. Pacheco SE, McIntosh K, Lu M, Mofenson LM, Diaz C, Foca M, et al. Effect of perinatal antiretroviral drug exposure on hematologic values in HIV-uninfected children: an analysis of the women and infants transmission study. J Infect Dis (2006) 194(8):1089–97. doi:10.1086/507645
29. Ackerman WIV, Kwiek JJ. Role of the placenta in adverse perinatal outcomes among HIV-1 seropositive women. J Nippon Med Sch (2013) 80(2):90–4. doi:10.1272/jnms.80.90
30. Sewankambo N, Gray RH, Wawer MJ, Paxton L, McNairn D, Wabwire-Mangen F, et al. HIV-1 infection associated with abnormal vaginal flora morphology and bacterial vaginosis. Lancet (1997) 350(9077):546–50. doi:10.1016/S0140-6736(97)01063-5
31. Nielsen SD, Jeppesen DL, Kolte L, Clark DR, Sørensen TU, Dreves AM, et al. Impaired progenitor cell function in HIV-negative infants of HIV-positive mothers results in decreased thymic output and low CD4 counts. Blood (2001) 98(2):398–404. doi:10.1182/blood.V98.2.398
32. Bunders M, Thorne C, Newell ML; European Collaborative Study. Maternal and infant factors and lymphocyte, CD4 and CD8 cell counts in uninfected children of HIV-1-infected mothers. AIDS (2005) 19(10):1071–9. doi:10.1097/01.aids.0000174454.63250.22
33. Le Chenadec J, Mayaux MJ, Guihenneuc-Jouyaux C, Blanche S; Enquête Périnatale Française Study Group. Perinatal antiretroviral treatment and hematopoiesis in HIV-uninfected infants. AIDS (2003) 17(14):2053–61. doi:10.1097/00002030-200309260-00006
34. Clerici M, Saresella M, Colombo F, Fossati S, Sala N, Bricalli D, et al. T-lymphocyte maturation abnormalities in uninfected newborns and children with vertical exposure to HIV. Blood (2000) 96(12):3866–71.
35. Kakkar F, Lamarre V, Ducruet T, Boucher M, Valois S, Soudeyns H, et al. Impact of maternal HIV-1 viremia on lymphocyte subsets among HIV-exposed uninfected infants: protective mechanism or immunodeficiency. BMC Infect Dis (2014) 14:236. doi:10.1186/1471-2334-14-236
36. Miles DJC, Gadama L, Gumbi A, Nyalo F, Makanani B, Heyderman RS. Human immunodeficiency virus (HIV) infection during pregnancy induces CD4 T cell differentiation and modulates responses to Bacille Calmette-Guérin (BCG) vaccine in HIV-uninfected infants. Immunology (2010) 129(3):446–54. doi:10.1111/j.1365-2567.2009.03186.x
37. Miyamoto M, Pessoa SD, Ono E, Machado DM, Salomao R, Succi RC, et al. Low CD4+ T cell levels and B-cell apoptosis in vertically HIV exposed noninfected children and adolescents. J Trop Pediatr (2010) 56(6):427–32. doi:10.1093/tropej/fmq024
38. Rich K, Siegel JN, Jennings C, Rydman RJ, Landay AL. Function and phenotype of immature CD4+ lymphocytes in healthy infants and early lymphocyte activation in uninfected infants of human immunodeficiency virus-infected mothers. Clin Diagn Lab Immunol (1997) 4(3):358–61.
