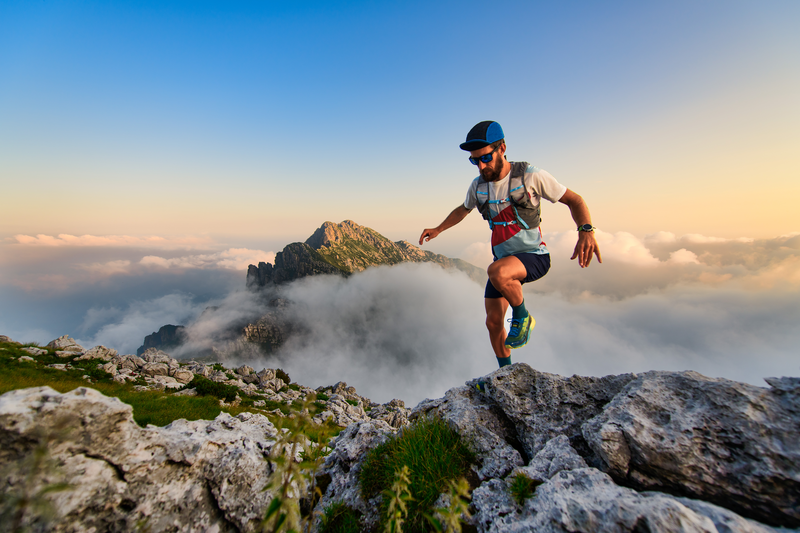
94% of researchers rate our articles as excellent or good
Learn more about the work of our research integrity team to safeguard the quality of each article we publish.
Find out more
REVIEW article
Front. Immunol. , 30 May 2014
Sec. Vaccines and Molecular Therapeutics
Volume 5 - 2014 | https://doi.org/10.3389/fimmu.2014.00254
This article is part of the Research Topic The neonatal Fc receptor (FcRn): From Biology to Therapy View all 13 articles
The biological activities of human IgG antibodies predominantly rely on a family of receptors for the Fc portion of IgG, FcγRs: FcγRI, FcγRIIA, FcγRIIB, FcγRIIC, FcγRIIIA, FcγRIIIB, FcRL5, FcRn, and TRIM21. All FcγRs bind IgG at the cell surface, except FcRn and TRIM21 that bind IgG once internalized. The affinity of FcγRs for IgG is determined by polymorphisms of human FcγRs and ranges from 2 × 104 to 8 × 107 M−1. The biological functions of FcγRs extend from cellular activation or inhibition, IgG-internalization/endocytosis/phagocytosis to IgG transport and recycling. This review focuses on human FcγRs and intends to present an overview of the current understanding of how these receptors may contribute to various pathologies. It will define FcγRs and their polymorphic variants, their affinity for human IgG subclasses, and review the associations found between FcγR polymorphisms and human pathologies. It will also describe the human FcγR-transgenic mice that have been used to study the role of these receptors in autoimmune, inflammatory, and allergic disease models.
Human myeloid cells, NK cells, and B cells are equipped with a variety of receptors that enable their interaction with monomeric or aggregated immunoglobulins, antigen–antibody immune complexes, and opsonized (antibody-coated) particles, cells, or surfaces. Most of these receptors bind the Fc portion of immunoglobulins (receptors for the Fc portion of immunoglobulins, FcR) and endow these cells with the capacity to interact with IgM, IgA, IgG, and/or IgE. This review will focus on IgG-binding human FcRs, FcγRs.
Humans express nine FcγRs: the six classical FcγRs, FcγRI, FcγRIIA, FcγRIIB, FcγRIIC, FcγRIIIA, and FcγRIIIB; as well as FcRn, FcRL5 (1, 2), and TRIM21 (3) (Figure 1). These FcγRs all bind IgG on the surface of the cells expressing them, except FcRn (4, 5) and TRIM21 (6, 7) that bind IgG once internalized. Notably, all IgG receptors bind at least two human IgG subclasses, albeit with varying binding affinity: the association constants (KA) of IgG–FcγR interactions range from 8 × 107 down to 2 × 104 M−1 (8) (Figure 1). Historically, FcγRs were categorized as either low-affinity receptors that can only bind IgG when present in an immune complex, aggregated, or opsonized; or high-affinity receptors that can also bind free or monomeric IgG. This terminology has become rather obsolete considering reports of high- and low-affinity interactions for a single receptor toward different Ig subclasses. Furthermore, although the prevailing belief was that occupancy of high-affinity receptors with pre-bound monomeric IgG prevents their participation in immediate IgG-dependent reactions; this has recently been refuted in vivo (9). Adding to this complexity, human FcγR polymorphisms that modulate affinity for some human IgG subclasses have been described (8) (refer to part 2; Figure 1).
Figure 1. Human IgG receptor family. Alleles are identified by the amino acid variant in the protein (e.g., H131), or by the name of the allelic variants (NA1, NA2, or SH). Binding affinities for the various immunoglobulin subclasses are given as M−1. High-affinity interactions are indicated in bold. –, no binding; ND, not determined; ♮No allelic variants have yet been described that affect binding affinity. #Associates with integrins. ITAM, immunoreceptor tyrosine-based activation motif; γ2, dimer of FcRγ subunits; ITIM, immunoreceptor tyrosine-based inhibitory motif; GPI, glycosyl-phosphatidylinositol; β2m, β2-microglobulin.
Human FcγR expression on different cell types has been fairly comprehensively described, mostly by the use of FcγR-specific monoclonal antibodies (mAb) but also from data using mRNA profiling (Figure 2). Generally, the following observations can be made: hFcγRI (CD64) is restricted to monocytes/macrophages and dendritic cells and is inducibly expressed on neutrophils (10) and mast cells (11); hFcγRIIA (CD32A) is expressed on all myeloid cells but not on lymphocytes; hFcγRIIB (CD32B) is expressed at high levels only on B cells (12) and basophils (13). It is also expressed on tissue macrophages and dendritic cells (12), but only at low levels on 20% of circulating monocytes and 4% of circulating neutrophils (12, 14), and is not expressed on primary skin mast cells (15); hFcγRIIC (CD32C; refer to Section “Human FcγR Polymorphisms” for its “stop13” polymorphism) is expressed on NK cells (16), monocytes, and neutrophils (17); hFcγRIIIA (CD16A) is expressed on NK cells and monocytes/macrophages; hFcγRIIIB (CD16B) is highly expressed on neutrophils and at low levels on some basophils (18). TRIM21 (aka Ro52) was described to be widely expressed among lymphoid and myeloid populations, but also on endothelial cells (19). FcRL5 has been reported to be restricted to B cells (2).
Figure 2. Human IgG receptor expression pattern. + indicates expression; (+), inducible expression; ±, very low percentages or rare subsets express the receptor; −, no expression; and NA, not analyzed; Mono/Macro, monocytes, and/or macrophages. §Refer to the review by Guilliams et al. for specific expression on human DC subtypes (20). ♮In Fcgr2c-ORF persons (17). #Detectable and functional expression in non-conventional Fcgr2c-Stop persons (17).
These expression patterns highlight that hFcγRIIA is the only activating IgG receptor constitutively expressed by mast cells, basophils, neutrophils, and eosinophils, and that FCRL5 is the only activating IgG receptor constitutively expressed by B cells. Importantly, signal transduction events induced by human activating IgG receptors may be negatively regulated by hFcγRIIB only in B cells, dendritic cells, and basophils, and rare fractions of monocytes and neutrophils. Indeed, mast cells, NK cells, and most neutrophils and monocytes do not express this inhibitory receptor. hFcRn has been reported in dendritic cells, monocytes/macrophages (21), neutrophils (22), and endothelial cells (23), but expression on platelets and mast cells has not been examined so far.
These patterns correspond to the expression of FcγRs in healthy individuals. These may be modified during pathological conditions or following therapeutic treatments. Certain cytokines for example have been reported to up-regulate or down-regulate some hFcγRs; e.g., B cells express higher levels of hFcγRIIB following IFN-γ but lower levels following IL-4 stimulation, whereas opposite effects have been reported for monocytes [reviewed in Ref. (24)]. On the latter cells, expression of hFcγRIIA is increased following IFN-γ and decreased following IL-4 stimulation (25). IL-3 stimulation, however, induces higher expression of both receptors (activating hFcγRIIA and inhibitory hFcγRIIB) on basophils (13). Mucosal mast cells express hFcγRI upon IFN-γ stimulation (11). Surprisingly, IL-3 stimulation of primary monocytes did not modify hFcγRI expression, but increased its ability to bind IgG-immune complexes and to induce intracellular activation signals (26).
Activating FcγRs signal through an immunoreceptor tyrosine-based activation motif (ITAM) that is either present in their intracytoplasmic domain or in associated signaling subunits, such as the FcRγ chain (Figure 1), the FcRβ chain (exclusively in mast cells and basophils), or the CD3ζ chain (exclusively in NK cells). These ITAM-containing structures allow FcγRs, once aggregated by multimeric ligands, to activate signaling cascades via SRC family kinases and spleen tyrosine kinase (SYK) leading to cell activation, cytokine/chemokine production, and cell migration (27–29). The inhibitory receptor FcγRIIB possesses instead an immunoreceptor tyrosine-based inhibition motif (ITIM) in its intracytoplasmic domain (30), which allows this receptor, once co-engaged with an activating FcγR, to recruit the inositol polyphosphate-5-phosphatase SHIP1 (31) that counteracts the signaling cascades initiated by activating FcγRs (24). FcRL5 possesses both an ITAM and two ITIMs; however, it has been reported to exert mainly negative regulatory functions (32). IgG receptors devoid of both ITAM and ITIM may induce cell activation by associating with other receptors at the cell membrane, for example the glycophosphatidylinositol-anchored FcγRIIIB (33, 34) associates with integrins (35); or by activating transcription pathways or proteasome-related mechanisms as does TRIM21 (7, 36).
