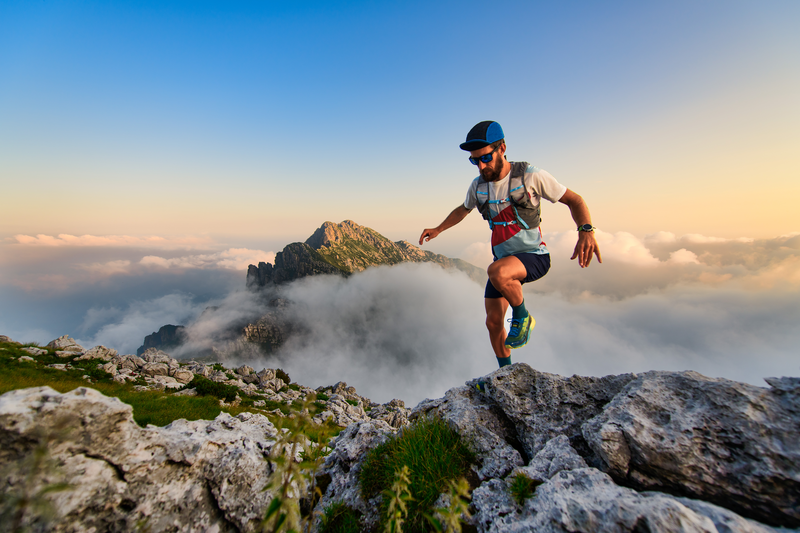
94% of researchers rate our articles as excellent or good
Learn more about the work of our research integrity team to safeguard the quality of each article we publish.
Find out more
REVIEW article
Front. Immunol. , 13 June 2016
Sec. Molecular Innate Immunity
Volume 7 - 2016 | https://doi.org/10.3389/fimmu.2016.00229
The transmembrane protein TIM-3 is a type I protein expressed by sub-types of lymphoid cells, such as lymphocytes Th1, Th17, Tc1, NK, as well as in myeloid cells. Scientific evidence indicates that this molecule acts as a negative regulator of T lymphocyte activation and that its expression is modified in viral infections or autoimmune diseases. In addition to evidence from lymphoid cells, the function of TIM-3 has been investigated in myeloid cells, such as monocytes, macrophages, and dendritic cells (DC), where studies have demonstrated that it can regulate cytokine production, cell activation, and the capture of apoptotic bodies. Despite these advances, the function of TIM-3 in myeloid cells and the molecular mechanisms that this protein regulates are not yet fully understood. This review examines the most recent evidence concerning the function of TIM-3 when expressed in myeloid cells, primarily macrophages, and the potential impact of that function on the field of basic immunology.
The proteins of the TIM family (T cell immunoglobulin and mucin domain) are expressed on the surface of diverse cell types of the immune system (1). They were first described in mice T lymphocytes (TIM-1, TIM-2, TIM-3, and TIM-4) and next in humans (TIM-1, TIM-3, and TIM-4) (2–5). Homologs of TIM proteins have been identified in other species as well, including monkeys and rats (2, 6). These proteins can regulate diverse effectors pathways of the immune response. TIM-3 is expressed in CD4+ T cells that secrete interferon-gamma (IFN-γ), CD8+ T cells, dendritic cell (DC), and macrophages, among others. Also, it has been proposed as a regulator of the Th1 type immune response (7). Evidence gathered to date suggests both negative (inhibition) and positive (activation) regulatory functions on diverse components of the immune response. These data underline the importance of determining whether the function of TIM-3 differs in the diverse cell types in which it is expressed.
The first protein of this family discovered (TIM-1) was described as a marker of renal damage and associated with adhesion functions in a murine model (3, 4). The association of TIM proteins with immunological functions began to be deduced from a study that compared the presence of polymorphisms in BALB/C and DBA/2 mice by the genetic mapping of congenic animals, in order to identify the locus responsible for variations in susceptibility to asthma (5). That study confirmed the presence of the locus called T cell and airway phenotype regulator (Tapr) that groups a series of genes found in a region previously associated with susceptibility to asthma (5), which includes, among others, genes of the TIM family (5). Since then, the function of these proteins has been studied in several in vivo and in vitro experimental models to evaluate its participation during the immune response of autoimmune diseases, bacterial and viral infections, and pathologies with oncogenic origin.
In mice, the family of TIM proteins includes eight genes, of which only four codify for functional proteins (TIM-1 to TIM-4). In humans, three genes have been identified that codify for TIM-1, TIM-3, and TIM-4 (8). Each one of these genes codifies for a type I protein localized in the cell’s plasma membrane. The TIM-3 protein is 281 amino acids in size, with a total homology of 77% with the murine protein (7). The structure of the TIM proteins consists of four well-defined regions.