39. Clerici M, Sison AV, Berzofsky JA, Rakusan TA, Brandt CD, Ellaurie M. Cellular immune factors associated with mother-to-infant transmission of HIV. AIDS (1993) 7(11):1427–33. doi:10.1097/00002030-199311000-00004
40. Kuhn L, Coutsoudis A, Moodley D, Trabattoni D, Mngqundaniso N, Shearer GM, et al. T-helper cell responses to HIV envelope peptides in cord blood: protection against intrapartum and breastfeeding transmission. AIDS (2001) 15(1):1–9. doi:10.1097/00002030-200101050-00003
41. Hygino J, Lima PG, Filho RG, Silva AA, Saramago CS, Andrade RM, et al. Altered immunological reactivity in HIV-1-exposed uninfected neonates. Clin Immunol (2008) 127(3):340–7. doi:10.1016/j.clim.2008.01.020
42. Chougnet C, Kovacs A, Baker R, Mueller BU, Luban NL, Liewehr DJ, et al. Influence of human immunodeficiency virus-infected maternal environment on development of infant interleukin-12 production. J Infect Dis (2000) 181(5):1590–7. doi:10.1086/315458
43. Mazzola TN, da Silva MTN, Abramczuk BM, Moreno YMF, Lima SCBS, Zorzeto TQ, et al. Impaired Bacillus Calmette-Guérin cellular immune response in HIV-exposed, uninfected infants. AIDS (2011) 25(17):2079–87. doi:10.1097/QAD.0b013e32834bba0a
44. Kidzeru EB, Hesseling AC, Passmore JAS, Myer L, Gamieldien H, Tchakoute CT, et al. In-utero exposure to maternal HIV infection alters T cell immune responses to vaccination in HIV-uninfected infants. AIDS (2014) 28(10):1421–30. doi:10.1097/QAD.0000000000000292
45. Cheynier R, Langlade-Demoyen P, Marescot MR, Blanche S, Blondin G, Wain-Hobson S, et al. Cytotoxic T lymphocyte responses in the peripheral blood of children born to human immunodeficiency virus-1-infected mothers. Eur J Immunol (1992) 22(9):2211–7. doi:10.1002/eji.1830220905
46. Rowland-Jones SL, Nixon DF, Aldhous MC, Gotch F, Ariyoshi K, Hallam N, et al. HIV-specific cyotoxic T-cell activity in an HIV-exposed but uninfected infant. Lancet (1993) 341(8849):860–1. doi:10.1016/0140-6736(93)93063-7
47. Aldhous MC, Watret KC, Mok JY, Bird AG, Froebel KS. Cytotoxic T lymphocyte activity and CD8 subpopulations in children at risk of HIV infection. Clin Exp Immunol (1994) 97(1):61–7. doi:10.1111/j.1365-2249.1994.tb06580.x
48. De Maria A, Cirillo C, Moretta L. Occurrence of human immunodeficiency virus type 1 (HIV-1)-specific cytolytic T cell activity in apparently uninfected children born to HIV-1-infected mothers. J Infect Dis (1994) 170(5):1296–9. doi:10.1093/infdis/170.5.1296
49. Boyman O, Sprent J. The role of interleukin-2 during homeostasis and activation of the immune system. Nat Rev Immunol (2012) 12(3):180–90. doi:10.1038/nri3156
50. Bunders MJ, van Hamme JL, Jansen MH, Boer K, Kootstra NA, Kuijpers TW. Fetal exposure to HIV-1 alters chemokine receptor expression by CD4+T cells and increases susceptibility to HIV-1. Sci Rep (2014) 4:6690. doi:10.1038/srep06690
51. Kuhn L, Coutsoudis A, Moodley D, Mngqundaniso N, Trabattoni D, Shearer GM, et al. Interferon-gamma and interleukin-10 production among HIV-1-infected and uninfected infants of HIV-1-infected mothers. Pediatr Res (2001) 50(3):412–6. doi:10.1203/00006450-200109000-00018
52. Jardetzky TS, Brown JH, Gorga JC, Stern LJ, Urban RG, Chi YI, et al. Three-dimensional structure of a human class II histocompatibility molecule complexed with superantigen. Nature (1994) 368(6473):711–8. doi:10.1038/368711a0
53. Hayball JD, Robinson JH, O’Hehir RE, Verhoef A, Lamb JR, Lake RA. Identification of two binding sites in staphylococcal enterotoxin B that confer specificity for TCR V beta gene products. Int Immunol (1994) 6(2):199–211. doi:10.1093/intimm/6.2.199
54. Hsu PN, Huber BT. Superantigens. Gazing into the crystal ball. Curr Biol (1995) 5(3):235–7. doi:10.1016/S0960-9822(95)00047-9
55. Krakauer T. Update on staphylococcal superantigen-induced signaling pathways and therapeutic interventions. Toxins (Basel) (2013) 5(9):1629–54. doi:10.3390/toxins5091629