Internalization of antibodies, and of the antigens they are bound to, represents the only shared function of IgG receptors expressed at the cell surface (that is, all except FcRn and TRIM21), whether ITAM-bearing, ITIM-bearing, or neither. FcγRs thereby enable antigen capture and internalization by all FcγR-expressing nucleated cells, as well as phagocytosis of opsonized bacteria, viruses, or cells by phagocytes. FcRn is the only receptor enabling transcytosis of IgG or IgG-IC by polarized cells (23). Enhanced uptake of antibody-bound antigen enables antigen-presenting cells to activate antigen-specific T cells considerably more efficiently than free antigen (37), signifying the pivotal role of FcγRs in the initial phase of humoral and cellular immune responses. Receptors that bind IgG only when it has already been internalized, FcRn (the topic of this review series) and the ubiquitously expressed intracellular receptor TRIM21, may possibly contribute to this phenomenon [reviewed in Ref. (20)].
The multiplicity of human FcγRs (Figure 1) is increased by a series of genetic polymorphisms, for which we will describe herein only those leading to known functional modifications. These are summarized in Table 1.
A polymorphism resulting in the presence of a histidine or an arginine residue at position 131 may also be referred to as low-responder (H131) or high-responder (R131) (38). The FcγRIIA-H131 allotype was originally reported to allow binding to IgG2 (53), subject to ethnic variation (54, 55), and was later described to also have increased binding for IgG3 (39). More recently, we have identified that only the binding to IgG1 and IgG2 are increased for H131 compared to R131 (8).
A novel splice variant of FCGR2A, FcγRIIA-exon 6*, containing an expressed cryptic exon 6* was identified in 2013 (41), and is associated with increased neutrophil sensitivity to IgG stimulation (56).
Single-nucleotide polymorphisms (SNPs) at positions 386 [IIB-386 (G/c)] and 120 [IIB-120 (T/a)], collectively constitute the 2B.4 promoter haplotype, which displays increased binding capacity for transcription factors GATA4 and Yin-Yang1, resulting in increased promoter activity and higher expression of FcγRIIB on monocytes, B lymphocytes, neutrophils, and myeloid DCs (24, 42).
A polymorphism encoding an isoleucine to threonine substitution at position 232 in the transmembrane domain of FcγRIIB (T232) may disable receptor function via exclusion from lipid rafts (43, 57).
In 20% of individuals FCGR2C encodes for a glutamine at position 13 (Q13 or ORF) and FcγRIIC is expressed; but in 80% of individuals a SNP generates a stop codon (stop13), in which case FCGR2C represents a pseudogene (16).
A subset of individuals carrying FCGR2C-ORF do not express FcγRIIC due to splice-site mutations and loss of exon 7. Inversely, this polymorphism leads to the expression of inhibitory hFcγRIIB expression on NK cells that has been shown to negatively regulate IgG-induced NK cell activation (17).
A SNP determines the presence of a valine or phenylalanine at position 158 (45). The FcγRIIIA-V158 variant demonstrates increased affinity for IgG1, IgG2, and IgG3, and increased IgG-induced cell activation and elimination of immune complexes (8, 46, 58).
FcγRIIIB bears the neutrophil antigen (NA) in its membrane-distal Ig-like domain, generating three variants termed NA1 (R36 N65 A78 D82 V106), NA2 (S36 S65 A78 N82 I106) (48, 59), and SH (S36 S65 D78 N82 I106) (50) that do not demonstrate detectable differences in affinity for hIgG subclasses (8). The NA1 allotype was, however, reported to increase phagocytosis of IgG-opsonized particles (49). The SH allotype has been associated with higher FcγRIIIB expression levels (51).
Recognized as an important indicator for inter-individual differences, can alter the expression of activating IgG receptors. The balance between activating and inhibitory FcγRs can therefore be perturbed, altering cellular responses toward IgG-immune complexes. CNV of FCGR2C, FCGR3A, and FCGR3B (Table 1) have been shown to correlate with protein expression levels. Duplications of the gene encoding FCGR3B can lead to the expression of the three different FcγRIIIB variants (NA1, NA2, and SH) in a single individual (51). CNV in FCGR3A (deletion of one allele) correlated with a reduced expression of FcγRIIIA on NK cells and impaired cytotoxic function (47). Deletion of a large portion of the FCGR locus, including FCGR2C and FCGR3B, also resulted in abnormal expression of FcγRIIB on NK cells, presumably due to deletion of upstream regulatory elements. Expression of this inhibitory receptor enabled negative regulation of IgG-induced NK cell activation (17). To the extent of our knowledge, CNV of the FCGR2A and FCGR2B genes have not been reported (47).
Several FCGR polymorphisms modify the affinity between FcγRs and human IgG, and therefore the efficacy of immune complex clearance can be affected. Reduced immune complex clearance is indeed a risk factor for diseases like Systemic Lupus Erythematosus and Wegener’s granulomatosis (60, 61). Other polymorphisms may favor detrimental inflammatory responses and thus predispose to autoimmunity. Diseases that have been associated with FcγR polymorphisms are presented in Table 1.
FcγR polymorphisms may also influence patients’ response to treatment with intravenous immunoglobulin and therapeutic mAb. Almost all mAb used in therapy are based on human IgG1 antibodies, either chimeric mouse/human or fully human, allowing their interaction with all human FcγRs (8, 62). The first report to assess the predictive value of FcγR polymorphisms in responses to antibody therapies associated homozygous FCGR3A-V/V158 individuals with better clinical responses to anti-CD20 therapy (Rituximab) in the treatment of non-Hodgkin lymphomas (63). Homozygous FCGR3A-V/V158 individuals have since been found to have improved biological responses to anti-CD20 therapy in immune thrombocytopenia (64) and rheumatoid arthritis (RA) (65); and anti-TNF-α therapy (Infliximab) to treat Crohn’s disease (66, 67); compared to carriers of one or two FCGR3A-F158 alleles. In arthritis patients, however, findings are controversial regarding the association of FCGR3A polymorphisms with clinical response to TNF-α inhibitors (infliximab, adalimumab, etanercept): although one study describes a better clinical response in FCGR3A-F/F158 patients (68); another, larger study with a more homogenous patient cohort found no association (69). Homozygous FCGR3A-V/V158 individuals were more likely to experience complete remission from immune thrombocytopenia following medication, but conversely remission rates after splenectomy were higher in homozygous FCGR3A-F/F158 or heterozygous individuals (70). The FCGR2A-H131 variant associates with susceptibility to Kawasaki Disease (Table 1), whereas responsiveness to IVIG therapy in Kawasaki Disease patients is strongly associated with the FCGR3B genotype: the NA1 variant significantly decreases the odds of an appropriate clinical outcome (71). Similarly, CNV of both FCGR3B and FCGR2C were associated with Kawasaki Disease susceptibility and influenced IVIG treatment response (72). Furthermore, the FCGR2B minor alleles (IIB-386c and IIB-120a) conferring increased promoter activity were positively correlated to IVIG therapeutic response, although with limited statistical power over a small sample size (73). Each of these genetic associations is also constrained by unequal polymorphic variation between the different ethnic groups studied.
Altogether, particular FcγR polymorphisms have been described to be associated with the induction or severity of antibody-related disease, or patient responsiveness to antibody-based therapies. Nonetheless one should keep in mind that most FcγR-encoding genes are located within the 1q23 locus (FCGR2A, FCGR3A, FCGR2B, FCGR2C, FCGR3B) and may display a high degree of linkage disequilibrium, as reported for FCGR2A and FCGR3A (74) and for FCGR2C and FCGR3B (44). Association studies of FcγR-encoding genes should therefore include analyses of all FcγR-encoding genes from the 1q23 locus, and not focus on one particular gene.
Transgenic mouse studies have greatly enhanced our understanding of the in vivo function of hFcγRs. In particular, these studies have highlighted the respective contributions of hFcγRs to antibody-mediated inflammatory and allergic diseases (refer to Section “Understanding the Role of hFcγRs In vivo Using Transgenic Mouse Models: Illustrated in Autoimmune, Inflammatory, and Allergic Diseases”). Over the last two decades, various transgenic mouse strains have been generated that carry single or multiple hFcγR-encoding genes (Table 2). Transgenic strains were initially generated on a wild-type mouse background; however, later studies have examined transgene expression in mice deficient for multiple endogenous mFcγRs, to specifically study the function of the transgenic human receptor.
Table 2. Association of FcγRs receptor variants with chronic inflammatory or immunological diseases.
The common approach to reproduce hFcγR expression patterns in mice is to use the genuine human promoter to drive transgene expression (Table 2). Whereas this strategy was successful for hFcγRIIAtg and hFcγRIIIBtg mice, both hFcγRItg mice and hFcγRIIBtg mice exhibit somewhat abnormal expression [discussed in Ref. (62)]. hFcγRItg mice, for example, constitutively express substantial amounts of this receptor on neutrophils (37), while in humans hFcγRI is only inducibly expressed on neutrophils in contexts of inflammation, infection and during particular therapies [reviewed in Ref. (62)]. An alternative strategy consists of using a cell-specific promoter to drive hFcγR expression. hFcγRIIAtg, hFcγRIIIBtg, or double-transgenic mice were generated using the human MRP8 promoter to express these receptors on neutrophils and, abnormally for hFcγRIIIB, on a proportion of monocytes (34). Finally, efforts made to cross the five single hFcγR-transgenic mouse strains with mFcγRnull mice – lacking mFcγRI, IIB, III, and IV – yielded a mouse model expressing most human IgG receptors – hFcγRI, IIA, IIB, IIIA, and IIIB – that preserves most human expression patterns (119) (Table 2).
