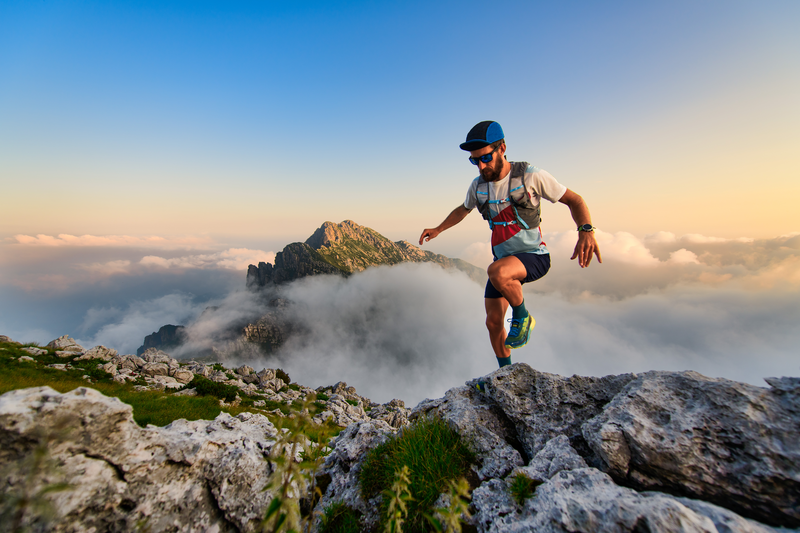
95% of researchers rate our articles as excellent or good
Learn more about the work of our research integrity team to safeguard the quality of each article we publish.
Find out more
BRIEF RESEARCH REPORT article
Front. Hum. Neurosci. , 08 August 2024
Sec. Cognitive Neuroscience
Volume 18 - 2024 | https://doi.org/10.3389/fnhum.2024.1421230
This article is part of the Research Topic Women in Brain Imaging and Stimulation Volume II: 2024 View all 5 articles
Purpose: Attention, a complex cognitive process, is linked to the functional activities of the brain’s dorsal attention network (DAN) and default network (DN). This study aimed to investigate the feasibility, safety, and blinding efficacy of a transcranial direct current stimulation (tDCS) paradigm designed to increase the excitability of the DAN while inhibiting the DN (DAN+/DN-tDCS) on attention function in healthy young adults.
Methods: In this randomized controlled experiment, participants were assigned to either the DAN+/DN-tDCS group or the sham group. A single intervention session was conducted at a total intensity of 4 mA for 20 min. Participants completed the Attention Network Test (ANT) immediately before and after stimulation. Blinding efficacy and adverse effects were assessed post-stimulation.
Results: Forty participants completed the study, with 20 in each group. Paired-sample t-test showed a significant post-stimulation improvement in executive effect performance (t = 2.245; p = 0.037) in the DAN+/DN-tDCS group. The sham group did not exhibit any significant differences in ANT performance. Participants identified the stimulation type with 52.50% accuracy, indicating no difference in blinding efficacy between groups (p = 0.241). Mild-to-moderate adverse effects, such as stinging, itching, and skin reddening, were reported in the DAN+/DN-tDCS group (p < 0.05).
Conclusion: DAN+/DN-tDCS enhanced attention function in healthy young individuals, particularly in improving executive effect performance. This study presents novel strategies for enhancing attentional performance and encourages further investigation into the mechanisms and outcomes of these interventions across diverse populations.
Attention is a critical component of human cognitive functions, involving the collection filtering, selecting, and processing of information from the external world, allowing us to focus on the most relevant and useful details (Buschman and Miller, 2007). Attention processes are supported by complex neural networks that interconnect and synchronize the activity of multiple brain regions, including the dorsal attention network (DAN) and the default network (DN) (Xuan et al., 2016; Zhao et al., 2021). The DAN exhibits heightened activity during tasks requiring external attention, facilitating the optimal allocation of cognitive resources and the prolonged maintenance of attention (Esterman et al., 2014; Dixon et al., 2017). Conversely, the DN, known as a task-negative correlation network, is typically suppressed during attention-demanding tasks but becomes more active during internal orientation and self-referential processes (Boord et al., 2017). The negatively correlated connectivity between the DAN and DN reflects the brain’s ability to switch between external attention and internally focused thought processes (Lo et al., 2021). This dynamic interconnectivity is essential for effectively allocating and maintaining attention (Esterman et al., 2014). Research has demonstrated that the stronger negative correlation between DAN and DN is related to highly productive cognitions (Kelly et al., 2008; Xu et al., 2015; Parkinson et al., 2019). Therefore, we hypothesized that enhancing the excitability of the DAN while concurrently suppressing the DN to coordinate the brain functional activities may effectively modulate individual attention function.