56. Kanellopoulos JM, De Petris S, Leca G, Crumpton MJ. The mitogenic lectin from Phaseolus vulgaris does not recognize the T3 antigen of human T lymphocytes. Eur J Immunol (1985) 15(5):479–86. doi:10.1002/eji.1830150512
57. Schneider OD, Millen SH, Weiss AA, Miller WE. Mechanistic insight into pertussis toxin and lectin signaling using T cells engineered to express a CD8α/CD3ζ chimeric receptor. Biochemistry (2012) 51(20):4126–37. doi:10.1021/bi3002693
58. Valentine MA, Tsoukas CD, Rhodes G, Vaughan JH, Carson DA. Phytohemagglutinin binds to the 20-kDa molecule of the T3 complex. Eur J Immunol (1985) 15(8):851–4. doi:10.1002/eji.1830150821
59. Linsley PS, Ledbetter JA. The role of the CD28 receptor during T cell responses to antigen. Annu Rev Immunol (1993) 11:191–212. doi:10.1146/annurev.iy.11.040193.001203
60. Mwanyumba F, Gaillard P, Inion I, Verhofstede C, Claeys P, Chohan V, et al. Placental inflammation and perinatal transmission of HIV-1. J Acquir Immune Defic Syndr (2002) 29(3):262–9. doi:10.1097/00042560-200203010-00006
61. Legrand FA, Nixon DF, Loo CP, Ono E, Chapman JM, Miyamoto M, et al. Strong HIV-1-specific T cell responses in HIV-1-exposed uninfected infants and neonates revealed after regulatory T cell removal. PLoS One (2006) 1:e102. doi:10.1371/journal.pone.0000102
62. Abu-Raya B, Smolen KK, Willems F, Kollmann TR, Marchant A. Transfer of maternal antimicrobial immunity to HIV-exposed uninfected newborns. Front Immunol (2016) 7:338. doi:10.3389/fimmu.2016.00338
63. Reikie BA, Naidoo S, Ruck CE, Slogrove AL, de Beer C, la Grange H, et al. Antibody responses to vaccination among South African HIV-exposed and unexposed uninfected infants during the first 2 years of life. Clin Vaccine Immunol (2013) 20(1):33–8. doi:10.1128/CVI.00557-12
64. Simani OE, Izu A, Violari A, Cotton MF, van Niekerk N, Adrian PV, et al. Effect of HIV-1 exposure and antiretroviral treatment strategies in HIV-infected children on immunogenicity of vaccines during infancy. AIDS (2014) 28(4):531–41. doi:10.1097/QAD.0000000000000127
65. Abramczuk BM, Mazzola TN, Moreno YM, Zorzeto TQ, Quintilio W, Wolf PS, et al. Impaired humoral response to vaccines among HIV-exposed uninfected infants. Clin Vaccine Immunol (2011) 18(9):1406–9. doi:10.1128/CVI.05065-11
66. Rutstein RM, Rudy B, Codispoti C, Watson B. Response to hepatitis B immunization by infants exposed to HIV. AIDS (1994) 8(9):1281–4. doi:10.1097/00002030-199409000-00010
67. Singh DK, Kumar R, Rai R, Maurya M, Bhargava A. Immunogenicity of hepatitis B vaccine in HIV exposed uninfected infants. Indian J Pediatr (2016) 83(2):172–4. doi:10.1007/s12098-015-1905-1
68. Jones CE, Naidoo S, De Beer C, Esser M, Kampmann B, Hesseling AC. Maternal HIV infection and antibody responses against vaccine-preventable diseases in uninfected infants. JAMA (2011) 305(6):576–84. doi:10.1001/jama.2011.100
69. Madhi SA, Izu A, Violari A, Cotton MF, Panchia R, Dobbels E, et al. Immunogenicity following the first and second doses of 7-valent pneumococcal conjugate vaccine in HIV-infected and -uninfected infants. Vaccine (2013) 31(5):777–83. doi:10.1016/j.vaccine.2012.11.076
70. Gaensbauer JT, Rakhola JT, Onyango-Makumbi C, Mubiru M, Westcott JE, Krebs NF, et al. Impaired haemophilus influenzae type b transplacental antibody transmission and declining antibody avidity through the first year of life represent potential vulnerabilities for HIV-exposed but -uninfected infants. Clin Vaccine Immunol (2014) 21(12):1661–7. doi:10.1128/CVI.00356-14
71. Simani OE, Adrian PV, Violari A, Kuwanda L, Otwombe K, Nunes MC, et al. Effect of in-utero HIV exposure and antiretroviral treatment strategies on measles susceptibility and immunogenicity of measles vaccine. AIDS (2013) 27(10):1583–91. doi:10.1097/QAD.0b013e32835fae26