FcR-mediated uptake of immune complexes and subsequent antigen presentation is a critical aspect of the immune response to foreign pathogens. Targeting of antigen to hFcγRI in hFcγRItg mice induced a strong antibody response, suggesting that hFcγRI on myeloid cells is capable of mediating antigen uptake and presentation in vivo (37, 120, 121). Various studies have demonstrated the capacity for hFcγRI and hFcγRIIIA to mediate cytotoxicity in the form of anti-tumor activity when engaged by bi-specific antibodies or antibodies with enhanced FcR binding, highlighting the effectiveness of such engineered antibody therapeutics in vivo (122–125). The role of FcγR in mediating anti-tumor therapies has recently been well-reviewed elsewhere (126, 127) and will not be discussed further in this review. hFcγR-transgenic mice have been useful both in understanding the in vivo function of these receptors and dissecting pathological mechanisms of disease; for illustration this section will describe results obtained in models of autoimmune thrombocytopenia, anaphylaxis, inflammation, and RA. Clearly, the biological responses to immobilized IgG are a function of their location, structure, and deposition, determining the subsequent recruitment and FcγR-mediated activation of immune cells: hFcγR-transgenic mice can assist us also in understanding the cell-specific role of FcγR in recruitment and immune complex clearance.
Mice deficient for the FcRγ-subunit that is necessary for the expression of all mouse activating FcγRs are resistant to antibody-mediated platelet destruction, demonstrating the importance of activating FcγRs in this model of autoimmune thrombocytopenia (128). Using transgenic mice, both hFcγRI and hFcγRIIA were found to be independently sufficient for platelet clearance (9, 129). In hFcγRItg mice, thrombocytopenia was mediated by monocyte/macrophages outside of the spleen (9), whereas in hFcγRIIAtg mice, splenectomy was found to provoke a more severe phenotype of thrombosis and systemic shock when thrombocytopenia was induced by activating anti-platelet antibodies (130). Importantly, hFcγRIIA is the only FcγR expressed on platelets, in humans and hFcγRIIAtg mice. It is likely, therefore, that the presence of this FcγR on the platelets themselves contributes to antibody-induced intravascular platelet activation that is most efficiently resolved by phagocytes in the spleen. These findings have implications for understanding human immune-mediated thrombocytopenic disorders, such as heparin-induced thrombocytopenia/thrombosis (HIT/T), a serious complication arising from the clinical use of heparin. Using hFcγRIIAtg mice it was identified that antibodies against heparin–platelet factor 4 complexes are responsible for hFcγRIIA-mediated platelet activation, thrombocytopenia, and thrombi formation in the lung vasculature (131, 132). Similarly, thromboembolic complications from the use of monoclonal antibody therapies may be a result of hFcγRIIA-dependent platelet activation due to circulating immune complexes (133, 134). Another important outcome of these mouse studies is that the density of hFcγRIIA expression in the transgenic animal affects the severity of antibody-induced disease (130), which has critical ramifications for understanding differences in immune reactions between individuals. Finally, a therapeutic intervention targeting the hFcγRIIA-signaling pathway proved successful for the prevention of thrombocytopenia in hFcγRIIAtg mice (135).
Individuals who have developed antibodies against a given allergen can, upon re-exposure, develop a severe systemic allergic reaction (anaphylaxis). Allergen re-exposure induces the rapid formation of immune complexes that leads to cellular activation and release of vasoactive mediators, which drives the phenotype of systemic shock, including symptoms of hypotension and respiratory distress. Although anaphylaxis is classically attributed to an IgE-mediated mast cell-dependent paradigm of allergic reactivity, the same systemic symptoms can be reproduced experimentally in mice by the transfer of specific IgG antibodies and allergen, of preformed immune complexes (passive systemic anaphylaxis, PSA), or by repeated immunization with an antigen prior to challenge (active systemic anaphylaxis, ASA). hFcγRI and hFcγRIIA expressed in transgenic mice were each individually sufficient to mediate PSA, the symptoms of which may be alleviated by pre-treatment with blocking antibodies (9, 136). PSA mediated by hFcγRIIA was found to be independent of mast cells and basophils, but rather dependent on neutrophils and monocytes/macrophages (136). Furthermore, hFcγRI and hFcγRIIA were identified as each individually sufficient to mediate ASA in transgenic mice, resulting in both hypothermia and death (9, 136). hFcγRI-dependent ASA required neutrophils and the release of platelet activating factor (9). These data demonstrate that hFcγR expressed on neutrophils and monocytes can mediate fatal anaphylactic reactions in vivo. Furthermore, in hFcγRItgIIAtgIIBtgIIIAtgIIIBtg mice (on the mFcγRnull background), administration of aggregated IgG was sufficient to trigger anaphylaxis (119). In addition, directly targeting either hFcγRI or hFcγRIIA by injection of agonistic mAb could induce anaphylaxis in transgenic mice (9, 136). Altogether, these data support the notion that anaphylaxis may also occur in humans in an hFcγR-dependent manner when allergen-specific IgGs are produced by an individual.
The formation of immune complexes is a hallmark of many human diseases, and their accumulation is an important trigger of inflammation-induced tissue damage. Pathogenic antibodies may bind directly to host cells, or immune complexes may deposit within tissues and trigger activation of local or circulating hFcγR-expressing cells. Using hFcγRIIAtg mice, it was demonstrated that hFcγRIIA expressed on skin mast cells could trigger their activation following intradermal injection of immune complexes resulting in an inflammatory reaction in the skin (136). Inflammation of the airways due to local formation of immune complexes is characterized by granulocyte infiltration, elevated levels of myeloperoxidase, and subsequent damage to the lung epithelium, mimicking symptoms of asthmatic disease in humans. Whereas FcRγ-subunit−/− mice are resistant to IC-induced airway inflammation, transgenic expression of either hFcγRI or hFcγRIIA was sufficient to restore this antibody-mediated pathology (9, 136).
Rheumatoid arthritis is an autoimmune disease in which the formation of immune complexes within the joints drives an inflammatory pathology. Autoantibodies directed against joint proteins such as collagen type II or glucose-6-phosphate isomerase (GPI) are found in RA patients, and the arthritis pathology may be modeled in mice by either active immunization with joint-associated components or by passive antibody transfer. hFcRntg mice provided direct evidence for the role of this receptor in serum persistence and transport of antibodies into tissues (23). Indeed, mFcRn−/− mice are resistant to passive arthritis induction, and transgenic expression of hFcRn could restore arthritis susceptibility (137, 138); suggesting that greater IgG serum persistence may have implications for many autoimmune and inflammatory conditions (139). Surprisingly, transgenic expression of hFcγRIIA-R131 on a wild-type mouse background was associated with the spontaneous development of an RA-like joint pathology (140). Expression of hFcγRIIA indeed renders mice highly susceptible to various models of arthritis (140, 141), even if its expression is purposely restricted to neutrophils (142). Small inhibitors designed to bind antagonistically to hFcγRIIA were found to be protective (143), proposing a hFcγR-targeted therapy for RA. Besides hFcγRIIAtg mice, other hFcγR-transgenic mice do not exhibit spontaneous joint inflammation. Nevertheless, hFcγRItg mice demonstrated that this receptor is sufficient to mediate arthritis induction in transgenic mice, dependent on the presence of both neutrophils and monocytes/macrophages (9). Therapeutic elimination of inflammatory macrophages by an hFcγRI-targeting immunotoxin inhibited the progression of experimental arthritis in hFcγRItg rats (144), and resolved cutaneous inflammation (145).
Studies using hFcγRtg mice have enabled the description of specific in vivo functions not only for these IgG receptors, but also the cells that express them. Neutrophils are a particularly relevant example: the two main human neutrophil IgG receptors, hFcγRIIA and hFcγRIIIB, were found to individually and cooperatively promote IC-induced neutrophil recruitment and accumulation in the tissues. hFcγRIIA alone, however, promoted associated injury and inflammation in multiple models of antibody-dependent autoimmunity. Importantly, neutrophil recruitment occurred despite the absence of FcγR expression on other cell types such as mast cells and macrophages, indicating a prominent role for hFcγRs on neutrophils in IC-induced recruitment (34). Furthermore, specialized functions may be attributed to these two neutrophil FcγR: hFcγRIIIB seems to play an important role in homeostatic clearance of immune complexes deposited within the vasculature, whereas in a complex environment of immune complex deposition within the tissue and the vasculature, hFcγRIIA was required for the formation of neutrophil extracellular traps (NETs) (146). Collectively, these data in hFcγRtg mice demonstrate the value of a transgenic approach to appreciate the role of human FcγR and the cells expressing them.
Although, it is tempting to draw conclusions from genetic association studies performed in humans, it would be overreaching to delineate causal relationships between particular FcγR variants and antibody-mediated human disease. Importantly, all the human FcγR-transgenic mouse strains that have been reported express a single polymorphic variant of each FcγR (Table 3). Thus, no comprehensive study can compare today the properties of a given polymorphism in mouse models of disease. Novel mouse models based on the exchange of the entire FCGR locus with that of humans may allow these comparison studies, or transgenic/knock-in mice expressing different polymorphic variants than the transgenic mice already reported, but remain to be generated. Still, when taking into account published data from both humans and animal models (referenced in Tables 2 and 3) several parallel observations have been described:
- Expression of hFcγRIIA (R131) renders mice susceptible to arthritis and autoimmune pathologies including thrombocytopenia (Table 3); and expression of hFcγRIIA-R131 allotype is similarly associated with inflammatory diseases, thrombocytopenia, and autoimmunity in humans (Table 2). The FcγRIIa-exon 6* polymorphic variant, which confers increased neutrophil sensitivity to IgG stimulation (Table 1) was also associated with anaphylactic responses in patients upon IVIG therapy (Table 2); consistent with data obtained in hFcγRIIAtg mice indicating that neutrophils can contribute to IgG-dependant anaphylaxis mediated by FcγRIIA.
- The NA1 allotypic variant of FcγRIIIB confers increased phagocytosis of IgG-immune complexes, and is associated with thrombocytopenia in humans; whereas FcγRIIIB-NA2 and CNV are associated with inflammatory and autoimmune conditions characterized by immune complex deposition. These data are congruent with findings in NA2-hFcγRIIIBtg mice (Table 2), demonstrating an important role for this receptor in mediating neutrophil recruitment as well as homeostatic clearance of immune complexes.