Transcranial direct current stimulation (tDCS) is a non-invasive and well-tolerated brain stimulation technique that induces significant changes in neuronal excitability by applying low-intensity (0.1 to 2.0 mA) anodal or cathodal stimulation to a target area (Paulus, 2003; Bikson et al., 2016). tDCS can modulate the excitability of the cerebral cortex, thereby enhancing the corresponding cortical motor or cognitive functions (Nitsche and Paulus, 2000; Trudgen et al., 2019). However, meta-analyses have concluded that there is insufficient evidence to assert that traditional tDCS significantly enhances attention (Majdi et al., 2022; Saleh et al., 2023). Previous research has applied traditional tDCS to target single brain regions, including the left dorsolateral prefrontal cortex (DLPFC) (Miler et al., 2018), the right posterior parietal cortex (PPC) (Lo et al., 2019) and the right prefrontal cortex (Coffman et al., 2014). These studies suggest that while traditional tDCS may improve attention to some extent, its effects on different aspects of attention are inconsistent. One explanation is that traditional tDCS, which focuses on a single target region, may not sufficiently enhance brain functional activities (Roy et al., 2015; Miler et al., 2018; Lu et al., 2020; Nawani et al., 2023). During attention processes, brain regions do not operate in isolation; rather, attention relies on the integration and collaboration of large-scale brain networks (Hutchison et al., 2013; Fiebelkorn and Kastner, 2020).
To address the need for brain network modulation, researchers have proposed a network-based tDCS model that combines specific algorithms to determine the optimal cortical electric fields and a set of optimized stimulation parameters. This technique utilizes simultaneous or coordinated stimulation of multiple task-related brain regions to alter excitability, enhancing connectivity in key cortical regions and amplifying the stimulation effect (Ruffini et al., 2014; Fischer et al., 2017; Mencarelli et al., 2020). Compared to traditional tDCS, network-based tDCS has been shown to have superior excitatory and inhibitory effects on brain networks (Mencarelli et al., 2020; Gregoret et al., 2023). For example, Zhou et al. (2022) demonstrated that network-based tDCS significantly reduced gait variability in healthy adults using a protocol known as DAN+/DN-tDCS, which is designed to simultaneously enhance DAN excitability while inhibiting DN excitability. While this approach theoretically offers potential benefits, its effects on attention function have not yet been clearly established.
Therefore, this randomized controlled trial aimed to investigate the feasibility and safety of the DAN+/DN-tDCS on attention function in healthy young adults. We hypothesized that this novel tDCS technique, designed to enhance coordination between the DAN and DN brain networks, would improve attention function. Additionally, we anticipated that this stimulation protocol would be safe without severe adverse effects.
This study recruited healthy young adults to assess the comfort and adverse effects associated with the stimulation protocols, which can offer better application to clinical populations. Participants had to be right-handed, as determined by the Edinburgh Handedness Inventory (Oldfield, 1971), possess normal or corrected vision, and have no history of neurological or psychological problems.
Exclusion criteria included: 1) hospitalization in the previous 6 months, 2) use of medications affecting brain states through central nervous system activity and neural activation, 3) severe neurological disorders (such as Parkinson’s disease and stroke), musculoskeletal conditions leading to gait impairment, or cerebrovascular and cardiovascular illnesses, 4) cognitive impairment (Mini-Mental Status Exam ≤24) (Arevalo-Rodriguez et al., 2021), and 5) any contraindication to tDCS stimulation (intracerebral metal implants) (Zhou et al., 2014).
All participants were required to have a basic understanding of network-based tDCS, comprehend the study objectives and procedures, and signed a written informed consent before testing. The study received ethical approval from the Ethics Committee of Shanghai University of Sports (102772020RT109).
Each enrolled participant attended the laboratory once for the experiment. Prior to the intervention, they underwent an Attention Network Test (ANT). Participants were randomly assigned to either the DAN+/DN-tDCS group or the sham group. Each group received a single 20-min intervention. Immediately after the intervention, participants completed another ANT and were provided a blinding efficacy and adverse event questionnaire (Figure 1). To minimize potential influences on attention function, participants refrained from intense physical activity for 24 h and from consuming caffeine-containing beverages for 4 h before the test.
Figure 1. Experimental protocol. Participants performed the Attention Network Test (ANT) before and after DAN+/DN-tDCS or sham stimulation. DAN, dorsal attention network; DN, default network; tDCS, transcranial direct current stimulation.
