- Singapore Immunology Network, Agency for Science, Technology and Research, Singapore
Dendritic cells (DCs) are uniquely potent antigen presenting cells that acquire microbial products and prime adaptive immune responses against pathogens. Furthermore, DCs also play a key role in induction and maintenance of tolerance. Although numerous studies have assessed the diverse functions of DCs, many unanswered questions remain regarding the molecular mechanisms that DCs use to achieve immunoregulation. While not widely regarded as a significant provider of T-cell growth factors, DCs have previously been identified as a potential source of IL-2 cytokine. Recent research indicates that microbes are the most effective stimuli to trigger IL-2 production in DCs by activating the calcineurin/NFAT signaling pathway. Herein we describe recent insights into the production and function of IL-2 cytokine and IL-2 receptor in DCs early after stimulation through pattern recognition receptors. These findings clarify how DCs fine-tune effector and regulatory responses by modulating IL-2 production in both tolerance and immunity.
Introduction
The emergence of multicellular organisms during evolution has been accompanied by the concomitant development of the innate immune system, which serves to protect the host from pathogen colonization. Co-evolution of multicellular systems and their associated microbiomes over millions of years has produced a sophisticated host receptor repertoire and signaling networks that respond to specific perturbations in homeostasis. This ability to sense the environment is best demonstrated by dendritic cells (DCs), which have developed the capacity to respond to ligation of specific pathogen recognition receptors (PRRs) in order to trigger adaptive immune responses. Among the many different responses induced by PRR ligation in DCs, we have found that specific agonists can stimulate translocation of the transcription factor “nuclear factor of activated T-cells” (NFAT) to the DC nucleus both in vitro and in vivo, resulting in the activation and regulation of gene expression (Granucci et al., 2001; Zanoni et al., 2009). Interestingly, one of the gene products regulated by NFAT in DCs is the interleukin (IL)-2 (Granucci et al., 2001), which is particularly crucial to the functioning of the immune system in both immune homeostasis and immunity (Malek, 2008). In the late 1970s, Kendall A. Smith and Steven Gillis described IL-2 cytokine as a T-cell growth factor produced by T lymphocytes (Smith, 1988). In the subsequent 40 years, the wider role played by IL-2 in regulating the adaptive immune response has been fully recognized. Although previous studies of this cytokine have focused primarily on T-cells, new findings have highlighted that IL-2 is also produced by other cell types and in various different settings. For these reasons, IL-2 can be defined as a pleiotropic cytokine with different functions extending from driving T-cell proliferation, supporting the activation of natural killer (NK) cells to the induction of pro-apoptotic gene transcription (Malek, 2008). Indeed, the many diverse functions of IL-2 are well demonstrated by the spontaneous development of autoimmune and inflammatory bowel diseases in mice that are deficient for IL-2 or the IL-2 receptor (IL-2R; O’Shea et al., 2002). Notably, adoptive transfer of IL-2-sufficient T-cells or administration of IL-2 cytokine can significantly ameliorate disease in these animals (Malek and Castro, 2010).
In the myeloid immune compartment, IL-2 gene transcription is inducible in DCs but not in macrophages or in other phagocytic cells (Granucci et al., 2001). The initial finding that IL-2 production by DCs is a major outcome of PRR ligation raised the question of whether it could contribute to adaptive immune responses. In this review we will summarize the current knowledge about the functional role of IL-2 as an innate cytokine.
Induction of IL-2 Transcription in DCs
Kinetic transcriptional profiling has shown that developmentally synchronized DCs grown in medium containing GM-CSF produce IL-2 within 4–6 h of stimulation with alive Gram-negative Escherichia coli bacteria. Interestingly, the kinetic of the IL-2 mRNA expression and of IL-2 protein production were similar. In contrast, macrophages are not able to produce IL-2 under these conditions (Granucci et al., 2001). Thus, the narrow window of transcription and secretion of IL-2 is unique to DCs.