While genetic association studies identify important risk factors and inform on the involvement of FcγR in human disease; hFcγRtg mice allow us to more precisely dissect pathological mechanisms, and describe the role of human FcγR and the cells expressing them in various clinically relevant pathologies. Together, these data in humans and transgenic models highlight the contribution of hFcγR to antibody-mediated diseases, and open avenues for understanding pathogenic mechanisms. Such data will continue to impact on therapeutic choices and potentially identify new interventional targets.
The authors declare that the research was conducted in the absence of any commercial or financial relationships that could be construed as a potential conflict of interest.
Works of our laboratory discussed in this review were supported by the Institut Pasteur, the Institut National de la Santé et de la Recherche Médicale (INSERM), the Agence Nationale pour la Recherche (grant GENOPAT-09-GENO-014-01), the Société Française d’Allergologie (SFA), and the company Balsan. Caitlin Gillis is a scholar of the Pasteur Paris University International Doctoral Program (PPUIDP) and received a stipend from the Institut Carnot Pasteur Maladies Infectieuses. Friederike Jönsson is a chargé de recherche (Investigator) at the Centre National de la Recherche Scientifique (CNRS).
1. Wilson TJ, Fuchs A, Colonna M. Cutting edge: human FcRL4 and FcRL5 are receptors for IgA and IgG. J Immunol (2012) 188:4741–5. doi: 10.4049/jimmunol.1102651
2. Franco A, Damdinsuren B, Ise T, Dement-Brown J, Li H, Nagata S, et al. Human Fc receptor-like 5 binds intact IgG via mechanisms distinct from those of Fc receptors. J Immunol (2013) 190:5739–46. doi:10.4049/jimmunol.1202860
3. Yang Y, Eversole T, Lee DJ, Sontheimer RD, Capra JD. Protein-protein interactions between native Ro52 and immunoglobulin G heavy chain. Scand J Immunol (1999) 49:620–8. doi:10.1046/j.1365-3083.1999.00547.x
4. Simister NE, Mostov KE. An Fc receptor structurally related to MHC class I antigens. Nature (1989) 337:184–7. doi:10.1038/337184a0
5. Ober RJ, Martinez C, Vaccaro C, Zhou J, Ward ES. Visualizing the site and dynamics of IgG salvage by the MHC class I-related receptor, FcRn. J Immunol (2004) 172:2021–9. doi:10.4049/jimmunol.172.4.2021
6. James LC, Keeble AH, Khan Z, Rhodes DA, Trowsdale J. Structural basis for PRYSPRY-mediated tripartite motif (TRIM) protein function. Proc Natl Acad Sci U S A (2007) 104:6200–5. doi:10.1073/pnas.0609174104
7. Mallery DL, McEwan WA, Bidgood SR, Towers GJ, Johnson CM, James LC. Antibodies mediate intracellular immunity through tripartite motif-containing 21 (TRIM21). Proc Natl Acad Sci U S A (2010) 107:19985–90. doi:10.1073/pnas.1014074107
8. Bruhns P, Iannascoli B, England P, Mancardi DA, Fernandez N, Jorieux S, et al. Specificity and affinity of human Fc{gamma} receptors and their polymorphic variants for human IgG subclasses. Blood (2009) 113:3716–25. doi:10.1182/blood-2008-09-179754
9. Mancardi DA, Albanesi M, Jonsson F, Iannascoli B, van Rooijen N, Kang X, et al. The high-affinity human IgG receptor FcgammaRI (CD64) promotes IgG-mediated inflammation, anaphylaxis, and antitumor immunotherapy. Blood (2013) 121:1563–73. doi:10.1182/blood-2012-07-442541
10. Ravetch JV, Kinet JP. Fc receptors. Annu Rev Immunol (1991) 9:457–92. doi:10.1146/annurev.iy.09.040191.002325
11. Okayama Y, Kirshenbaum AS, Metcalfe DD. Expression of a functional high-affinity IgG receptor, Fc gamma RI, on human mast cells: up-regulation by IFN-gamma. J Immunol (2000) 164:4332–9. doi:10.4049/jimmunol.164.8.4332
12. Veri MC, Gorlatov S, Li H, Burke S, Johnson S, Stavenhagen J, et al. Monoclonal antibodies capable of discriminating the human inhibitory Fcgamma-receptor IIB (CD32B) from the activating Fcgamma-receptor IIA (CD32A): biochemical, biological and functional characterization. Immunology (2007) 121:392–404. doi:10.1111/j.1365-2567.2007.02588.x
13. Cassard L, Jonsson F, Arnaud S, Daeron M. Fcgamma receptors inhibit mouse and human basophil activation. J Immunol (2012) 189:2995–3006. doi:10.4049/jimmunol.1200968
14. Magnusson SE, Engstrom M, Jacob U, Ulfgren AK, Kleinau S. High synovial expression of the inhibitory FcgammaRIIb in rheumatoid arthritis. Arthritis Res Ther (2007) 9:R51. doi:10.1186/ar2206
15. Zhao W, Kepley CL, Morel PA, Okumoto LM, Fukuoka Y, Schwartz LB. Fc gamma RIIa, not Fc gamma RIIb, is constitutively and functionally expressed on skin-derived human mast cells. J Immunol (2006) 177:694–701. doi:10.4049/jimmunol.177.1.694
16. Metes D, Ernst LK, Chambers WH, Sulica A, Herberman RB, Morel PA. Expression of functional CD32 molecules on human NK cells is determined by an allelic polymorphism of the FcgammaRIIC gene. Blood (1998) 91:2369–80.
17. van der Heijden J, Breunis WB, Geissler J, De Boer M, van den Berg TK, Kuijpers TW. Phenotypic variation in IgG receptors by nonclassical FCGR2C alleles. J Immunol (2012) 188:1318–24. doi:10.4049/jimmunol.1003945
18. Meknache N, Jönsson F, Laurent J, Guinnepain MT, Daëron M. Human basophils express the glycosylphosphatidylinositol-anchored low-affinity IgG receptor Fc{gamma}RIIIB (CD16B). J Immunol (2009) 182:2542–50. doi:10.4049/jimmunol.0801665
19. Espinosa A, Dardalhon V, Brauner S, Ambrosi A, Higgs R, Quintana FJ, et al. Loss of the lupus autoantigen Ro52/Trim21 induces tissue inflammation and systemic autoimmunity by disregulating the IL-23-Th17 pathway. J Exp Med (2009) 206:1661–71. doi:10.1084/jem.20090585
20. Guilliams M, Bruhns P, Saeys Y, Hammad H, Lambrecht BN. The function of Fcgamma receptors in dendritic cells and macrophages. Nat Rev Immunol (2014) 14:94–108. doi:10.1038/nri3582
21. Zhu X, Meng G, Dickinson BL, Li X, Mizoguchi E, Miao L, et al. MHC class I-related neonatal Fc receptor for IgG is functionally expressed in monocytes, intestinal macrophages, and dendritic cells. J Immunol (2001) 166:3266–76. doi:10.4049/jimmunol.166.5.3266
22. Vidarsson G, Stemerding AM, Stapleton NM, Spliethoff SE, Janssen H, Rebers FE, et al. FcRn: an IgG receptor on phagocytes with a novel role in phagocytosis. Blood (2006) 108:3573–9. doi:10.1182/blood-2006-05-024539
23. Roopenian DC, Akilesh S. FcRn: the neonatal Fc receptor comes of age. Nat Rev Immunol (2007) 7:715–25. doi:10.1038/nri2155
24. Smith KG, Clatworthy MR. FcgammaRIIB in autoimmunity and infection: evolutionary and therapeutic implications. Nat Rev Immunol (2010) 10:328–43. doi:10.1038/nri2762
25. Pricop L, Redecha P, Teillaud JL, Frey J, Fridman WH, Sautes-Fridman C, et al. Differential modulation of stimulatory and inhibitory Fc gamma receptors on human monocytes by Th1 and Th2 cytokines. J Immunol (2001) 166:531–7. doi:10.4049/jimmunol.166.1.531
26. van der Poel CE, Karssemeijer RA, Boross P, van der Linden JA, Blokland M, van de Winkel JG, et al. Cytokine-induced immune complex binding to the high-affinity IgG receptor, FcgammaRI, in the presence of monomeric IgG. Blood (2010) 116:5327–33. doi:10.1182/blood-2010-04-280214
27. Daëron M. Fc receptor biology. Annu Rev Immunol (1997) 15:203–34. doi:10.1146/annurev.immunol.15.1.203
28. Nimmerjahn F, Ravetch JV. Fcgamma receptors as regulators of immune responses. Nat Rev Immunol (2008) 8:34–47. doi:10.1038/nri2206