This experiment employed the Neuroelectrics Stimweaver® platform to implement the DAN+/DN-tDCS and sham stimulation protocols. For this purpose, a 3.14 cm2 Ag/AgCl gel electrode was positioned within a neoprene cap at a location specified by the International 10–20 electroencephalography system (Ulate-Campos and Loddenkemper, 2024). The DAN+/DN-tDCS protocol involved placing seven electrodes on key regions of the DAN and DN based on the international 10–20 EEG system. Anode electrodes were situated at F7, CP1, and CP2, while cathode electrodes were placed at FPZ, FZ, CP5, and AF3. This arrangement aimed to excite the DAN and inhibit the DN, thereby modulating these networks (Figure 2) (Zhou et al., 2022). Active tDCS parameters included a peak current of 1999 μA per electrode, culminating in a total current of 3,999 μA across the seven electrodes, with a 20-min stimulation duration that included 30-s ramp-up and ramp-down periods. Sham stimulation used the same electrode positions and current intensity as the DAN+/DN-tDCS, with current applied only during the first and last 30 s to simulate the sensation of real stimulation. The tDCS device was operated by personnel proficient in its use but not involved in the study, ensuring that neither the participants nor researchers knew the type of stimulation. After the intervention, participants completed the adverse event questionnaire to assess potential adverse effects (Woods et al., 2016), and they were asked to predict whether they received real or sham stimulation, to assess the blinding efficacy.
Figure 2. DAN+/DN-tDCS intervention, stimulation targets, and electric field modeling. (A) the electrode placement for the DAN+/DN-tDCS protocol, with red circles indicating anodes and blue circles indicating cathodes; (B) the red and blue parts represent the areas where the DAN and DN are located, respectively; (C) simulation of the electric field for the DAN+/DN-tDCS protocol and the color represents the current intensity; darker color indicates a greater current. DAN, dorsal attention network; DN, default network; tDCS, transcranial direct current stimulation.
The ANT (Fan et al., 2002)1 was obtained from the Sackler Institute for Developmental Psychobiology and created using Java software. The test typically consists of a 24-trial practice with feedback and three 96-trial experimental blocks with no feedback. To enhance experiment efficiency and prevent mental fatigue, participants completed a practice and two experimental blocks, each lasting 6 min, with a 1-min rest between the two blocks. The test required participants to sit in front of a computer monitor and focus on a fixation cross (indicated by a cue and a target). When a target arrow appeared above or below the center fixation cross, they were required to provide a response as quickly as possible by pressing buttons on the keyboard corresponding to the direction of the target arrow (left or right) (Pauletti et al., 2017; De Souza Almeida et al., 2021). The ANT program recorded participants’ cognitive metrics automatically. Reaction times were measured to calculate the efficiency of the alerting, orienting, and executive networks using different conditions in each trial. The recorded values of two experimental blocks were averaged to determine the influence of the three attention network effects. The alerting effect refers to the ability to maintain vigilance, calculated as the difference in reaction time between no-cue and double-cue conditions. The orienting effect reflects the ability to select spatial information, calculated as the difference in reaction time between central-cue and spatial-cue conditions. The executive effect refers to the ability to deal with conflicting situations, calculated as the difference in reaction time between incongruent and congruent conditions (Posner and Petersen, 1990; Fan et al., 2002). Larger values of the alerting or orienting effect indicate stronger effects, demonstrating better performance in response to relevant cues. A lower value of the executive effect indicates better performance, reflecting quicker responses to conflict situations (Roy et al., 2015; Pauletti et al., 2017).
The primary outcome indicators of the study included the alerting, orienting, and executive effects. Additionally, reaction times for the six conditions and average accuracy for the entire ANT task were recorded. These conditions included no-cue, center-cue, double-cue, spatial-cue, incongruent, and congruent conditions.
To evaluate normal distribution, the data underwent the Shapiro–Wilk test. Non-normally distributed data were presented as the median (quartiles) using the non-parametric test, while normally distributed data were presented as the mean ± standard error using the t-test. Independent-sample t-tests were employed to evaluate differences in basic information between the two groups. To examine the effects of DAN+/DN-tDCS on ANT, independent samples t-tests were utilized to compare the absolute changes before and after stimulation to quantify between-group differences. Paired samples t-tests were used to compare within-group differences of the metrics. Furthermore, Fisher’s exact test and the Kruskal-Wallis test were employed to assess differences in blinding efficacy and adverse effect between the DAN+/DN-tDCS and sham groups. The significance threshold α was established at 0.05, with effect magnitudes quantified in terms of Cohen’s d. Statistical analyses were performed using SPSS version 27.0 (IBM Corp., Armonk, NY, United States).
The study enrolled 40 participants, with 20 individuals in each group. All participants completed the experimental intervention and questionnaire (Table 1). Before the intervention, independent-sample t-tests revealed no significant differences in the baseline characteristics between the two groups (p > 0.05).