1. Variable immunoglobulin domain (IgV),
2. Mucin domain,
3. Transmembrane region, and
4. Intracellular stem.
The IgV domain contains two anti-parallel chains, where four cysteines can be identified in the variable region of all members of this family, suggesting that they are highly conserved regions. Although it shares some characteristics with the IgV domains of a more conventional structure, the IgV domain of TIM-3 contains two disulfide bridges in the four cysteines conserved from the TIM proteins (9), a characteristic shared by the entire TIM family. In most IgV domains of diverse proteins, there are well-defined structures called CC′ loop and FG loop, named after the folded beta sheets that make up interactions established by the disulfide bonds. In the super-family of conventional immunoglobulins, the CC′ and FG loops are located at opposite ends of the IgV domain, at an approximate distance of 25 Å, but in TIM-1, 3, and 4 proteins, a distinct spatial arrangement has been identified due to the presence of disulfide bonds formed between the four cysteines, which re-orient the CC′ loop more proximal to the FG loop, creating a unique cleft in the IgV domain of the proteins of this family (9) (Figure 1). This characteristic cleft of the TIM proteins is stabilized by disulfide and hydrogen bonds (9). It is possible that this peculiar structure may modify the biological function of the IgV domain of TIM-3, though this has not yet been demonstrated. The two ligands known for TIM-3 – galectin-9 (Gal-9) and phosphatidylserine (PS) – bond to the protein’s IgV domain. Although there are glycosylation sites at the extracellular domain of TIM-3, it has been shown that only IgV glycosylation is required for Gal-9 binding (10). On the other hand, the PS binding to TIM-3 does not require additional glycosylations (9, 11).
Figure 1. Comparative schemes of the PD-1 (left panel) and TIM-3 (right panel) proteins showing the different conformation of the variable immunoglobulin domain (IgV). In a conventional IgV domain like that of PD-1, the FG and CC′ loops are spatially distant (25 Å), while in the IgV domain of TIM-3, the distance between them is smaller due to the presence of disulfide bonds. Thus, a distinct spatial conformation is formed in TIM-3 compared to the other proteins that contain immunoglobulin domains (9, 17). [Glycosylation sites within the IgV domain (9) have been omitted to simplify.]
The mucin domain varies considerably in length among different members of this family. TIM-3 is the molecule with the smallest domain (8). This region is especially rich in threonine, proline, and serine (9). TIM proteins cross the cell membrane by means of a tail embedded in the lipid bilayer. This tail is formed primarily of hydrophobic amino acids and penetrates into the interior of the cell with a cytoplasmic domain of ~42–77 amino acids in size. This is the most similar domain in humans and mice (8). Within the intracellular region of TIM-1 and TIM-3, there are phosphorylation sites, but in the former case, they are of two sub-types: one called TIM-1a that is expressed mainly in human liver cells and does not contain the amino acid sequence on which the kinase enzyme acts and the other, TIM-1b, is found primarily in human kidneys and conserves two threonine residues that make it susceptible to phosphorylation (8).
Based on the knowledge that TIM-2 transduces intracellular signals by phosphorylation of tyrosine residues, it was considered that this mechanism could also be present in TIM-3 (12). It has been demonstrated that the intracellular signaling of TIM-3 begins after its interaction with Gal-9 and when the tyrosine 265 (Y265) located at the cytoplasmic tail is phosphorylated by the interleukin inducible T-cell kinase (ITK) (13). Additionally, it has also been documented that the kinases Fyn and Lck can mediate a more efficient phosphorylation of TIM-3 (13). Finally, Rangachari et al. demonstrated that the Bat-3 protein (human leukocyte antigen B-associated transcript 3) is associated with the intracellular portion of TIM-3 in T lymphocytes and Bat-3 recruits a kinase of the Src family (Lck) (14). Thus, determining whether the intracellular signaling of TIM-3 in the macrophage involves Bat-3 and some kinase of the Src family will be fundamental as we strive to achieve a better understanding of the functioning of this protein. There is evidence to suggest that the Lck protein is not expressed in macrophages, but may express some isoform; therefore, the functioning of the TIM-3 receptor could differ in cells of myeloid origin. Conducting research in this area will allow us to learn more about the differences that exist in the function of TIM-3 between lymphocytes and myeloid cells. All these evidences allow us to consider that intracellular phosphorylation of TIM-3 is mediated by different kinases and with different levels of efficiency. This final regulation may have a direct impact on TIM-3 function when expressed by different cells.
Although the TIM-3 protein was initially identified as a membrane marker specific for Th1 and Tc1 (7) lymphocytes, its expression was soon confirmed in other cells (Table 1) (7, 15, 16). Today, we know that it is expressed in monocytes, macrophages, DC, NK cells, and even in a variety of cells in different types of tumors (3, 13–15, 17). Scientific findings suggest that TIM-3 and its ligand Gal-9 function as a negative regulatory pathway in T cell activation. The failure, or absence, of this pathway has been associated with the development of autoimmune diseases (18–22); however, it is important to determine its function in other cells, such as monocytes or macrophages, since some reports suggest that TIM-3 can function as a molecule that participates in cell activation and the elimination of different pathogens (23, 24).