29. Blank U, Launay P, Benhamou M, Monteiro RC. Inhibitory ITAMs as novel regulators of immunity. Immunol Rev (2009) 232:59–71. doi:10.1111/j.1600-065X.2009.00832.x
30. Amigorena S, Bonnerot C, Drake JR, Choquet D, Hunziker W, Guillet JG, et al. Cytoplasmic domain heterogeneity and functions of IgG Fc receptors in B lymphocytes. Science (1992) 256:1808–12. doi:10.1126/science.1535455
31. Ono M, Bolland S, Tempst P, Ravetch JV. Role of the inositol phosphatase SHIP in negative regulation of the immune system by the receptor Fc(gamma)RIIB. Nature (1996) 383:263–6. doi:10.1038/383263a0
32. Zhu Z, Li R, Li H, Zhou T, Davis RS. FCRL5 exerts binary and compartment-specific influence on innate-like B-cell receptor signaling. Proc Natl Acad Sci U S A (2013) 110:E1282–90. doi:10.1073/pnas.1215156110
33. Kimberly RP, Ahlstrom JW, Click ME, Edberg JC. The glycosyl phosphatidylinositol-linked Fc gamma RIIIPMN mediates transmembrane signaling events distinct from Fc gamma RII. J Exp Med (1990) 171:1239–55. doi:10.1084/jem.171.4.1239
34. Tsuboi N, Asano K, Lauterbach M, Mayadas TN. Human neutrophil Fcgamma receptors initiate and play specialized nonredundant roles in antibody-mediated inflammatory diseases. Immunity (2008) 28:833–46. doi:10.1016/j.immuni.2008.04.013
35. Todd RF III, Petty HR. Beta 2 (CD11/CD18) integrins can serve as signaling partners for other leukocyte receptors. J Lab Clin Med (1997) 129:492–8. doi:10.1016/S0022-2143(97)90003-2
36. McEwan WA, Tam JC, Watkinson RE, Bidgood SR, Mallery DL, James LC. Intracellular antibody-bound pathogens stimulate immune signaling via the Fc receptor TRIM21. Nat Immunol (2013) 14:327–36. doi:10.1038/ni.2548
37. Heijnen IA, van Vugt MJ, Fanger NA, Graziano RF, De Wit TP, Hofhuis FM, et al. Antigen targeting to myeloid-specific human Fc gamma RI/CD64 triggers enhanced antibody responses in transgenic mice. J Clin Invest (1996) 97:331–8. doi:10.1172/JCI118420
38. Warmerdam PA, van de Winkel JG, Gosselin EJ, Capel PJ. Molecular basis for a polymorphism of human Fc gamma receptor II (CD32). J Exp Med (1990) 172:19–25. doi:10.1084/jem.172.1.19
39. Parren PW, Warmerdam PA, Boeije LC, Arts J, Westerdaal NA, Vlug A, et al. On the interaction of IgG subclasses with the low affinity Fc gamma RIIa (CD32) on human monocytes, neutrophils, and platelets. Analysis of a functional polymorphism to human IgG2. J Clin Invest (1992) 90:1537–46. doi:10.1172/JCI116022
40. Salmon JE, Edberg JC, Brogle NL, Kimberly RP. Allelic polymorphisms of human Fc gamma receptor IIA and Fc gamma receptor IIIB. Independent mechanisms for differences in human phagocyte function. J Clin Invest (1992) 89:1274–81. doi:10.1172/JCI115712
41. van der Heijden J, Geissler J, van Mirre E, van Deuren M, van der Meer JW, Salama A, et al. A novel splice variant of FcgammaRIIa: a risk factor for anaphylaxis in patients with hypogammaglobulinemia. J Allergy Clin Immunol (2013) 131(1408–1416):e1405. doi:10.1016/j.jaci.2013.02.009
42. Su K, Wu J, Edberg JC, Li X, Ferguson P, Cooper GS, et al. A promoter haplotype of the immunoreceptor tyrosine-based inhibitory motif-bearing FcγRIIb alters receptor expression and associates with autoimmunity. I. Regulatory FCGR2B polymorphisms and their association with systemic lupus erythematosus. J Immunol (2004) 172:7186–91. doi:10.4049/jimmunol.172.11.7186
43. Li X, Wu J, Carter RH, Edberg JC, Su K, Cooper GS, et al. A novel polymorphism in the Fcγ receptor IIB (CD32B) transmembrane region alters receptor signaling. Arthritis Rheum (2003) 48:3242–52. doi:10.1002/art.11313
44. Breunis WB, van Mirre E, Bruin M, Geissler J, De Boer M, Peters M, et al. Copy number variation of the activating FCGR2C gene predisposes to idiopathic thrombocytopenic purpura. Blood (2008) 111:1029–38. doi:10.1182/blood-2007-03-079913
45. Wu J, Edberg JC, Redecha PB, Bansal V, Guyre PM, Coleman K, et al. A novel polymorphism of FcgammaRIIIa (CD16) alters receptor function and predisposes to autoimmune disease. J Clin Invest (1997) 100:1059–70. doi:10.1172/JCI119616
46. Ferrara C, Stuart F, Sondermann P, Brunker P, Umana P. The carbohydrate at FcgammaRIIIa Asn-162. An element required for high affinity binding to non-fucosylated IgG glycoforms. J Biol Chem (2006) 281:5032–6. doi:10.1074/jbc.M510171200
47. Breunis WB, van Mirre E, Geissler J, Laddach N, Wolbink G, van der Schoot E, et al. Copy number variation at the FCGR locus includes FCGR3A, FCGR2C and FCGR3B but not FCGR2A and FCGR2B. Hum Mutat (2009) 30:E640–50. doi:10.1002/humu.20997
48. Ory PA, Clark MR, Kwoh EE, Clarkson SB, Goldstein IM. Sequences of complementary DNAs that encode the NA1 and NA2 forms of Fc receptor III on human neutrophils. J Clin Invest (1989) 84:1688–91. doi:10.1172/JCI114350
49. Salmon JE, Edberg JC, Kimberly RP. Fc gamma receptor III on human neutrophils. Allelic variants have functionally distinct capacities. J Clin Invest (1990) 85:1287–95. doi:10.1172/JCI114566
50. Bux J, Stein EL, Bierling P, Fromont P, Clay M, Stroncek D, et al. Characterization of a new alloantigen (SH) on the human neutrophil Fc gamma receptor IIIb. Blood (1997) 89:1027–34.
51. Koene HR, Kleijer M, Roos D, De Haas M, Von Dem Borne AEGK. FcγRIIIB gene duplication: evidence for presence and expression of three distinct FcγRIIIB genes in NA(1+,2+)SH(+) individuals. Blood (1998) 91:673–9.
52. Willcocks LC, Lyons PA, Clatworthy MR, Robinson JI, Yang W, Newland SA, et al. Copy number of FCGR3B, which is associated with systemic lupus erythematosus, correlates with protein expression and immune complex uptake. J Exp Med (2008) 205:1573–82. doi:10.1084/jem.20072413
53. Warmerdam PA, van de Winkel JG, Vlug A, Westerdaal NA, Capel PJ. A single amino acid in the second Ig-like domain of the human Fc gamma receptor II is critical for human IgG2 binding. J Immunol (1991) 147:1338–43.
54. Osborne JM, Chacko GW, Brandt JT, Anderson CL. Ethnic variation in frequency of an allelic polymorphism of human Fc gamma RIIA determined with allele specific oligonucleotide probes. J Immunol Methods (1994) 173:207–17. doi:10.1016/0022-1759(94)90299-2
55. Lehrnbecher T, Foster CB, Zhu S, Leitman SF, Goldin LR, Huppi K, et al. Variant genotypes of the low-affinity Fcγ receptors in two control populations and a review of low-affinity Fcγ receptor polymorphisms in control and disease populations. Blood (1999) 94:4220–32.
56. van der Heijden J, Nagelkerke S, Zhao X, Geissler J, Rispens T, van den Berg TK, et al. Haplotypes of FcgammaRIIa and FcgammaRIIIb polymorphic variants influence IgG-mediated responses in neutrophils. J Immunol (2014) 192(6):2715–21. doi:10.4049/jimmunol.1203570
57. Floto RA, Clatworthy MR, Heilbronn KR, Rosner DR, Macary PA, Rankin A, et al. Loss of function of a lupus-associated Fc[gamma]RIIb polymorphism through exclusion from lipid rafts. Nat Med (2005) 11:1056–8. doi:10.1038/nm1288
58. Takai T. Fc receptors and their role in immune regulation and autoimmunity. J Clin Immunol (2005) 25:1–18. doi:10.1007/s10875-005-0353-8
59. Huizinga T, Kleijer M, Tetteroo P, Roos D, Von Dem Borne A. Biallelic neutrophil Na-antigen system is associated with a polymorphism on the phospho-inositol-linked Fc gamma receptor III (CD16). Blood (1990) 75:213–7.