Paired-sample t-tests indicated that DAN+/DN-tDCS significantly enhanced executive effect performance (t = 2.245, p = 0.037, Cohen’s d = 0.502) and yielded marginally significant changes in orienting effect (t = −0.861, p = 0.078, Cohen’s d = −0.416). However, the three attention network effects showed no significant defferences in the sham group (p > 0.388) (Figure 3). Additionally, compared to that before stimulation, the DAN+/DN-tDCS group demonstrated a significant reduction in reaction times for the double cue, center cue, spatial cue, congruent cue, and incongruent cue conditions after stimulation (p < 0.05). The sham group showed significantly lower reaction times only for the spatial cue condition (p = 0.010) (Table 2). Furthermore, the average accuracy for judging target arrow direction in the ANT test significantly improved in the DAN+/DN-tDCS group after stimulation (t = −2.965; p = 0.008), while no significant changes were observed in the sham group.
Figure 3. DAN+/DN-tDCS on alerting, orientastion, and executive effects. DAN+/DN-tDCS: excitatory frontoparietal network + inhibitory default network; sham: sham stimulus; pre: pre-test; post: post-test; *indicates a significant difference within the group. DAN, dorsal attention network; DN, default network; tDCS, transcranial direct current stimulation.
Independent samples t-tests indicated no significant differences between the DAN+/DN-tDCS group and the sham group for the absolute changes in alerting, orienting, and executive effects (0.162 < p < 0.872). However, compared to the sham group, the DAN+/DN-tDCS group showed greater improvements in all three effects, with change values in orienting being 6.89 (Cohen’s d = −0.450) and executive effect being 9.81 (Cohen’s d = 0.451) (Table 3).
Table 3. The change values of network-based tDCS effects on alerting, orienting, and executive effect.
Participants correctly guessed the type of stimulation with an accuracy rate of 52.50%; Fisher’s test showed p = 0.241, indicating a good blinding efficacy. The adverse event questionnaire revealed that the vast majority of all participants did not report severe discomfort during the experiment. However, significant differences were observed between the two groups in the severity of sensations such as stinging, itching, and skin reddening (p < 0.05) (Table 4). One participant in the DAN+/DN-tDCS group reported more severe itching, skin reddening, and fatigue (Table 4).
This study aimed to explore the effects of network-based tDCS on attention function in healthy young adults. The results showed a significant reduction in reaction time on the ANT test and a significant enhancement in the executive network effect after DAN+/DN-tDCS. These findings suggested that the executive effect of attention function may be related to the coordinated activity between the DAN and the DN.
In this study, the DAN+/DN-tDCS protocol significantly enhanced executive effect in healthy adults. The ANT combines the cued reaction time test (Posner and Petersen, 1990) and the flanker task (Eriksen and Eriksen, 1974), measuring the efficiency of three subsystems of attention: alerting, orienting, and executive effect. The improvement in executive effect aligns with previous research findings, where the executive effect monitors and resolves task conflicts, such as error detection, decision-making, and planning (Fan et al., 2002; Callejas et al., 2005). The frontoparietal control network (FPCN) plays a crucial role in executive control, particularly in conflict resolution and task-switching processes (Zhao et al., 2019). Within the FPCN, there are two distinct subsystems with different functional connectivity patterns. FPCNA exhibits stronger connectivity with the DN than the DAN, while FPCNB shows the opposite pattern (Dixon, 2015; Spreng et al., 2016; Dixon et al., 2017). The DAN+/DN-tDCS protocol likely regulated the dynamic balance between the DN and DAN by flexibly coupling the FPCN with the connection patterns of the DN and DAN, thereby driving internal or external cognitive orientation. This flexible coupling may have enhanced the executive effect by optimizing the network’s ability to manage task conflict and improve performance on the ANT in healthy adults (Dixon et al., 2018).
Although the DAN+/DN-tDCS intervention did not significantly enhance the orienting effect, there was a trend of improvement (t = −0.861; p = 0.078), suggesting some modulatory efficiency on orienting effect. However, no significant difference was observed in the alerting effect after DAN+/DN-tDCS. Previous studies have reported that traditional tDCS targeting the right PPC significantly enhanced the orienting effect (Yin et al., 2012), indicating the positive role of the right PPC in orienting attention function. Yin et al. (2012) also found that the alerting effect was primarily linked to the right thalamus and supplementary motor regions, while the orienting effect was significantly correlated with the right parietal cortex, particularly the inferior parietal areas (Lo et al., 2019). Studies implicated that the alerting network may be associated with subcortical structures (the brainstem locus ceruleus) and circuits involving the right parietal and prefrontal cortex (Posner, 2008, 2012; Petersen and Posner, 2012). The DAN includes bilateral inferior parietal sulci, superior parietal lobules, and frontal eye fields, while the DN comprises the medial prefrontal cortex, precuneus, posterior cingulate cortex, and lateral parietal regions (Eryurek et al., 2022). Consequently, the activity of the DAN and the DN may not be the key neural mechanisms underlying the control of the alerting and orienting networks.