Efferocytosis or elimination of apoptotic cells and bodies is a physiologic and vital mechanism that avoids tissue inflammation and autoimmune responses. It was first described by de Cathelineau and Henson (25) to differentiate between macropinocytosis and opsonization. Efferocytosis is a mechanism that maintains immune tolerance while allowing development of antibacterial immune responses (26, 27). Recent evidence suggests that TIM proteins play a role in efferocytosis. The receptors utilized by phagocytic cells to internalize the detritus of apoptotic cells include some members of the TIM family. TIM-1 and 4 were the first proteins of this family in which the bonding site to PS was identified. PS is a phospholipid that translocates to the external face of the plasma membrane of apoptotic cells, and the principal “eat me” signal that triggers their capture and elimination (28). This early evidence suggested that TIM-3 could bond to phospholipids. Since then, crystallography studies have proven that TIM-3 does indeed bond to PS via an FG loop located in the IgV domain (9, 29). This mechanism was identified initially in CD8+ DC and macrophages in an in vitro model, and its presence was confirmed later in a murine model. Since PS is the principle signal for the phagocytosis of apoptotic bodies or cells, when recognition of this TIM-3-mediated phospholipid is blocked with specific antibodies apoptotic bodies are not removed, and this can induce immunological abnormalities, such as the generation of autoantibodies (29, 30). This scientific evidence agrees with the results obtained on lymphocytes upon evaluating the function of TIM-3 in autoimmune-type diseases, such as experimental autoimmune encephalitis (EAE) and multiple sclerosis, where research has documented that reducing TIM-3 expression is associated with an aggravated state of the disease and a high frequency of autoreactive cells (18). While these studies support the function of TIM-3 promoting immunological tolerance, they also raise new questions about this protein. For example, it is necessary to clarify why CD8+ DC can capture, phagocyte, and favor antigen presentation, while CD8− DC do not perform these functions although they also express TIM-3 and are capable of responding to the presence of apoptotic bodies by producing inflammatory cytokines (29). Studies have also confirmed that allelic variants of TIM-3, that differ in seven amino acids in the FG loop and come from two strains of mice (BALB/c and HBA), possess distinct abilities to interact with PS (31). It has been demonstrated that phagocytic cells from the BALB/c strain bond to, and phagocyte, apoptotic cells by means of TIM-3, while the cells that express the HBA variant of TIM-3 can form complexes with PS, but are incapable of internalizing apoptotic bodies (31). But these findings are not enough to clarify the differences in the function of TIM-3 in the different cell types that express it. For example, TIM-3 expression has been demonstrated on peritoneal exudative macrophages, but not on peritoneal resident macrophages. However, TIM-4 is highly expressed on peritoneal resident macrophages and binds to PS on apoptotic cells from the peritoneal cavity. The different expression profile and function of TIM-3 compared with TIM-4 is not redundant and is important for maintaining immune tolerance. These results may indicate that different macrophage subsets could use different molecules (TIM-3 or TIM-4) to recognize PS (29).
Available evidence seems to suggest that the function of TIM-3 changes depending on the type of cell in which it is expressed but that the level of expression seems to contribute as well; for example, CD8+ DC have a level of expression of TIM-3 three times greater than CD8− DC (29). Moreover, phagocytic activity of each cell subset is the result of various considerations as the level of expression of the phagocytic receptors, the microenvironment where the cells are located as well as their cytoskeletal architecture. For example, when TIM-3 is expressed by lymphocytes (32), these cells do not recognize apoptotic cells or bodies; however, macrophages or DCs expressing TIM-3 do phagocyte. For this reason, it is important to conduct functional studies comparing TIM-3+ vs. TIM-3− cells and identify the signaling pathways that define the role of this protein in each cell type.
It has been demonstrated that TIM-3 expression is dynamic, regulates the activation of diverse cells, and has the capacity to induce the production of distinct cytokines. This has been shown for monocytes and macrophages that express TIM-3 in a constant manner (Figure 2). In both the THP-1 cell line and CD14+ cells purified from human peripheral blood, studies have confirmed that monocytes/macrophages in a quiescent state have a high expression of TIM-3 with low cytokine production. When stimulated in vitro with LPS and the R848 compound (ligands for TLR4 and TLR7/8, respectively), these macrophages reduce the expression of TIM-3 in a concentration-dependent manner, while also beginning to produce IL-12 after stimulus reception (33). Blocking TIM-3 with monoclonal antibodies and posterior stimulation with TLR results in an increase in the production of IL-12, IL-6, and IL-10, but a reduced expression of PD-1, an inhibitor molecule of the T cell function. However, experiments made on THP-1 macrophages demonstrated that after silencing TIM-3 expression with siRNA, the production of IL-12 and IL-10 was increased (33, 34). These findings confirm that IL-12 expression is negatively regulated by TIM-3, contrary to what occurs with PD-1, which increases together with TIM-3. Another important finding is that when TIM-3 is expressed on other cells (trans) or on the macrophage itself (cis), the association of TIM-3 with its ligand, Gal-9, has a distinct effect as a regulator of TLR-mediated activation (35). For example, the trans association of TIM-3 and Gal-9 negatively regulates TLR signaling, leading to an IL-12 reduction, increase in the IL-23 production, decrease in the phosphorylation of STAT-1, and/or activation of STAT-3. Meanwhile, the association in cis favors the correct TLR signaling, and it has been demonstrated that expression of Gal-9 increases through this mechanism (35).
Figure 2. Potential role of TIM-3 on macrophages. (A) Macrophages with high expression of TIM-3 do not produce cytokines, favoring immune tolerance and reduce TLR activation. In the presence of the TLR ligands, LPS and R848, macrophage activation reduces expression of TIM-3 and lessens the regulatory effect mediated by this molecule, allowing correct activation and the consequent production of cytokines. (B) By silencing the expression of TIM-3 with siRNA or blocking antibodies, it is possible to induce correct macrophage activation similar to that observed on T cells.
Unlike the negative regulator PD-1, which has been proposed as a therapeutic target in different types of cancer, the function of TIM-3 in cancer has just begun to be studied, so we know very little about its role in this pathology. However, the fact that its function and changes in the profile of cellular expression in pathological states is similar to those of PD-1 suggests that its function in the tumor microenvironment should be initially evaluated in the same context. We know that TIM-3 expression is not restricted to mononuclear cells, because it has also been detected in cancer cells. TIM-3 can be expressed in distinct types of cancer cells, including myeloid leukemia, sarcoma, gastric, cervical and pulmonary cancer, and osteosarcoma, among others (36–39). In many of these types of cancer, the increased expression of TIM-3 has been associated with disease progression and shorter survival.