60. Dijstelbloem HM, Scheepers RHM, Oost WW, Stegeman CA, van der Pol WL, Sluiter WJ, et al. Fcγ receptor polymorphisms in Wegener’s granulomatosis: risk factors for disease relapse. Arthritis Rheum (1999) 42:1823–7. doi:10.1002/1529-0131(199909)42:9<1823::AID-ANR5>3.0.CO;2-X
61. Dijstelbloem HM, Bijl M, Fijnheer R, Scheepers RHM, Oost WW, Jansen MD, et al. Fcγ receptor polymorphisms in systemic lupus erythematosus: association with disease and in vivo clearance of immune complexes. Arthritis Rheum (2000) 43:2793–800. doi:10.1002/1529-0131(200012)43:12<2793::AID-ANR20>3.0.CO;2-6
62. Bruhns P. Properties of mouse and human IgG receptors and their contribution to disease models. Blood (2012) 119:5640–9. doi:10.1182/blood-2012-01-380121
63. Cartron G, Dacheux L, Salles G, Solal-Celigny P, Bardos P, Colombat P, et al. Therapeutic activity of humanized anti-CD20 monoclonal antibody and polymorphism in IgG Fc receptor FcgammaRIIIa gene. Blood (2002) 99:754–8. doi:10.1182/blood.V99.3.754
64. Cooper N, Stasi R, Cunningham-Rundles S, Cesarman E, McFarland JG, Bussel JB. Platelet-associated antibodies, cellular immunity and FCGR3a genotype influence the response to rituximab in immune thrombocytopenia. Br J Haematol (2012) 158:539–47. doi:10.1111/j.1365-2141.2012.09184.x
65. Ruyssen-Witrand A, Rouanet S, Combe B, Dougados M, Le Loët X, Sibilia J, et al. Fcγ receptor type IIIA polymorphism influences treatment outcomes in patients with rheumatoid arthritis treated with rituximab. Ann Rheum Dis (2012) 71:875–7. doi:10.1136/annrheumdis-2011-200337
66. Louis E, El Ghoul Z, Vermeire S, Dall’ozzo S, Rutgeerts P, Paintaud G, et al. Association between polymorphism in IgG Fc receptor IIIa coding gene and biological response to infliximab in Crohn’s disease. Aliment Pharmacol Ther (2004) 19:511–9. doi:10.1111/j.1365-2036.2004.01871.x
67. Moroi R, Endo K, Kinouchi Y, Shiga H, Kakuta Y, Kuroha M, et al. FCGR3A-158 polymorphism influences the biological response to infliximab in Crohn’s disease through affecting the ADCC activity. Immunogenetics (2013) 65:265–71. doi:10.1007/s00251-013-0679-8
68. Tutuncu Z, Kavanaugh A, Zvaifler N, Corr M, Deutsch R, Boyle D. Fcγ receptor type IIIA polymorphisms influence treatment outcomes in patients with inflammatory arthritis treated with tumor necrosis factor α-blocking agents. Arthritis Rheum (2005) 52:2693–6. doi:10.1002/art.21266
69. Kastbom A, Bratt J, Ernestam S, Lampa J, Padyukov L, Söderkvist P, et al. Fcγ receptor type IIIA genotype and response to tumor necrosis factor α-blocking agents in patients with rheumatoid arthritis. Arthritis Rheum (2007) 56:448–52. doi:10.1002/art.22390
70. Fujimoto T-T, Inoue M, Shimomura T, Fujimura K. Involvement of Fcγ receptor polymorphism in the therapeutic response of idiopathic thrombocytopenic purpura. Br J Haematol (2001) 115:125–30. doi:10.1046/j.1365-2141.2001.03109.x
71. Shrestha S, Wiener H, Shendre A, Kaslow RA, Wu J, Olson A, et al. Role of activating FcγR gene polymorphisms in Kawasaki disease susceptibility and intravenous immunoglobulin response. Circ Cardiovasc Genet (2012) 5:309–16. doi:10.1161/CIRCGENETICS.111.962464
72. Makowsky R, Wiener HW, Ptacek TS, Silva M, Shendre A, Edberg JC, et al. FcgammaR gene copy number in Kawasaki disease and intravenous immunoglobulin treatment response. Pharmacogenet Genomics (2013) 23:455–62. doi:10.1097/FPC.0b013e328363686e
73. Shrestha S, Wiener HW, Olson AK, Edberg JC, Bowles NE, Patel H, et al. Functional FCGR2B gene variants influence intravenous immunoglobulin response in patients with Kawasaki disease. J Allergy Clin Immunol (2011) 128:677–80. doi:10.1016/j.jaci.2011.04.027
74. Lejeune J, Thibault G, Ternant D, Cartron G, Watier H, Ohresser M. Evidence for linkage disequilibrium between Fcgamma RIIIa-V158F and Fcgamma RIIa-H131R polymorphisms in white patients, and for an Fcgamma RIIIa-restricted influence on the response to therapeutic antibodies. J Clin Oncol (2008) 26:5489–91. doi:10.1200/JCO.2008.19.4118
75. Raknes G, Skeie GO, Gilhus NE, Aadland S, Vedeler C. FcγRIIA and FcγRIIIB polymorphisms in myasthenia gravis. J Neuroimmunol (1998) 81:173–6. doi:10.1016/S0165-5728(97)00174-4
76. van der Pol W-L, van den Berg LH, Scheepers RHM, van der Bom JG, van Doorn PA, van Koningsveld R, et al. IgG receptor IIa alleles determine susceptibility and severity of Guillain-Barré syndrome. Neurology (2000) 54:1661–5. doi:10.1212/WNL.54.8.1661
77. Foster CB, Zhu S, Erichsen HC, Lehrnbecher T, Hart ES, Choi E, et al. Polymorphisms in inflammatory cytokines and Fcγ receptors in childhood chronic immune thrombocytopenic purpura: a pilot study. Br J Haematol (2001) 113:596–9. doi:10.1046/j.1365-2141.2001.02807.x
78. Bournazos S, Grinfeld J, Alexander K, Murchison J, Wallace W, McFarlane P, et al. Association of FcgammaRIIa R131H polymorphism with idiopathic pulmonary fibrosis severity and progression. BMC Pulm Med (2010) 10:51. doi:10.1186/1471-2466-10-51
79. Khor CC, Davila S, Breunis WB, Lee Y-C, Shimizu C, Wright VJ, et al. Genome-wide association study identifies FCGR2A as a susceptibility locus for Kawasaki disease. Nat Genet (2011) 43:1241–6. doi:10.1038/ng.981
80. Sanders LAM, van de Winkel JGJ, Rijkers GT, Voorhorst-Ogink MM, De Haas M, Capel PJA, et al. Fcã receptor Iia (Cd32) heterogeneity in patients with recurrent bacterial respiratory tract infections. J Infect Dis (1994) 170:854–61. doi:10.1093/infdis/170.4.854
81. Duits AJ, Bootsma H, Derksen RH, Spronk PE, Kater L, Kallenberg CG, et al. Skewed distribution of IgG Fc receptor IIa (CD32) polymorphism is associated with renal disease in systemic lupus erythematosus patients. Arthritis Rheum (1995) 38:1832–6. doi:10.1002/art.1780381217
82. Edberg JC, Wainstein E, Wu J, Csernok E, Sneller MC, Hoffman GS, et al. Analysis of FcgammaRII gene polymorphisms in Wegener’s granulomatosis. Exp Clin Immunogenet (1997) 14:183–95.
83. Haseley LA, Wisnieski JJ, Denburg MR, Michael-Grossman AR, Ginzler EM, Gourley MF, et al. Antibodies to C1q in systemic lupus erythematosus: characteristics and relation to Fc gamma RIIA alleles. Kidney Int (1997) 52:1375–80. doi:10.1038/ki.1997.464
84. Williams Y, Lynch S, McCann S, Smith O, Feighery C, Whelan A. Correlation of platelet FcγRIIA polymorphism in refractory idiopathic (immune) thrombocytopenic purpura. Br J Haematol (1998) 101:779–82. doi:10.1046/j.1365-2141.1998.00802.x
85. Myhr K-M, Raknes G, Nyland H, Vedeler C. Immunoglobulin G Fc-receptor (FcγR) IIA and IIIB polymorphisms related to disability in MS. Neurology (1999) 52:1771. doi:10.1212/WNL.52.9.1771
86. Norsworthy P, Theodoridis E, Botto M, Athanassiou P, Beynon H, Gordon C, et al. Overrepresentation of the Fcγ receptor type IIA R131/R131 genotype in Caucasoid systemic lupus erythematosus patients with autoantibodies to C1q and glomerulonephritis. Arthritis Rheum (1999) 42:1828–32. doi:10.1002/1529-0131(199909)42:9<1828::AID-ANR6>3.0.CO;2-F
87. Yun HR, Koh HK, Kim SS, Chung WT, Kim DW, Hong KP, et al. FcgammaRIIa/IIIa polymorphism and its association with clinical manifestations in Korean lupus patients. Lupus (2001) 10:466–72. doi:10.1191/096120301678416015
88. Karassa FB, Trikalinos TA, Ioannidis JPA. Role of the Fcγ receptor IIa polymorphism in susceptibility to systemic lupus erythematosus and lupus nephritis: a meta-analysis. Arthritis Rheum (2002) 46:1563–71. doi:10.1002/art.10306
89. Karassa FB, Bijl M, Davies KA, Kallenberg CGM, Khamashta MA, Manger K, et al. Role of the Fcγ receptor IIA polymorphism in the antiphospholipid syndrome: an international meta-analysis. Arthritis Rheum (2003) 48:1930–8. doi:10.1002/art.11059
90. Tanaka Y, Suzuki Y, Tsuge T, Kanamaru Y, Horikoshi S, Monteiro RC, et al. FcgammaRIIa-131R allele and FcgammaRIIIa-176V/V genotype are risk factors for progression of IgA nephropathy. Nephrol Dial Transplant (2005) 20:2439–45. doi:10.1093/ndt/gfi043
91. Morgan AW, Robinson JI, Barrett JH, Martin J, Walker A, Babbage SJ, et al. Association of FCGR2A and FCGR2A-FCGR3A haplotypes with susceptibility to giant cell arteritis. Arthritis Res Ther (2006) 8:R109. doi:10.1186/ar1996
92. Gulen F, Tanac R, Altinoz S, Berdeli A, Zeyrek D, Koksoy H, et al. The FcγRIIa polymorphism in Turkish children with asthma bronchial and allergic rhinitis. Clin Biochem (2007) 40:392–6. doi:10.1016/j.clinbiochem.2006.11.014
93. Aksu K, Kitapcioglu G, Keser G, Berdeli A, Karabulut G, Kobak S, et al. FcgammaRIIa, IIIa and IIIb gene polymorphisms in Behcet’s disease: do they have any clinical implications? Clin Exp Rheumatol (2008) 26:S77–83.