We observed a significant enhancement in average accuracy on the ANT task after DAN+/DN-tDCS. This finding may be associated with a learning effect induced by the repetitive nature of the ANT test the relative simplicity of the test tasks. Therefore, the significant improvement in average accuracy should be considered cautiously. Additionally, the timing of stimulation intervention and ANT test was self-determined by participants, which may have influenced their task performance.
This study has several limitations. First, the subjective judgment of electrical stimulation may significantly influence the results. During stimulation, participants may have subjectively assessed their received stimulation type, and their perceptions of skin sensations might enhance the placebo effect, improving performance in subsequent trials. Future studies should aim to optimize the stimulation protocol for the sham group, ensuring that both DAN+/DN-tDCS and sham stimulations elicit equivalent or similar somatosensory perceptions, thereby minimizing subjective biases among participants. Furthermore, this study employed a novel DAN+/DN-tDCS protocol targeting brain networks for modulation as our intervention approach. However, we did not incorporate a traditional tDCS protocol as a control to assess the disparities between the two methods. Consequently, we were unable to evaluate the superiority of the innovative network tDCS utilized in this investigation for modulating attention function. Lastly, the study only explored the immediate post-intervention effects of DAN+/DN-tDCS on attention function in healthy adults; further research is required to explore the long-term intervention or cumulative effects of this novel stimulation protocol and its potential for application in clinical populations.
The DAN+/DN-tDCS protocol may enhance attention function in healthy young adults, particularly showing improvement in executive effect. This study offers a novel intervention strategy for improving attention function based on brain network modulation. Attention function may rely on the functional activities of disparate brain networks. Therefore, establishing the relationships between different attention network effects and their corresponding brain networks in a rational and targeted manner is crucial for the effectiveness of stimulation protocols. Future studies should further explore the intervention effects of network-based tDCS on attention function in different populations and elucidate the potential underlying mechanisms to facilitate its widespread application in clinical rehabilitation.
The original contributions presented in the study are included in the article/supplementary material, further inquiries can be directed to the corresponding author.
The studies involving humans were approved by Institutional Review Board of the Shanghai University of Sports (102772020RT109). The studies were conducted in accordance with the local legislation and institutional requirements. The participants provided their written informed consent to participate in this study. Written informed consent was obtained from the individual(s) for the publication of any potentially identifiable images or data included in this article.
XW: Writing – original draft, Writing – review & editing, Formal analysis, Validation. RZ: Data curation, Investigation, Writing – original draft. SZ: Data curation, Methodology, Software, Writing – original draft. YZ: Data curation, Investigation, Software, Supervision, Writing – original draft. XF: Data curation, Methodology, Writing – original draft. JL: Funding acquisition, Project administration, Resources, Supervision, Writing – original draft, Writing – review & editing.
The author(s) declare that financial support was received for the research, authorship, and/or publication of this article. This research received funding from the National Natural Science Foundation of China (grant numbers 12302418 and 11932013) as well as the Sports Science & Technology Project of Shanghai (grant number 24J013).
The authors express their gratitude to all the study participants. Additionally, we are thankful to On-Yee Lo (Harvard Medical School, Harvard University, Boston, MA, United States) for her help in the process of design stimulation protocol, and to Editage (www.editage.cn) for their support with English language editing.
The authors declare that the research was conducted in the absence of any commercial or financial relationships that could be construed as a potential conflict of interest.
All claims expressed in this article are solely those of the authors and do not necessarily represent those of their affiliated organizations, or those of the publisher, the editors and the reviewers. Any product that may be evaluated in this article, or claim that may be made by its manufacturer, is not guaranteed or endorsed by the publisher.