72. Siberry GK, Patel K, Bellini WJ, Karalius B, Purswani MU, Burchett SK, et al. Immunity to measles, mumps, and rubella in US children with perinatal HIV infection or perinatal HIV exposure without infection. Clin Infect Dis (2015) 61(6):988–95. doi:10.1093/cid/civ440
73. David MC, Ha SH, Paynter S, Lau C. A systematic review and meta-analysis of management options for adults who respond poorly to hepatitis B vaccination. Vaccine (2015) 33(48):6564–9. doi:10.1016/j.vaccine.2015.09.051
74. Bunders MJ, Bekker V, Scherpbier HJ, Boer K, Godfried M, Kuijpers TW. Haematological parameters of HIV-1-uninfected infants born to HIV-1-infected mothers. Acta Paediatr (2005) 94(11):1571–7. doi:10.1080/08035250510042951
75. European Collaborative Study. Levels and patterns of neutrophil cell counts over the first 8 years of life in children of HIV-1-infected mothers. AIDS (2004) 18(15):2009–17. doi:10.1097/00002030-200410210-00005
76. Reikie BA, Adams RCM, Leligdowicz A, Ho K, Naidoo S, Ruck CE, et al. Altered innate immune development in HIV-exposed uninfected infants. J Acquir Immune Defic Syndr (2014) 66(3):245–55. doi:10.1097/QAI.0000000000000161
77. Velilla PA, Montoya CJ, Hoyos A, Moreno ME, Chougnet C, Rugeles MT. Effect of intrauterine HIV-1 exposure on the frequency and function of uninfected newborns’ dendritic cells. Clin Immunol (2008) 126(3):243–50. doi:10.1016/j.clim.2007.11.004
78. Slyker JA, Lohman-Payne B, John-Stewart GC, Dong T, Mbori-Ngacha D, Tapia K, et al. The impact of HIV-1 infection and exposure on natural killer (NK) cell phenotype in Kenyan infants during the first year of life. Front Immunol (2012) 3:399. doi:10.3389/fimmu.2012.00399
79. Gribaldo L, Malerba I, Collotta A, Casati S, Pessina A. Inhibition of CFU-E/BFU-E by 3′-azido-3′-deoxythymidine, chlorpropamide, and protoporphirin IX zinc (II): a comparison between direct exposure of progenitor cells and long-term exposure of bone marrow cultures. Toxicol Sci (2000) 58(1):96–101. doi:10.1093/toxsci/58.1.96
80. Fowler DA, Xie MY, Sommadossi JP. Protection and rescue from 2′,3′-dideoxypyrimidine nucleoside analog toxicity by hemin in human bone marrow progenitor cells. Antimicrob Agents Chemother (1996) 40(1):191–5.
81. Collin M, McGovern N, Haniffa M. Human dendritic cell subsets. Immunology (2013) 140(1):22–30. doi:10.1111/imm.12117
Keywords: HIV, HIV-exposed infants, innate immune system, cell-mediated immune system, humoral immune response
Citation: Abu-Raya B, Kollmann TR, Marchant A and MacGillivray DM (2016) The Immune System of HIV-Exposed Uninfected Infants. Front. Immunol. 7:383. doi: 10.3389/fimmu.2016.00383
Received: 01 July 2016; Accepted: 13 September 2016;
Published: 28 September 2016
Edited by:
Claire Anne Chougnet, Cincinnati Children’s Hospital Medical Center Research Foundation, USAReviewed by:
Andrea Cossarizza, University of Modena and Reggio Emilia, ItalyCopyright: © 2016 Abu-Raya, Kollmann, Marchant and MacGillivray. This is an open-access article distributed under the terms of the Creative Commons Attribution License (CC BY). The use, distribution or reproduction in other forums is permitted, provided the original author(s) or licensor are credited and that the original publication in this journal is cited, in accordance with accepted academic practice. No use, distribution or reproduction is permitted which does not comply with these terms.
*Correspondence: Bahaa Abu-Raya, YmFoYWEuYWJ1cmF5YUBjdy5iYy5jYQ==
Disclaimer: All claims expressed in this article are solely those of the authors and do not necessarily represent those of their affiliated organizations, or those of the publisher, the editors and the reviewers. Any product that may be evaluated in this article or claim that may be made by its manufacturer is not guaranteed or endorsed by the publisher.
Research integrity at Frontiers
Learn more about the work of our research integrity team to safeguard the quality of each article we publish.