Because of the crucial role played by DCs in initiating and regulating immune responses, it is key to clarify the kinetics of IL-2 production as well as the stimuli which elicit this cytokine and the signaling machinery which support IL-2-secretion by DCs. Indeed, DCs are well established, as critical mediators of antimicrobial immunity, and it would appear that microbial products are the most potent stimuli for IL-2 production by myeloid DCs. In this regard, Granucci et al. (2003) showed that LPS, peptidoglycan, and CpG ligands for Toll-like receptors (TLRs) were able to trigger variable levels of IL-2 release. In contrast, pro-inflammatory cytokines including TNF-α, IFN-α, and IL-1β failed to stimulate IL-2 production by DCs. Interestingly, myeloid DCs were also shown to produce very high amounts of IL-2 in response to zymosan or following challenge with live yeast Saccharomyces cerevisiae (Granucci et al., 2003). Immature DCs exhibit marked phagocytic activity, but phagocytosis of inert particles per se does not constitute a trigger for IL-2 by DCs (Granucci et al., 2003). In contrast, blockade of DC phagocytic activity by treatment with Latrunculin B inhibits antigen uptake and IL-2 production by these cells (Rogers et al., 2005) but does not impair the production of IL-12p40 and IL-10 in response to zymosan. This study also investigated the intracellular signaling pathway that leads to IL-2 production in DCs, showing that murine bone marrow-derived and splenic DCs grown in GM-CSF-enriched medium were able to produce large amounts of IL-2 cytokine via Syk kinase signaling and receptor dectin-1 binding. Dectin-1, also known as CLEC7A, is a C-type lectin receptor expressed in myeloid cells and specifically binds 1–3 β-glucan on the cell wall of fungi (Sancho and Reis e Sousa, 2012). The main feature of this PRR is that it expresses a hemITAM motif, which recruits Src kinase and Syk kinase (Figure 1). Syk then recruits CARD9 to the membrane and activates the canonical NF-κB signaling pathway through the CARD9/Blc10/Malt-1 complex. Dectin-1 can also activate the non-canonical NF-κB pathway through RelB. The pro-inflammatory response triggered by NF-κB signaling, with subsequent increases in IL-23p19, TNF-α, and IL-6 production, is counterbalanced by a regulatory response characterized by the production of IL-2 and IL-10 (Sancho and Reis e Sousa, 2012). In this context, IL-2 is likely to be transcribed through the NFAT transcriptional gene program (Granucci et al., 2001; Goodridge et al., 2007). The binding of 1–3 β-glucan to dectin-1 induces the dephosphorylation of Src kinases through the surface molecule CD45. Once activated, Src kinases phosphorylate the ITAM motif of dectin-1, which then forms a docking site for Syk. Phospholipase C (PLC)γ-2 is subsequently activated by Syk to stimulate increases in intracellular Ca2+ flux, culminating in the translocation of NFAT into the nucleus (Figure 1). Thus, uptake of fungal particles via dectin-1 is reminiscent of the synapse formed between T-cells and antigen presenting cells (Goodridge et al., 2011). This is due to the exclusion of CD45 from the phagocytic synapse when particles are recruited to the phagocytic cup, which facilitates prolonged dectin-1 signaling via Syk and subsequent NFAT translocation.
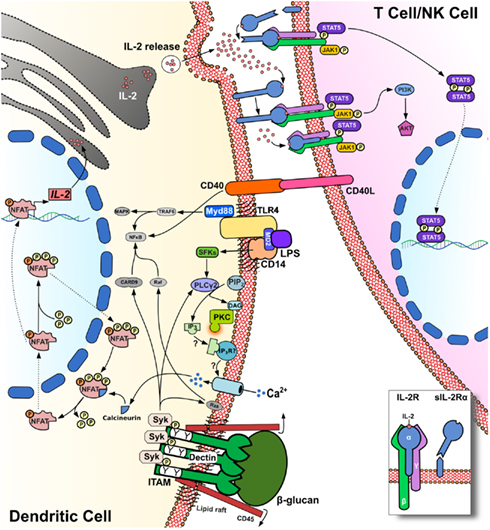
Figure 1. IL-2 release by DCs stimulation of PRRs triggers the calcineurin/NFAT pathway via PLC-γ2 and intracellular Ca2+ flux. NFAT dephosphorylation by calcineurin leads to IL-2 transcription and release, which is increased in response to CD40/CD40L interactions. CD25 (IL-2Rα) can capture IL-2 release in the synaptic cleft on the surface of DCs for presentation in trans to adjacent T-cells.