Arevalo-Rodriguez, I., Smailagic, N., Roqué-Figuls, M., Ciapponi, A., Sanchez-Perez, E., Giannakou, A., et al. (2021). Mini-mental state examination (Mmse) for the early detection of dementia in people with mild cognitive impairment (mci). Cochrane Database Syst. Rev. 7:Cd010783. doi: 10.1002/14651858.CD010783.pub3
Bikson, M., Grossman, P., Thomas, C., Zannou, A. L., Jiang, J., Adnan, T., et al. (2016). Safety of transcranial direct current stimulation: evidence based update 2016. Brain Stimul. 9, 641–661. doi: 10.1016/j.brs.2016.06.004
Boord, P., Madhyastha, T. M., Askren, M. K., and Grabowski, T. J. (2017). Executive attention networks show altered relationship with default mode network in Pd. Neuroimage Clin. 13, 1–8. doi: 10.1016/j.nicl.2016.11.004
Buschman, T. J., and Miller, E. K. (2007). Top-down versus bottom-up control of attention in the prefrontal and posterior parietal cortices. Science 315, 1860–1862. doi: 10.1126/science.1138071
Callejas, A., Lupiàñez, J., Funes, M. J., and Tudela, P. (2005). Modulations among the alerting, orienting and executive control networks. Exp. Brain Res. 167, 27–37. doi: 10.1007/s00221-005-2365-z
Coffman, B. A., Clark, V. P., and Parasuraman, R. (2014). Battery powered thought: enhancement of attention, learning, and memory in healthy adults using transcranial direct current stimulation. NeuroImage 85, 895–908. doi: 10.1016/j.neuroimage.2013.07.083
De Souza Almeida, R., Faria-Jr, A., and Klein, R. M. (2021). On the origins and evolution of the attention network tests. Neurosci. Biobehav. Rev. 126, 560–572. doi: 10.1016/j.neubiorev.2021.02.028
Dixon, M. L. (2015). Cognitive control, emotional value, and the lateral prefrontal cortex. Front. Psychol. 6:758. doi: 10.3389/fpsyg.2015.00758
Dixon, M. L., Andrews-Hanna, J. R., Spreng, R. N., Irving, Z. C., Mills, C., Girn, M., et al. (2017). Interactions between the default network and dorsal attention network vary across default subsystems, time, and cognitive states. NeuroImage 147, 632–649. doi: 10.1016/j.neuroimage.2016.12.073
Dixon, M. L., De La Vega, A., Mills, C., Andrews-Hanna, J., Spreng, R. N., Cole, M. W., et al. (2018). Heterogeneity within the frontoparietal control network and its relationship to the default and dorsal attention networks. Proc. Natl. Acad. Sci. USA 115, E1598–e1607. doi: 10.1073/pnas.1715766115
Eriksen, B. A., and Eriksen, C. W. (1974). Effects of noise letters upon the identification of a target letter in a nonsearch task. New York City, NY: Psychonomic Society.
Eryurek, K., Ulasoglu-Yildiz, C., Matur, Z., Öge, A. E., Gürvit, H., and Demiralp, T. (2022). Default mode and dorsal attention network involvement in visually guided motor sequence learning. Cortex 146, 89–105. doi: 10.1016/j.cortex.2021.10.006
Esterman, M., Rosenberg, M. D., and Noonan, S. K. (2014). Intrinsic fluctuations in sustained attention and distractor processing. J. Neurosci. 34, 1724–1730. doi: 10.1523/JNEUROSCI.2658-13.2014
Fan, J., Mccandliss, B. D., Sommer, T., Raz, A., and Posner, M. I. (2002). Testing the efficiency and independence of attentional networks. J. Cogn. Neurosci. 14, 340–347. doi: 10.1162/089892902317361886
Fiebelkorn, I. C., and Kastner, S. (2020). Functional specialization in the attention network. Annu. Rev. Psychol. 71, 221–249. doi: 10.1146/annurev-psych-010418-103429
Fischer, D. B., Fried, P. J., Ruffini, G., Ripolles, O., Salvador, R., Banus, J., et al. (2017). Multifocal tdcs targeting the resting state motor network increases cortical excitability beyond traditional tdcs targeting unilateral motor cortex. NeuroImage 157, 34–44. doi: 10.1016/j.neuroimage.2017.05.060
Gregoret, L., Zamorano, A. M., and Graven-Nielsen, T. (2023). Multifocal tdcs targeting the motor network modulates event-related cortical responses during prolonged pain. J. Pain 24, 226–236. doi: 10.1016/j.jpain.2022.09.010
Hutchison, R. M., Womelsdorf, T., Gati, J. S., Everling, S., and Menon, R. S. (2013). Resting-state networks show dynamic functional connectivity in awake humans and anesthetized macaques. Hum. Brain Mapp. 34, 2154–2177. doi: 10.1002/hbm.22058