In T lymphocytes, it has been documented that the interaction of TIM-3 with Gal-9 inhibits cell activation and induces apoptosis via a mechanism that involves calpain and caspase-1 (40). Hence, the activity of the T lymphocytes could modify the tumor microenvironment, but how does TIM-3 participate in cancer progression? and what is its role in the tumor microenvironment when this molecule is expressed in macrophages and DC? These issues remain to be clarified. With respect to the innate immune system, studies have been conducted with distinct types of cancer to analyze the role of TIM-3 as a negative regulator of the function of T lymphocyte-mediated adaptive immunity and innate response when this molecule is expressed in macrophages and DC. One murine model of hepatic cancer documented an increase in TIM-3 expression in peripheral blood monocytes and tumor-associated macrophages (TAMs), a finding that was positively correlated with disease progression (41). TAMs are characterized by presenting a phenotype denominated M2, characterized by the expression of CD163, CD206, Arg-1, and IL-10 (Figure 3) (41). Scientific evidence suggests that this phenotype, together with the increase in the expression of TIM-3, are induced by the TGF-β (41) and other cytokines, such as IL-4, IL-10, IL-13, and M-CSF found in the tumor microenvironment [reviewed by Allavena et al. (42)], which favor the alternative activation of macrophages. It is feasible that the M1 type macrophages that are activated by IFN-γ and that perform innate functions as phagocytosis, promoting antitumor responses, and eliminating pathogens and transformed cells, could re-direct their M1 phenotype to the M2 phenotype, since they lose or reduce many of the protective functions in cancer, thus promoting tumor development and progression (41). These events are enabled by the characteristic plasticity of macrophages that – unlike lymphocytes which are polarized toward one of the known effector profile and suffer significant modifications at the level of the chromatin – show a genetic profile that changes depending on the type, concentration, and time of exposure to diverse stimuli (43, 44).
Figure 3. TIM-3+ macrophages and its role in cancer progression. The M1 macrophages recruited to eliminate cancer cells have a low expression of TIM-3 and perform diverse functions, such as phagocytosis, presentation of antigens, and production of pro-inflammatory cytokines, such as IFN-γ, TNF-α, and IL-12. When cancer cells and lymphocytes release anti-inflammatory cytokines into the environment such as IL-4 and TGF-β, they induce a change in the macrophages toward a regulator or tolerogenic profile (M2), accompanied by an increase in TIM-3 expression and in the expression of the specific markers of the M2 phenotype, such as DC 163 and DC 206, among others (41, 50, 51).
Other mechanisms that may favor tumor development involve exhausted T lymphocytes and DC. There is evidence that in vitro interaction between monocytes and macrophages with autologous T lymphocytes with senescent phenotype induces higher production of pro-inflammatory cytokines, such as TNF-α, IL-1b, and IL-6, as well as a greater production of angiogenic molecules, such as VEGF-α, MMP9, and IL-8, compared to monocytes cocultivated with control cells (45). TIM-3 can also favor the development of metastasis through a still unknown mechanism. For example, it has been reported that the HeLa cell line reduces the capacity for metastasis when TIM-3 is silenced by antisense cDNA (37).
In summary, TIM-3 participates in diverse suppressor pathways of the antitumor response in both myeloid cells and lymphocytes. As in the case of infectious diseases, it has been documented that simultaneous blocking of TIM-3 and PD-1 is more efficient in restoring the functionality of exhausted lymphocytes (46) and perhaps also in counteracting some inhibitory molecules in the macrophages and DC. It is possible that in macrophages, TIM-3 functions as a receptor that, upon being treated with antibodies or agonist molecules, induces a more efficient immunological response due to an increase of the inflammatory response, similar to that observed in T lymphocytes when treated with anti-PD-1 and anti-TIM-3 antibodies.
The innate immune response to bacterial and viral infections is one of the most important mechanisms for controlling the pathogens that invade organisms every day. TIM-3 participates significantly in the response to these pathogens, since we know that it can regulate the activation of macrophages and the effectors function of these cells. Chronic bacterial infections like that of the intracellular pathogen Mycobacterium tuberculosis (M.tb) require a highly efficient Th1-type response; however, M.tb has developed diverse evasion strategies that allow it to survive the macrophage’s elimination systems, which are generally activated by IFN-γ produced by T lymphocytes with the Th1 profile. In an experimental model of in vitro infection with M.tb (H37Rv) performed in 2010, Jayaraman et al. demonstrated that when peritoneal macrophages were infected with M.tb (in the absence of T lymphocytes) and treated with TIM-3 in the form of a fusion protein, which is composed of the immunoglobulin V portion of mouse TIM-3 fused with the human IgG1 Fc tail to form the soluble TIM-3–Ig fusion protein, this protein limited bacterial growth through a mechanism that involved caspase-1-dependent IL-1b production (24). Blocking the activation of caspase-1, or treatment with IL-1b-blocking antibodies, eliminated the effect of the treatment with the TIM-3 fusion protein (24). This phenomenon has also been identified in human macrophages (23) infected with M.tb-H37Rv; moreover, studies have confirmed that IL-1b is the principal soluble factor that mediates inhibition of the growth of M.tb (47).