94. Woo J-H, Sung Y-K, Lee J-S, Chung WT, Choe J-Y, Song GG, et al. Association of Fcγ receptor polymorphisms with adult onset still’s disease in Korea. J Rheumatol (2009) 36:347–50. doi:10.3899/jrheum.071254
95. Kyogoku C, Dijstelbloem HM, Tsuchiya N, Hatta Y, Kato H, Yamaguchi A, et al. Fcγ receptor gene polymorphisms in Japanese patients with systemic lupus erythematosus: contribution of FCGR2B to genetic susceptibility. Arthritis Rheum (2002) 46:1242–54. doi:10.1002/art.10257
96. Siriboonrit U, Tsuchiya N, Sirikong M, Kyogoku C, Bejrachandra S, Suthipinittharm P, et al. Association of Fcγ receptor IIb and IIIb polymorphisms with susceptibility to systemic lupus erythematosus in Thais. Tissue Antigens (2003) 61:374–83. doi:10.1034/j.1399-0039.2003.00047.x
97. Kono H, Kyogoku C, Suzuki T, Tsuchiya N, Honda H, Yamamoto K, et al. FcγRIIB Ile232Thr transmembrane polymorphism associated with human systemic lupus erythematosus decreases affinity to lipid rafts and attenuates inhibitory effects on B cell receptor signaling. Hum Mol Genet (2005) 14:2881–92. doi:10.1093/hmg/ddi320
98. Willcocks LC, Carr EJ, Niederer HA, Rayner TF, Williams TN, Yang W, et al. A defunctioning polymorphism in FCGR2B is associated with protection against malaria but susceptibility to systemic lupus erythematosus. Proc Natl Acad Sci U S A (2010) 107:7881–5. doi:10.1073/pnas.0915133107
99. Zhou X-J, Lv J-C, Yu L, Cui Z, Zhao J, Yang R, et al. FCGR2B gene polymorphism rather than FCGR2A, FCGR3A and FCGR3B is associated with anti-GBM disease in Chinese. Nephrol Dial Transplant (2010) 25:97–101. doi:10.1093/ndt/gfp374
100. Blank M, Stefanescu R, Masuda E, Marti F, King P, Redecha P, et al. Decreased transcription of the human FCGR2B gene mediated by the -343 G/C promoter polymorphism and association with systemic lupus erythematosus. Hum Genet (2005) 117:220–7. doi:10.1007/s00439-005-1302-3
101. Tackenberg B, Jelcic I, Baerenwaldt A, Oertel WH, Sommer N, Nimmerjahn F, et al. Impaired inhibitory Fcγ receptor IIB expression on B cells in chronic inflammatory demyelinating polyneuropathy. Proc Natl Acad Sci U S A (2009) 106:4788–92. doi:10.1073/pnas.0807319106
102. Koene HR, Kleijer M, Swaak AJ, Sullivan KE, Bijl M, Petri MA, et al. The Fc gammaRIIIA-158F allele is a risk factor for systemic lupus erythematosus. Arthritis Rheum (1998) 41:1813–8. doi:10.1002/1529-0131(199810)41:10<1813::AID-ART13>3.3.CO;2-Y
103. Nieto A, Cáliz R, Pascual M, Matarán L, García S, Martín J. Involvement of Fcγ receptor IIIA genotypes in susceptibility to rheumatoid arthritis. Arthritis Rheum (2000) 43:735–9. doi:10.1002/1529-0131(200004)43:4<735::AID-ANR3>3.0.CO;2-Q
104. Edberg JC, Langefeld CD, Wu J, Moser KL, Kaufman KM, Kelly J, et al. Genetic linkage and association of Fcγ receptor IIIA (CD16A) on chromosome 1q23 with human systemic lupus erythematosus. Arthritis Rheum (2002) 46:2132–40. doi:10.1002/art.10438
105. van Sorge NM, van der Pol WL, Jansen MD, Geleijns KPW, Kalmijn S, Hughes RAC, et al. Severity of Guillain-Barré syndrome is associated with Fcγ receptor III polymorphisms. J Neuroimmunol (2005) 162:157–64. doi:10.1016/j.jneuroim.2005.01.016
106. Morgan AW, Griffiths B, Ponchel F, Montague BMN, Ali M, Gardner PP, et al. Fcγ receptor type IIIA is associated with rheumatoid arthritis in two distinct ethnic groups. Arthritis Rheum (2000) 43:2328–34. doi:10.1002/1529-0131(200010)43:10<2328::AID-ANR21>3.0.CO;2-Z
107. Lee YH, Ji JD, Song GG. Associations between FCGR3A polymorphisms and susceptibility to rheumatoid arthritis: a meta analysis. J Rheumatol (2008) 35:2129–35. doi:10.3899/jrheum.080186
108. Bronner IM, Hoogendijk JE, De Visser M, van de Vlekkert J, Badrising UA, Wintzen AR, et al. Association of the leukocyte immunoglobulin G (Fcγ) receptor IIIa-158V/F polymorphism with inflammatory myopathies in Dutch patients. Tissue Antigens (2009) 73:586–9. doi:10.1111/j.1399-0039.2009.01236.x
109. Thabet MM, Huizinga TWJ, Marques RB, Stoeken-Rijsbergen G, Bakker AM, Kurreeman FA, et al. Contribution of Fcγ receptor IIIA gene 158V/F polymorphism and copy number variation to the risk of ACPA-positive rheumatoid arthritis. Ann Rheum Dis (2009) 68:1775–80. doi:10.1136/ard.2008.099309
110. Zhou X-J, Lv J-C, Bu D-F, Yu L, Yang Y-R, Zhao J, et al. Copy number variation of FCGR3A rather than FCGR3B and FCGR2B is associated with susceptibility to anti-GBM disease. Int Immunol (2010) 22:45–51. doi:10.1093/intimm/dxp113
111. Hatta Y, Tsuchiya N, Ohashi J, Matsushita M, Fujiwara K, Hagiwara K, et al. Association of Fc gamma receptor IIIB, but not of Fc gamma receptor IIA and IIIA polymorphisms with systemic lupus erythematosus in Japanese. Genes Immun (1999) 1:53–60. doi:10.1038/sj.gene.6363639
112. Tse WY, Abadeh S, Jefferis R, Savage CO, Adu D. Neutrophil FcgammaRIIIb allelic polymorphism in anti-neutrophil cytoplasmic antibody (ANCA)-positive systemic vasculitis. Clin Exp Immunol (2000) 119:574–7. doi:10.1046/j.1365-2249.2000.01182.x
113. Xu G, He Q, Shou Z, Wang H, Zhang X, Wang Y, et al. NA1/NA2 heterozygote of Fcgr3b is a risk factor for progression of IgA nephropathy in Chinese. J Clin Lab Anal (2007) 21:298–302. doi:10.1002/jcla.20189
114. Aitman TJ, Dong R, Vyse TJ, Norsworthy PJ, Johnson MD, Smith J, et al. Copy number polymorphism in Fcgr3 predisposes to glomerulonephritis in rats and humans. Nature (2006) 439:851–5. doi:10.1038/nature04489
115. Fanciulli M, Norsworthy PJ, Petretto E, Dong R, Harper L, Kamesh L, et al. FCGR3B copy number variation is associated with susceptibility to systemic, but not organ-specific, autoimmunity. Nat Genet (2007) 39:721–3. doi:10.1038/ng2046
116. Bournazos S, Bournazou I, Murchison JT, Wallace WA, McFarlane P, Hirani N, et al. Copy number variation of <i>FCGR3B</i> is associated with susceptibility to idiopathic pulmonary fibrosis. Respiration (2011) 81:142–9. doi:10.1159/000321997
117. Graf SW, Lester S, Nossent JC, Hill CL, Proudman SM, Lee A, et al. Low copy number of the FCGR3B gene and rheumatoid arthritis: a case-control study and meta-analysis. Arthritis Res Ther (2012) 14:R28. doi:10.1186/ar3731
118. McKinney C, Broen JCA, Vonk MC, Beretta L, Hesselstrand R, Hunzelmann N, et al. Evidence that deletion at FCGR3B is a risk factor for systemic sclerosis. Genes Immun (2012) 13:458–60. doi:10.1038/gene.2012.15
119. Smith P, Dilillo DJ, Bournazos S, Li F, Ravetch JV. Mouse model recapitulating human Fcgamma receptor structural and functional diversity. Proc Natl Acad Sci U S A (2012) 109(16):6181–6. doi:10.1073/pnas.1203954109
120. Guyre PM, Graziano RF, Goldstein J, Wallace PK, Morganelli PM, Wardwell K, et al. Increased potency of Fc-receptor-targeted antigens. Cancer Immunol Immunother (1997) 45:146–8. doi:10.1007/s002620050418
121. Keler T, Guyre PM, Vitale LA, Sundarapandiyan K, van de Winkel JG, Deo YM, et al. Targeting weak antigens to CD64 elicits potent humoral responses in human CD64 transgenic mice. J Immunol (2000) 165:6738–42. doi:10.4049/jimmunol.165.12.6738
122. Heijnen IA, van de Winkel JG. A human Fc gamma RI/CD64 transgenic model for in vivo analysis of (bispecific) antibody therapeutics. J Hematother (1995) 4:351–6. doi:10.1089/scd.1.1995.4.351
123. Stavenhagen JB, Gorlatov S, Tuaillon N, Rankin CT, Li H, Burke S, et al. Fc optimization of therapeutic antibodies enhances their ability to kill tumor cells in vitro and controls tumor expansion in vivo via low-affinity activating Fcgamma receptors. Cancer Res (2007) 67:8882–90. doi:10.1158/0008-5472.CAN-07-0696
124. Junttila TT, Parsons K, Olsson C, Lu Y, Xin Y, Theriault J, et al. Superior in vivo efficacy of afucosylated trastuzumab in the treatment of HER2-amplified breast cancer. Cancer Res (2010) 70:4481–9. doi:10.1158/0008-5472.CAN-09-3704
125. Gerdes CA, Nicolini VG, Herter S, van Puijenbroek E, Lang S, Roemmele M, et al. GA201 (RG7160): a novel, humanized, glycoengineered anti-EGFR antibody with enhanced ADCC and superior in vivo efficacy compared with cetuximab. Clin Cancer Res (2013) 19:1126–38. doi:10.1158/1078-0432.CCR-12-0989
126. Kim JM, Ashkenazi A. Fcgamma receptors enable anticancer action of proapoptotic and immune-modulatory antibodies. J Exp Med (2013) 210:1647–51. doi:10.1084/jem.20131625
127. Mellor JD, Brown MP, Irving HR, Zalcberg JR, Dobrovic A. A critical review of the role of Fc gamma receptor polymorphisms in the response to monoclonal antibodies in cancer. J Hematol Oncol (2013) 6:1. doi:10.1186/1756-8722-6-1
128. Clynes R, Ravetch JV. Cytotoxic antibodies trigger inflammation through Fc receptors. Immunity (1995) 3:21–6. doi:10.1016/1074-7613(95)90155-8
129. McKenzie SE, Taylor SM, Malladi P, Yuhan H, Cassel DL, Chien P, et al. The role of the human Fc receptor Fc gamma RIIA in the immune clearance of platelets: a transgenic mouse model. J Immunol (1999) 162:4311–8.