Kelly, A. M., Uddin, L. Q., Biswal, B. B., Castellanos, F. X., and Milham, M. P. (2008). Competition between functional brain networks mediates behavioral variability. NeuroImage 39, 527–537. doi: 10.1016/j.neuroimage.2007.08.008
Lo, O. Y., Halko, M. A., Devaney, K. J., Wayne, P. M., Lipsitz, L. A., and Manor, B. (2021). Gait variability is associated with the strength of functional connectivity between the default and dorsal attention brain networks: evidence from multiple cohorts. J. Gerontol. A Biol. Sci. Med. Sci. 76, e328–e334. doi: 10.1093/gerona/glab200
Lo, O.-Y., Van Donkelaar, P., and Chou, L.-S. (2019). Effects of transcranial direct current stimulation over right posterior parietal cortex on attention function in healthy young adults. Eur. J. Neurosci. 49, 1623–1631. doi: 10.1111/ejn.14349
Lu, H., Liu, Q., Guo, Z., Zhou, G., Zhang, Y., Zhu, X., et al. (2020). Modulation of repeated anodal Hd-tdcs on attention in healthy young adults. Front. Psychol. :11:564447. doi: 10.3389/fpsyg.2020.564447
Majdi, A., Van Boekholdt, L., Sadigh-Eteghad, S., and Mc Laughlin, M. (2022). A systematic review and meta-analysis of transcranial direct-current stimulation effects on cognitive function in patients with Alzheimer's disease. Mol. Psychiatry 27, 2000–2009. doi: 10.1038/s41380-022-01444-7
Mencarelli, L., Menardi, A., Neri, F., Monti, L., Ruffini, G., Salvador, R., et al. (2020). Impact of network-targeted multichannel transcranial direct current stimulation on intrinsic and network-to-network functional connectivity. J. Neurosci. Res. 98, 1843–1856. doi: 10.1002/jnr.24690
Miler, J. A., Meron, D., Baldwin, D. S., and Garner, M. (2018). The effect of prefrontal transcranial direct current stimulation on attention network function in healthy volunteers. Neuromodulation Technol. Neural Interface 21, 355–361. doi: 10.1111/ner.12629
Nawani, H., Mittner, M., and Csifcsák, G. (2023). Modulation of mind wandering using transcranial direct current stimulation: a meta-analysis based on electric field modeling. NeuroImage 272:120051. doi: 10.1016/j.neuroimage.2023.120051
Nitsche, M. A., and Paulus, W. (2000). Excitability changes induced in the human motor cortex by weak transcranial direct current stimulation. J. Physiol. 527, 633–639. doi: 10.1111/j.1469-7793.2000.t01-1-00633.x
Oldfield, R. C. (1971). The assessment and analysis of handedness: the Edinburgh inventory. Neuropsychologia 9, 97–113. doi: 10.1016/0028-3932(71)90067-4
Parkinson, T. D., Kornelsen, J., and Smith, S. D. (2019). Trait mindfulness and functional connectivity in cognitive and attentional resting state networks. Front. Hum. Neurosci. 13:112. doi: 10.3389/fnhum.2019.00112
Pauletti, C., Mannarelli, D., Locuratolo, N., Pollini, L., Currà, A., Marinelli, L., et al. (2017). Attention in Parkinson's disease with fatigue: evidence from the attention network test. J. Neural Transm. (Vienna) 124, 335–345. doi: 10.1007/s00702-016-1637-z
Paulus, W. (2003). Transcranial direct current stimulation (tdcs). Suppl. Clin. Neurophysiol. 56, 249–254. doi: 10.1016/S1567-424X(09)70229-6
Petersen, S. E., and Posner, M. I. (2012). The attention system of the human brain: 20 years after. Annu. Rev. Neurosci. 35, 73–89. doi: 10.1146/annurev-neuro-062111-150525
Posner, M. I. (2008). Measuring alertness. Ann. N. Y. Acad. Sci. 1129, 193–199. doi: 10.1196/annals.1417.011
Posner, M. I. (2012). Imaging attention networks. NeuroImage 61, 450–456. doi: 10.1016/j.neuroimage.2011.12.040
Posner, M. I., and Petersen, S. E. (1990). The attention system of the human brain. Annu. Rev. Neurosci. 13, 25–42. doi: 10.1146/annurev.ne.13.030190.000325
Roy, L. B., Sparing, R., Fink, G. R., and Hesse, M. D. (2015). Modulation of attention functions by anodal tdcs on right Ppc. Neuropsychologia 74, 96–107. doi: 10.1016/j.neuropsychologia.2015.02.028
Ruffini, G., Fox, M. D., Ripolles, O., Miranda, P. C., and Pascual-Leone, A. (2014). Optimization of multifocal transcranial current stimulation for weighted cortical pattern targeting from realistic modeling of electric fields. NeuroImage 89, 216–225. doi: 10.1016/j.neuroimage.2013.12.002
Saleh, O., Assaf, M., Alzoubi, A., Anshase, A., Tarkhan, H., Ayoub, M., et al. (2023). The effects of transcranial direct current stimulation on cognitive function for mild cognitive impairment: a systematic review and meta-analysis of randomized controlled trials. Aging Clin. Exp. Res. 35, 2293–2306. doi: 10.1007/s40520-023-02528-2