Another murine model, this one with infection by Toxoplasma gondii, an intracellular parasite that induces a Th1-type response, identified that mice of the C57BL/6 strain (susceptible) have a lower frequency of TIM-3+ immune cells in the spleen and mesenteric lymphatic nodes (NK, CD3+, CD11b, and CD11c+) compared to resistant BALB/c mice (48). The C57BL/6 mice developed an increased inflammatory condition mediated primarily by IFN-γ that induced extensive tissue damage in the small intestine with symptoms similar to Crohn’s disease (48). In contrast, the BALB/c mice that expressed a higher frequency of TIM-3+ cells did not develop this type of damage in the small intestine. These results suggest that TIM-3 functions as a molecule that favors tolerance by preventing an exacerbated inflammatory response in diverse tissues. One of the mechanisms by which TIM-3 can promote immunological tolerance in cells of the innate immune system consists in negatively regulating the activation induced by TLR signaling (35). This mechanism of negative regulation of activation in cells of the immune system that expresses TIM-3 is achieved by inhibiting the NF-Kb transcription factor, thus blocking the TLR-mediated response (49). Research has also documented a reduction in the release of pro-inflammatory cytokines, such as IFN-γ and IL-12, in macrophages with higher TIM-3 expression, compared to macrophages with a low expression of this molecule (33, 35). Finally, it has been demonstrated that the blocking or silencing of TIM-3 favors the development of sepsis, a severe inflammatory condition with a high mortality rate (49).
This evidence leads us to suggest that the TIM-3 molecule may play a dual role, since it participates in the activation of infected macrophages by inducing IL-1b-dependent bacteriostatic and bactericidal mechanisms. Also, when expressed in T lymphocytes, TIM-3 can negatively regulate the Th1 immune response. It is not yet possible to clarify the factors that produce these apparently contradictory findings and the possibility exists that the mechanisms induced by TIM-3 could occur simultaneously. The level of TIM-3 expression may be an important factor that affects the results and function of the TIM-3-dependent pathway. No simultaneous or comparative studies have yet been conducted to analyze the role of TIM-3 in macrophages and other cells of the innate immune system in the context of bacterial infections.
Since the discovery of the TIM-3 molecule, numerous studies performed have made it possible to determine some aspects of the functioning of this molecule. At present, TIM-3 is considered an inhibitory molecule of the activation of diverse cells, or an immune repressor system. On the other hand, there is evidence that TIM-3 can favor the activation of some mechanisms of the immune response, as reported for macrophages. Although these findings seem contradictory, one could propose that the level of TIM-3 expression is an important factor that must be considered, since studies have shown that a reduction in the level of expression of TIM-3 favors the production of IL-12 and the activation of monocytes and macrophages (33). Although research has shown that the union of Gal-9 with TIM-3 induces activation of caspase-1 and the posterior release of IL-1b, we do not yet know whether this mechanism is reproduced in the diverse sub-populations of macrophages, or even monocytes, since TIM-3 expression is dynamic and there are antecedents indicating that high expression of this molecule produces a state of tolerance or anergy to diverse antigenic stimuli. We require studies designed to evaluate the mechanisms of activation of phagocytic cells that take into account the level of expression and signaling of TIM-3. Finally, no one has yet demonstrated that TIM-3 is associated with the Bat-3 (14) protein in macrophages and DCs nor its interaction with the Lck protein, a very important kinase for the activation of transcription factors such as NF-kb. Analyzing and clarifying these issues will provide a broader perspective on the functions of TIM-3 and its role in myeloid cells. Such research is important because this field of study is lagging slightly behind analyses of the TIM-3 molecule in lymphoid cells.
AO-G: text and drawings. IS-O: design and text. LT-B and IS-O: text revision and final approval.
The authors declare that the research was conducted in the absence of any commercial or financial relationships that could be construed as a potential conflict of interest.
ARG-1, arginase 1; BAT-3, HLA-B 3-associated transcript; CD, cluster of differentiation; cDNA, complementary deoxyribonucleic acid; DC, dendritic cell; DNA, deoxyribonucleic acid; EAE, experimental autoimmune encephalitis; Gal-9, galectin 9; HMGB1, high mobility group box 1 protein; huHAVcr-1, human homolog of the receptor of the hepatitis A 1 virus; IFN-γ, gamma interferon; IgV, immunoglobulin domain; IL, interleukin; iNOS, inducible nitric oxide synthase; ITAM, tyrosine-based activation motif; LPS, lipopolysaccharide; M.tb, Mycobacterium tuberculosis; M-CSF, macrophage colony-stimulating factor; MMP-9, matrix metalloproteinase 9; NK, natural killer cell; PD-1, programed death receptor 1; RNA, ribonucleic acid; siRNA, interference ribonucleic acid; STAT, signal transducer and transcription activator; TAM, tumor-associated macrophage; TB, tuberculosis; Tc, cytotoxic T lymphocyte; TGF-β, transforming growth factor beta; Th, T lymphocyte cooperator or collaborator; TIM-3, T cell molecule with immunoglobulin and mucin domain 3; TLR, toll-like receptor; TNF-α, tumor necrosis factor alpha; VEGF, vascular endothelial growth factor; VIH, human immunodeficiency virus.