130. Taylor SM, Reilly MP, Schreiber AD, Chien P, Tuckosh JR, McKenzie SE. Thrombosis and shock induced by activating antiplatelet antibodies in human Fc gamma RIIA transgenic mice: the interplay among antibody, spleen, and Fc receptor. Blood (2000) 96:4254–60.
131. Reilly MP, Taylor SM, Hartman NK, Arepally GM, Sachais BS, Cines DB, et al. Heparin-induced thrombocytopenia/thrombosis in a transgenic mouse model requires human platelet factor 4 and platelet activation through FcgammaRIIA. Blood (2001) 98:2442–7. doi:10.1182/blood.V98.8.2442
132. Rauova L, Zhai L, Kowalska MA, Arepally GM, Cines DB, Poncz M. Role of platelet surface PF4 antigenic complexes in heparin-induced thrombocytopenia pathogenesis: diagnostic and therapeutic implications. Blood (2006) 107:2346–53. doi:10.1182/blood-2005-08-3122
133. Meyer T, Robles-Carrillo L, Robson T, Langer F, Desai H, Davila M, et al. Bevacizumab immune complexes activate platelets and induce thrombosis in FCGR2A transgenic mice. J Thromb Haemost (2009) 7:171–81. doi:10.1111/j.1538-7836.2008.03212.x
134. Robles-Carrillo L, Meyer T, Hatfield M, Desai H, Davila M, Langer F, et al. Anti-CD40L immune complexes potently activate platelets in vitro and cause thrombosis in FCGR2A transgenic mice. J Immunol (2010) 185:1577–83. doi:10.4049/jimmunol.0903888
135. Stolla M, Stefanini L, Andre P, Ouellette TD, Reilly MP, McKenzie SE, et al. CalDAG-GEFI deficiency protects mice in a novel model of Fcgamma RIIA-mediated thrombosis and thrombocytopenia. Blood (2011) 118:1113–20. doi:10.1182/blood-2011-03-342352
136. Jönsson F, Mancardi DA, Zhao W, Kita Y, Iannascoli B, Khun H, et al. Human FcgammaRIIA induces anaphylactic and allergic reactions. Blood (2012) 119:2533–44. doi:10.1182/blood-2011-07-367334
137. Petkova SB, Akilesh S, Sproule TJ, Christianson GJ, Al Khabbaz H, Brown AC, et al. Enhanced half-life of genetically engineered human IgG1 antibodies in a humanized FcRn mouse model: potential application in humorally mediated autoimmune disease. Int Immunol (2006) 18:1759–69. doi:10.1093/intimm/dxl110
138. Mancardi DA, Jonsson F, Iannascoli B, Khun H, van Rooijen N, Huerre M, et al. The murine high-affinity IgG receptor Fc(gamma)RIV is sufficient for autoantibody-induced arthritis. J Immunol (2011) 186:1899–903. doi:10.4049/jimmunol.1003642
139. Christianson GJ, Blankenburg RL, Duffy TM, Panka D, Roths JB, Marshak-Rothstein A, et al. beta2-microglobulin dependence of the lupus-like autoimmune syndrome of MRL-lpr mice. J Immunol (1996) 156:4932–9.
140. Tan Sardjono C, Mottram PL, van de Velde NC, Powell MS, Power D, Slocombe RF, et al. Development of spontaneous multisystem autoimmune disease and hypersensitivity to antibody-induced inflammation in Fcgamma receptor IIa-transgenic mice. Arthritis Rheum (2005) 52:3220–9. doi:10.1002/art.21344
141. van de Velde NC, Mottram PL, Powell MS, Lim B, Holmdahl R, Hogarth PM. Transgenic mice expressing human FcgammaRIIa have enhanced sensitivity to induced autoimmune arthritis as well as elevated Th17 cells. Immunol Lett (2010) 130:82–8. doi:10.1016/j.imlet.2009.12.005
142. Tsuboi N, Ernandez T, Li X, Nishi H, Cullere X, Mekala D, et al. Regulation of human neutrophil Fcgamma receptor IIa by C5a receptor promotes inflammatory arthritis in mice. Arthritis Rheum (2011) 63:467–78. doi:10.1002/art.30141
143. Pietersz GA, Mottram PL, van de Velde NC, Sardjono CT, Esparon S, Ramsland PA, et al. Inhibition of destructive autoimmune arthritis in FcgammaRIIa transgenic mice by small chemical entities. Immunol Cell Biol (2009) 87:3–12. doi:10.1038/icb.2008.82
144. van Vuuren AJ, van Roon JA, Walraven V, Stuij I, Harmsen MC, McLaughlin PM, et al. CD64-directed immunotoxin inhibits arthritis in a novel CD64 transgenic rat model. J Immunol (2006) 176:5833–8. doi:10.4049/jimmunol.176.10.5833
145. Thepen T, van Vuuren AJ, Kiekens RC, Damen CA, Vooijs WC, van de Winkel JG. Resolution of cutaneous inflammation after local elimination of macrophages. Nat Biotechnol (2000) 18:48–51. doi:10.1038/71908
146. Chen K, Nishi H, Travers R, Tsuboi N, Martinod K, Wagner DD, et al. Endocytosis of soluble immune complexes leads to their clearance by FcgammaRIIIB but induces neutrophil extracellular traps via FcgammaRIIA in vivo. Blood (2012) 120:4421–31. doi:10.1182/blood-2011-12-401133
147. van Vugt MJ, Heijnen AF, Capel PJ, Park SY, Ra C, Saito T, et al. FcR gamma-chain is essential for both surface expression and function of human Fc gamma RI (CD64) in vivo. Blood (1996) 87:3593–9.
148. van Royen-Kerkhof A, Sanders EA, Walraven V, Voorhorst-Ogink M, Saeland E, Teeling JL, et al. A novel human CD32 mAb blocks experimental immune haemolytic anaemia in FcgammaRIIA transgenic mice. Br J Haematol (2005) 130:130–7. doi:10.1111/j.1365-2141.2005.05571.x
149. Zhi H, Rauova L, Hayes V, Gao C, Boylan B, Newman DK, et al. Cooperative integrin/ITAM signaling in platelets enhances thrombus formation in vitro and in vivo. Blood (2013) 121:1858–67. doi:10.1182/blood-2012-07-443325
150. Horton HM, Chu SY, Ortiz EC, Pong E, Cemerski S, Leung IW, et al. Antibody-mediated coengagement of FcgammaRIIb and B cell receptor complex suppresses humoral immunity in systemic lupus erythematosus. J Immunol (2011) 186:4223–33. doi:10.4049/jimmunol.1003412
151. Li F, Ravetch JV. Inhibitory Fcgamma receptor engagement drives adjuvant and anti-tumor activities of agonistic CD40 antibodies. Science (2011) 333:1030–4. doi:10.1126/science.1206954
152. Li F, Ravetch JV. Antitumor activities of agonistic anti-TNFR antibodies require differential FcgammaRIIB coengagement in vivo. Proc Natl Acad Sci U S A (2013) 110:19501–6. doi:10.1073/pnas.1319502110
153. Li M, Wirthmueller U, Ravetch JV. Reconstitution of human Fc gamma RIII cell type specificity in transgenic mice. J Exp Med (1996) 183:1259–63. doi:10.1084/jem.183.3.1259
154. Roopenian DC, Christianson GJ, Sproule TJ, Brown AC, Akilesh S, Jung N, et al. The MHC class I-like IgG receptor controls perinatal IgG transport, IgG homeostasis, and fate of IgG-Fc-coupled drugs. J Immunol (2003) 170:3528–33. doi:10.4049/jimmunol.170.7.3528
Keywords: IgG receptors, transgenic mice, anaphylaxis, autoimmune diseases, genetic polymorphisms and disease association, human IgG receptors
Citation: Gillis C, Gouel-Chéron A, Jönsson F and Bruhns P (2014) Contribution of human FcγRs to disease with evidence from human polymorphisms and transgenic animal studies. Front. Immunol. 5:254. doi: 10.3389/fimmu.2014.00254
Received: 11 March 2014; Paper pending published: 29 March 2014;
Accepted: 14 May 2014; Published online: 30 May 2014.
Edited by:
Jan Terje Andersen, Oslo University Hosiptal, NorwayReviewed by:
Beatrice Jahn-Schmid, Medical University of Vienna, AustriaCopyright: © 2014 Gillis, Gouel-Chéron, Jönsson and Bruhns. This is an open-access article distributed under the terms of the Creative Commons Attribution License (CC BY). The use, distribution or reproduction in other forums is permitted, provided the original author(s) or licensor are credited and that the original publication in this journal is cited, in accordance with accepted academic practice. No use, distribution or reproduction is permitted which does not comply with these terms.
*Correspondence: Friederike Jönsson and Pierre Bruhns, Laboratoire Anticorps en Thérapie et Pathologie, Département d’Immunologie, Institut Pasteur, 25 rue du Docteur Roux, Paris 75015, France e-mail:am9lbnNzb25AcGFzdGV1ci5mcg==;YnJ1aG5zQHBhc3RldXIuZnI=
†Caitlin Gillis and Aurélie Gouel-Chéron have contributed equally to this work.
‡Friederike Jönsson and Pierre Bruhns are Co-senior authors.
Disclaimer: All claims expressed in this article are solely those of the authors and do not necessarily represent those of their affiliated organizations, or those of the publisher, the editors and the reviewers. Any product that may be evaluated in this article or claim that may be made by its manufacturer is not guaranteed or endorsed by the publisher.
Research integrity at Frontiers
Learn more about the work of our research integrity team to safeguard the quality of each article we publish.