Spreng, R. N., Stevens, W. D., Viviano, J. D., and Schacter, D. L. (2016). Attenuated anticorrelation between the default and dorsal attention networks with aging: evidence from task and rest. Neurobiol. Aging 45, 149–160. doi: 10.1016/j.neurobiolaging.2016.05.020
Trudgen, A., Cirillo, J., and Byblow, W. D. (2019). Somatosensory and transcranial direct current stimulation effects on manual dexterity and motor cortex function: a metaplasticity study. Brain Stimul. 12, 938–947. doi: 10.1016/j.brs.2019.02.010
Ulate-Campos, A., and Loddenkemper, T. (2024). Review on the current long-term, limited lead electroencephalograms. Epilepsy Behav. 150:109557. doi: 10.1016/j.yebeh.2023.109557
Woods, A. J., Antal, A., Bikson, M., Boggio, P. S., Brunoni, A. R., Celnik, P., et al. (2016). A technical guide to tdcs, and related non-invasive brain stimulation tools. Clin. Neurophysiol. 127, 1031–1048. doi: 10.1016/j.clinph.2015.11.012
Xu, J., Yin, X., Ge, H., Han, Y., Pang, Z., Tang, Y., et al. (2015). Attentional performance is correlated with the local regional efficiency of intrinsic brain networks. Front. Behav. Neurosci. 9:200. doi: 10.3389/fnbeh.2015.00200
Xuan, B., Mackie, M. A., Spagna, A., Wu, T., Tian, Y., Hof, P. R., et al. (2016). The activation of interactive attentional networks. NeuroImage 129, 308–319. doi: 10.1016/j.neuroimage.2016.01.017
Yin, X., Zhao, L., Xu, J., Evans, A. C., Fan, L., Ge, H., et al. (2012). Anatomical substrates of the alerting, orienting and executive control components of attention: focus on the posterior parietal lobe. PLoS One 7:e50590. doi: 10.1371/journal.pone.0050590
Zhao, Q., Sang, X., Metmer, H., Swati, Z. N. N. K., and Lu, J. (2019). Functional segregation of executive control network and frontoparietal network in Alzheimer's disease. Cortex 120, 36–48. doi: 10.1016/j.cortex.2019.04.026
Zhao, P., Yu, R. S., Liu, Y., Liu, Z. H., Wu, X., Li, R., et al. (2021). The functional hierarchy of the task-positive networks indicates a core control system of top-down regulation in visual attention. J. Integr. Neurosci. 20, 43–53. doi: 10.31083/j.jin.2021.01.297
Zhou, J., Hao, Y., Wang, Y., Jor'dan, A., Pascual-Leone, A., Zhang, J., et al. (2014). Transcranial direct current stimulation reduces the cost of performing a cognitive task on gait and postural control. Eur. J. Neurosci. 39, 1343–1348. doi: 10.1111/ejn.12492
Keywords: transcranial direct current stimulation, brain network modulation, attention, dorsal attention network, default network
Citation: Wei X, Zhou R, Zheng S, Zhang Y, Feng X and Lü J (2024) Network-based transcranial direct current stimulation enhances attention function in healthy young adults: a preliminary study. Front. Hum. Neurosci. 18:1421230. doi: 10.3389/fnhum.2024.1421230
Received: 22 April 2024; Accepted: 30 July 2024;
Published: 08 August 2024.
Edited by:
Wen Li, University of Texas Health Science Center at Houston, United StatesReviewed by:
Bernadette Cortese, Medical University of South Carolina, United StatesCopyright © 2024 Wei, Zhou, Zheng, Zhang, Feng and Lü. This is an open-access article distributed under the terms of the Creative Commons Attribution License (CC BY). The use, distribution or reproduction in other forums is permitted, provided the original author(s) and the copyright owner(s) are credited and that the original publication in this journal is cited, in accordance with accepted academic practice. No use, distribution or reproduction is permitted which does not comply with these terms.
*Correspondence: Jiaojiao Lü, bGpqMjdAMTYzLmNvbQ==
Disclaimer: All claims expressed in this article are solely those of the authors and do not necessarily represent those of their affiliated organizations, or those of the publisher, the editors and the reviewers. Any product that may be evaluated in this article or claim that may be made by its manufacturer is not guaranteed or endorsed by the publisher.
Research integrity at Frontiers
Learn more about the work of our research integrity team to safeguard the quality of each article we publish.