1. Anderson AC, Anderson DE, Bregoli L, Hastings WD, Kassam N, Lei C, et al. Promotion of tissue inflammation by the immune receptor Tim-3 expressed on innate immune cells. Science (2007) 318(5853):1141–3. doi:10.1126/science.1148536
2. Feigelstock D, Thompson P, Mattoo P, Zhang Y, Kaplan GG. The human homolog of HAVcr-1 codes for a hepatitis A virus cellular receptor. J Virol (1998) 72(8):6621–8.
3. Ichimura T, Bonventre JV, Bailly V, Wei H, Hession CA, Cate RL, et al. Kidney injury molecule-1 (KIM-1), a putative epithelial cell adhesion molecule containing a novel immunoglobulin domain, is up-regulated in renal cells after injury. J Biol Chem (1998) 273(7):4135–42. doi:10.1074/jbc.273.7.4135
4. Han WK, Bailly V, Abichandani R, Thadhani R, Bonventre JV. Kidney injury molecule-1 (KIM-1): a novel biomarker for human renal proximal tubule injury. Kidney Int (2002) 62(1):237–44. doi:10.1046/j.1523-1755.2002.00433.x
5. McIntire JJ, Umetsu SE, Akbari O, Potter M, Kuchroo VK, Barsh GS, et al. Identification of Tapr (an airway hyperreactivity regulatory locus) and the linked Tim gene family. Nat Immunol (2001) 2(12):1109–16. doi:10.1038/ni739
6. Gielen AW, Lobell A, Lidman O, Khademi M, Olsson T, Piehl F. Expression of T cell immunoglobulin- and mucin-domain-containing molecules-1 and -3 (TIM-1 and -3) in the rat nervous and immune systems. J Neuroimmunol (2005) 164(1–2):93–104. doi:10.1016/j.jneuroim.2005.04.004
7. Monney L, Sabatos CA, Gaglia JL, Ryu A, Waldner H, Chernova T, et al. Th1-specific cell surface protein Tim-3 regulates macrophage activation and severity of an autoimmune disease. Nature (2002) 415(6871):536–41. doi:10.1038/415536a
8. Kuchroo VK, Umetsu DT, DeKruyff RH, Freeman GJ. The TIM gene family: emerging roles in immunity and disease. Nat Rev Immunol (2003) 3(6):454–62. doi:10.1038/nri1111
9. Cao E, Zang X, Ramagopal UA, Mukhopadhaya A, Fedorov A, Fedorov E, et al. T cell immunoglobulin mucin-3 crystal structure reveals a galectin-9-independent ligand-binding surface. Immunity (2007) 26(March):311–21. doi:10.1016/j.immuni.2007.01.016
10. Zhu C, Anderson AC, Schubart A, Xiong H, Imitola J, Khoury SJ, et al. The Tim-3 ligand galectin-9 negatively regulates T helper type 1 immunity. Nat Immunol (2005) 6(12):1245–52. doi:10.1038/ni1271
11. Lee MJ, Heo YM, Hong S, Kim K, Park S. The binding properties of glycosylated and non-glycosylated Tim-3 molecules on CD4CD25 T cells. Immune Netw (2009) 9(2):58–63. doi:10.4110/in.2009.9.2.58
12. Kumanogoh A, Marukawa S, Suzuki K, Takegahara N, Watanabe C, Ch’ng E, et al. Class IV semaphorin Sema4A enhances T-cell activation and interacts with Tim-2. Nature (2002) 419(6907):629–33. doi:10.1038/nature01037
13. Lee J, Su EW, Zhu C, Hainline S, Phuah J, Moroco JA, et al. Phosphotyrosine-dependent coupling of tim-3 to T-cell receptor signaling pathways. Mol Cell Biol (2011) 31(19):3963–74. doi:10.1128/MCB.05297-11
14. Rangachari M, Zhu C, Sakuishi K, Xiao S, Karman J, Chen A, et al. Bat3 promotes T cell responses and autoimmunity by repressing Tim-3 – mediated cell death and exhaustion. Nat Med (2012) 18(9):1394–400. doi:10.1038/nm.2871
15. Sabatos CA, Chakravarti S, Cha E, Schubart A, Sánchez-Fueyo A, Zheng XX, et al. Interaction of Tim-3 and Tim-3 ligand regulates T helper type 1 responses and induction of peripheral tolerance. Nat Immunol (2003) 4(11):1102–10. doi:10.1038/ni988
16. Chiba S, Baghdadi M, Akiba H, Yoshiyama H, Kinoshita I, Dosaka-Akita H, et al. Tumor-infiltrating DCs suppress nucleic acid-mediated innate immune responses through interactions between the receptor TIM-3 and the alarmin HMGB1. Nat Immunol (2012) 13(9):832–42. doi:10.1038/ni.2376
17. Kane LP. T cell Ig and mucin domain proteins and immunity. J Immunol (2010) 184(6):2743–9. doi:10.4049/jimmunol.0902937
18. Yang L, Anderson DE, Kuchroo J, Hafler DA. Lack of TIM-3 immunoregulation in multiple sclerosis. J Immunol (2008) 180:4409–14. doi:10.4049/jimmunol.180.7.4409
19. Oomizu S, Arikawa T, Niki T, Kadowaki T, Ueno M, Nishi N, et al. Galectin-9 suppresses Th17 cell development in an IL-2-dependent but Tim-3-independent manner. Clin Immunol (2012) 143(1):51–8. doi:10.1016/j.clim.2012.01.004
20. Elola MT, Wolfenstein-Todel C, Troncoso MF, Vasta GR, Rabinovich GA. Galectins: matricellular glycan-binding proteins linking cell adhesion, migration, and survival. Cell Mol Life Sci (2007) 64(13):1679–700. doi:10.1007/s00018-007-7044-8