The molecules involved in NFAT signaling were primarily described in T-cells about 20 years ago. In contrast, NFAT expression in myeloid cells has been identified only recently (Muller and Rao, 2010). Furthermore we have shown that NFAT controls the development and homeostasis of the myeloid compartment (Fric et al., 2012). In resting DCs, NFAT remains phosphorylated in the cytoplasm, and the translocation of NFAT between cytoplasm and nuclei is tightly regulated by a number of protein kinases and phosphatases. The NFAT family of transcription factors comprises five members; NFAT1 (NFATc2 or NFATp), NFAT2 (NFATc or NFATc1), NFAT3 (NFATc4), NFAT4 (NFATx or NFATc3), and NFAT5 (TonEBP; Muller and Rao, 2010; Buxade et al., 2012). In 2009, it was reported that smooth LPS could trigger Ca2+ flux through CD14, independently of TLR4 ligation. LPS signaling through this pathway drives NFAT1 translocation and regulates various transcripts that control DC survival and apoptosis (Zanoni et al., 2009; Figure 1). LPS-induced Ca2+ mobilization was dependent on PLCγ-2 and required CD14 localization in lipid rafts. More recently, CD14 has also been shown to play an essential role for the internalization of TLR4, which is endocytosed in response to LPS stimulation via Syk and PLCγ-2 (Zanoni et al., 2011).
Re-phosphorylation of NFAT in the nucleus is also regulated by several kinases; CK1, GSK3, and DYRK. The phosphorylation status of NFAT in the cytoplasm of DCs can be further modulated by Leucine-rich repeat kinase 2 (also known as dardarin), a recently described negative regulator of NFAT1 signaling in myeloid cells (Liu et al., 2011). Calcineurin is a key calcium-dependent phosphatase, which mediates NFAT signaling (Jain et al., 1993; McCaffrey et al., 1993). Calcium flux stimulates calcineurin to dephosphorylate NFAT, thereby promoting NFAT translocation into nuclei. In T-cells, activation of this cascade induces gene transcription and leukocyte activation, thereby driving the production of NFAT-dependent cytokines including IL-2, IL-4, GM-CSF, and TNF-α (Rao et al., 1997).
More recently, mice that conditionally lack NFAT or calcineurin in myeloid cells were also studied and a key role for calcineurin/NFAT signaling in the innate immune compartment has been demonstrated (Greenblatt et al., 2010). Indeed the stimulation of myeloid cells with live fungi or with fungal cell wall extracts such as curdlan or zymosan results in the activation of a number of genes regulated through NFAT. In macrophages exposed to zymosan, transcription targets include genes which encode IL-10, IL-12, cyclooxygenase-2 (Cox-2), and members of the early growth response (Egr) family of transcription factors, Egr2 and Egr3 (Goodridge et al., 2007). In bone marrow-derived DCs, zymosan was similarly found to trigger IL-2, IL-10, and IL-12p70 production in an NFAT-dependent manner. Importantly, the levels of IL-2 released in response to β-glucan particles were comparable to the known levels of IL-2 normally detected in T-cell cultures in response to lectins (Slack et al., 2007). The intracellular levels were detected in response to zymosan particles at the same time points as in response to LPS (Rogers et al., 2005). Since zymosan also activates TLR2 and Myd88 signaling, the IL-2 production detected by these assays was found to be partially dependent on Myd88 expression. Intriguingly, IL-2 was seemingly co-produced with IL-10 in a Myd88-independent manner (Rogers et al., 2005). Zymosan-stimulated cytokine production was also found to depend at least in part on TLR2 expression (Goodridge et al., 2007). In DCs, ERK activation is required for maximal production of IL-2, which is Myd88-independent but does require Syk (Slack et al., 2007). These data clearly show that the Syk-dependent pattern recognition is extremely important for TLR-independent anti-fungal immune responses.