21. Wu W, Shi Y, Li S, Zhang Y, Liu Y, Wu Y, et al. Blockade of Tim-3 signaling restores the virus-specific CD8+ T-cell response in patients with chronic hepatitis B. Eur J Immunol (2012) 42(5):1180–91. doi:10.1002/eji.201141852
22. Khademi M, Illés Z, Gielen AW, Marta M, Takazawa N, Baecher-Allan C, et al. T Cell Ig- and mucin-domain-containing molecule-3 (TIM-3) and TIM-1 molecules are differentially expressed on human Th1 and Th2 cells and in cerebrospinal fluid-derived mononuclear cells in multiple sclerosis. J Immunol (2004) 172(11):7169–76. doi:10.4049/jimmunol.172.11.7169
23. Sada-Ovalle I, Chávez-Galán L, Torre-Bouscoulet L, Nava-Gamiño L, Barrera L, Jayaraman P, et al. The Tim3-galectin 9 pathway induces antibacterial activity in human macrophages infected with Mycobacterium tuberculosis. J Immunol (2012) 189:5896–902. doi:10.4049/jimmunol.1200990
24. Jayaraman P, Sada-Ovalle I, Beladi S, Anderson AC, Dardalhon V, Hotta C, et al. Tim3 binding to galectin-9 stimulates antimicrobial immunity. J Exp Med (2010) 207(11):2343–54. doi:10.1084/jem.20100687
25. de Cathelineau AM, Henson PM. The final step in programmed cell death: phagocytes carry apoptotic cells to the grave. Essays Biochem (2003) 39:105–17. doi:10.1042/bse0390105
26. van den Eijnde SM, Boshart L, Baehrecke EH, De Zeeuw CI, Reutelingsperger CP, Vermeij-Keers C. Cell surface exposure of phosphatidylserine during apoptosis is phylogenetically conserved. Apoptosis (1998) 3:9–16. doi:10.1023/A
27. Hoffmann PR, deCathelineau AM, Ogden CA, Leverrier Y, Bratton DL, Daleke DL, et al. Phosphatidylserine (PS) induces PS receptor-mediated macropinocytosis and promotes clearance of apoptotic cells. J Cell Biol (2001) 155(4):649–59. doi:10.1083/jcb.200108080
28. Kobayashi N, Karisola P, Peña-cruz V, Dorfman DM, Jinushi M, Umetsu SE, et al. T cell immunoglobulin mucin protein (TIM)-4 binds phosphatidylserine and mediates uptake of apoptotic cells Norimoto. Immunity (2009) 27(6):617–32. doi:10.1016/j.immuni.2007.11.011.T
29. Nakayama M, Akiba H, Takeda K, Kojima Y, Hashiguchi M, Azuma M, et al. Tim-3 mediates phagocytosis of apoptotic cells and cross-presentation. Blood (2009) 113(16):3821–30. doi:10.1182/blood-2008-10-185884
30. Asano K, Miwa M, Miwa K, Hanayama R, Nagase H, Nagata S, et al. Masking of phosphatidylserine inhibits apoptotic cell engulfment and induces autoantibody production in mice. J Exp Med (2004) 200(4):459–67. doi:10.1084/jem.20040342
31. DeKruyff RH, Bu X, Ballesteros A, Santiago C, Chim YL, Lee HH, et al. T cell/transmembrane, Ig, and mucin-3 allelic variants differentially recognize phosphatidylserine and mediate phagocytosis of apoptotic cells. J Immunol (2010) 184(4):1918–30. doi:10.4049/jimmunol.0903059
32. Wu W, Shi Y, Li J, Chen F, Chen Z, Zheng M. Tim-3 expression on peripheral T cell subsets correlates with disease progression in hepatitis B infection. Virol J (2011) 8(1):113. doi:10.1186/1743-422X-8-113
33. Zhang Y, Ma CJ, Wang JM, Ji XJ, Wu XY, Jia ZS, et al. Tim-3 negatively regulates IL-12 expression by monocytes in HCV infection. PLoS One (2011) 6(5):e19664. doi:10.1371/journal.pone.0019664
34. Zhang Y, Ma CJ, Wang JM, Ji XJ, Wu XY, Moorman JP, et al. Tim-3 regulates pro- and anti-inflammatory cytokine expression in human CD14+ monocytes. J Leukoc Biol (2012) 91(2):189–96. doi:10.1189/jlb.1010591
35. Ma CJ, Li GY, Cheng YQ, Wang JM, Ying RS, Shi L, et al. Cis association of galectin-9 with Tim-3 differentially regulates IL-12/IL-23 expressions in monocytes via TLR signaling. PLoS One (2013) 8(8):e72488. doi:10.1371/journal.pone.0072488
36. Shang Y, Li Z, Li H, Xia H, Lin Z. TIM-3 expression in human osteosarcoma: correlation with the expression of epithelial-mesenchymal transition-specific biomarkers. Oncol Lett (2013) 6(2):490–4. doi:10.3892/ol.2013.1410
37. Cao Y, Zhou X, Huang X, Li Q, Gao L, Jiang L, et al. Tim-3 expression in cervical cancer promotes tumor metastasis. PLoS One (2013) 8(1):e53834. doi:10.1371/journal.pone.0053834
38. Zhuang X, Zhang X, Xia X, Zhang C, Liang X, Gao L, et al. Ectopic expression of TIM-3 in lung cancers: a potential independent prognostic factor for patients with NSCLC. Am J Clin Pathol (2012) 137(6):978–85. doi:10.1309/AJCP9Q6OVLVSHTMY