Immuno-Regulatory Role of DC-Produced IL-2
The role played by DC-derived IL-2 in immune responses has been difficult to interpret since many other innate and adaptive cell types can produce large quantities of this cytokine in vivo (Boyman and Sprent, 2012). Indirect evidence of a contribution from DC-derived IL-2 to host protection comes from the observation that DCs infected with murine cytomegalovirus (MCMV) lose the ability to produce IL-2 in response to LPS (Andrews et al., 2001). The finding that MCMV inhibits IL-2 production implies that DC production of this cytokine is detrimental to the pathogen and may therefore contribute to protective immunity. It is possible then that one strategy employed by MCMV to escape immune surveillance is by decreasing DC potential for T-cell priming (Andrews et al., 2001). In addition, it is known that IL-2 supports T-cell proliferation, drives the production of cytokines including IFN-γ and TNF-α, and regulates the homeostatic survival of lymphoid lineage cells. Recent investigations of how regulatory T-cells (Tregs) are induced and maintained have now demonstrated how IL-2 contributes to both tolerance and immunity (Cheng et al., 2011).
Several roles have been proposed for IL-2 cytokine produced by DCs. One hypothesis is that DC-derived IL-2 can enhance NK cell functions, and accordingly, cross talk between NK cells and IL-2-producing DCs increases IFN-γ production by the NK cells (Zanoni et al., 2005). DCs appear then to provide important stimuli for the priming and cytotoxic function of NK cells (Granucci et al., 2008). Notably it has been observed that IL-2-deficient DCs are impaired in their ability to stimulate the proliferation of allogeneic T-cells (Granucci et al., 2001). In mixed lymphocyte reactions, DCs primed with bacteria were used to stimulate allo-reactive CD4+ and CD8+ T-cells. These experiments confirmed that IL-2-deficient DCs were poor antigen presenting cells compared with wild-type DCs. Similarly, when T-cell proliferation was tested by flow cytometry, very few cycling T-cells were detected in co-cultures stimulated by IL-2-deficient DCs (Granucci et al., 2001). These data clearly indicate that IL-2 produced by DCs significantly affects T-cell activation in mice. In humans, the production of IL-2 by activated human DCs has been shown (Feau et al., 2005) and more recently the activation of human T-cells by DC-produced IL-2 was also revealed using an in vitro IL-2-secretion assay (Wuest et al., 2011). IL-2 also regulates important aspects of Treg biology including the thymic development and suppressive function of these cells (Cheng et al., 2011). This has led to the evaluation of potential therapeutic strategies that employ IL-2 cytokine to expand Tregs (Yamazaki et al., 2007). Guiducci et al. (2005) reported that DCs impaired in IL-2 production fail to expand Tregs in vivo, suggesting an important role for DC-derived IL-2 in the regulation of Treg biology. It has also been reported that IL-2 produced by DCs enhances CD40/CD40L interaction thus promoting Treg expansion (Guiducci et al., 2005). When DCs are loaded with cognate antigens and are co-cultured with T-cells IL-2-secretion may be detected in the immune synapse (Wuest et al., 2011). In the synaptic cleft, IL-2 released by DCs has been found to bind in an autocrine manner to the α-subunit of the IL-2R (CD25). This subunit is expressed on the surface of DCs and presents IL-2 in trans to the other two signaling chains of the IL-2R on T-cells (Figure 1). The IL-2-related cytokine IL-15 can signal in a similar fashion (Stonier and Schluns, 2010), and it is possible that DC secretion of IL-2 in the synaptic cleft may provide an efficient and previously unexpected “signal 3” for T-cells, including T effector and Tregs. Other than transpresentation, it might be also possible that DC IL-2R is important for autocrine maturation (Mnasria et al., 2008) and eventually may function as decoy receptor. It remains to be determined how the IL-2-secretion that follows PRR ligation in DCs can lead to either the activation of effectors or regulatory T-cell functions.
Innate IL-2 as a Potential New Target in Clinical Studies
We have shown that early IL-2 production by DCs in response to PRR ligation exerts important functions in both innate and adaptive leukocytes. The potential key role for the early produced IL-2 in innate responses requires tight regulation at the transcriptional level to control the absolute levels of this cytokine in the microenvironment and in the immune synapse. Immune homeostasis may depend in part on the ongoing production of IL-2, which exerts a critical influence on the differentiation and function of Tregs (Malek, 2008). Indeed, in a recent observational cohort study, daily sub-cutaneous administration of low-dose IL-2 was found to be associated with Treg expansion and a reduction in clinical manifestations of graft-versus-host disease (Koreth et al., 2011). More importantly, drugs such as cyclosporin A (CsA) and tacrolimus (FK506; Flanagan et al., 1991; McCaffrey et al., 1993) potently block the calcineurin/NFAT signaling pathway and are widely used to prevent transplant rejection and autoimmune diseases, but these drugs also increase risk of infection. Intriguingly, treatment with CsA can also increase susceptibility to Candida albicans infection in mice that lack conventional lymphocytes (Greenblatt et al., 2010) suggesting that the drug must also act on non-lymphoid cell types.
The newly uncovered functions of IL-2 might therefore explain how drugs that modify the calcineurin/NFAT pathway impact on immune homeostasis and induce side effects in treated patients. Indeed, the ability of DCs to produce IL-2 and other NFAT regulated proteins is impaired upon such treatment (Goodridge et al., 2007). The quantitative and qualitative effects of DC-derived IL-2 thus constitute a new level of regulation of the immune response after microbial encounter and open new paths for identification of new therapeutic targets.
Early IL-2 Production in Myeloid DCs in Different Tissues
• In the skin there are dermal DCs, epidermal DCs, and Langerhans cells (LCs) with very different functional capabilities (Kaplan, 2010a,b). Murine LCs stimulated with LPS in vitro and analyzed by flow cytometry display IL-2 production by intracellular staining. IL-2 production was abrogated by CsA treatment (Granucci et al., 2003).
• In the spleen, both CD8+ and CD8− subsets of CD11c+ DCs were found to produce IL-2 following injection with LPS, zymosan, or bacteria (Granucci et al., 2003).
• Genomic profiling of DCs and macrophages has confirmed that IL-2 transcription following PRR activation is an exclusive feature of DCs (Granucci et al., 2001).
• Human myeloid DCs produce IL-2 in response to LPS via a mechanism that depends on CD40L and IL-15 (Feau et al., 2005).
Conflict of Interest Statement
The authors declare that the research was conducted in the absence of any commercial or financial relationships that could be construed as a potential conflict of interest.
Acknowledgments
We wish to thank Dr. Neil McCarthy of Insight Editing London for critical review of the paper.
References
Andrews, D. M., Andoniou, C. E., Granucci, F., Ricciardi-Castagnoli, P., and Degli-Esposti, M. A. (2001). Infection of dendritic cells by murine cytomegalovirus induces functional paralysis. Nat. Immunol. 2, 1077–1084.
Boyman, O., and Sprent, J. (2012). The role of interleukin-2 during homeostasis and activation of the immune system. Nat. Rev. Immunol. 12, 180–190.
Buxade, M., Lunazzi, G., Minguillon, J., Iborra, S., Berga-Bolanos, R., Del Val, M., Aramburu, J., and Lopez-Rodriguez, C. (2012). Gene expression induced by Toll-like receptors in macrophages requires the transcription factor NFAT5. J. Exp. Med. 209, 379–393.
Cheng, G., Yu, A., and Malek, T. R. (2011). T-cell tolerance and the multi-functional role of IL-2R signaling in T-regulatory cells. Immunol. Rev. 241, 63–76.
Feau, S., Facchinetti, V., Granucci, F., Citterio, S., Jarrossay, D., Seresini, S., Protti, M. P., Lanzavecchia, A., and Ricciardi-Castagnoli, P. (2005). Dendritic cell-derived IL-2 production is regulated by IL-15 in humans and in mice. Blood 105, 697–702.
Flanagan, W. M., Corthesy, B., Bram, R. J., and Crabtree, G. R. (1991). Nuclear association of a T-cell transcription factor blocked by FK-506 and cyclosporin A. Nature 352, 803–807.
Fric, J., Lim, C. X., Koh, E. G., Hofmann, B., Chen, J., Tay, H. S., Mohammad Isa, S. A., Mortellaro, A., Ruedl, C., and Ricciardi-Castagnoli, P. (2012). Calcineurin/NFAT signalling inhibits myeloid haematopoiesis. EMBO Mol. Med. 4, 269–282.