39. Jiang J, Jin MS, Kong F, Cao D, Ma HX, Jia Z, et al. Decreased Galectin-9 and increased Tim-3 expression are related to poor prognosis in gastric cancer. PLoS One (2013) 8(12):e81799. doi:10.1371/journal.pone.0081799
40. Kashio Y, Nakamura K, Abedin MJ, Seki M, Nishi N, Yoshida N, et al. Galectin-9 induces apoptosis through the calcium-calpain-caspase-1 pathway. J Immunol (2003) 170(7):3631–6. doi:10.4049/jimmunol.170.7.3631
41. Yan W, Liu X, Ma H, Zhang H, Song X, Gao L, et al. Tim-3 fosters HCC development by enhancing TGF-β-mediated alternative activation of macrophages. Gut (2015) 64:1–12. doi:10.1136/gutjnl-2014-307671
42. Solinas G, Germano G, Mantovani A, Allavena P. Tumor-associated macrophages (TAM) as major players of the cancer-related inflammation. J Leukoc Biol (2009) 86(5):1065–73. doi:10.1189/jlb.0609385
43. Cassetta L, Cassol E, Poli G. Macrophage polarization in health and disease. Sci World J (2011) 11:2391–402. doi:10.1100/2011/213962
44. Martinez FO, Gordon S, Locati M, Mantovani A. Transcriptional profiling of the human monocyte-to-macrophage differentiation and polarization: new molecules and patterns of gene expression. J Immunol (2006) 177(10):7303–11. doi:10.1016/S1077-9108(08)70760-6
45. Ramello MC, Tosello Boari J, Canale FP, Mena HA, Negrotto S, Gastman B, et al. Tumor-induced senescent T cells promote the secretion of pro-inflammatory cytokines and angiogenic factors by human monocytes/macrophages through a mechanism that involves Tim-3 and CD40L. Cell Death Dis (2014) 5:e1507. doi:10.1038/cddis.2014.451
46. Sakuishi K, Apetoh L, Sullivan JM, Blazar BR, Kuchroo VK, Anderson AC. Targeting Tim-3 and PD-1 pathways to reverse T cell exhaustion and restore anti-tumor immunity. J Exp Med (2010) 207(10):2187–94. doi:10.1084/jem.20100643
47. Jayaraman P, Sada-Ovalle I, Nishimura T, Anderson AC, Kuchroo VK, Remold HG, et al. IL-1β promotes antimicrobial immunity in macrophages by regulating TNFR signaling and caspase-3 activation. J Immunol (2013) 190:4196–204. doi:10.4049/jimmunol.1202688
48. Berrocal Almanza LC, Muñoz M, Kühl AA, Kamradt T, Heimesaat MM, Liesenfeld O. Tim-3 is differently expressed in genetically susceptible C57BL/6 and resistant BALB/c mice during oral infection with Toxoplasma gondii. Eur J Microbiol Immunol (Bp) (2013) 3(3):211–21. doi:10.1556/EuJMI.3.2013.3.10
49. Yang X, Jiang X, Chen G, Xiao Y, Geng S, Kang C, et al. T cell Ig mucin-3 promotes homeostasis of sepsis by negatively regulating the TLR response. J Immunol (2013) 190(5):2068–79. doi:10.4049/jimmunol.1202661
50. Hu J, Jo M, Eastman BM, Gilder AS, Bui JD, Gonias SL. UPAR induces expression of transforming growth factor beta and interleukin-4 in cancer cells to promote tumor-permissive conditioning of macrophages. Am J Pathol (2014) 184(12):3384–93. doi:10.1016/j.ajpath.2014.08.003
Keywords: TIM-3, macrophages, immune regulation, innate immune response, tolerance mechanisms
Citation: Ocaña-Guzman R, Torre-Bouscoulet L and Sada-Ovalle I (2016) TIM-3 Regulates Distinct Functions in Macrophages. Front. Immunol. 7:229. doi: 10.3389/fimmu.2016.00229
Received: 17 February 2016; Accepted: 26 May 2016;
Published: 13 June 2016
Edited by:
Charles Dudley Mills, BioMedical Consultants, USAReviewed by:
William L. Redmond, Earle A. Chiles Research Institute, USACopyright: © 2016 Ocaña-Guzman, Torre-Bouscoulet and Sada-Ovalle. This is an open-access article distributed under the terms of the Creative Commons Attribution License (CC BY). The use, distribution or reproduction in other forums is permitted, provided the original author(s) or licensor are credited and that the original publication in this journal is cited, in accordance with accepted academic practice. No use, distribution or reproduction is permitted which does not comply with these terms.
*Correspondence: Isabel Sada-Ovalle, aXNhZGFteEBpbmVyLmdvYi5teA==
Disclaimer: All claims expressed in this article are solely those of the authors and do not necessarily represent those of their affiliated organizations, or those of the publisher, the editors and the reviewers. Any product that may be evaluated in this article or claim that may be made by its manufacturer is not guaranteed or endorsed by the publisher.
Research integrity at Frontiers
Learn more about the work of our research integrity team to safeguard the quality of each article we publish.