Goodridge, H. S., Reyes, C. N., Becker, C. A., Katsumoto, T. R., Ma, J., Wolf, A. J., Bose, N., Chan, A. S., Magee, A. S., Danielson, M. E., Weiss, A., Vasilakos, J. P., and Underhill, D. M. (2011). Activation of the innate immune receptor Dectin-1 upon formation of a “phagocytic synapse.” Nature 472, 471–475.
Goodridge, H. S., Simmons, R. M., and Underhill, D. M. (2007). Dectin-1 stimulation by Candida albicans yeast or zymosan triggers NFAT activation in macrophages and dendritic cells. J. Immunol. 178, 3107–3115.
Granucci, F., Feau, S., Angeli, V., Trottein, F., and Ricciardi-Castagnoli, P. (2003). Early IL-2 production by mouse dendritic cells is the result of microbial-induced priming. J. Immunol. 170, 5075–5081.
Granucci, F., Vizzardelli, C., Pavelka, N., Feau, S., Persico, M., Virzi, E., Rescigno, M., Moro, G., and Ricciardi-Castagnoli, P. (2001). Inducible IL-2 production by dendritic cells revealed by global gene expression analysis. Nat. Immunol. 2, 882–888.
Granucci, F., Zanoni, I., and Ricciardi-Castagnoli, P. (2008). Central role of dendritic cells in the regulation and deregulation of immune responses. Cell. Mol. Life Sci. 65, 1683–1697.
Greenblatt, M. B., Aliprantis, A., Hu, B., and Glimcher, L. H. (2010). Calcineurin regulates innate antifungal immunity in neutrophils. J. Exp. Med. 207, 923–931.
Guiducci, C., Valzasina, B., Dislich, H., and Colombo, M. P. (2005). CD40/CD40L interaction regulates CD4+CD25+ T reg homeostasis through dendritic cell-produced IL-2. Eur. J. Immunol. 35, 557–567.
Jain, J., Mccaffrey, P. G., Miner, Z., Kerppola, T. K., Lambert, J. N., Verdine, G. L., Curran, T., and Rao, A. (1993). The T-cell transcription factor NFATp is a substrate for calcineurin and interacts with Fos and Jun. Nature 365, 352–355.
Kaplan, D. H. (2010a). In vivo function of Langerhans cells and dermal dendritic cells. Trends Immunol. 31, 446–451.
Koreth, J., Matsuoka, K., Kim, H. T., Mcdonough, S. M., Bindra, B., Alyea, E. P. III, Armand, P., Cutler, C., Ho, V. T., Treister, N. S., Bienfang, D. C., Prasad, S., Tzachanis, D., Joyce, R. M., Avigan, D. E., Antin, J. H., Ritz, J., and Soiffer, R. J. (2011). Interleukin-2 and regulatory T cells in graft-versus-host disease. N. Engl. J. Med. 365, 2055–2066.
Liu, Z., Lee, J., Krummey, S., Lu, W., Cai, H., and Lenardo, M. J. (2011). The kinase LRRK2 is a regulator of the transcription factor NFAT that modulates the severity of inflammatory bowel disease. Nat. Immunol. 12, 1063–1070.
Malek, T. R., and Castro, I. (2010). Interleukin-2 receptor signaling: at the interface between tolerance and immunity. Immunity 33, 153–165.
McCaffrey, P. G., Luo, C., Kerppola, T. K., Jain, J., Badalian, T. M., Ho, A. M., Burgeon, E., Lane, W. S., Lambert, J. N., Curran, T., Verdine, G. L., Rao, A., and Hogan, P. G. (1993). Isolation of the cyclosporin-sensitive T cell transcription factor NFATp. Science 262, 750–754.
Mnasria, K., Lagaraine, C., Manaa, J., Lebranchu, Y., and Oueslati, R. (2008). Anti CD25 treatment of human dendritic cells modulates both their cytokine synthesis profiles and their capacity to activate allogeneic CD4 T cells: a potential tolerogenic effect. Int. Immunopharmacol. 8, 414–422.
Muller, M. R., and Rao, A. (2010). Nfat, immunity and cancer: a transcription factor comes of age. Nat. Rev. Immunol. 10, 645–656.
O’Shea, J. J., Ma, A., and Lipsky, P. (2002). Cytokines and autoimmunity. Nat. Rev. Immunol. 2, 37–45.
Rao, A., Luo, C., and Hogan, P. G. (1997). Transcription factors of the NFAT family: regulation and function. Annu. Rev. Immunol. 15, 707–747.
Rogers, N. C., Slack, E. C., Edwards, A. D., Nolte, M. A., Schulz, O., Schweighoffer, E., Williams, D. L., Gordon, S., Tybulewicz, V. L., Brown, G. D., and Reis e Sousa, C. (2005). Syk-dependent cytokine induction by dectin-1 reveals a novel pattern recognition pathway for C type lectins. Immunity 22, 507–517.
Sancho, D., and Reis e Sousa, C. (2012). Signaling by myeloid C-type lectin receptors in immunity and homeostasis. Annu. Rev. Immunol. 30, 491–529.
Slack, E. C., Robinson, M. J., Hernanz-Falcon, P., Brown, G. D., Williams, D. L., Schweighoffer, E., Tybulewicz, V. L., and Reis e Sousa, C. (2007). Syk-dependent ERK activation regulates IL-2 and IL-10 production by DC stimulated with zymosan. Eur. J. Immunol. 37, 1600–1612.
Stonier, S. W., and Schluns, K. S. (2010). Trans-presentation: a novel mechanism regulating IL-15 delivery and responses. Immunol. Lett. 127, 85–92.
Wuest, S. C., Edwan, J. H., Martin, J. F., Han, S., Perry, J. S., Cartagena, C. M., Matsuura, E., Maric, D., Waldmann, T. A., and Bielekova, B. (2011). A role for interleukin-2 trans-presentation in dendritic cell-mediated T cell activation in humans, as revealed by daclizumab therapy. Nat. Med. 17, 604–609.
Yamazaki, S., Bonito, A. J., Spisek, R., Dhodapkar, M., Inaba, K., and Steinman, R. M. (2007). Dendritic cells are specialized accessory cells along with TGF- for the differentiation of Foxp3+ CD4+ regulatory T cells from peripheral Foxp3 precursors. Blood 110, 4293–4302.
Zanoni, I., Foti, M., Ricciardi-Castagnoli, P., and Granucci, F. (2005). TLR-dependent activation stimuli associated with Th1 responses confer NK cell stimulatory capacity to mouse dendritic cells. J. Immunol. 175, 286–292.
Zanoni, I., Ostuni, R., Capuano, G., Collini, M., Caccia, M., Ronchi, A. E., Rocchetti, M., Mingozzi, F., Foti, M., Chirico, G., Costa, B., Zaza, A., Ricciardi-Castagnoli, P., and Granucci, F. (2009). CD14 regulates the dendritic cell life cycle after LPS exposure through NFAT activation. Nature 460, 264–268.
Keywords: IL-2, dendritic cell, LPS, dectin-1, calcineurin, NFAT
Citation: Zelante T, Fric J, Wong AYW and Ricciardi-Castagnoli P (2012) Interleukin-2 production by dendritic cells and its immuno-regulatory functions. Front. Immun. 3:161. doi: 10.3389/fimmu.2012.00161
Received: 02 March 2012; Paper pending published: 05 April 2012;
Accepted: 30 May 2012; Published online: 18 June 2012.
Edited by:
Francesca Granucci, University of Milano-Bicocca, ItalyReviewed by:
Thomas Malek, University of Miami, USADiana Dudziak, University Hospital of Erlangen, Germany
Irmgard Förster, University of Bonn, Germany
Copyright: © 2012 Zelante, Fric, Wong and Ricciardi-Castagnoli. This is an open-access article distributed under the terms of the Creative Commons Attribution Non Commercial License, which permits non-commercial use, distribution, and reproduction in other forums, provided the original authors and source are credited.
*Correspondence: Paola Ricciardi-Castagnoli, Singapore Immunology Network, Agency for Science, Technology and Research, 8A Biomedical Grove, Immunos Building, Biopolis, Singapore 138648. e-mail:cGFvbGFfY2FzdGFnbm9saUBpbW11bm9sLmEtc3Rhci5lZHUuc2c=